DOI:
10.1039/D2RA06563K
(Review Article)
RSC Adv., 2022,
12, 35123-35150
Review of photoresponsive and glycoside dendrimers in biomaterials and sensors applications
Received
18th October 2022
, Accepted 1st December 2022
First published on 7th December 2022
Abstract
Dendrimers are branched molecules with well-defined lengths, shapes, molecular weights, and monodispersity in comparison to linear polymers. The dual effect of the chromophore luminescence and the morphology of the synthesized dendrimers has drawn a lot of interest towards the design of dendrimers with different chromophores. Furthermore, the stimulus-responsive systems can sequester drug molecules under a preset set of parameters and release them in a different environment in response to either an exogenous or endogenous stimulus. The addition of photoresponsive moieties to different dendrimer components, such as the core or branches, magnifies the importance of the dendrimer in several related sectors of nanotechnology, such as sensors, photoswitches, electronic gadgets, and drug delivery systems. This review article focused on photoresponsive/glycoside dendrimer structures and their biomaterial applications.
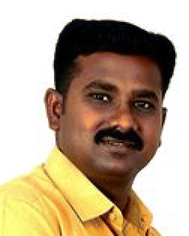 Mani Rajasekar | Dr M. Rajasekar is currently working as Scientist-C in the Centre for Molecular and Nanomedical Sciences, International Research Centre, Sathyabama Institute of Science and Technology (Deemed to be University), Chennai since 2018. He was born (1984) in the village of Agatheripattu, Thiruvannamalai district in Tamil Nadu, India, and his main research interest's in the area of Synthetic Organic Chemistry. He was received his BSc degree from the Department of Chemistry, Arignar Anna Govt. Arts College, Cheyyar, affiliated to the University of Madras in 2005 and MSc Degree from the Department of Chemistry, C. Abdul Hakeem College of Arts and Science, Vellore, affiliated to Thiruvalluvar University in 2007. He received his M.Phil degree from the Department of Chemistry, The New College, affiliated with the University of Madras, Chennai, in 2008. He was worked as a Visiting Lecturer at, the Department of Chemistry, Anna University, Chennai from 26.06.2008 to 30.12.2008, and a PhD degree from the Department of Organic Chemistry, the University of Madras in 2015. He worked as a National Postdoctoral Fellow (NPDF) at Sathyabama Institute of Science and Technology (Deemed to be University), Chennai from 2016–2018 and B.Ed., a degree completed from Tamil Nadu Teachers Education University (TNTEU) in 2021. He was awarded UGC-JRF, UGC-SRF, NET, SLET, SET, NPDF, DSKPDF, Nehru PDF, Young Scientist, and National Educational Star Award by “The Glorious Organization for Accelerated to Literacy (GOAL)”, New Delhi. He served as a doctoral committee member for graduate students, and reviewer for various international journals published by ACS, RSC, Wiley, and Elsevier. He has delivered several invited scientific lectures in various National/International Symposium/Conferences/refresher courses and on other occasions. |
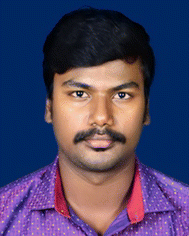 Saravanan Geetha Sree Agash | Sree Agash S. G. was born in Tamil Nadu, India in 1999. He received his master's in Medical Biotechnology and Clinical Research from Sathyabama Institute of Science and Technology (Deemed to be University), Chennai in 2022 and He also received his Bachelors in Medical Biotechnology from Chettinad Academics of Research and Education (Deemed to be University), Chennai in 2020. He has done a project on Molecular Detection of Mycobacterium bovis by using Duplex PCR and also on Studies on the role of antioxidants in oxidative stress-induced neurodegeneration in the zebrafish model. His current research interests are Bio-Organic Chemistry and Medicinal Chemistry. |
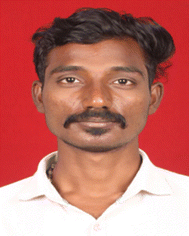 Kumarasan Rajasekar | Rajasekar K. was raised in Kanchipuram district, Tamil Nadu, India in 1998. He received his Master degree in Medical Biotechnology and Clinical Research from Sathyabama Institute of Science and Technology (Deemed to be University), Chennai in 2022 and He also received his Bachelor degree in Microbiology from Kanchi Shri Krishna College of arts and Science, Kanchipuram in 2019. He completed a Diploma in Medical Laboratory Technology from Kanchi Shri Krishna College in 2018. He was done a project on “Unculturable Yeast and Their Characterization form plant Extract” and also on Studies on the role of beta-lactamase Enzyme and Efflux Pump Inhibitors and their Mechanisms. His current research interests are in the Total synthesis of natural products, Organic synthesis, Biosensors, and their biological applications. |
1. Introduction
In recent years, dendritic materials have distinct tree-like structures and exhibit new physical and chemical characteristics. Since Vogtle et al. discovered them in the late 1970s, they have been extensively explored.1 The pioneers in this field of study were the Newkome and Tomalia groups.2–4 These materials have a special structure that includes a core, several active end groups, and well-defined branched functional groups (Fig. 1).5 This structure gives rise to the use of dendrimers. Further, while the polydispersity, molecular weight, and end groups of linear or branched polymers are typically difficult to manage, dendrimers allow for simple control of these variables. Due to their unique structural and functional properties, dendrimers have found widespread scientific and technological use in a variety of fields of study, including the development of magnetic materials, photovoltaic and light-emitting devices, catalysts, and therapeutic drug delivery.6,7
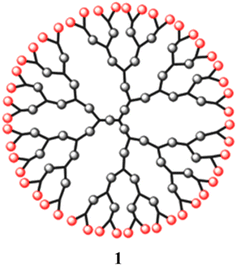 |
| Fig. 1 Structure of dendrimer (1). | |
The development of luminous macromolecules is the consequence of the incorporation of photoactive functions in dendrimer structures.8 These macromolecule chromophores can be carefully positioned in a number of locations, such as the core, the periphery, or even the branching sites, within the dendrimer structure. A mimic of the photosynthetic light-harvesting system, in which antenna chromophores surround the inner reaction centre, is provided by such dendrimeric structures with integrated chromophores.9,10 Because of the near spatial closeness to neighbouring moieties, each photoactive group inside a dendrimer might have substantially different emission and energy-transfer properties compared to those of the same chromophore within small molecules.11
For instance, in properly designed dendrimers, photoexcited chromophores can transfer energy to other units, directing the energy through the antenna framework toward the core, which can be the basis for solar energy conversion, luminescent sensors, and photoinduced electron transfer (PET) between the dendrimer units.12–14 This technique can also produce the important species excimer and exciplex, which have incredibly distinct emission wavelengths within the dendrimer. In order to combine the redox properties of metals with additional dendrimeric properties, metal-ion-containing dendrimers have also been generated.15,16 This review mainly focuses on the structures and biomaterial applications of dendrimer derivatives.
1.1. Overview of structural dendrimers
Dendrimers are one of the most capable materials because of their multiple applications. The structure is divided into three main parts (i) a core, (ii) an internal layer of repeating units or generations, and (iii) an external layer or ends functional group (EFG) of the periphery. They have a nearly flawless 3D structure, mono-dispersed with highly branching dendrons. The synthesis was a step-by-step process and resulted in a generation of dendrimer (Fig. 2).17 It showed the core of the dendritic molecule has low density than the “terminal functional group” (TFG).18 The exclusive structure of well as a large number of TFG with their dendritic structures. It could be applied to achieve estimated properties of dendrimers such as definite shape and internal voids spaces.
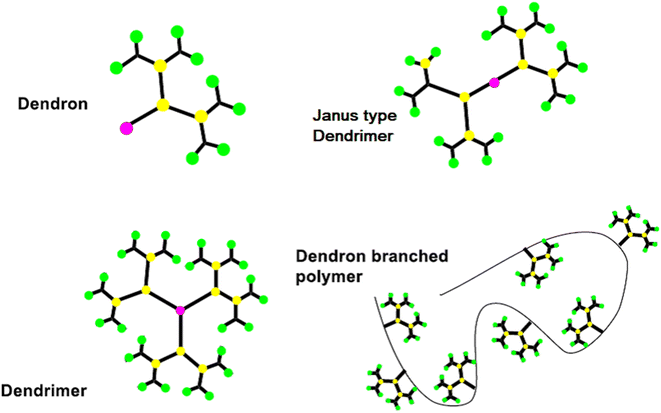 |
| Fig. 2 Different types of dendritic molecules. | |
1.1.1. Core. The central core unit is a multifunctional moiety that gives the focal point to grow the structure and affects the shape of the dendrimer.19 It may cover specific properties/functionality such as chromophores and metal atoms. Its shape, size, functional group, and focal point will surely affect the structure of the dendrimer throughout its synthesis.20
1.1.2. Internal layers or cavities. The internal layers are repeating building blocks or growing functional units that are coupled with the core to yield exponential development in generation and TFG. It helps to increase the generation of dendrimers with their structure advantage.21 Depending on the branching unit of dendrons, the shape of the dendrimer becomes spherical, and the void spaces in nanometres generated by its help for drug binding, encapsulation, metal catalyst, etc.22
1.1.3. Terminal groups or periphery. The terminal functional group (TFG) dendrimer is highly significant for many applications.23 It can change functionality as required for their specific applications. The functional units are developed by increasing branches of the internal layer in a stepwise chemical process according to multiplicity rules. Due to the terminal functional group at the periphery of the dendrimer, it can engage in interactions such as electrostatic interaction, hydrophobic interaction, π–π interaction, and H-bonding interaction. These terminal groups possess many rare properties such as high reactivity, high solubility, low viscosity, interior cavity, etc. They were monodispersing and consist of a densely packed structure.24
1.2. Synthetic process of dendrimers
The synthesis of the dendrimer occurs in a wide variety of methods. In the past, the main areas of research were to materialize the purpose functionality of dendrimer structures, such as POPAM, and PAMAM dendrimers as well as Frechet dendrons.25 Moreover, other dendrimers have not received so much attention from additional groups. It is an important factor to synthesize new materials with familiar synthetic techniques to handy and commercially available dendrimers. To equipment with new functionalities, size, shape, topology, or with particular properties like solubility, aggregation behavior, luminescence, rigidity, back folding, chirality, guest inclusion, gel formation, etc.,26 Recently many synthetic processes of dendrimers were published, yet more detailed research remains to be performed in this aspect. It still needs an organized approach for higher generations of dendrimers and dendrons with targeted functionalization.27
1.2.1. Divergent method. In the divergent method was multifunctional core moiety coupled with stepwise addition of branching units called monomers. It is a type of the ABn (n ≥ 2), where A and B are two different functional groups are shown in Fig. 3.28
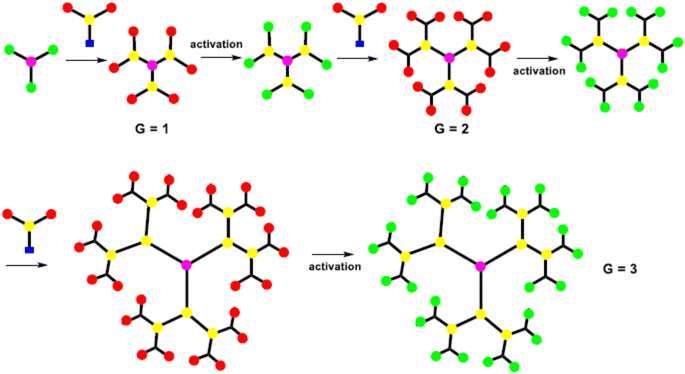 |
| Fig. 3 Structure of dendrimers in divergent approach method. | |
The A-group of the ABn monomer is an active functional group (AFG), while the B-groups are inactive/protected, to increase dendrimer generation. The activation of the B-functional group can be attained by bond formation with another molecule. Bond formation between the A-functional group and the activated B-functional groups of the higher generation was used to couple the monomers to a core. The most frequent monomers are trifunctional or tetrafunctional monomers like AB2 or AB3 compounds. After the multiple additions of these monomers with core, a higher-generation dendrimer is obtained. Tomalia et al. were synthesize dendrimers using the divergent approach were poly(amidoamine) dendrimers (PAMAM).29 PAMAM dendrimers were synthesized via a Michael-type addition of ethylenediamine (EDA) as core and methyl acrylate as branching unit afterward aminolysis of the resulting methyl ester with excess ethylenediamine. With increasing the generation of dendrimers via a divergent method, the purity of the dendrimers decreases due to compact molecular structure which creates the structural defect in the molecular. To synthesize the dendrimer without defect, it must use a quantitative amount of reactant at each generation. However, the density of the dendrimer within a molecule increased with the increasing generation, which reduced the ability of EFG to further react.30
1.2.2. Convergent method. The convergent method was described by Frechet et al. in 1990, which was a different route than the divergent method to synthesize the dendrimers.31 In this method, the independent dendrimer called dendrons is first synthesized and then coupled to multifunctional core molecules (Fig. 4). It is directed by using the same AB2 monomer as in divergent, but in this method, B-functional groups are active where A-functional groups are inactivated/protected.
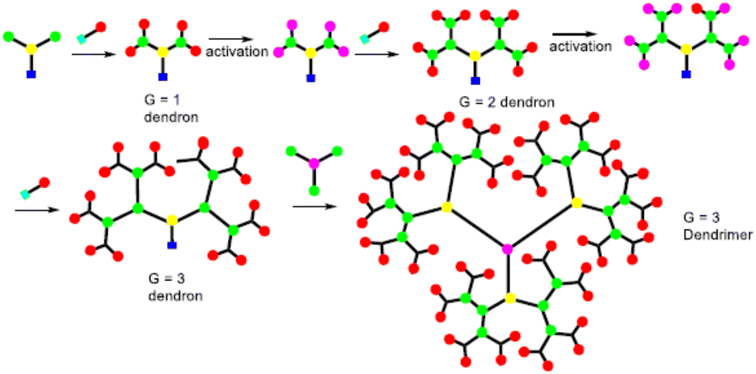 |
| Fig. 4 Structure of dendrimers in the Convergent approach method. | |
Initially, the B-functional group reacts with a molecule that will eventually build the terminal functional group (TFG) of the dendrimer. The main step in this method is the formation of protecting groups on the B-functional group. This way a 1st generation dendron is formed. After 1st generation dendron is formed, which activates the focal point of TFG and further reacts with the AB2 monomers to grow 2nd generation dendron. By repeating these steps will increase the generation of dendrons. In lastly, after the synthesized appropriate generation of the dendron A-function group is activated this is coupled with a core to form the complete dendrimer. By repeating the same reaction on AB2 monomer from generation to generation it is possible to build a defect-free dendrimer. This method has excessive control over the synthesis step which leads to high purity and defect-free dendrimer compared to the divergent method. Due to the bulky structure of the higher-generation dendron, the focal points reactivity is reduced and can result in core shielding.
1.3. Dendrimers as the molecular tweezers
Molecular tweezers are known as host–guest molecules with open void spaces capable of binding guest molecules.32 The term “molecular tweezers” was first used by Whitlock et al. to define a molecular receptor characterized by two more or less parallel aromatic or aliphatic side-walls, identical pincers separated by some more or less rigid chains.33 Non-covalent bonding, which includes π–π interaction, fluorophobic, solvophobic, hydrophobic forces, ion-dipole, hydrogen bonding, metal–metal coordination, van der Waals forces, and electrostatic effects, may be used to bind guests in the open cavity of the molecular tweezers.34,35 It can involve guests in molecular recognition phenomena despite having large structures. When a dendrimer is involved, the guest molecule only interacts with some dendritic units and not the entire dendritic structure. Kaifer and co-workers are mostly active in this field and have prepared numerous dendrimers containing a particular site as a potential guest unit.36
1.4. Dendritic effect
The existence of a large number of EFG on the periphery of dendrimers can generate several special effects. Characteristic physicochemical properties of dendrimers are depending on generation. The term ‘‘dendritic effect’’ is used to define rare physical and chemical property patterns of dendrimers as their generation increases.37 It is affected by multifunctional-group, steric shielding or blocking, changes like internal microenvironment, etc.38,39 It can be positive or negative depending on an increase or decrease in generation number. Drug delivery, molecular recognition, gene encapsulation, catalysis, photo-therapy, etc. are applications the dendritic effect improves the limitation of these applications.40,41
2. Pyrene-based dendrimers
The pyrene donor dendrimers (2) serve as effective light-harvesting antennae, transmitting light electricity through space from their outside edge to their center. Moreover, the mild-harvesting capability will increase with every generation because of growth inside the wide variety of peripheral pyrenes. So it evaluated the photovoltaic properties of the compounds, and the voltage reaction within the presence of visible light was obtained. The electricity switch efficiency became found to be nearly quantitative for the primary and second generations. It can end up as an essential additive to molecular photonic devices are shown in Fig. 5a.42 Moreover, the original series of Frechet-type dendrimers (3) bearing a cyclen center and fringe pyrene bunches were blended. They were described by different spectral techniques. The optical and photophysical properties of these mixtures were considered by assimilation and fluorescence spectroscopy. The force of the absorption increments straightly with the number of pyrenes present in the dendrimers. Furthermore, the outcome of these dendrimers is high quantum yields with an expanded extent of the excimer band as the age increases. The cyclen is a fascinating community for photoactive dendrimers. Since it is quiet in the ingestion and emanation range. However, it can associate with the dendron metal complex and adjust the photophysical properties of the dendrimers (Fig. 5b).43
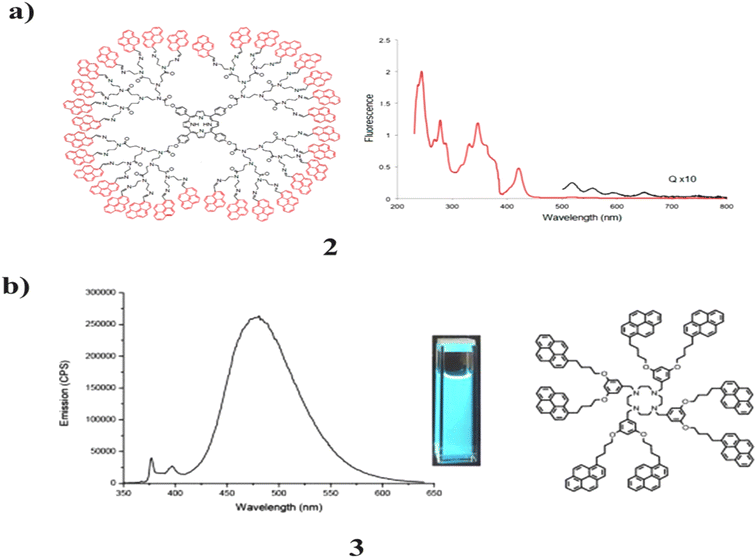 |
| Fig. 5 (a) Photovoltaic application of pyrene-porphyrin based dendrimers (2). (b) Photophysical study of pyrene-based dendrimers (3). | |
The dendrimers, which have a fullerene C60 and a porphyrin moiety at their core were synthesized using the pyrene-labeled dendrons (4). NMR spectrometry, MALDI-TOF spectrometry, and photophysical investigations were used to characterize the sample. The pyrene units and the porphyrin did not interact in the ground state, although there was a modest interaction between the pyrene units and fullerene C60. A significant reduction in the fluorescence intensity of the pyrene unit was observed in the occurrence of the fullerene C60 and porphyrin acceptor. So, it was possible to observe the FRET process from pyrene to fullerene C60 as well as from pyrene to porphyrin. They are substituted as able light-harvesting antennas (Fig. 6a).44 The critical aggregation concentration of pyrene-modified zero- and first-generation polyamidoamine (PAMAM) dendrimers (5). They are attached to the dendrimer skeleton by imine bonds, which play a key role in enhancing the aggregation propensity of the PAMAM dendrimers. The SEM studies suggest that pyrene-modified PAMAM dendrimers aggregate into doughnut-shaped assemblies. Since they are already aligned face-to-face in the pyrene chromophore ground state. The static pyrene excimer emits a quantum yield that has never been seen before and displays astounding positive solvatochromism from 498 to 638 nm. The bathochromic shift for pyrene excimer emission in solution is increasing as a result. Additionally, Lippert-Mataga's analysis of the system suggests that general and specific solvent effects play a crucial role in the progressive solvatochromism exhibited by the system. The investigations on luminescence quenching were conducted with different metal ions present. According to the results, mercury (Hg) ions can be selectively detected using pyrene-modified PAMAM dendrimers in the presence of a range of transition earth metal ions. The first dendrimer-based chromophoric system to display positive solvatochromism over a range of 140 nm is described in this report. It demonstrates that wavelength-tunable emitting systems with bluish-green, greenish-yellow, and orange-red colors at room temperature may be successfully synthesized using pyrene-modified PAMAM dendrimers are shown in Fig. 6b.45
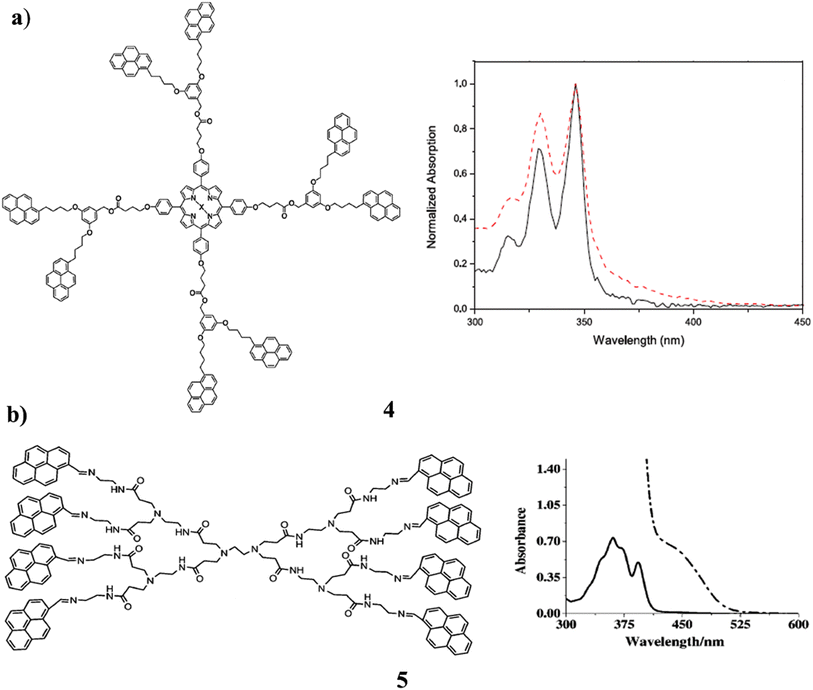 |
| Fig. 6 (a) UV spectra of the pyrene-porphyrins dendron (4). (b) Structure of pyrene-modified polyamidoamine dendrimer (5). | |
A poly(thiophenylene) dendron is attached to a polysulfated pyrene core in a new class of dendrimers (6). It exhibits remarkable photophysical properties. They have shown a significant dendron-localized absorption band with a maximum of around 260 nm and a band in the visible spectrum with a maximum at 435 nm. They can be attributed to the pyrene core that has been significantly affected by the four sulphur substituents. In addition, a significant amount of fluorescence was seen in the DCM solution at 293 K at ambient temperature. With the creation of a vibrantly colored deep blue radical cation, they underwent reversible chemical and electrochemical one-electron oxidation. More positive potential levels result in reversible one-electron oxidation. The three dendrimers' branch lengths allow for precise control over their photophysical and redox characteristics. For optoelectronic devices, it is possible to use the strong blue fluorescence to deep blue colour change that results from reversible one-electron oxidation (Fig. 7a).46 Design and synthesis of two constitutionally isomeric bis-pyrenyl phenol dendron derivatives (7) with various architectural motifs on the periphery of the cyclo-triphosphazene ring. Standard spectroscopic methods are used to carry out the structural characterization. Density functional theory calculations at the wB97XD/6-31G(d) level are used to accomplish geometric optimization of the constitutional isomeric dendrons and their dendrimer-like derivatives, HOMO–LUMO energy levels, and molecular interactions. Moreover, the measurements reveal that nitro-aromatic explosions effectively blocked fluorescence emission. Additionally, other competing species that were evaluated did not cause any spectrum alterations, indicating that these cyclo-triphosphazene derivatives resembled dendrimers. It could be used as a very sensitive and selective sensor for explosive nitro-aromatics are shown in Fig. 7b.47
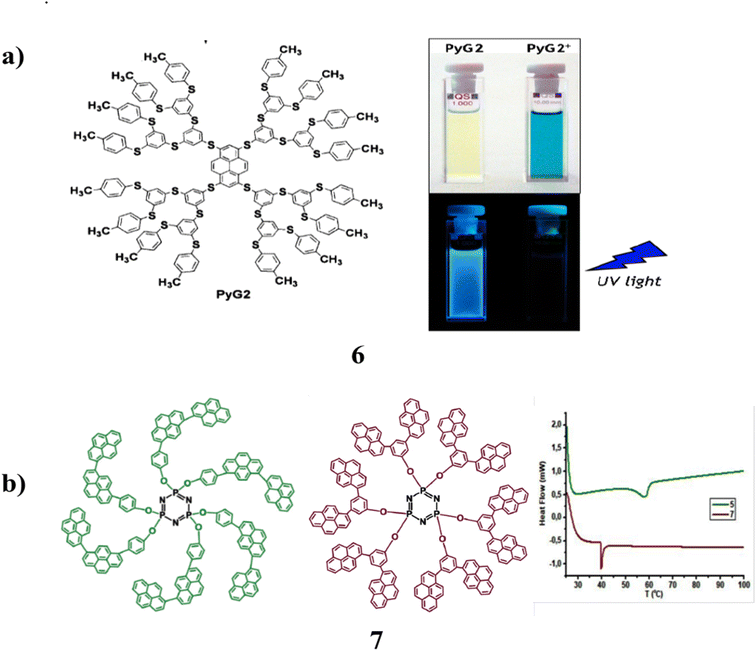 |
| Fig. 7 (a) Chemical structure of poly-sulfurated pyrene dendrimer (6). (b) DSC thermograms in the N2 atmosphere of compounds (7). | |
However, the pyrene-terminated dendrimers with ester linkage (8) and (S)-BINOL core have been synthesized in good yields by employing carbodiimide as the coupling reagent. The fluorescence spectra showed monomeric emission, which indicated the absence of π–π stacking. It is altered by the presence of a pyrene group. The application of dendrimers as an additive in the redox coupling of dye-sensitized solar cells. The absence of “π–π stacking” was demonstrated by the fluorescence spectra is monomeric emission. The presence of a pyrene group causes it to change. The use of dendrimers as a supplement in the redox coupling of dye-sensitized solar cells (Fig. 8a).48 The pyrene-based dendrimer (9) with ester branching points further demonstrates that the number of pyrene pendants had an impact on the power conversion efficiency. The carbonyl group is conjugated in this process. The structural alterations on conjugated pyrene units will make the power conversion practicable. The findings also imply that conjugated systems may have improved light harvesting capabilities. As a result, solar cells perform better than other molecular systems (Fig. 8b).49
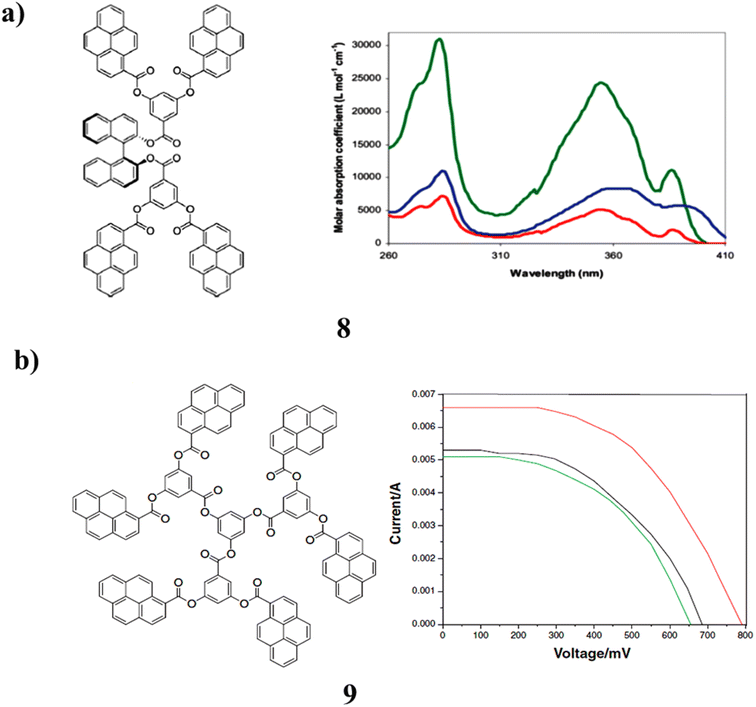 |
| Fig. 8 (a) Structures of (S)-BINOL-based pyrene ester dendrimer (8). (b) Structure of pyrene-based ester dendrimer (9). | |
A dendrimer (10) with pyrene as the core and 9-phenylcarbazole (PCZ) on the outside is demonstrated to be a multipurpose fluorescent probe for iodide, iron(III), and mercury(II). It functions as the dual-channel fluorometric and colorimetric probe for these ions. The fluorescence of the dendrimer acts as a fluorometric probe and is inhibited by both iodide and iron(III). After that, when mercury is added, the dendrimer to which iodide has been added will regain its fluorescence (II). For iodide, the colour change is perceptible at 0.1 mM. Additionally, it serves as an electroactive precursor for the electro-polymerization process used to create luminous films. The generated films can be utilized to detect ions as fluorescent films. This is explained by the existence of an increased conjugation and a strongly cross-linked microstructure. The blue fluorescence on the electro-polymerized film has an excitation/emission maxima at 365/460 nm (Fig. 9a).50 In addition, the 1,4,7,10-tetraazacyclododecane (cyclen) unit of a pyrene-based dendrimer (11) was studied using steady-state fluorescence and single-photon counting fluorescence. Pyrene-labeled signals from the molecules were recorded in each solvent and showed the expected fluorescence spectra. The pyrene chromophores matching monomer and excimer emissions are observed. Unexpectedly, these dendrimers did not exhibit the normal quenching of tertiary amine on the pyrene emission (Fig. 9b).51
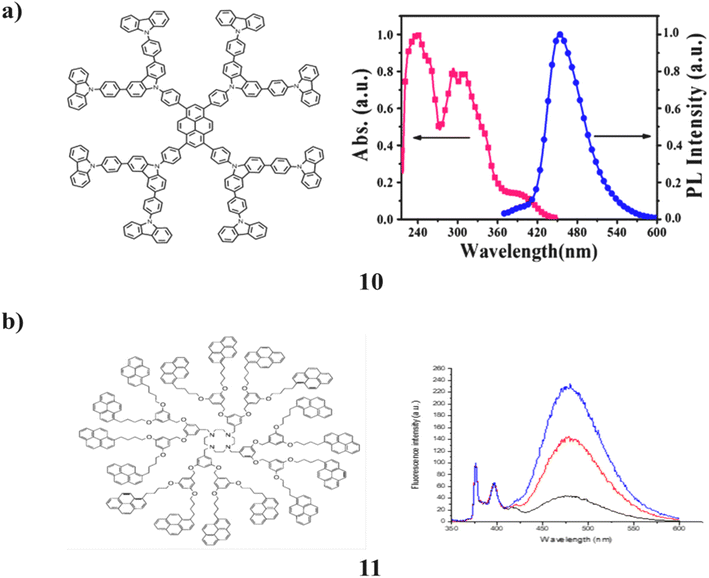 |
| Fig. 9 (a) Absorption and photoluminescence (PL) spectra of pyrene core-dendrimer (10). (b) Fluorescence behavior of pyrene-amine-containing dendrimer (11). | |
The pyrene-cored chiral dendrimer (12) has a higher hydrophobic impact than benzene and is more likely to gel than benzene itself. But because of its poor solubility, the forms only gel in DMSO. Although the poor alignment of the pyrenyl moieties in the gel phase prevents excimer production, excimer formation was reported for the dendrimer gel. A lamellar structure of the gelators in each gel phase was visible in the gels (Fig. 10a).52 The synthesis of a dendrimer (13) surface modified by pyrene units. In addition to a distinct redshift, a fluorescence increase in pyrene excimer emission was also seen. Dynamic pyrene excimer production caused it to occur. Because of the intramolecular π-stacking contact between two pyrenyl rings at high water fractions, the aggregation increased the excimer formation efficiency of dendrimers. Additionally, dendrimer intra- and intermolecular interactions result in strong and weak excimer creation as well as simple aggregation-enhanced excimer emission (AEEE). In the dendrimer, it was observable very clearly that monomer emission was quenched while excimer emission was increased. The concentration of the solvents as well as their dielectric constant affects the emissions. It is anticipated that it will be used with photoactive dendrimers (Fig. 10b).53
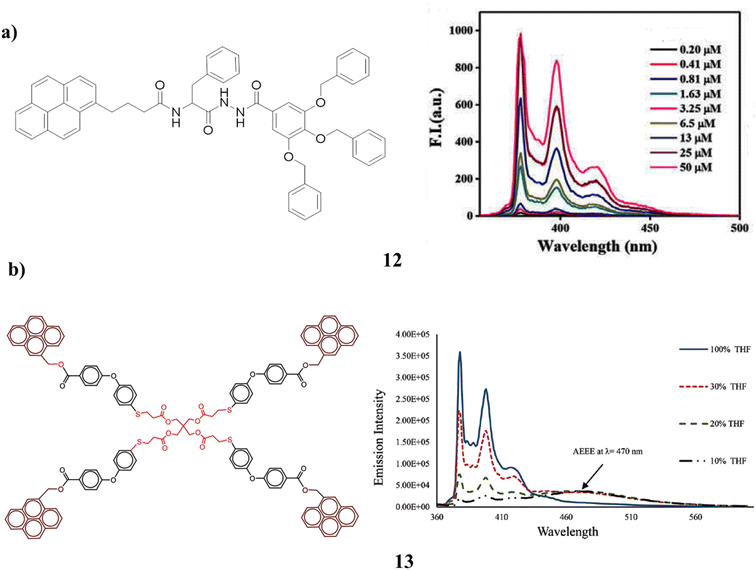 |
| Fig. 10 (a) Gelation of pyrene-cored chiral dendrimer (12). (b) Blue emission based on pyrene dendrimer (13). | |
A pyrene-labeled ruthenium(II) tris bipyridine complex and pyrene-labeled ruthenium(II) tris bipyridine cored dendrimer were both synthesized as novel bipyridine ligands (14). They were characterized using MALDI-TOF mass spectrometry and NMR spectroscopy. By using absorption and fluorescence spectroscopy, the optical and photophysical characteristics of the luminescent macromolecules were investigated. There is no interaction in the ground state, as evidenced by the fact that the absorption spectra of the obtained complexes add up to the total absorption spectra of their constituents. The typical ruthenium bipyridine emission band was found upon stimulation at the pyrene absorption wavelength as a result of the efficient energy transfer that was seen from the pyrene units to the metal complex core. Furthermore, the first-generation dendrimer's effective protection from oxygen quenching was noted (Fig. 11a).54 The effects of the amphiphilic polyphenylene dendrimers (PPDs) (15) on protein adsorption in blood plasma were investigated. These PPDs have different lipophilic and positively charged surface groups. By increasing the adsorption of proteins that regulate cellular uptake based on their surface patches, the PPD corona decreased the binding of certain opsonins and enhanced the binding of others (Fig. 11b).55
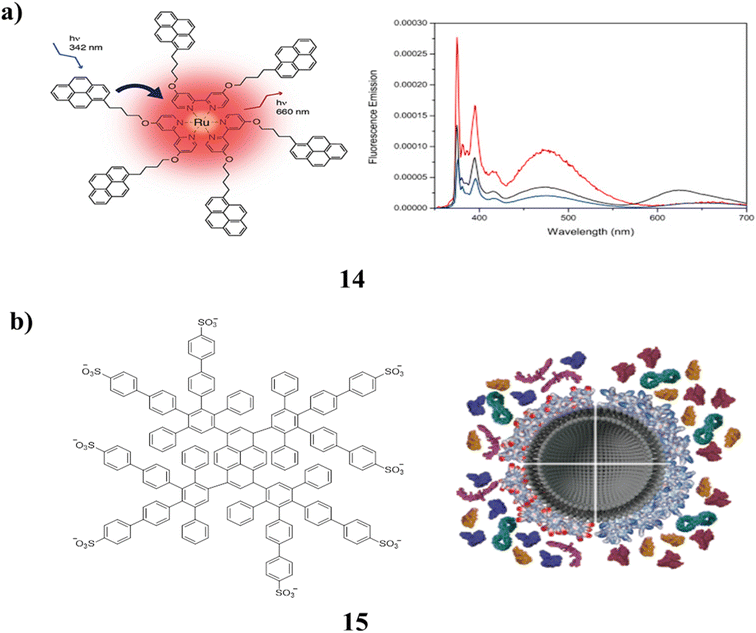 |
| Fig. 11 (a) Pyrene labeled ruthenium(II) tris bipyridine complex cored dendrimers (14). (b) Structure of amphiphilic dendrimers (15). | |
For T-series dendrimers made up of nearly hundreds of atoms, TD-DFT (16) techniques and semi-empirical approaches have been used to establish the structure-related spectral characteristics. These dendrimer molecules one- and two-photon absorption spectra have been accurately reconstructed. Theoretical research has been done on the “antenna effect” in the dendrimer molecule. Using the contour of charge difference density (CDD) between electronic states, the process of excitation energy localization from chromophores in branches to the pyrene core prior to the fluorescence emission is observed. The measured photophysical characteristics of the T-series dendrimer have been used to inform the theoretical model (Fig. 12a).56 The PPI(core)–PAMAM(shell) hybrid dendrimers' (17) structural characteristics for use in drug delivery. Its ability to encapsulate medicinal guest molecules like pyrene is researched by altering the core (PPI) in the PPI–PAMAM hybrids. It is studied using coarse-grained molecular dynamics simulation. The size of hybrid dendrimers, the depth of water penetration, the level of back folding of their chain terminals, and the size and distribution of generated holes are only a few of the structural features that can be predicted using PPI core sizes. The findings demonstrate that the PPI core size has no effect on where pyrene is located in the hybrid internal structure. In the PPI shell, the branching chains act as a defence against the molecules that penetrate it. The PPI barrier is then reduced by adding PAMAM to the surface, which improves the ability of the hybrid to encapsulate substances (Fig. 12b).57
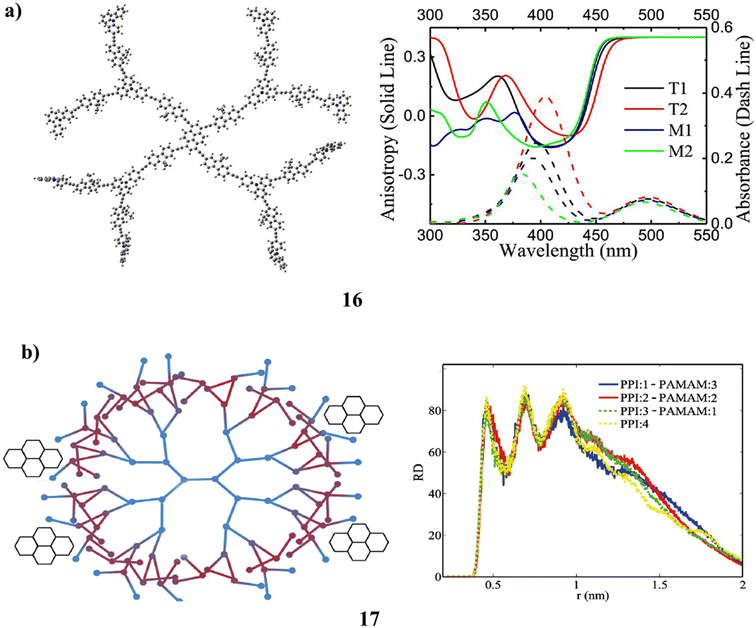 |
| Fig. 12 (a) Absorption (2PA) spectra of T-series dendrimer (16). (b) Hybrid dendrimer of PPI(core)–PAMAM(shell) (17). | |
3. Coumarin-based dendrimers
The porphyrin-cored dendrimer (18) was investigated by UV/Vis absorption and fluorescence spectroscopy in diluted DCM solutions and thin neat films. Two parameters that affect the efficiency of energy transfer are revealed by the intramolecular energy transfer from the coumarin units to the porphyrin core. High energy transfer efficiency is produced by the spectral overlap between the coumarin moiety is emission spectrum and the porphyrin core absorption spectrum. Second, while a long alkyl side chain makes dendrimers more soluble, it also stops coumarins from self-quenching. As a result, the dendrimer with N-octyl groups is more effective than the one with N-ethyl ones. Compared to unbound porphyrin, it emits red light with higher fluorescence quantum yields (Fig. 13a).58 A number of carbazole dendrimers (19) with thiophenyl coumarin cores and carbazole dendrons up to the third generation as substituents were synthesized and described. The non-doped solution-processed light emitters optical, thermal, electrochemical, and electroluminescent characteristics were investigated. By including carbazole dendrons in the molecule, it is possible to improve the thermal stability of the compound while also reducing crystallization and maintaining the high emissivity of a planar thiophenyl coumarin fluorescent core in the solid form. Its fluorescence was bright green and had a glass transition temperature of up to 285 °C, which allows it to form structurally stable amorphous thin films. With BCP acting as a buffer layer and these materials serving as hole-transporting non-doped emitters, simple structured solution-processed OLEDs provide stable green electroluminescence with excellent brightness efficiency and high green color purity (Fig. 13b).59
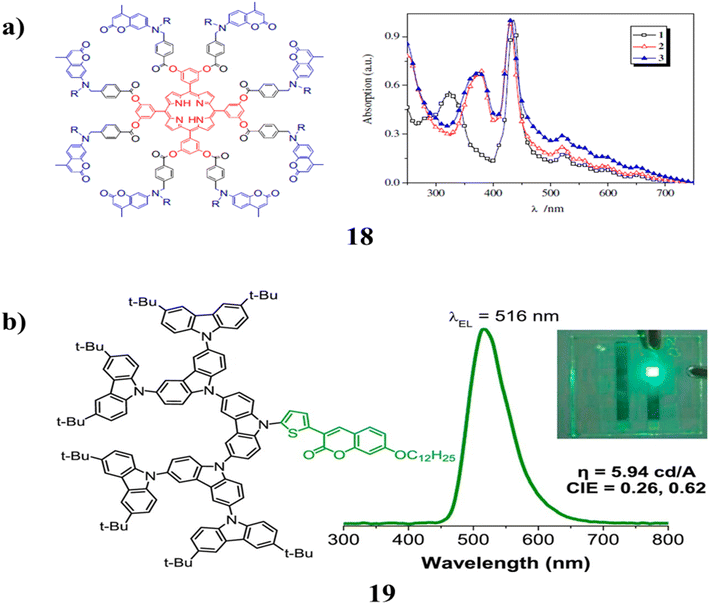 |
| Fig. 13 (a) Non-conjugated dendrimer with a porphyrin core and coumarin chromophore (18). (b) Structure of coumarin cored carbazole dendrimer (19). | |
Additionally, a coumarin dendrimer-based FLISA assay (20) was appropriate for the quantification of parasite counts in addition to the diagnosis of Plasmodium infection since fluorescence density was closely linked with parasite numbers. The correct diagnosis of Plasmodium infection could thus be facilitated by FLISA as a malaria diagnostic. It would be an obvious substitute assay for figuring out Plasmodium parasite density. FLISA may be seen as a workable approach for high throughput analysis of malaria infection during blood transfusion in ROK since fluorescent intensity is highly sensitively connected with malaria density. Additional research should evaluate the diagnostic performance of patients with asymptomatic parasitaemia and other Plasmodium spp. Infections (Fig. 14a).60 Additionally, non-invasive biological manipulation is possible because of photoactivation employing two NIR photons. The materials sensitivity to NIR light will be increased by the dendritic amplification. When 4-bromo-7-hydroxycoumarin (BHC) was combined with self-immolative dendrimers (21) as opposed to BHC that was directly conjugated to L-glutamic acid, light-induced uncaging otherwise release of L-glutamic acid was 2.8 fold higher (Fig. 14b).61
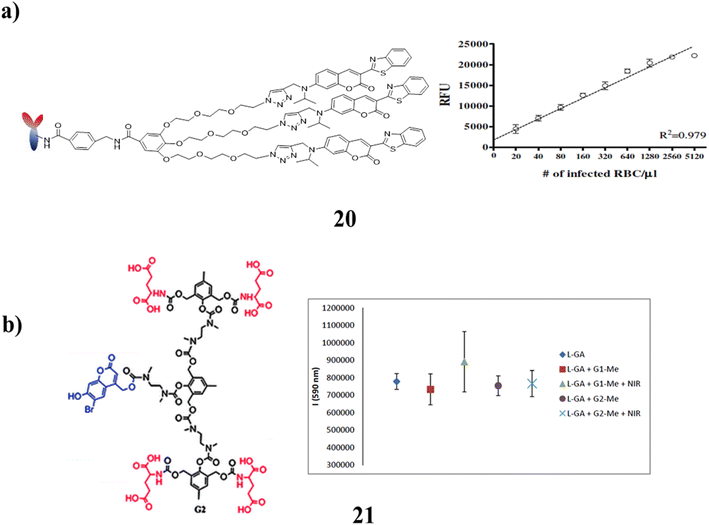 |
| Fig. 14 (a) Performance of coumarin-derived dendrimer (20). (b) Structure of self-immolative dendritic scaffolds (21). | |
A poly(amidoamine) (PAMAM) dendritic core functionalized with bisMPA dendrons containing cholesterol and coumarin moieties makes up a series of amphiphilic hybrid dendrimers (22). Investigations were made into their self-assembly behavior in water and bulk. Depending on the generation of the dendrimer, it has shown both smectic A and hexagonal columnar liquid crystal structures. These dendrimers self-assembled in water to create solid, spherical micelles that could encapsulate the hydrophobic model substance Nile Red. The cell viability in vitro of the micelles was studied in the HeLa cell line and showed to be non-toxic up to 72 h of incubation. These spherical micelles enable the encapsulation of hydrophobic molecules as a result. The potential of these dendrimers as nano-carriers for drug delivery applications is also shown by the fact that they simultaneously give fluorescent trace ability due to the presence of coumarin units in their chemical structure (Fig. 15).62
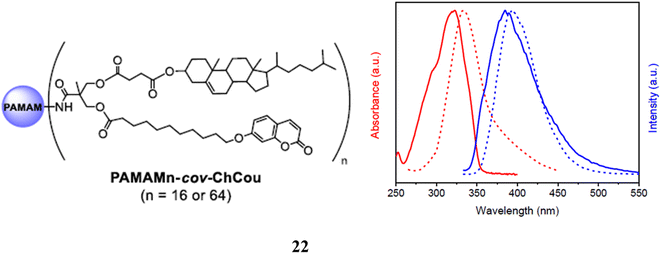 |
| Fig. 15 Dendritic molecule of fluorescent coumarin moieties (22). | |
4. Perylene-based dendrimers
Two types of polyimide dyes, decacyclene and perylene-containing dendrimers were used in polyimide dendrimers (23). The solubilizing chains in these constructions are N-9-heptadecanyl-substituted perylene diimides, and the branching unit is trisphenylamine. It has been studied using pump–probe transient absorption spectroscopy, steady-state absorption, and time-resolved emission spectroscopy. When visible excitation occurs, the photoinduced charge-separated (CS) states are created on a timeframe of femtoseconds. In particular, two distinct CS states can develop, involving various components that decay separately and have various lifetimes (Fig. 16a).63 Additionally, the luminous characteristics of the perylene bisimide cored dendrimers (24) with polyphenylene dendrons and peripheral functional groups of carbazole were investigated. The excellent site-isolation effect of the bulky dendrons on the perylene bisimide core was demonstrated by the hypsochromic effect seen in both the absorption and emission of these dendrimer films. Due to the intramolecular charge transfer process, the carbazole units do not have a high luminous quantum yield. But having them there helped the EL performance. It is hypothesized that the hole-transporting carbazoles enhanced the charge balance state in OLEDs and served as an antenna group to transfer energy to the core, improving EL emission as a result. It is important to remember that the majority of PBI derivatives published so far include the current dendrimers. When compared to other types of materials with comparable quantum yields, they have great fluorescence quantum yields, but their EL capabilities are still far from perfect. This is most likely a result of the comparatively tiny Stokes shifts and high self-absorption seen by this group of compounds. Another potential explanation for the EL performance of these PBI dendrimers is the fact that the dendrimer structures would typically reduce the mobility of the charge carriers due to the site-isolation effect. Consequently, it could be to modify the structure of PBI derivatives to increase the Stokes shift and thereby improve performance (Fig. 16b).64
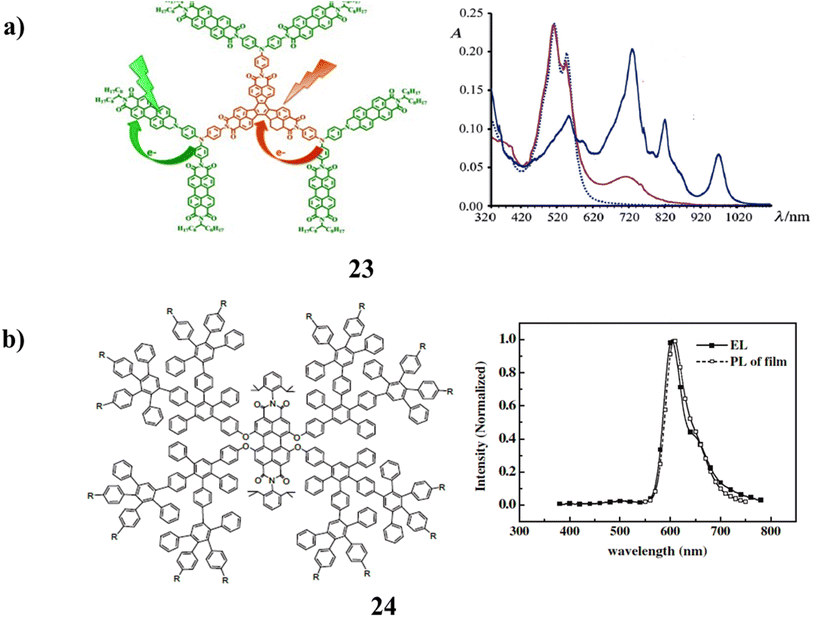 |
| Fig. 16 (a) UV spectrum of Polyimide dendrimers (23). (b) Carbazole surface groups of perylene-bisimide-cored dendrimers (24). | |
A clickable mono-functional PGD-capacity PDIs to be singly connected to a biomolecule in a specific manner allowed for the synthesis of extremely water-soluble fluorescent PGD-PDIs (25). However, a single biotinylated PGD-PDI was used for both the single-molecule and live-bacteria imaging. The most important finding was that, unlike earlier ionic non-dendronized PDI analogs, site-isolated, uncharged PGD-PDIs can act as highly specific protein labels on the surfaces of living bacteria cells. A new generation of bio labels with a mono-functional fluorescent core and a multivalent peripheral made up of cellular receptors, therapeutic drugs, targeting groups, and ligands are made possible by this method of encapsulating dyes in dendrimers, which improves their performance (Fig. 17a).65 Nevertheless, there is a brand-new class of poly(aryl ether) dendrimers (26) that contain both donor and acceptor moieties. P-type terthiophene, n-type perylene, and naphthalene diimides are combined to form a dual electrochromic material. They have the electroactive terthiophene group, which may be cross-linked electrochemically to make conjugated polymer network (CPN) films. They were created to serve as electrochemically active precursor polymers. The inclusion of donor and acceptor moieties in the same molecule while maintaining doping properties is demonstrated by the optical and redox properties. Both the p- and n-doping techniques take use of the high activity of the films produced by electrochemical polymerization (Fig. 17b).66
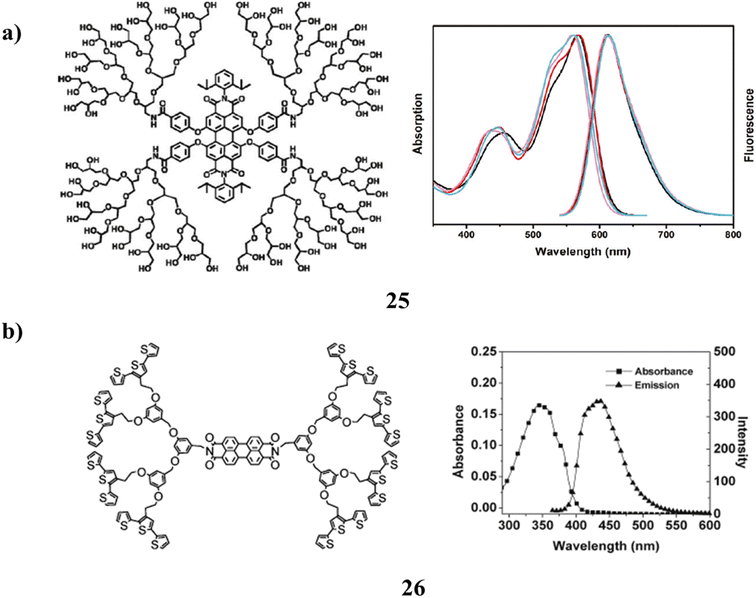 |
| Fig. 17 (a) Structure of water-soluble perylene diimide cored dendrimer (25). (b) Electropolymerizable terthiophene-based poly(aryl ether) dendrimer (26). | |
The synthesis and characterization of a class of polyphenylene dendrons with pentafluorophenyl and cyano surface groups were found in dendrimers with perylene-bisimide cores (27) that include these molecules. It has strong film-forming and soluble qualities. They have high electron affinities, extensive light absorption that nearly encompasses the entire visible light spectrum, and high fluorescent quantum yields. All of these advantages suggest that they are potential multifunctional materials, both as red emitters for organic light-emitting diodes and as light-harvesting electron acceptors in solution-processible solar cells (Fig. 18a).67 Rigid polyphenylene dendrimers (PPDs) (28) have been used to synthesis a host that is fluorescent and photoresponsive. A complex tetracycline containing azobenzenyl, pyridyl, and ethynyl entities is the main component in the formation of the divergent dendrimer. The site-specific insertion of various functions in eight azobenzene (AB) moieties into the rigid scaffold, a fluorescent perylenetetracarboxdiimide (PDI) into the core, and eight pyridine functions into the interior cavities depends critically on the poly-phenylenes rigidity. Additionally, dendrimers AB moieties go through reversible cis–trans photo-isomerization. Various spectrum approaches have demonstrated that they are photostable. In addition to enabling highly sensitive FCS to track the impact of cis–trans photo-isomerization on molecular size, the presence of PDI also enables time-resolved optical spectroscopy to track the effectiveness of the intramolecular energy transfer mechanism. Additionally, the pyridyl functionalities were added to promote guest uptake via hydrogen connections between the host and guests. Additionally, photo-switchability can be used to actively encapsulate guest molecules into their internal cavities. This light-driven encapsulation method might make it possible to synthesis new drug delivery systems (Fig. 18b).68
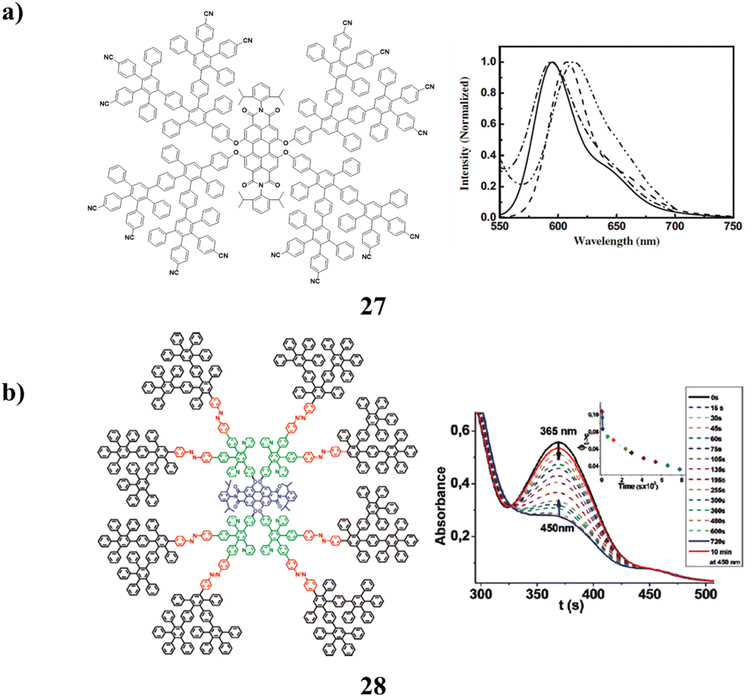 |
| Fig. 18 (a) Structure of perylene-bisimide-cored dendrimer (27). (b) Photo-switchable encapsulation of guest in dendrimer (28). | |
A novel perylene-diimide naphthalimide molecule (29) has been described using high-resolution mass spectrometry (HRMS). With an energy transfer efficiency of 55%, it demonstrated a quick fluorescence response to Fe3+ ions and three times enhanced core emission (at 600 nm). According to the two-photon fluorescence tests, the dendritic compound exhibited good two-photon fluorescence characteristics. The relationship between a molecule structure and its fluorescence characteristic has been discussed in the meantime. Additionally, the substance was successfully used to image Fe3+ ions in HeLa cells (Fig. 19a).69 It has been confirmed that the seventh to ninth generations can be produced by the catalyst-free Diels–Alder synthesis of polyphenylene dendrimers (30) with a chromophore core upon divergent growth. MALDI-TOF mass spectrometry was used to characterize the entire series of nine generations because conventional analytical instruments like size-exclusion chromatography do not produce accurate molecular weights. At such great masses, perfection and mono-dispersity were thus clarified. These molecularly defined nano-sized “particles” of up to 33 nm in diameter were measured using transmission electron microscope imaging (Fig. 19b).70
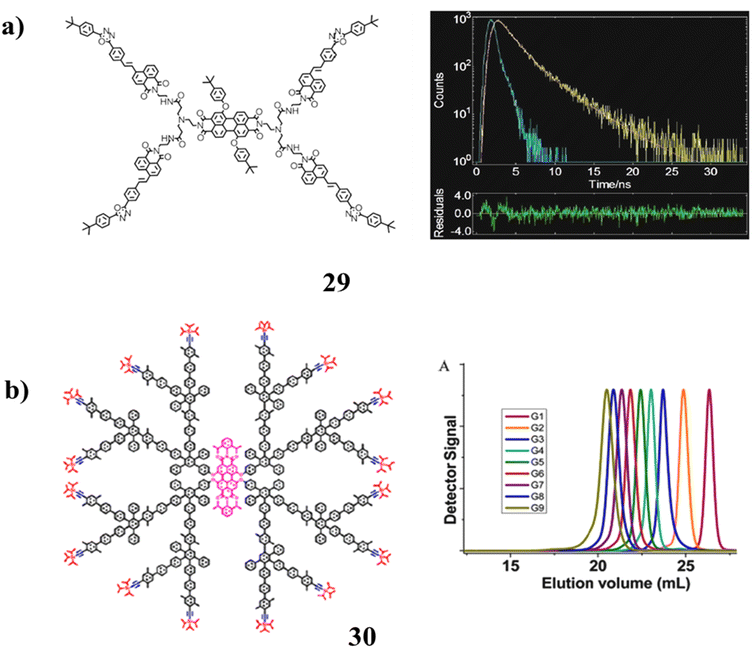 |
| Fig. 19 (a) Fluorescence property of perylenediimide-naphthalimide dendrimer (29). (b) Structure of giant polyphenylene dendrimers (30). | |
The dendritic C60-perylene diimide (PDI) conjugates (31) were made using fullerenes with a carboxylic acid function at the focal point and a perylene diol building block (Per) under esterification conditions. The cyclic voltammograms for the dendrimer exhibit the distinctive electrochemical characteristics of both constituent units. The oxidation of the dendrimers is focused on the PDI core, as evidenced by the comparison with the comparable model compounds. The initial reduction is consistent with a process that is fullerene-centered. In a toluene solution, the photophysical characteristics of the related reference systems Per and F were examined. In the Vis and NIR spectral ranges, Per and F show nearly overlaid fluorescence bands, demonstrating that the respective singlet states are almost iso-energetic (B1.75 eV). The fluorescence quantum yields and singlet lifetimes of the two bands are considerably different from one another. Regardless of the excitation wavelength, a very unique luminescence behavior is always observed in dendrimers due to the iso-energetic singlet levels of the PDI and fullerene moieties. While the intensity of the observed fluorescence band is around 20 times lower than that of Per, its lifetime is that of the periphery fullerene unit. The fluorescence band is always the form of the central core of the PDI. This unconventional behaviour at 298 K is explained by a kinetic model including fast equilibration of the singlet levels, with the PDI-centered level being the highest and separated by around 0.06 eV. Studies on bimolecular transient absorption in solutions containing F and Per have revealed that the photo-excited fullerene transfers triplet energy to the PDI. Light excitation is followed by inbound energy transfer to the central core and creation of the PDI triplet state, showing that the photophysical behaviour is independent of dendrimer size (Fig. 20).71
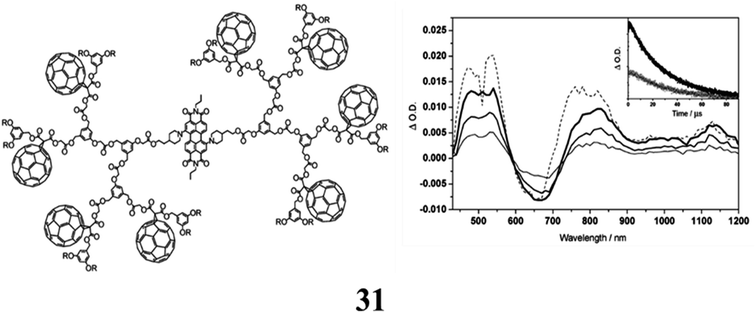 |
| Fig. 20 Fullero-dendrimers of a perylene-diimide core (31). | |
5. Rhodamine-based dendrimers
By using a divergent process, rhodamine B decorated mesitylene cored and methylene p-phenoxy bridged dendrimers (32) were successfully synthesized up to the second generation. It was discovered to be more effective than dendrimers of lower generation against both Gram-positive and Gram-negative bacteria (Fig. 21a).72 The triazole bridged dendrimers with rhB derivative surface groups were synthesized via click chemistry. Spiro-lactam grafted at the terminal was also used to synthesis RhB-decorated dendrimers (33) up to the second generation. With an increase in dendritic generation, the UV and fluorescence intensity also increases. By using the DPPH radical scavenging assay and hydroxyl radical scavenging assay techniques, it exhibits substantial antioxidant behaviour in comparison to the standards of butylated hydroxytoluene (BHT) and gallic acid. Due to the presence of more triazole branching units and rhodamine B derivative surface units, RhB-decorated higher-generation dendrimers have better antioxidant activity than lower-generation dendrimers (Fig. 21b).73
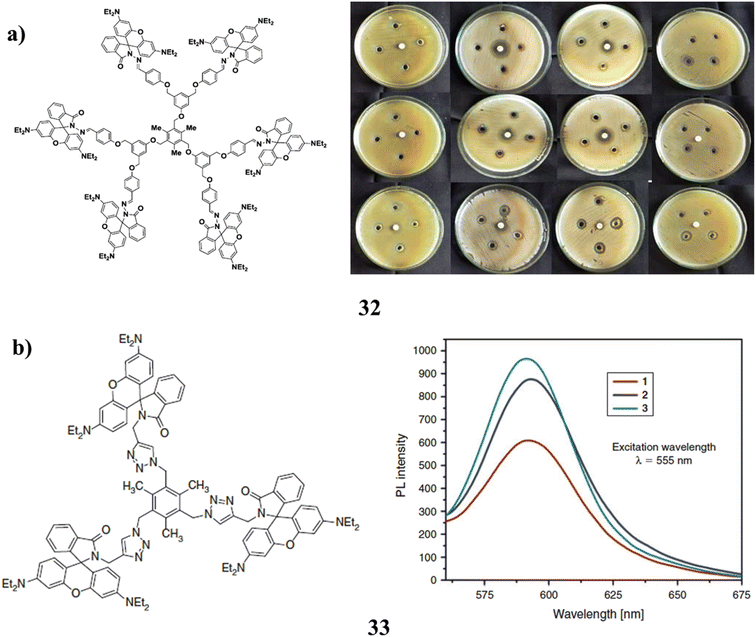 |
| Fig. 21 (a) Antibacterial study of Rhodamine B decorated mesitylene cored dendrimers (32). (b) Antioxidant properties of RhB decorated dendrimers (33). | |
Additionally, the (S)-BINOL cored rhodamine B decorated triazole bridging dendrimer (34) was successfully synthesized by click reaction up to the second generation. According to the photophysical characteristics, the dendrimer's generation increased along with its capacity to absorb light and fluorescence intensity. The specific rotation increases as dendrimer generation increase, as shown by the chiro-optical characteristics. It shows that the dihedral angle at the (S)-BINOL unit widens as the density of the dendritic wedges increases. In cyclic voltammetry, all dendrimers exhibit quasi-reversible behaviour. As the dendrimer generation develops from the zeroth to the second generation, the anticancer activity against human hepatocellular carcinoma cells increases (Fig. 22a).74 In addition, the synthesis of RhB functionalized entirely shifted the equilibrium between the open form and the ring-closed (spiro-lactam) form in favour of the latter, whereas the other rhodamine derivative structure prevents ring closure (35). The first-generation phosphorus-containing dendrimer was grafted with the spiro-lactam derivative as a terminal group. The rhodamine substitute was only protonated as a result of the spiro-lactam forms attempt to include HCl (Fig. 22b).75
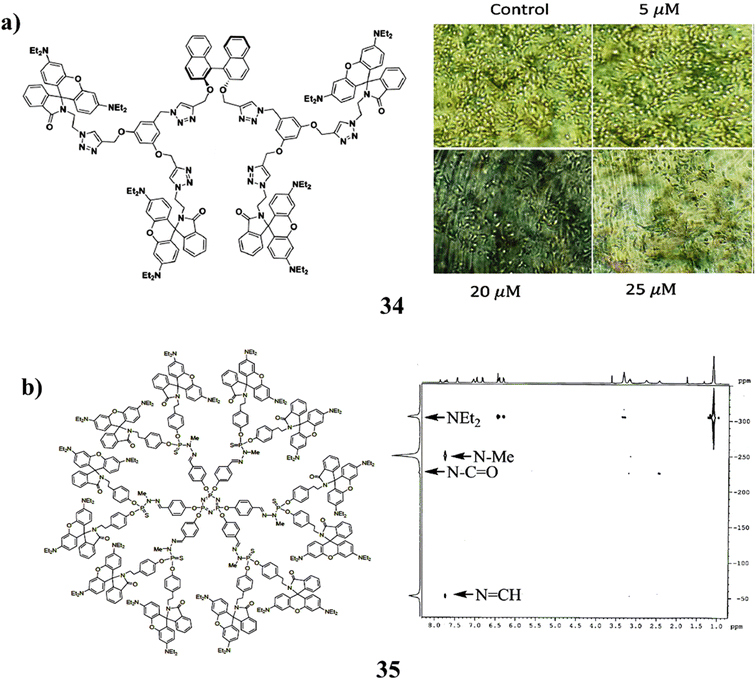 |
| Fig. 22 (a) Anticancer study of triazole-based dendrimer (34). (b) Structure of phosphorus-containing dendrimer (35). | |
Additionally, the fluorescent PAMAM dendrimer derivative (36) proved a useful substance capable of monitoring the transport of nucleic acids invitro. Similar to the pure molecule, it is capable of complexing miRNAs. The advanced material was also used to cover a PLA scaffold that had been 3D printed in order to provide a surface for cell growth and proliferation. It could be made rapidly, cheaply, and biocompatible using conventional procedures, and it could lead to healthcare products with several uses. The cutting-edge material created in this work has a potential value not just in the biological field but also in applications for tissue regeneration (Fig. 23a).76 The platform provides a method for modularly tethering bioactive cargos to the dendrimersomes (37) membrane-mimicking surface. Proteins, nucleic acids, and hydrophobic compounds of all molecular weights are among the cargoes carried by these particles. Thin film hydration of dendrimersomes results in loading that is greater than that possible with routinely used stealth liposome formulations. It is easily functionalized with ligands, such as NTA-conjugated Janus dendrimers, and integrated into the lamellar structure of dendrimersomes to bind proteins with common molecular biology tag residues (Fig. 23b).77
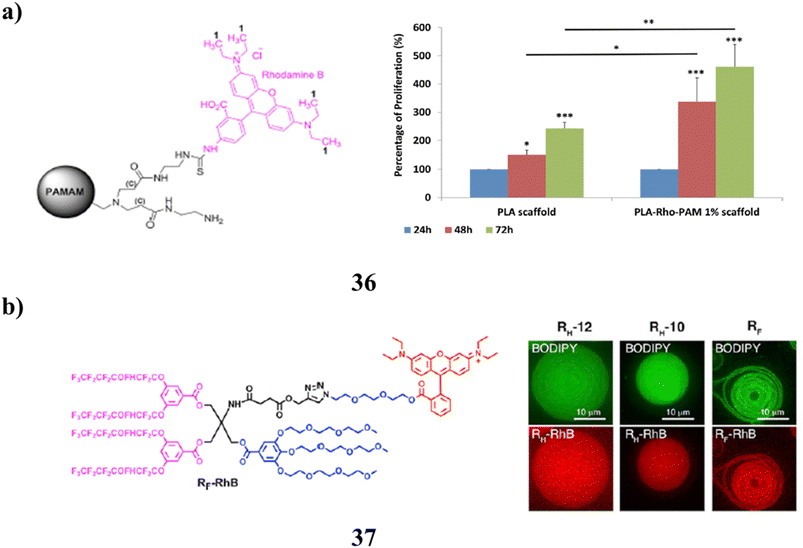 |
| Fig. 23 (a) Fluorescent PAMAM dendrimer for biomedical applications (36). (b) Molecular biology study of dendrimersomes (37). | |
As non-viral vectors for siRNA delivery in primary neurons, astrocytes, glioma, and glioblastoma cell lines, the amino-functional bis-MPA dendrimer (38) of generations one to four has also been studied. In N/P ratios of 1.5
:
3, it was effective at forming charge–interaction complexes with siRNA for complete siRNA complexation. They weren't harmful to glioma, glioblastoma, or astrocyte cell lines. It demonstrated cytotoxicity toward primary neurons that was dose-dependent. Efficacious endo-lysosomal escape by the dendrimers, with unique mitochondrial subcellular localization, was suggested by fluorescence microscopy investigations. Primary neurons did not exhibit transfection or protein down-regulation. In contrast, transfection was successful in rat glioma and human glioblastoma cells, which was followed by a 20% drop in the expression of the target protein. Evaluation of the benefits and challenges of bis-MPA dendrimers for the intracellular delivery of siRNA (Fig. 24).78
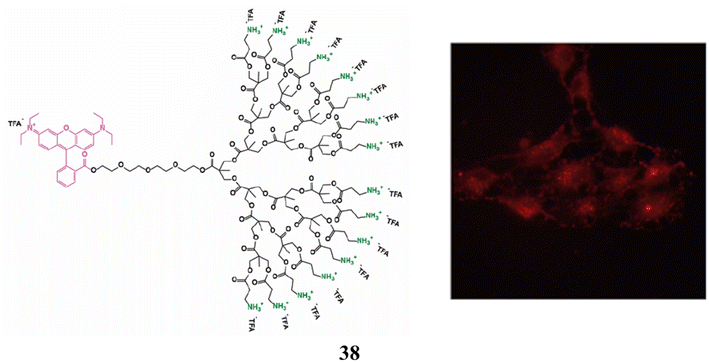 |
| Fig. 24 RhB-based polyester dendrimer (38). | |
6. Glycoside-based dendrimers
The design of a low-technology dendrimer (39) is primarily based on a simple calix[4]arene scaffold with the inclusion of the specified C2-symmetric imino sugar analogue 3,4-dihydroxypyrrolidine. This approach allows for the quick inclusion of a large number of iminosugar-like moieties in a reduced quantity with a well-defined shape (Fig. 25a). It was confirmed that alkali steel ions were present within the polar hollow area created by the acetamide moieties at the calixarene's lower rim. Additionally, it enables the rigidification of the dendrimer form and the presentation of iminosugar in the clusters. It is possible to synthesis correctly characterized multivalent and multidimensional structures with more complicated and biologically appropriate iminosugars when the supramolecular features of calixarenes are combined with the addition of a dendrimeric presentation of repeated units.79 The synthesis of quite mannosylated polyester dendrimer (40) with 2, 4, 8, and 16 D-mannopyranose residues on their peripheries connected using specific linker palms is presented are shown in Fig. 25b.80 Additionally, those systems' appealing qualities as possible uropathogenic Escherichia coli (UPEC) inhibitors include the use of risk-free and biocompatible polyester dendrimer backbones. They are made to fit between the tyrosine gate and a few copies of D-mannopyranose residues connected to them. It is successfully bound to the exposed mannose-touchy kind 1 pilus on the outer floor of UPEC.
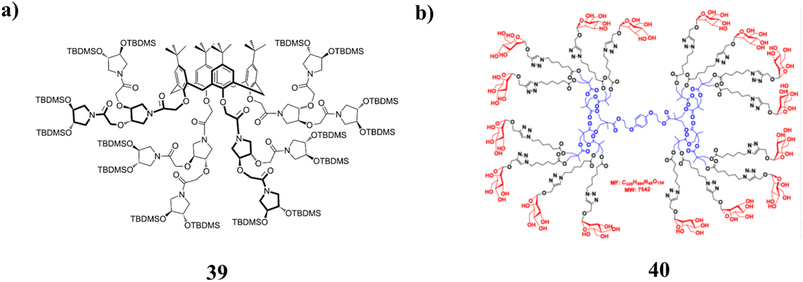 |
| Fig. 25 (a) Imino-sugar-based low-generation dendrimer (39). (b) Structure of polyester glyco-dendrimer (40). | |
Additionally, the green synthesis of multi-tasking dendrimer (41) is at the core of nano-molecular healing packages and serves as one of the supports for the distribution of pills and genes. The additional functions of glyco-dendrimers are primarily their ability to inhibit biofilms and disrupt viral and bacterial adhesion mechanisms. A single strategy that combines the adaptability of the recently discovered “onion peel” (hetero-layered) dendrimer construction with the addition of ground hybrid glyco-ligands for focusing on various bacterial strains. Then select processes, such as the photolytic thiol–ene reaction using orthogonally functionalized scaffolds and the Cu(I) catalyzed azide–alkyne [1,3]-dipolar cycloaddition (CuAAc). Additionally, the two families of glyco-dendrimers have synthesized them using a convergent approach. The special layers consist of hexachlorocyclophospazene, pentaerythritol, and cyanuric chloride. They were made with both optimized D-galactopyranoside and -D-mannopyranoside ligands. The resulting hybrid glyco-dendrimers were successfully tested utilizing dynamic light scattering against two different lectins (DLS) (Fig. 26a).81 A green investigation of the interactions between glycoproteins was carried out using the multivalent glyco-dendrimer library (42). The one-of-a-kind dendrimers with different peripheral glyco-densities and linkers provided a variety of potent potential therapeutic retailers that would be useful for more specific motion and higher binding affinities against their corresponding protein receptors. Additionally, the fundamental technique for the synthesis of mannosylated dendrimers is click chemistry. Scaffolds that have been propargylated and range in glyco-density from 2 to 18 for the attachment of azido manno-pyranoside derivatives the application of a click cycloaddition using a copper catalyst. The effects of the manno-pyranosides on the kinetics of binding have been evaluated using these compounds. Different scaffolds have been used to build the mannosylated dendrons in an effort to extend and combine the “onion peel” technique of those glycosides. By preventing the growth of bacterial biofilms, it was designed to combat E. coli urinary infections. As a result, the herbal multiantennary oligomannosides of the uroplakin receptors expressed on uroepithelial tissues are not able to bind to the FimH-type lectin gifted on the tip of their fimbriae. Initial DLS research on the mannosylated dendrimers to go hyperlink the leguminous lectin Con A utilized as a model revealed their excessive effectiveness as candidates to prevent the adhesion and biofilm formation of E. coli. are shown in Fig. 26b.82
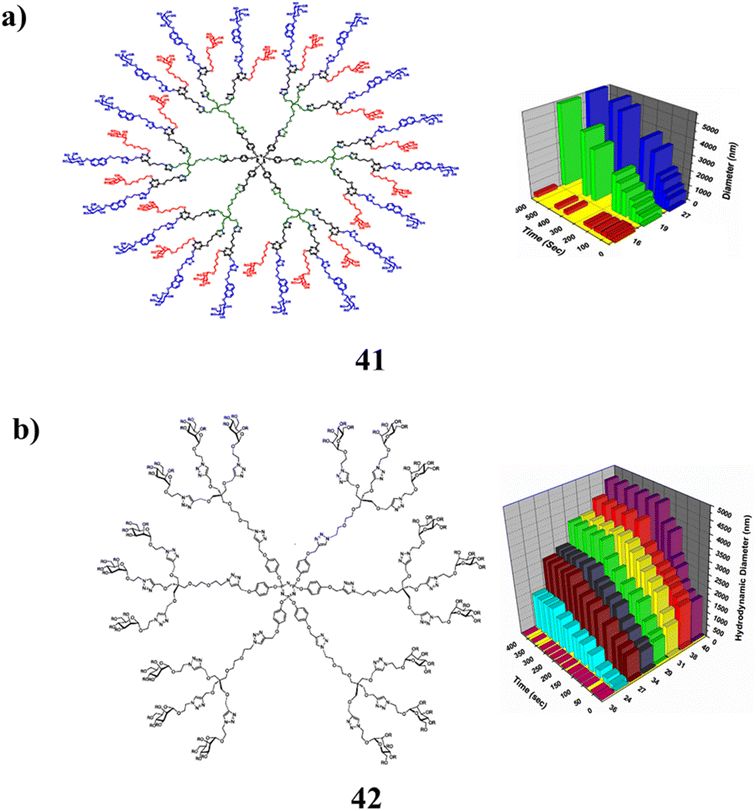 |
| Fig. 26 (a) Structure of hetero-layered hybrid glyco-dendrimer (41). (b) Chemical structure of mannosylated dendrimer (42). | |
The cationic polymer (43) with sugar is a possible carrier of nucleic acids both invitro and invivo. For environmentally friendly and biocompatible transport of short interfering RNA, they have been chemically coupled to several materials of synergistic poly-lysine dendrimer structures (siRNA) (Fig. 27a). The beneficial effects on cells are significantly reduced by the synergistic dendrimers, which also comprise lipid-attached glucose terminalized lysine dendrimers while retaining effective mobile access. Additionally, the synergistic dendrimers complexed to siRNA caused RNA interference (RNAi) within the cells and severely shut down the endogenously expressed disease-related gene Plk1 as well as green fluorescence protein (GFP). It might be a technique for effective and biocompatible siRNA delivery.83 Maltose-changed PPI dendrimers (44) are also glyco-nanoparticles as a result of their unmodified congeners dramatic increase in biocompatibility. However, as demonstrated by the discovery of PPI-m G4 dendrimers inside the fully modified dense shell variation stimulate the NF-κB pathway in a cell version of monocytes – the traditional effectors of innate immunity (Fig. 27b). The expression of genes regulated by this pathway is moderately elevated as a result of the enhanced NF-κB transcriptional activity. When pro-inflammatory stimuli are applied this induction is not accompanied by substantial activation and does not promote the release of pro-inflammatory cytokines in cells. Sensitive signalling homeostasis is present in myeloid cells. Its ability to interact and communicate with various immunomodulatory stimuli is a significant discovery that opens up both potential in vivo study paths for dendrimer application as clinical retailers and cautions for systemic dendrimer use as hypothetically bio-orthogonal drug delivery vehicles.84
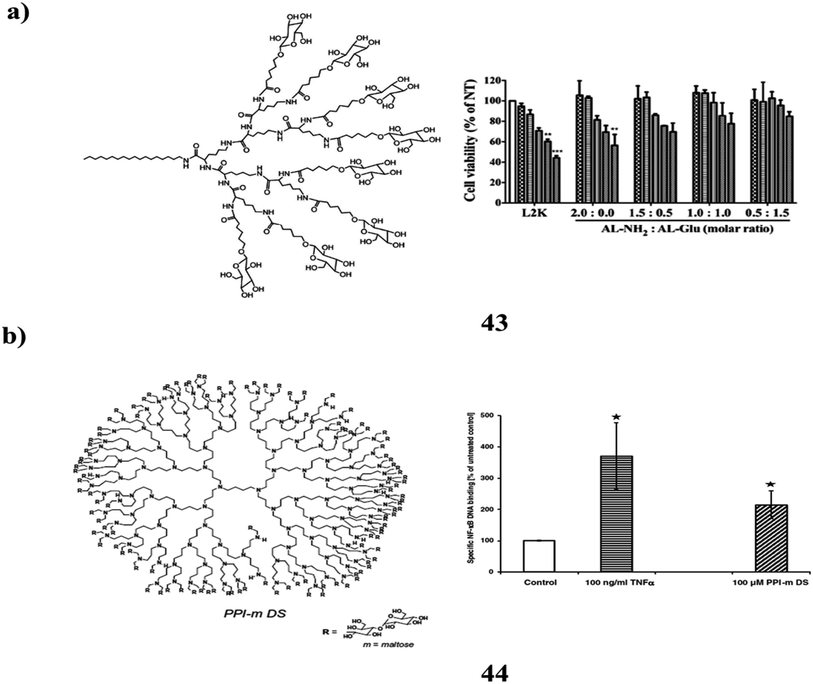 |
| Fig. 27 (a) Sugar functionalized synergistic dendrimers for biocompatible delivery of nucleic acid therapeutics (43). (b) Structure of maltose-changed PPI dendrimers (44). | |
A chain of dendrimers including amino-sugar and amino-polyol was also present in the solid dendrimer cores G0 (45). Using NMR combined with 2D NMR spectroscopy and MALDI-TOF mass spectrometry, the composition of the response aggregate is determined. In order to identify typical fragmentation patterns of [M + H]+ ions, fragmentation studies were carried out in positive ion mode are shown in Fig. 28.85–87
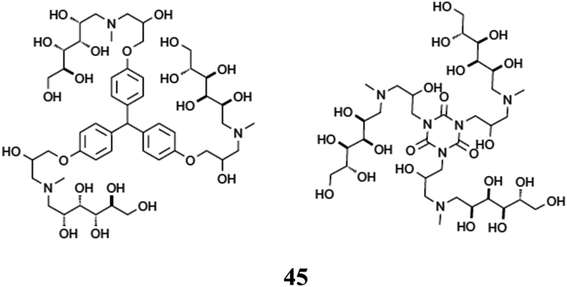 |
| Fig. 28 Structure of G0 amino-polyol and aminosugar dendrimers (45). | |
A summary of selected types of dendrimers and applications referenced is found in Table 1 below.
Table 1 Summary of types of dendrimers, and applications involved in this review
Types of chromophores |
Applications |
Ref. |
(S)-BINOL-based pyrene ester dendrimer |
Dye-sensitized solar cells |
48 |
Fluorescent coumarin dendrimer |
Drug-delivery |
62 |
Perylenediimide cored dendrimer |
Cellular receptors |
65 |
Polyphenylene dendrimers |
Drug delivery |
68 |
Perylenediimide-naphthalimide dendrimer |
HeLa cells |
69 |
RhB decorated dendrimer |
Antibacterial activity |
72 |
RhB (S)-BINOL cored triazole bridged dendrimer |
Anticancer activity |
74 |
Fluorescent RhB PAMAM dendrimer |
Biomedical |
76 |
Maltose-changed PPI dendrimers |
Drug delivery |
84 |
7. Conclusion
In this focused review, the photoresponsive and glycoside dendrimers have a wide variety of structural and functional characteristics. Indeed, a number of fluorescent groups can serve as the core, terminal groups, or branches. Some dendrimers have even been discovered to possess intrinsic fluorescence properties. Most of these compounds were prepared for purely theoretical purposes, such as investigating the dendritic structure that affects fluorescence characteristics, particularly the potential for internal FRET, determining the structural permeability of the structure, or investigating the development of thin films or self-assemblies. They were also specifically designed for biological applications, including photon imaging and studying the biological mechanisms involved in their activation. Furthermore, the scientists have more recognition of dendrimers themselves, structural and functional groups can be incorporated into dendrimers in specific positions. It may allow the chemist a great deal of control over the dendritic architecture and functionality, which may enhance certain peripheral group functions. Future studies on dendrimers are still required since more needs to be done to make the functionalization of dendrimers more economically viable.
Abbreviations
NMR | Nuclear magnetic resonance |
DLS | Dynamic light scattering |
MALDI-TOF MS | Matrix-assisted laser desorption ionization time of flight mass spectrometry |
FRET | Fluorescence resonance energy transfer |
PAMAM | Polyamidoamine |
DSC | Differential scanning calorimetry |
NACs | Nitro aromatic compounds |
BINOL | 1,1′-Bi-2-naphthol |
PCZ | 9-Phenylcarbazole |
DMSO | Dimethyl sulfoxide |
SPC | Single photon counting fluorescence |
PL | Photoluminescence |
HOMO | Highest occupied molecular orbital |
AEEE | Aggregation enhanced excimer emission |
TDDFT | Time dependent density functional theory |
PPDs | Polyphenylene dendrimers |
UV/Vis | Ultraviolet-visible |
CDD | Charge different density |
LUMO | Lowest unoccupied molecular orbital |
DCM | Dichloromethane |
OLEDs | Organic light emitting diodes |
FLISA | Fluorescence linked immunosorbent assay |
NIR | Near-infrared |
BHC | 4-Bromo-7-hydroxycoumarin |
CPN | Conjugated polymer network |
AB | Azobenzene |
PDI | Perylenetetracarboxdiimide |
HRMS | High resolution mass spectrometry |
DPPH | 1,1-Diphenyl-2-picryl hydrazyl |
RNA | Ribonucleic acid |
SiRNA | Small interfering RNA |
HCl | Hydrochloric acid |
UPEC | Uropathogenic Escherichia coli |
CuAAc | Cu(I) catalyzed azide–alkyne[1,3]-dipolar cycloaddition |
MTT | 3-(4,5-Dimethylthiazol-2-yl)-2,5-diphenyl tetrazolium bromide |
ESI-MS | Electrospray ionisation mass spectrometry |
SSF | Steady state fluorescence |
BHT | Butylated hydroxy toluene |
EFG | Ends functional group |
TFG | Terminal functional group |
Conflicts of interest
There are no conflicts to declare.
Acknowledgements
M. R. thanks Prof. T. Sasiprabha, Vice-chancellor, Sathyabama Institute of Science and Technology (Deemed to be University), for her encouragement.
References
- E. Buhleier, W. Wehner and F. Vogtle, “Cascade”- and “Nonskid-Chain-like” Syntheses of Molecular Cavity Topologies, Synthesis, 1978, 1978, 155 CrossRef.
- D. A. Tomalia, A. M. Naylor and W. A. Goddard, Starburst Dendrimers: Molecular-Level Control of Size, Shape, Surface Chemistry, Topology, and Flexibility from Atoms to Macroscopic Matter, Angew. Chem., Int. Ed. Engl., 1990, 29, 138 CrossRef.
- D. A. Tomalia, H. Baker, J. Dewald, M. Hall, G. Kallos, S. Martin, J. Roeck, J. Ryder and P. Smith, A New Class of Polymers: Starburst-Dendritic Macromolecules, Polym. J., 1985, 17, 117 CrossRef CAS.
- G. R. Newkome, Z. Yao, G. R. Baker and V. K. Gupta, Micelles. Part 1. Cascade molecules: a new approach to micelles. A [27]-arborol, J. Org. Chem., 1985, 50, 2003 CrossRef CAS.
- A. S. Abd-El-Aziz, A. A. Abdelghani, B. D. Wagner and R. Bissessur, Advances in Light-Emitting Dendrimers, Macromol. Rapid Commun., 2019, 40, 1800711 CrossRef PubMed.
- H. M. E. Azzazy, A. M. Sawy, A. Abdelnaser, M. R. Meselhy, T. Shoeib and S. A. Fahmy, Peganum harmala Alkaloids and Tannic Acid Encapsulated in PAMAM dendrimer: Improved Anticancer Activities as Compared to Doxorubicin, ACS Appl. Polym. Mater., 2022, 4, 7228–7239 CrossRef CAS.
- C. Morfill, S. Pankratova, P. Machado, N. K. Fernando, A. Regoutz, F. Talamona, A. Pinna, M. Klosowski, R. J. Wilkinson, R. A. Fleck, F. Xie, A. E. Porter and D. Kiryushko, Nanostars Carrying Multifunctional Neurotrophic dendrimer Protect Neurons in Preclinical In Vitro Models of Neurodegenerative Disorders, ACS Appl. Mater. Interfaces, 2022, 14, 47445–47460 CrossRef CAS PubMed.
- R. M. England, S. Sonzini, D. Buttar, K. E. Treacher and M. B. Ashford, Investigating the properties of l-lysine dendrimers through physico-chemical characterisation techniques and atomistic molecular dynamics simulations, Polym. Chem., 2022, 13, 2626–2636 RSC.
- D. Dhumal, B. Maron, E. Malach, Z. Lyu, L. Ding, D. Marson, E. Laurini, A. Tintaru, B. Ralahy, S. Giorgio, S. Pricl, Z. Hayouka and L. Peng, Dynamic self-assembling supramolecular dendrimer nanosystems as potent antibacterial candidates against drug-resistant bacteria and biofilms, Nanoscale, 2022, 14, 9286–9296 RSC.
- Y. Feng, Z. X. Liu, H. Chen and Q. H. Fan, Functional supramolecular gels based on poly(benzyl ether) dendrons and dendrimers, Chem. Commun., 2022, 58, 8736–8753 RSC.
- J. W. Strasser and R. M. Crooks, Single atoms and small clusters of atoms may accompany Au and Pd dendrimer-encapsulated nanoparticles, Soft Matter, 2022, 18, 5067–5073 RSC.
- D. P. Poudel and R. T. Taylor, Low-generation fluorescent polyurethane dendrimers via late-stage modification using azide–alkyne click chemistry, RSC Adv., 2022, 12, 28043–28051 RSC.
- L. G. Reventlow, M. Koodalingam, C. Siebert, P. Marlow, E. V. Puttock, P. L. Burn and A. Colsmann, Influence of chromophore spacing on the stability and efficiency of host-free sky-blue dendrimer organic light emitting diodes, J. Mater. Chem. C, 2022, 10, 8278–8287 RSC.
- K. Albrecht, M. Taguchi, T. Tsukamoto, T. Moriai, N. Yoshida and K. Yamamoto, Poly-phenylene jacketed tailor-made dendritic phenylazomethine ligand for nanoparticle synthesis, Chem. Sci., 2022, 13, 5813–5817 RSC.
- L. Zhang, S. Hu, L. Zhang, W. Wu, Q. Cheng, J. Li and R. Narain, Synergistic size and charge conversions of functionalized PAMAM dendrimers under the acidic tumor microenvironment, Biomater. Sci., 2022, 10, 4271–4283 RSC.
- C. Li, L. Zhao, L. Jia, Z. Ouyang, Y. Gao, R. Guo, S. Song, X. Shi and X. Cao, 68Ga-labeled dendrimer-entrapped gold nanoparticles for PET/CT dual-modality imaging and immunotherapy of tumors, J. Mater. Chem. B, 2022, 10, 3648–3656 RSC.
- M. Sowinska and Z. Urbanczyk-Lipkowska, Advances in the Chemistry of Dendrimers, New J. Chem., 2014, 38, 2168–2203 RSC.
- J. M. J. Frechet, Dendrimers and Other Dendritic Macromolecules: From, J. Polym. Sci., Part A: Polym. Chem., 2003, 41, 3713–3725 CrossRef CAS.
- S. S. Gillani, M. A. Munawar, K. M. Khan and J. A. Chaudhary, Synthesis, Characterization, and Applications of Poly-Aliphatic Amine Dendrimers and Dendrons, J. Iran. Chem. Soc., 2020, 17, 2717–2736 CrossRef CAS.
- R. M. Crooks, B. I. Lemon, L. Sun, L. K. Yeung and M. Zhao, Dendrimer-Encapsulated Metals and Semiconductors: Synthesis, Characterization, and Applications, Top. Curr. Chem., 2001, 212, 81–135 CrossRef CAS.
- L. M. Bronstein and Z. B. Shifrina, Dendrimers as Encapsulating, Stabilizing, or Directing Agents for Inorganic Nanoparticles, Chem. Rev., 2011, 111, 5301–5344 CrossRef CAS PubMed.
- G. R. Newkome and C. N. Moorefield, From 1 → 3 Dendritic Designs to Fractal Supramacromolecular Constructs: Understanding the Pathway to the Sierpiński Gasket, Chem. Soc. Rev., 2015, 44, 3954–3967 RSC.
- S. Palchoudhury, Z. Zhou, K. Ramasamy, F. Okirie, P. E. Prevelige and A. Gupta, Self-Assembly of P22 Protein Cages with Polyamidoamine Dendrimer and Inorganic Nanoparticles, J. Mater. Res., 2017, 32, 465–472 CrossRef CAS.
- I. N. Kurniasih, J. Keilitz and R. Haag, Dendritic Nanocarriers Based on Hyperbranched Polymers, Chem. Soc. Rev., 2015, 44, 4145–4164 RSC.
- A. M. Caminade, D. Yan and D. K. Smith, Dendrimers and Hyperbranched Polymers, Chem. Soc. Rev., 2015, 44, 3870–3873 RSC.
- M. V. Walter and M. Malkoch, Simplifying the Synthesis of Dendrimers: Accelerated Approaches, Chem. Soc. Rev., 2012, 41, 4593–4609 RSC.
- K. Madaan, S. Kumar, N. Poonia, V. Lather and D. Pandita, Dendrimers in Drug Delivery and Targeting: Drug-Dendrimer Interactions and Toxicity Issues, J. Pharm. BioAllied Sci., 2014, 6, 139–150 CrossRef PubMed.
- L. Wang and X. He, Investigation of AB n (n = 2, 4) Type Hyperbranched Polymerization with Cyclization and Steric Factors: Influences of Monomer Concentration, Reactivity, and Substitution Effect, J. Polym. Sci., Part A: Polym. Chem., 2009, 47, 523–533 CrossRef CAS.
- D. A. Tomalia, H. Baker, J. Dewald, M. Hall, G. Kallos, S. Martin, J. Roeck, J. Ryder and P. A. Smith, New Class of Polymers: Starburst-Dendritic Macromolecules, Polym. J., 1985, 17, 117–132 CrossRef CAS.
- D. A. Tomalia, H. Baker, M. Hall, G. Kallos, S. Martin, J. Ryder and P. Smith, Dendritic Macromolecules:1 Synthesis of Starburst Dendrimers, Macromolecules, 1986, 19, 2466–2468 CrossRef CAS.
- C. J. Hawker and J. M. J. Frechet, Preparation of Polymers with Controlled Molecular Architecture. A New Convergent Approach to Dendritic Macromolecules, J. Am. Chem. Soc., 1990, 112, 7638–7647 CrossRef CAS.
- M. Hardouin-Lerouge, P. Hudhomme and M. Salle, Molecular Clips and Tweezers Hosting Neutral Guests, Chem. Soc. Rev., 2011, 40, 30–43 RSC.
- V. Balzani, H. Bandmann, P. Ceroni, C. Giansante, U. Hahn, F. G. Klärner, U. Muller, W. M. Muller, C. Verhaelen and V. Vicinelli, Host-Guest Complexes between an Aromatic Molecular Tweezer and Symmetric and Unsymmetric Dendrimers with a 4,4′-Bipyridinium Core, J. Am. Chem. Soc., 2006, 128, 637–648 CrossRef CAS PubMed.
- V. Percec, M. R. Imam, T. K. Bera, V. S. K. Balagurusamy, M. Peterca and P. A. Heiney, Self-Assembly of Semifluorinated Janus-Dendritic Benzamides into Bilayered Pyramidal Columns, Angew. Chem., 2005, 117, 4817–4823 CrossRef.
- J. G. Hardy, A. R. Hirst, D. K. Smith, C. Brennan and I. Ashworth, Controlling the Materials Properties and Nanostructure of a Single-Component Dendritic Gel by Adding a Second Component, Chem. Commun., 2005, 2, 385–387 RSC.
- W. Ong, M. Gómez-Kaifer and A. E. Kaifer, Dendrimers as Guests in Molecular Recognition Phenomena, Chem. Commun., 2004, 4, 1677–1683 RSC.
- D. A. Tomalia, Dendritic Effects: Dependency of Dendritic Nano-Periodic Property Patterns on Critical Nanoscale Design Parameters (CNDPs), New J. Chem., 2012, 36, 264–281 RSC.
- H. F. Chow, C. F. Leung, G. X. Wang and Y. Y. Yang, Dendritic Effects in Functional Dendrimer Molecules, Compt. Rendus Chem., 2003, 6, 735–745 CrossRef CAS.
- B. Helms and J. M. J. Fréchet, The Dendrimer Effect in Homogeneous Catalysis, Adv. Synth. Catal., 2006, 348, 1125–1148 CrossRef CAS.
- Y. Cheng and C. Burda, Nanoparticles for Photodynamic Therapy, Compr. Nanosci. Nanotechnol., 2011, 5, 1–28 Search PubMed.
- S. B. Undre, M. Singh, R. K. Kale and M. Rizwan, Silibinin Binding and Release Activities Moderated by Interstices of Trimesoyl, Tridimethyl, and Tridiethyl Malonate First-Tier Dendrimers, J. Appl. Polym. Sci., 2013, 130, 3537–3554 CrossRef CAS.
- M. E. M. Klimov, U. O. Mateos, A. B. Miranda, M. Rivera, O. A. Sarria and M. M. Garcia, Electrical Properties of Multi-Pyrene/Porphyrin-Dendrimers, Molecules, 2015, 20, 17533–17543 CrossRef PubMed.
- A. C. Vallejo, M. Vonlanthen, P. Porcu, A. Ruiu and E. Rivera, New cyclen-cored dendrimers functionalized with pyrene: synthesis characterization, optical and photophysical properties, Tetrahedron Lett., 2017, 58, 1319–1323 CrossRef.
- M. Vonlanthen, J. G. Ortega, P. Porcu, A. Ruiu, E. R. Alba, A. C. Vallejo and E. Rivera, Pyrene-labeled dendrimers functionalized with fullerene C60 or porphyrin core as light harvesting antennas, Synth. Met., 2018, 245, 195–201 CrossRef CAS.
- P. K. Lekha and E. Prasad, Tunable Emission of Static Excimer in a Pyrene-Modified Polyamidoamine Dendrimer Aggregate through Positive Solvatochromism, Chem.–Eur. J., 2011, 117, 8609–8617 CrossRef PubMed.
- M. Gingras, V. Placide, J. M. Raimundo, G. Bergamini, P. Ceroni and V. Balzani, Polysulfurated Pyrene-Cored Dendrimers: Luminescent and Electrochromic Properties, Chem.–Eur. J., 2008, 14, 10357–10363 CrossRef CAS PubMed.
- H. A. Alidagi, S. O. Tümay, A. Şenocak, O. F. Çiftbudak, B. Çoşut and S. Yeşilot, Constitutional isomers of dendrimer-like pyrene substituted cyclotriphosphazenes: synthesis, theoretical calculations, and use as fluorescence receptors for the detection of explosive nitroaromatics, New J. Chem., 2019, 43, 16738–16747 RSC.
- P. Rajakumar and K. Visalakshi, Synthesis and Photophysical Studies of S-(-)-1,1′-Binaphthol-Based Pyrene- Terminated Dendrimers, Synth. Commun., 2013, 1, 2226–2235 CrossRef.
- P. Rajakumar, K. Visalakshi, S. Ganesan, P. Maruthamuthu and S. A. Suthanthiraraj, Synthesis of Pyrene-Based Ester Dendrimers for Applications in Dye-Sensitized Solar Cells, Bull. Chem. Soc. Jpn., 2012, 85, 902–911 CrossRef CAS.
- Z. Zhang, W. Tan, R. Zhang, S. Guan, J. Miao and M. Zhang, A dendrimer consisting of a pyrene core and a 9-phenylcarbazole periphery as a multi-functional fluorescent probe for iodide, iron(III) and mercury(II), Microchim. Acta, 2019, 186, 586 CrossRef PubMed.
- A. Ruiu, M. Vonlanthen, S. M. R. Montoya, I. G. Méndez and E. Rivera, Unusual Fluorescence Behavior of Pyrene-Amine Containing Dendrimers, Molecules, 2019, 24, 4083 CrossRef CAS PubMed.
- S. Bhattacharjee, B. Maiti, D. Biswakarma and S. Bhattacharya, Gelation of Novel Pyrene-Cored Chiral Dendrimers: Dendritic Effect in Gelation and Shear Thinning Behavior, Macromol. Symp., 2016, 369, 14–18 CrossRef CAS.
- A. S. A. E. Aziz, A. A. Abdelghani, B. D. Wagner and E. M. Abdelrehim, Aggregation enhanced excimer emission (AEEE) with efficient blue emission based on pyrene dendrimers, Polym. Chem., 2016, 7, 3277–3299 RSC.
- M. Vonlanthen, A. C. Vallejo, E. A. Ortíz, A. Ruiu, P. Porcu and E. Rivera, Synthesis, characterization and photophysical studies of novel pyrene labeled ruthenium (II) trisbipyridine complex cored dendrimers, Polymer, 2016, 99, 13–20 CrossRef CAS.
- J. Wagner, M. Dillenburger, J. Simon, J. Oberlander, K. Landfester, V. Mailander, D. Y. W. N. Klaus Mullen and T. Weil, Amphiphilic dendrimers control protein binding and corona formation on liposome nanocarriers, Chem. Commun., 2020, 56, 8663–8666 RSC.
- L. Yan, Y. Wan, A. Xia, S. H. Lin and R. Huang, Excited-state localization and energy transfer in pyrene core dendrimers with fluorene/carbazole as the dendrons and acetylene as the linkages, Phys. Chem. Chem. Phys., 2016, 18, 4134–4143 RSC.
- S. Kavyani, S. A. Iranagh, M. Dadvar and H. Modarress, Hybrid Dendrimers of PPI(core)-PAMAM(shell): A Molecular Dynamics Simulation Study, J. Phys. Chem. B, 2016, 120, 9564–9575 CrossRef CAS PubMed.
- M. Mao and Q. H. Song, Non-conjugated dendrimers with a porphyrin core and coumarin chromophores as peripheral units: synthesis and photophysical properties, Dyes Pigm., 2012, 92, 975–981 CrossRef CAS.
- N. Prachumrak, S. Potjanasopa, R. Rattanawan, S. Namuangruk, S. Jungsuttiwong, T. Keawin, T. Sudyoadsuk and V. Promarak, Coumarin-cored carbazole dendrimers as solution-processed non-doped green emitters for electroluminescent devices, Tetrahedron, 2014, 70, 6249–6257 CrossRef CAS.
- S. J. Yeo, D. T. Huong, J. H. Han, J. Y. Kim, W. J. Lee, H. J. Shin, E. T. Han and H. Park, Performance of coumarin-derived dendrimer-based fluorescence-linked immunosorbent assay (FLISA) to detect malaria antigen, Malaria J., 2014, 13, 266 CrossRef PubMed.
- N. Fomina, C. L. McFearin and A. Almutairi, Increasing materials' response to two-photon NIR light via self-immolative dendritic scaffolds, Chem. Commun., 2012, 48, 9138–9140 RSC.
- A. Concellon, M. S. Anselmo, S. H. Ainsa, P. Romero, M. Marcos and J. L. Serrano, Micellar Nanocarriers from Dendritic Macromolecules Containing Fluorescent Coumarin Moieties, Polymers, 2020, 12, 2872 CrossRef CAS PubMed.
- F. M. Toma, F. Puntoriero, T. V. Pho, M. La Rosa, Y. S. Jun, B. J. T. Villers, J. Pavlovich, G. D. Stucky, S. Campagna and F. Wudl, Polyimide Dendrimers Containing Multiple Electron Donor-Acceptor Units and Their Unique Photophysical Properties, Angew. Chem., Int. Ed., 2015, 54, 1–6 CrossRef PubMed.
- H. Ren, J. Li, R. Wang, T. Zhang, Z. Gao and D. Liu, Synthesis and luminescent properties of perylene bisimide-cored dendrimers with carbazole surface groups, Polymer, 2011, 52, 3639–3646 CrossRef CAS.
- S. K. Yang, X. Shi, S. Park, S. Doganay, T. Ha and S. C. Zimmerman, Monovalent, Clickable, Uncharged, Water-Soluble Perylenediimide-Cored Dendrimers for Target-Specific Fluorescent Biolabeling, J. Am. Chem. Soc., 2011, 133, 9964–9967 CrossRef CAS PubMed.
- R. Ponnapati, M. J. Felipe and R. Advincula, Electropolymerizable Terthiophene-Terminated Poly(aryl ether) Dendrimers with Naphthalene and Perylene Cores, Macromolecules, 2011, 44, 7530–7537 CrossRef CAS.
- H. Ren, J. Li, T. Zhang, R. Wang, Z. Gao and D. Liu, Synthesis and properties of novel perylenebisimide-cored dendrimers, Dyes Pigm., 2011, 91, 298–303 CrossRef CAS.
- T. T. T. Nguyen, D. Turp, D. Wang, B. Nolscher, F. Laquai and K. Mullen, A Fluorescent, Shape-Persistent Dendritic Host with Photoswitchable Guest Encapsulation and Intramolecular Energy Transfer, J. Am. Chem. Soc., 2011, 133, 11194–11204 CrossRef CAS PubMed.
- H. R. Cheng, X. J. Lu, Y. Zhang and X. Y. Deng, Synthesis and Fluorescence Property of a New Dendritic Perylenediimide-Naphthalimide Derivative, ChemistrySelect, 2018, 3, 3596–3602 CrossRef CAS.
- T. T. T. Nguyen, M. Baumgarten, A. Rouhanipour, H. J. Rader, I. Lieberwirth and K. Mullen, Extending the Limits of Precision Polymer Synthesis: Giant Polyphenylene Dendrimers in the Megadalton Mass Range Approaching Structural Perfection, J. Am. Chem. Soc., 2013, 135, 4183–4186 CrossRef CAS PubMed.
- U. Hahn, J. F. Nierengarten, B. D. Nicot, F. Monti, C. Chiorboli and N. Armaroli, Fullerodendrimers with a perylenediimide core, New J. Chem., 2011, 35, 2234–2244 RSC.
- J. Sathiya Savithri and P. Rajakumar, Synthesis, Photophysical Property and Antibacterial Activity of Rhodamine B Decorated, Mesitylene Cored and Methylene p-Phenoxy Bridged Dendrimers, ChemistrySelect, 2019, 4, 10143–10148 CrossRef.
- J. Sathiya Savithri and P. Rajakumar, Synthesis, Photophysical, and Antioxidant Properties of Rhodamine B Decorated Novel Dendrimers, Aust. J. Chem., 2018, 71, 399–406 CrossRef.
- J. Sathiya Savithri and P. Rajakumar, Synthesis, optical, electrochemical properties and anticancer activity of (S)-BINOL cored triazole bridged dendrimers decorated with rhodamine B surface group, RSC Adv., 2019, 9, 36994–37002 RSC.
- Y. Wei, R. Laurent, J. P. Majoral and A. M. Caminade, Synthesis and characterization of phosphorus-containing dendrimers bearing rhodamine derivatives as terminal groups, ARKIVOC, 2010, 10, 318–327 Search PubMed.
- A. Paolini, L. Leoni, I. Giannicchi, Z. Abbaszadeh, V. Doria, F. Mura, A. D. Cort and A. Masotti, MicroRNAs delivery into human cells grown on 3D-printed PLA scaffolds coated with a novel fluorescent PAMAM dendrimer for biomedical applications, Sci. Rep., 2018, 8, 13888 Search PubMed.
- P. Torre, Q. Xiao, I. Buzzacchera, S. E. Sherman, K. Rahimi, N. Y. Kostina, C. R. Emmenegger, M. Moller, C. J. Wilson, M. L. Klein, M. C. Good and V. Percec, Encapsulation of hydrophobic components in dendrimersomes and decoration of their surface with proteins and nucleic acids, Proc. Natl. Acad. Sci. U.S.A., 2019, 116, 15378–15385 CrossRef CAS PubMed.
- P. Stenstrom, D. Manzanares, Y. Zhang, V. Cena and M. Malkoch, Evaluation of Amino-Functional Polyester Dendrimers Based on Bis-MPA as Nonviral Vectors for siRNA Delivery, Molecules, 2018, 23, 2028 CrossRef PubMed.
- M. Marradi, S. Cicchi, F. Sansone, A. Casnati and A. Goti, Low-generation dendrimers with a calixarene core and based on a chiral C2-symmetric pyrrolidine as iminosugar mimics, Beilstein J. Org. Chem., 2012, 8, 951–957 CrossRef CAS PubMed.
- J. Twibanire, B. Grindley and N. K. Paul, Synthesis of Novel Types of Polyester Glycodendrimers as Potential Inhibitors of Urinary Tract Infections, New J. Chem., 2015, 39, 4115–4127 RSC.
- R. S. Bagul, M. Hosseini, T. C. Shiao, N. K. Saadeh and R. Roy, Hetero-layered Hybrid Dendrimers with Optimized Sugar Head Groups for Enhancing Carbohydrate-Protein Interactions, Polym. Chem., 2017, 8, 5354–5366 RSC.
- C. Sehad, T. C. Shiao, L. M. Sallam, A. Azzouz and R. Roy, Effect of Dendrimer Generation and Aglyconic Linkers on the Binding Properties of Mannosylated Dendrimers Prepared by a Combined
Convergent and Onion Peel Approach, Molecules, 2018, 23, 1890 CrossRef PubMed.
- S. Han, T. Ganbold, Q. Bao, T. Yoshida and H. Baigude, Sugar Functionalized Synergistic Dendrimers for Biocompatible Delivery of Nucleic Acid Therapeutics, Polymers, 2018, 10, 1034 CrossRef PubMed.
- I. J. Pawlik, M. Gorzkiewicz, M. Studzia, D. Appelhans, B. Voit, L. Pulaski and B. K. Maculewicz, Sugar-Modified Poly(propylene imine) Dendrimers Stimulate the NF-κB Pathway in a Myeloid Cell Line, Pharm. Res., 2017, 34, 136–147 CrossRef PubMed.
- M. A. Pyziak, G. Bartkowiak, Ł. Popenda, S. Jurga and G. Schroeder, Synthesis of G0 aminopolyol and aminosugar dendrimers, controlled by NMR and MALDI TOF mass spectrometry, Des. Monomers Polym., 2017, 20, 144–156 CrossRef CAS PubMed.
- A. Ohyama, T. Higashi, K. Motoyama and H. Arima, Ternary complexes of folate-PEG-appended dendrimer (G4)/rmalpha-cyclodextrin conjugate, siRNA and low-molecular-weight polysaccharide sacran as a novel tumor-selective siRNA delivery system, Int. J. Biol. Macromol., 2017, 99, 21–28 CrossRef CAS PubMed.
- M. H. Sleimana, R. Csonka, C. Arbez-Gindre, G. A. Heropoulos, T. Calogeropoulou, M. Signorelli, A. Schiraldi, B. R. Steele, D. Fessas and M. Micha-Screttas, Binding and stabilisation effects of glycodendritic compounds with peanut agglutinin, Int. J. Biol. Macromol., 2015, 80, 692–701 CrossRef PubMed.
|
This journal is © The Royal Society of Chemistry 2022 |
Click here to see how this site uses Cookies. View our privacy policy here.