DOI:
10.1039/C8RA07661H
(Paper)
RSC Adv., 2019,
9, 9253-9259
Poly(arylene ether)s with aromatic azo-coupled cobalt phthalocyanines in the side chain: synthesis, characterization and nonlinear optical and optical limiting properties
Received
14th September 2018
, Accepted 13th March 2019
First published on 21st March 2019
Abstract
A novel poly(arylene ether) with azo-coupled cobalt phthalocyanine in the side chain (PAE-azo-CoPc) was prepared by 1,2-benzodinitrile, anhydrous cobaltous chloride and a novel azobenzene-containing poly(aryl ether) (PAE-azo-DCN) via a nucleophilic substitution polycondensation based on a novel bisfluoro monomer, 4-[(3,4-cyanophenyl)diazenyl]phenyl-2,6-difluorobenzoate. The obtained polymers were characterized and evaluated by FT-IR, 1H NMR, DSC and TGA. PAE-azo-CoPc exhibited higher glass transition temperature and better thermal stability than PAE-azo-DCN because of the introduction of cobalt phthalocyanine groups. The results of Z-scan measurements demonstrated that PAE-azo-CoPc showed reversible saturable absorption and positive refraction, and PAE-azo-DCN showed saturable absorption and negative refraction. By calculation, PAE-azo-CoPc exhibited larger third-order nonlinear optical susceptibilities than that of PAE-azo-DCN. The results of optical limiting measurements demonstrated that the PAE-azo-CoPc exhibited an excellent optical limiting response.
1. Introduction
With the fast development of laser technology, optical limiting materials, which could protect optical sensors and human eyes from harmful laser pulses, have received considerable attention.1–4 Many kinds of optical limiting materials such as carbon nanotubes, graphene, azo-polymer, porphyrins, phthalocyanines and metal nanoparticles have been studied.5–12 A perfect optical limiting material requires fast response time, low limiting threshold, large nonlinear optical susceptibilities and high transmittance for low intensity ambient light.13,14 In addition, excellent mechanical properties and thermal stabilities are also necessary. Up to now, developing ideal optical limiting materials is still a challenge.
Polymers containing push–pull type azobenzene chromophores have received considerable attention due to their large third-order nonlinear susceptibility of the push–pull type azobenzene chromophores.15,16 The push–pull type azobenzene chromophores exhibit excellent third-order nonlinear optical properties because of their conjugate π-electron systems and good fluidity of π electrons. Unless these, the easy processing and good mechanical properties of azo-polymers are also the reason why they have been considered as promising third-order nonlinear optical materials.
Phthalocyanines (Pcs) was one of the most promising optical limiting materials because of their large nonlinear susceptibility.17–19 It is reported that the combination of azobenzene and phthalocyanines possessed the advantages of the two constituents and showed larger nonlinear optical properties than that of phthalocyanines in small molecules.20 However, it is difficult to fabricate optical devices with single phthalocyanines molecule, so many kinds of polymers have been selected as solid state matrices for phthalocyanines.21–23 However, the direct doping of phthalocyanines and polymers usually brings about poor dispersion and aggregation, which could lead to undesirable performance. In addition, most of the polymer matrices are vulnerable to high energy density laser due to their inferior thermal stabilities. Introducing the phthalocyanines into polymers with good thermal stability by covalent bond may be a helpful strategy to solve these problem.24,25
Poly(aryl ether)s (PAEs) are a family of high-performance polymers with excellent thermal and mechanical properties. Functionalized PAEs have received considerable attention due to their potential applications in optical storage materials, fluorescent materials and proton-exchange membrane.26–29 However, only a little nonlinear optical materials and optical limiting materials based on PAEs have been reported,30–32 which need more development and research. In this paper, a novel optical limiting material with good thermal stability was designed and synthesized by introducing azobenzene chromophores and cobalt phthalocyanines into PAEs system. Firstly, azobenzene-containing poly(aryl ether) (PAE-azo-DCN) was prepared via a nucleophilic aromatic substitution polycondensation based on a novel bisfluoro monomer, 4-[(3,4-cyanophenyl)diazenyl]phenyl-2,6-difluorobenzoate. Then, poly(arylene ether)s with aromatic azo-coupled cobalt phthalocyanines in the side chain (PAE-azo-CoPc) was prepared by PAE-azo-DCN, 1,2-benzodinitrile and cobaltous chloride anhydrous. The obtained polymers were characterized and evaluated by UV-vis, 1H NMR, DSC and TGA. The third-order optical properties of PAE-azo-DCN and PAE-azo-CoPc were evaluated by Z-scan. And the optical limiting property of PAE-azo-CoPc was also investigated.
2. Materials
2.1. Materials
4-Aminophthalonitrile was purchased from Meryer (Shanghai) Chemical Technology Co., Ltd. 2,6-Difluorobenzoyl chloride was purchased from Ruiding Chemicals Co., Ltd. 4,4′-(Hexafluoroisopropylidene)diphenol (bisphenol AF) was purchased from Tianjin Heowns Biochemical Technology Co., Ltd. Cobalt phthalocyanine, cobalt(II) chloride, triethylamine and 1,2-dicyanobenzene were purchased from Aladdin Chemistry Co. Ltd. All the solvents were purchased from commercial sources.
2.2. Measurements
1H NMR spectra were recorded on a Mercury-Vx300-NMR instrument using DMSO-d6 as solvent, and the chemical shifts (δ) were given in ppm using TMS as internal reference. FI-IR spectra (KBr pellet) were recorded on a Perkin Elmer Spectre one FT-IR spectrophotometer. UV-visible absorption spectra were recorded on a Shimadzu UV-2501 UV-vis spectrophotometer. Glass transition temperatures (Tgs) were determined by a Model Mettler DSC instrument under nitrogen atmosphere at a heating rate of 20 °C min. Thermo-gravimetric analysis was performed on a Perkin Elmer Pyris 1 TGA analyzer with a heating rate of 10 °C min and under nitrogen atmosphere.
The nonlinear optical property of the sample was evaluated by a Z-scan technique performed using a Q-switched ns Nd:YAG laser system continuum with pulse width of 5 ns at 10 Hz repetition rate and 532 nm wavelength. The solution of the sample was contained in a 2 mm quartz cell and moved along the axis of the incident beam (z direction). The incident and transmitted energies were detected simultaneously by an energy meter (Laser Probe, Rj-7620 ENERGY RATIOMETER, RjP-735). The optical limiting property was performed with the same Z-scan technique system as in the nonlinear absorption experiments, where the intensity of the incident energy is varied continuously and the output energy is captured by a large aperture photodetector. The input energy was 16.5 μJ.
2.3. Synthesis
2.3.1. Synthesis of 4-((3,4-dicyanophenyl)diazenyl)phenol (monomer 1) (monomer 1, Scheme 1). Monomer 1 was synthesized as follow. Hydrochloric acid (0.4 mol, 33.6 mL) was added into the mixture of 4-aminophthalonitrile (14.315 g, 0.1 mol) and 300 mL water. Then an aqueous solution of NaNO2 (6.9 g, 0.1 mol) was added at 0–5 °C. The obtained solution was filtered and added into a mixture of NaOH (8.0 g, 0.2 mol), phenol (9.4 g, 0.1 mol), and NaHCO3 (25.2 g, 0.3 mol) in 200 mL water. After the obtained mixture was stirred at 0–5 °C for 1 h, hydrochloric acid (0.4 mol, 33.6 mL) was added into the final mixture. The resulting precipitate was collected and dried. The crude product was recrystallized from ethanol (yield: 58%). IR (KBr, cm−1): 2239 (–CN), 3327 (–OH); 1H NMR (DMSO-d6, δ, ppm): 10.75 (s, 1H), 8.44 (d, 1H), 8.30 (d, 1H), 8.21 (d, 1H), 7.89 (d, 2H), 6.99 (d, 2H).
2.3.2. 4-((3,4-Dicyanophenyl)diazenyl)phenyl-2,6-difluorobenzoate (monomer 2, Scheme 1). Under N2 atmosphere, a solution of 2,6-difluorobenzoyl chloride (8.8275 g, 0.05 mol) in 30 mL CH2Cl2 was added dropwise into a mixture of monomer 1 (12.4125 g, 0.05 mol) and triethylamine (5 mL) in 80 mL CH2Cl2. Then the mixture was refluxed for 20 h. The resulting insoluble inorganic salt was removed by filtering. After evaporating the filtrate, the crude product was recrystallized from toluene, and the monomer 2 was obtained as red crystal (yield: 67%). IR (KBr, cm−1): 2231 (–CN), 1749 (–CO–); 1H NMR (DMSO-d6, δ, ppm): 8.60 (s, 1H), 8.39 (d, 1H), 8.34 (d, 1H), 8.12 (d, 2H), 7.81–7.83 (m, 1H), 7.62 (d, 2H), 7.38 (t, 2H).
2.3.3. Synthesis of azobenzene-containing poly(aryl ether) (PAE-azo-DCN, Scheme 2). Bisphenol AF (3.3623 g, 0.01 mol), monomer 2 (3.8843 g, 0.01 mol), K2CO3 (1.5201 g, 0.011 mol), DMAc (22 mL) and toluene (10 mL) were put into a three-necked flask. The reaction mixture was refluxed at 130 °C for 3 h to ensure complete dehydration. After removal of toluene, the reaction mixture was heated at 150–160 °C for 8 h. After being poured into the deionized water, the precipitate was collected and washed with hot water and ethanol several times. The resulting product was dried at 80 °C under vacuum for 24 h and PAE-azo-DCN was obtained as orange powder. The yield was 83%.
2.3.4. Azobenzene-containing poly(arylene ether)s with phthalocyanine cobalt(II) in the side chain (PAE-azo-CoPc, Scheme 2). The synthetic procedure of PAE-azo-CoPc was shown as follows. For PAE-azo-DCN (0.5205 g, 0.002 mol), 1,2-dicyanobenzene (0.436 g, 0.006 mol), CoCl2 (0.1546 g, 0.002 mol) and quinoline (20 mL) were put into a three-necked flask. The mixture was heated at 210 °C for 8 h under nitrogen atmosphere. After being poured into methanol, the precipitate was collected by filtration. The crude product was washed with acetone, ethanol and deionized water. Then the crude product was collected by filtration and extracted by chloroform with a Soxhlet extractor. The chloroform solution was concentrated and precipitated in methanol. The resulting product was dried at 100 °C under vacuum for 24 h and PAE-azo-CoPc was obtained.
3. Results and discussion
3.1. Synthesis and characterization
We designed a new bisfluoro monomer (monomer 2) containing azobenzene group and the synthetic route of the monomer is illustrated in Scheme 1. The structure of monomer 2 was confirmed by IR and 1H NMR, as shown in Fig. 1 and 2. The IR spectrum showed the characteristic bands of –CN, C–F and –CO– stretching vibrations at 2231, 1013 and 1748 cm−1, respectively. It also could be observed the characteristic bands of –C
C– of benzene at 1624 cm−1 and 1588 cm−1. In the 1H NMR spectrum of monomer 2, all of the signals are well agreement with the expected structure.
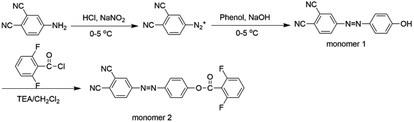 |
| Scheme 1 Synthetic route of monomer 1 and monomer 2. | |
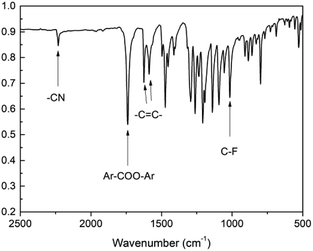 |
| Fig. 1 IR (KBr) spectrum of monomer 2. | |
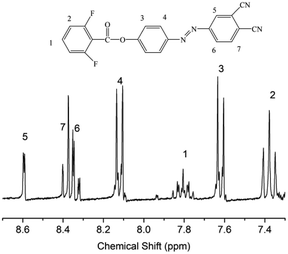 |
| Fig. 2 1H NMR spectrum of monomer 2 in DMSO-d6. | |
PAE-azo-DCN and PAE-azo-CoPc were synthesized as shown in Scheme 2. From Table 1, it could be seen that both PAE-azo-DCN and PAE-azo-CoPc had number average molecular weights above 1 × 104 g mol−1. Both PAE-azo-DCN and PAE-azo-CoPc showed good solubility in common organic solvents such as tetrahydrofuran, cyclohexanone, dimethylacetamide, N,N-dimethylformamide and N-methyl-2-pyrrolidone.
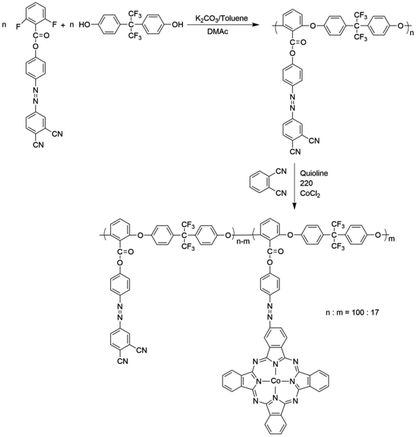 |
| Scheme 2 Synthesis routes to PAE-azo-DCN and PAE-azo-CoPc. | |
Table 1 Properties of PAE-azo-DCN and PAE-azo-CoPc
Polymer |
Mn |
Mw/Mn |
Tga (°C) |
Td5b (°C) |
Glass transition temperature by DSC. 5% weight-loss temperatures were detected at a heating rate of 10 °C min−1 in nitrogen. |
PAE-azo-DCN |
1.01 × 104 |
1.5 |
158 |
381 |
PAE-azo-CoPc |
1.05 × 104 |
2.1 |
164 |
387 |
The chemical structures of PAE-azo-DCN and PAE-azo-CoPc were confirmed by 1H NMR and UV-vis spectra. Fig. 3 shows typical 1H NMR spectra of PAE-azo-DCN and PAE-azo-CoPc in DMSO-d6 with signal assignment. Compared to the 1H NMR spectrum of PAE-azo-DCN, it was observed that the signals of protons a and b appear at δ 9.28 and 8.87 ppm in the spectrum of PAE-azo-CoPc. The grafting ratio of the phthalocyaninecobalt unites is about 17% as estimated from the NMR characterization. The UV-vis spectra of PAE-azo-DCN and PAE-azo-CoPc in DMF solution are shown in Fig. 4. As shown in Fig. 4, the characteristic absorption bands at around 360 nm could be observed which corresponded to π–π* transitions resulted from the intramolecular charge transfer of the azobenzene groups. Compared to the UV-vis spectrum of PAE-azo-DCN, the UV-vis spectrum of PAE-azo-CoPc showed a new peak at around 664 nm corresponding to the characteristic absorption bands of phthalocyaninecobalt unites.
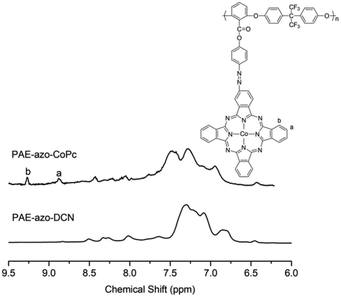 |
| Fig. 3 1H NMR spectra of PAE-azo-DCN and PAE-azo-CoPc. | |
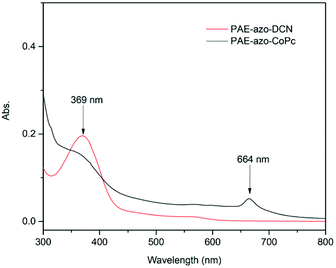 |
| Fig. 4 UV-vis spectra of PAE-azo-DCN and PAE-azo-CoPc in DMF solution. | |
3.2. Thermal properties of PAE-azo-DCN and PAE-azo-CoPc
Fig. 5 shows the DSC curves of the polymers, and the corresponding experimental data are listed in Table 1. Due to the aromatic structure, both PAE-azo-DCN and PAE-azo-CoPc showed high Tgs. Compared to the Tg of PAE-azo-DCN, Tg of PAE-azo-CoPc increased from 158 °C to 163 °C after the introduction of phthalocyaninecobalt groups. Fig. 6 shows the TGA analysis curves of PAE-azo-DCN and PAE-azo-CoPc, and the detailed experimental data from the TGA analysis are also listed in Table 1. From Fig. 6 and Table 1, it could be observed that both of the 5% weight loss (Td5) of PAE-azo-DCN and PAE-azo-CoPc were above 380 °C, indicating their good thermal stability. Compared to Td5 of PAE-azo-DCN, Td5 of PAE-azo-CoPc increased from 381 °C to 387 °C after the introduction of phthalocyaninecobalt groups. Compared to the other azo-polymer optical limiting materials,33–36 both of PAE-azo-DCN and PAE-azo-CoPc showed better thermal stabilities, which could be attributed to the aromatic rigid structure of poly(arylene ether)s and phthalocyanines.
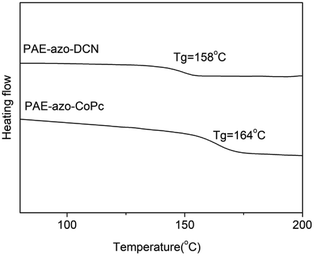 |
| Fig. 5 DSC curves of PAE-azo-DCN and PAE-azo-CoPc. | |
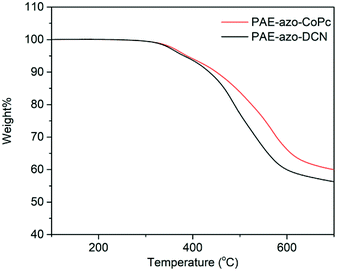 |
| Fig. 6 TGA curve of PAE-azo-DCN and PAE-azo-CoPc in nitrogen. | |
3.3. Transparency of the films of the polymers
To verify the feasibility of the material application, the films of the polymers was prepared as follow. The polymers were dissolved in dried DMF (10 wt%). After stirring for 1 h, the solution was filtered through 0.45 μm syringe filter membranes. The films were obtained via spin-coating the solution onto clean silica glass substrates. After drying under vacuum for 24 h, the films were obtained. The transparency of the films was measured with a Shimadzu UV-2501 UV-vis spectrophotometer. The films were about 5 μm thick. As shown Fig. 7, the films of the polymers showed good transmittance (above 40%) at 450–800 nm.
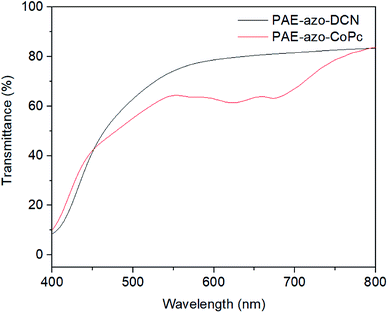 |
| Fig. 7 The transmittance (%) of the films of the polymers. | |
3.4. Nonlinear optical properties
The nonlinear absorption coefficients of the polymers in DMF were measured using Z scan technique. As shown in Fig. 8, the results of Z scan with and without an aperture indicated that all the polymers had both nonlinear absorption and refraction. Thus, the third-order nonlinear optical susceptibilities χ(3) of the polymers should be attributed to nonlinear absorption (α2) and refractive (n2). The nonlinear absorption coefficient α2 of the polymers can be determined by the experimental data based on eqn (1) and (2): |
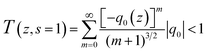 | (1) |
|
q0(z) = α2I0(t)Leff/(1+z2 + z02)
| (2) |
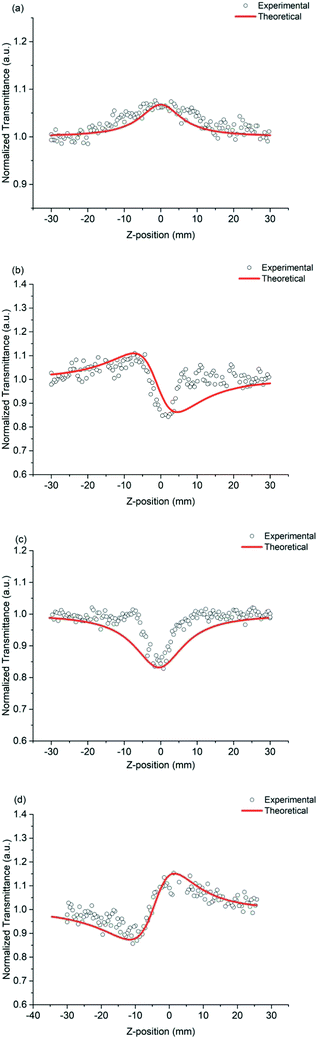 |
| Fig. 8 Z-scan data of (a) PAE-azo-DCN (open aperture), (b) PAE-azo-DCN (close aperture), (c) PAE-azo-CoPc (open aperture), and (d) PAE-azo-CoPc (close aperture). | |
In the equation, I0(t) was the intensity of laser beam at focus (z = 0); Leff = [1 − exp(−α0L)]/α0 was the effective thickness; α0 was the linear absorption coefficient; L was the sample thickness; z was the sample position; z0 was the diffraction length of the beam. The curves in Fig. 8(a) and (c) are obtained from eqn (1) and (2). As shown in Table 2, the nonlinear absorption coefficient α2 of PAE-azo-DCN was −6 × 10−11 esu which corresponded to saturable absorption, and the nonlinear absorption coefficient α2 of PAE-azo-CoPc was 2 × 10−10 esu which corresponded to reverse saturable absorption of cobalt phthalocyanine.
|
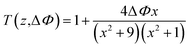 | (3) |
Table 2 Nonlinear Properties of PAE-azo-DCN and PAE-azo-CoPc
Polymer |
α2 |
n2 |
χ(3) |
PAE-azo-DCN |
−6 × 10−11 |
−2.5 × 10−17 |
4.27 × 10−11 |
PAE-azo-CoPc |
2 × 10−10 |
3 × 10−17 |
5.18 × 10−11 |
The nonlinear refractive coefficient n2 of the polymers can be determined by the experimental data based on eqn (3). In the equation, x = z/z0 and ΔΦ is the on-axis phase change caused by the nonlinear refractive index of the sample and ΔΦ = [2πI0(1 − e−α0L)n2]/(λα0). The curves in Fig. 8(b) and (d) are obtained from eqn (3). As shown in Table 2, the nonlinear refractive coefficient n2 of PAE-azo-DCN was −2.5 × 10−17, and the nonlinear refractive coefficient n2 of PAE-azo-CoPc was 3 × 10−17.
|
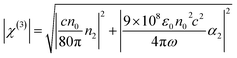 | (4) |
The χ(3) can be calculated by the eqn (4). In the equation, ε0 is the permittivity of vacuum; c is the speed of light; n0 is the refractive index of the medium; ω = 2πc/λ. By calculated from eqn (4), the nonlinear susceptibilities of PAE-azo-CoPc and PAE-azo-DCN are 5.18 × 10−11 esu and 4.27 × 10−11 esu, respectively. The reason of the improving of nonlinear susceptibilities was the introduction of phthalocyaninecobalt groups. In additon, both of the two polymers showed larger nonlinear susceptibilities than those of other azobenzene-containing poly(aryl ether) (azo-PAE)29 or other azo-polymer systems.37–39 The increase of the nonlinear susceptibilities could be attributed to the aromatic rigid structure of poly(arylene ether)s and the large conjugated structure of the phthalocyanine.
3.5. Optical limiting properties
The optical limiting performance of PAE-azo-CoPc were measured in DMF with the same Z-scan technique system as in the nonlinear absorption experiment. The curves of normalized transmittance versus input fluence of PAE-azo-CoPc was show in Fig. 9. As shown in Fig. 9, PAE-azo-CoPc showed well OL performance with the limiting threshold (incident fluence at which the transmittance falls to 50% of the linear transmittance) of 0.038 J cm−2. The curves of output fluence versus input fluence of PAE-azo-CoPc was show in Fig. 10. As shown in Fig. 10, the output fluence linearly increased with the incident fluence obeying the Beer–Lambert law at low-incident fluence. However, at high-incident fluence, the output fluence deviated from linearity, showed typical behavior of optical limiting response.
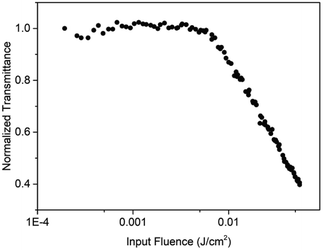 |
| Fig. 9 Optical limiting response of PAE-azo-CoPc in DMF. | |
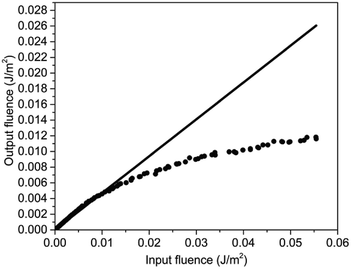 |
| Fig. 10 The curves of output fluence versus input fluence of PAE-azo-CoPc in DMF. | |
4. Conclusions
In this work, a novel kind of poly(arylene ether)s with azo-coupled cobalt phthalocyanine in the side chain (PAE-azo-CoPc) was prepared by 1,2-benzodinitrile, cobaltous chloride anhydrous and a novel azobenzene-containing poly(aryl ether) (PAE-azo-DCN). Both of the polymers exhibited high glass transition temperature (Tg > 158 °C) and good thermal stability (Td5 > 380 °C). PAE-azo-CoPc exhibited higher glass transition temperature and better thermal stability than PAE-azo-DCN due to the introduction of cobalt phthalocyanine groups. The results of Z-scan measurements demonstrated that PAE-azo-CoPc showed reverse saturable absorption and positive refraction, and PAE-azo-DCN showed saturable absorption and negative refraction. PAE-azo-CoPc exhibited larger third-order nonlinear optical susceptibilities than that of PAE-azo-DCN. The results of optical limiting measurements demonstrated that PAE-azo-CoPc showed well OL performance with the limiting threshold of 0.038 J cm−2. PAE-azo-CoPc could be expected to be a potential material for optical limiting, optical switching and other optical applications. This work provided a novel path for designing new excellent optical limiting materials with good thermal stability.
Conflicts of interest
There are no conflicts to declare.
Acknowledgements
The authors gratefully acknowledge the National Natural Science Foundation of China (Grant No. 51703089 and No. 51773085) for financial support.
References
- L. W. Tutt and A. Kost, Nature, 1992, 356, 225–226 CrossRef CAS.
- L. W. Tutt and T. F. Boggess, Prog. Quantum Electron., 1993, 17, 299–338 CrossRef CAS.
- C. W. Spangle, J. Mater. Chem., 1999, 9, 2013–2020 RSC.
- T. J. Mckay, J. Staromlynska, P. Wilson and J. Davy, J. Appl. Phys., 1999, 85, 1337–1341 CrossRef CAS.
- J. Wang, Y. Chen and W. J. Blau, J. Mater. Chem., 2009, 19, 7425–7443 RSC.
- Y. Chen, T. Bai, N. N. Dong, F. Fan, S. F. Zhang, X. D. Zhuang, J. Sun, B. Zhang, X. Y. Zhang, J. Wang and W. J. Blau, Prog. Mater. Sci., 2016, 84, 118–157 CrossRef CAS.
- G. Muruganandi, M. Saravanan, G. Vinitha, M. S. J. Raj and T. C. S. Girisun, Opt. Mater., 2018, 75, 612–618 CrossRef CAS.
- M. V. Srinivasan and P. Kannan, J. Mater. Sci., 2011, 46, 5029–5043 CrossRef.
- P. Gautam, B. Dhokale, V. Shukla, C. P. Singh, K. S. Bindra and R. Misra, J. Photochem. Photobiol., A, 2012, 239, 24–27 CrossRef CAS.
- Z. H. Shi, Y. S. Zhou, L. J. Zhang, C. C. Mu, H. Z. Ren, D. Hassan, D. Yang and H. M. Asifa, RSC Adv., 2014, 4, 50277–50284 RSC.
- Y. Chen, M. Hanack, W. J. Blau, D. Dini, J. J. Doyle, Y. Liu, Y. Lin and J. R. Bai, J. Mater. Sci., 2006, 41, 2169–2185 CrossRef CAS.
- Y. X. Zhang and Y. H. Wang, RSC Adv., 2017, 7, 45129–45144 RSC.
- M. O. Senge, M. Fazekas, E. G. A. Notaras, W. J. Blau, M. Zawadzka, O. B. Locos and E. M. N. Mhuircheartaigh, Adv. Mater., 2007, 19, 2737–2774 CrossRef CAS.
- M. Calvete, G. Y. Yang and M. Hanack, Synth. Met., 2004, 141, 231–243 CrossRef CAS.
- N. J. Li, J. M. Lu, Q. F. Xu and L. H. Wang, Opt. Mater., 2006, 28, 1412–1416 CrossRef CAS.
- T. Ushiwata, E. Okamoto, K. Komatsu, T. Kaino and A. K. Y. Jen, Opt. Mater., 2002, 21, 61–65 CrossRef.
- Y. Chen, M. Hanack, Y. Araki and O. Ito, Chem. Soc. Rev., 2005, 34, 517–529 RSC.
- N. Nwaji, J. Mack, T. Nyokong and T. Nyokong, J. Photochem. Photobiol., A, 2005, 352, 73–85 CrossRef.
- P. Zhao, Z. H. Wang, J. S. Chen, Y. Zhou and F. S. Zhang, Opt. Mater., 2017, 66, 98–105 CrossRef CAS.
- Z. H. Chen, C. Zhong, Z. Zhang, Z. Y. Li, L. H. Niu, Y. J. Bin and F. S. Zhang, J. Phys. Chem. B, 2008, 112, 7387–7394 CrossRef CAS PubMed.
- M. A. Ozdag, A. Mehmet Ali, T. Ceyhan, H. G. Yaglioglu, A. Elmali and O. Bekaroglu, Opt. Laser Technol., 2011, 43, 992–995 CrossRef CAS.
- J. Britton, C. Litwinski, E. Antunes, M. Durmus, V. Chaukea and T. Nyokong, J. Macromol. Sci., Part A: Pure Appl. Chem., 2013, 50, 110–120 CrossRef CAS.
- O. M. Bankole and T. Nyokong, J. Coord. Chem., 2015, 68, 3727–3740 CrossRef CAS.
- E. M. Maya, G. de la Torre, A. E. Lozano, T. Torres, J. G. de la Campa and J. de Abajo, Macromol. Rapid Commun., 2006, 27, 1852–1858 CrossRef CAS.
- T. F. Wang, X. Wang, J. L. Zhang, C. R. Wang, J. F. Shao, Z. H. Jiang and Y. H. Zhang, Dyes Pigm., 2018, 154, 75–81 CrossRef CAS.
- Y. X. Zhang, S. H. Pei, Y. P. Wang, Z. D. Cui, N. Li, Y. Zhu, H. B. Zhang and Z. H. Jiang, Dyes Pigm., 2013, 99, 1117–1123 CrossRef CAS.
- Y. X. Zhang, J. J. Zhang, Z. D. Cui, Q. D. Chen, H. B. Zhang and Z. H. Jiang, J. Polym. Sci., Part A: Polym. Chem., 2015, 53, 936–943 CrossRef CAS.
- D. Liu, Z. G. Wang, H. Yu and J. You, Eur. Polym. J., 2009, 45, 2260–2268 CrossRef CAS.
- H. B. Zhang, J. H. Pang, D. Wang, X. F. Li and Z. H. Jiang, J. Membr. Sci., 2006, 264, 56–64 CrossRef.
- X. B. Chen, J. J. Zhang, H. B. Zhang, Z. H. Jiang, G. Shi and Y. L. Song, Dyes Pigm., 2008, 77, 223–228 CrossRef CAS.
- Y. L. Du, K. Zhu, Y. Fang, S. L. Zhang, X. R. Zhang, T. N. Lu, Y. C. Yang, Y. L. Song and G. B. Wang, RSC Adv., 2015, 5, 48311–48322 RSC.
- Y. L. Du, S. L. Zhang, X. Jiang, K. Zhu, Z. Geng, Y. Fang, P. F. Huo, C. Liu, Y. L. Song and G. B. Wang, J. Polym. Sci., Part A: Polym. Chem., 2014, 52, 1282–1290 CrossRef CAS.
- G. Zhao, G. Wei, W. J. Zhu, F. Y. Ke, S. Y. Guang, F. Y. Zhang and H. Y. Xu, J. Appl. Polym. Sci., 2019, 135, 46100 CrossRef.
- Y. L. Lei, F. D. Ma, Y. Tian, Q. L. Niu, H. Y. Mi, I. Nurulla and . Shi, J. Appl. Polym. Sci., 2013, 129, 1763–1772 CrossRef CAS.
- F. A. Nicolescu, V. V. Jerca, I. Dancus, A. Petris, T. V. Nicolescu, I. B. Rau, V. I. Vlad, D. S. Vasilescu and D. M. Vuluga, J. Polym. Res., 2011, 18, 1009–1016 CrossRef CAS.
- J. Illesca, Y. S. Ramirez-Fuentes, E. Rivera, O. G. Morales-Saavedra, A. A. R. Guez-Rosales, V. Alzari, D. Nuvoli, S. Scognamillo and A. Mariani, J. Polym. Sci., Part A: Polym. Chem., 2011, 49, 3291–3298 CrossRef.
- C. Zhang, Y. L. Song, X. Wang, F. E. Kuhn, Y. X. Wang, Y. Xu and X. Q. Xin, J. Mater. Chem., 2003, 13, 571–579 RSC.
- F. Cherioux, P. Audebert, H. Maillotte, L. Grossard, F. E. Hermamdez and A. Lacourt, Chem. Mater., 1997, 9, 2921–2927 CrossRef CAS.
- O. Varnavski, R. G. Ispasoiu, M. Narewal, J. Fugaro, Y. Jin, H. Pass and T. Goodson III, Macromolecules, 2000, 33, 4061–4068 CrossRef CAS.
|
This journal is © The Royal Society of Chemistry 2019 |