DOI:
10.1039/D3NH00192J
(Communication)
Nanoscale Horiz., 2023,
8, 1253-1261
Colloidal gold-based immunochromatographic biosensor for quantitative detection of S100B in serum samples†
Received
20th May 2023
, Accepted 11th July 2023
First published on 12th July 2023
Abstract
Traumatic brain injury has become a serious public health problem. Timely detection, diagnosis and treatment of brain injury are closely related to the prognosis of patients, so identification of highly sensitive and specific biochemical markers of brain injury has important clinical value. Currently, the most studied and most promising marker is the protein S100B. In this study, a rapid quantitative biosensor for S100B was established using colloidal gold labeling and double antibody (8C10-6B8) sandwich immunochromatography. The biosensor was capable of quantifying S100B within 15 min, and showed no cross-reactivity with S100A, NSE, GFAP, or PGP9.5. The detection limit was determined to be 4.6 pg mL−1 with a linear range of 0.01–2 ng mL−1. Recovery experiments also indicated that the method had an acceptable accuracy. Moreover, the quantitative colloidal gold assay correlated well with the results of a chemiluminescence immunoassay when testing 40 clinical serum samples. Our developed colloidal gold quantitative immunochromatographic biosensor is a rapid, sensitive, specific and accurate method for the detection of S100B protein in serum, which is useful in the clinic for early diagnosis, as well as assessment of disease progression and prognosis of traumatic brain injury.
New concepts
A rapid quantitative assay for S100B was established using colloidal gold labeling and double antibody sandwich immunochromatography. The assay was capable of quantifying S100B within 15 min, and showed no cross-reactivity with S100A, NSE, GFAP, or PGP9.5. The detection limit was determined to be 5 pg mL−1 with a linear range of 0.005–100 ng mL−1. The quantitative colloidal gold assay correlated well with the results of a chemiluminescence immunoassay when testing the clinical serum samples.
|
1. Introduction
Traumatic brain injury (TBI) is a relatively frequent clinical neurosurgical problem, and the number of new patients with TBI is expected to exceed 10 million each year worldwide. The majority of patients are left with different degrees of disability, which poses a serious risk to the patients’ daily life and physical and mental health.1 How to effectively assess the extent of TBI and evaluate patients’ prognosis for recovery has become a subject of intensive clinical research. In the past, patients were mostly diagnosed using the Glasgow Coma Scale or neuroimaging,2 but the accuracy was not satisfactory. Patients with mild symptoms or diffuse axonal injury are difficult to diagnose by imaging and are highly susceptible to problems of underdiagnosis and misdiagnosis, which can delay effective patient treatment.3 Scale assessments are limited by subjective factors of the assessor, making it difficult to ensure the accuracy of the results.4 After TBI occurs, biomarkers such as proteins, nucleic acids, and metabolites can be released from neurons and glial cells into the extracellular space, where mechanical forces coupled with neurometabolic and inflammatory cascades can disrupt blood–brain barrier integrity and allow biomarkers to enter the bloodstream.5 Thus, biomarkers representing cranial injury can be present in peripheral blood at the time of TBI, making it possible to assess TBI using peripheral blood tests.
In recent years, great progress has been made in the study of certain specific proteins involved in the nervous system and in craniosynostosis, of which S100B protein is a representative example.6–8 S100B is a calcium-binding protein and is one of the most active members of the S100 family. It is a specific protein mainly found in glial cells, and the content of S100B protein in normal human serum is <0.2 μg L−1; however it can enter the extracellular fluid in large amounts when cell integrity is disrupted by glial cell damage.6–8 The ideal marker should be sensitive to TBI and highly selective for brain tissue, with an expression level which changes rapidly, allowing detection when TBI occurs, while the concentration of the marker or its magnitude above the level of healthy people should change with the severity of the disease and independent of other factors, such as the patient's age and gender. S100B protein is fully compatible with the above requirements.9,10
With continuous technical advances, there has been a significant improvement in the detection of S100B. Currently, the main methods for detection of S100B are: radioimmunoassay (RIA), immunoradiometric assay (IRMA), enzyme-linked immunosorbent assay (ELISA)11 and electrochemiluminescence assay (ECLIA). RIA and IRMA are now less commonly used in assays due to the problems of radiation and contamination; the ELISA assay takes 4–6 hours, which is time consuming and labor intensive and the method has poor functional sensitivity and exhibits a high coefficient of variation (CV);12 the ECLIA test requires a large instrument, and the expensive initial outlay to buy the instrument, reagents and electrodes and the high cost of the test limit its implementation in primary hospitals, which is also its shortcoming. In view of the above problems, there is an urgent need to find a more convenient, rapid and accurate test for clinical use, such as Point-of-Care Testing (POCT) lateral flow immunoassay strips. Over the past few decades, lateral flow immunoassay strips have been widely used in POCT because of their simplicity, convenience, ease of operation, rapidity and relatively low price.13–15 Gold nanoparticles are easy to conjugate with immunoglobulins, toxins, antibiotics, hormones and other biological macromolecules through electrostatic interaction without affecting their biological activity, and owing to the advantages of easy synthesis, rapid detection and low cost, hold nanoparticles have been widely used in POCT.16–18 The traditional colloidal gold immunochromatographic strips can only produce qualitative or semi-quantitative results, mainly through the naked eye providing a “yes/no” result and thus the detection sensitivity is limited to a certain extent.19 The application principle of a colloidal gold quantitative immunoassay is mainly based on the proportional relationship between the color shade of the detection line on the nitrocellulose membrane and the concentration of the substance to be detected, and the results of quantitative analysis are obtained by scanning the reagent strip through an optical sensor and subsequently comparing with a standard curve.20,21 There are various modes of quantitative immunochromatographic assays. Among them, the T/C value (ratio of a signal on the test line to that on the control line) is the more commonly used quantitative analysis method, because the specific immune reaction between antigen and antibody in the chromatographic process is a dynamic process, and the color of the T and C lines changes simultaneously with time and external environment. Therefore, the T/C value can, to a certain extent, eliminate the differences in test strip results caused by environmental factors and sample matrix effects.22,23
The aim of this study was to prepare anti-S100B monoclonal antibodies (mAbs), pair the resulting mAbs, and develop an immunochromatographic biosensor for the rapid quantitative detection of S100B using colloidal gold as the marker, in order to produce highly-sensitive immunochromatographic biosensor for the detection of S100B. This measurement of serum levels of S100B is important for the determination of the severity and prognosis of patients with TBI.
2. Materials and methods
2.1. Main reagents and apparatus
HEK293F cells and reagents required for the preparation of hybridoma cells were all purchased from Gibco (Thermo Fisher Scientific, Shanghai, China). Cells were adapted and cultured with SMM 293-TII Expression Medium (Sino Biological, Beijing, China) in suspension. Proteins used in the detection of specificity were also purchased from Sino Biological Inc. (Beijing, China). Materials required for the preparation of colloidal gold test strips were all purchased from JieYi Biotechnology Co., Ltd (Shanghai, China). Polyethylenimine (PEI) was purchased from Polysciences, Inc. (Warrington, PA, USA). Ni-NTA was purchased from Sangon Biotech Co., Ltd (Shanghai, China). All other reagents were purchased from the National Pharmaceutical Group Chemical Reagent Co., Ltd (Shanghai, China).
2.2. Ethical approval
Six-week-old BALB/c mice were purchased from Beijing Vital River Laboratory Animal Technology Co., Ltd (Beijing, China). All animal procedures were performed in accordance with the Guidelines for Care and Use of Laboratory Animals of Jiangnan University and approved by the Animal Ethics Committee of Jiangnan University.
2.3. Production of monoclonal antibodies against S100B
Expression and purification of S100B protein were performed as described previously.24,25 Briefly, based on the genome sequence of S100B (NCBI reference sequence: NP_006263.1), a codon-optimized gene was designed and synthesized by Genecefe Biotechnology Co., Ltd (Wuxi, China). The fragment was then cloned into the expression vector pCMV3. Recombinant S100B with a His tag at the N-terminus was transiently expressed in HEK293F cells following assisted transfection with linear 25 kDa PEI. The supernatant was passed through a metal chelate affinity chromatography column (Ni-NTA agarose) to enrich the protein, and then the target protein was obtained by using a gel filtration chromatography column to separate the heteroproteins. Five Balb/c mice in good condition and normal growth were selected, and the above purified recombinant S100B protein was used as the immune antigen, mixed with Freund's complete adjuvant in equal volume, emulsified thoroughly and then injected intraperitoneally. Four immunizations were performed at two-week intervals and the titer of mouse serum was determined by indirect ELISA. The mouse with the highest titer was selected for punch immunization, and spleen cells were fused with SP2/0 myeloma cells 3 days later. Hybridoma cells were screened with HAT selective medium, and after three rounds of subcloning, the hybridoma cell lines with the highest absorbance at 450 nm were selected.26–28 Finally, the mAbs were obtained by protein G purification.
2.4. Anti-S100B mAb pairing
2.4.1 Preparation of colloidal gold.
Chloroauric acid (HAuCl4) is reduced to gold nanoparticles of a certain size under the action of reducing agents, which form a negatively-charged hydrophobic colloidal solution due to electrostatic reactions.29 In this study, the colloidal gold solution was prepared by the trisodium citrate reduction method.30 First, 1000 mL of ultrapure water was heated to boiling on a magnetic stirrer, then 40 mL of 1% chloroauric acid solution was added, and when the solution returned to the boil, 36 mL of 1% trisodium citrate solution was quickly added. When the color of the solution turned purple, the solution was boiled for a further 5 min, then removed from the heat, and made up to the original volume with ultrapure water after cooling. Colloidal gold nanoparticles were characterized by ultraviolet spectrophotometry (UV-Vis) and transmission electron microscopy (TEM).
2.4.2 Colloidal gold-labeled anti-S100B mAbs.
To produce the colloidal gold-labeled anti-S100B mAbs, 1 mL of colloidal gold was added to each of ten centrifuge tubes, the pH was adjusted to 7.0 with 0.1 M K2CO3 and the solution was mixed well. Next, 10 μg of one of the anti-S100B mAbs (1E8, 2A6, 3H11, 4D12, 5C3, 6B8, 7F2, 8C10, 9D9 or 10G11) was added to each tube. After coupling on a shaker for 1 h, 100 μL of 10% BSA was added and incubated for 2 h. Finally, the mixture was centrifuged at 8500 × g, 4 °C for 45 min, the supernatant was discarded, and the labeled antibody was resuspended with 100 μL of 0.02 M tris–HCl buffer (containing 1% BSA, 2% Tween-20 and 5% alginate) and stored at 4 °C until required.31
2.4.3 Preparation and assembly of immunochromatographic biosensor.
The structure diagram and detection principle of the quantitative detection of S100B using the immunochromatographic biosensor are shown in Fig. 1. In brief, computer and sensing technology is used to prepare the colloidal gold quantification instrument, the test strip color development reaction is converted into a chromatographic signal, and the strength of the chromatographic signal is positively correlated with the concentration of the sample to be tested, so as to achieve accurate quantification of colloidal gold technology.32 And the colloidal gold immunochromatographic test strips consist of five main parts: PVC backing plate, absorbent pad, nitrocellulose (NC) membrane, conjugate pad and sample pad. Before assembly, the gold-labeled antibodies were diluted in appropriate multiples and sprayed onto the glass fiber pad with a three-dimensional spraying machine, then dried at 37 °C for 12 h to form conjugate pads. The ten anti-S100B mAbs diluted to 2.0 mg mL−1 and the goat anti-mouse antibody diluted to 0.6 mg mL−1 were sprayed onto the NC membrane to form the detection line (T line) and the control line (C line), respectively, with the two lines spaced 5 mm apart, then the membrane was dried at 37 °C overnight. The prepared NC membrane was then pasted in the middle area of the PVC backing plate, then the absorbent pad and the conjugate pad were attached on either side of the PVC backing plate so that they overlapped with the NC membrane by 1 mm, and finally the sample pad was fixed near the conjugate pad so that it overlapped with the end of the pad by 1 mm. The whole assembly was cut into test strips 3 mm wide using a chopping machine and put into plastic card cases for later use.33
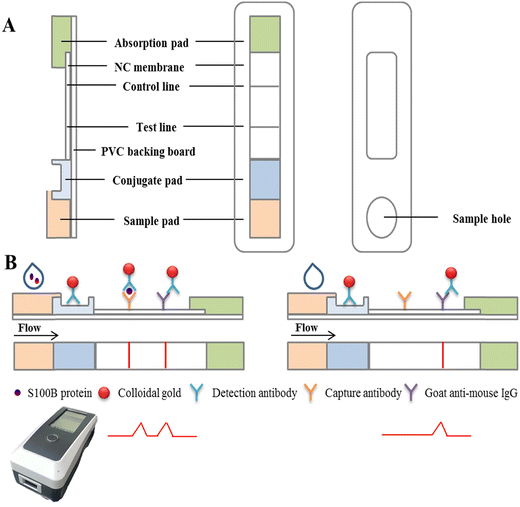 |
| Fig. 1 The assembly diagram of double antibody sandwich colloidal gold immunochromatographic strips. (A) Structure and (B) the quantitative detection principle of immunochromatographic biosensor. | |
2.4.4 MAb pairing.
One hundred microliters of S100B protein was added to the sample pad; running buffer (0.01 M PBS containing 1% On-870) was used as negative control. Antibody pairing was performed according to the double antibody sandwich principle, and the color development of T and C lines was observed 15 min after sample addition.
2.5. Optimization of colloidal gold immunochromatographic biosensor
To obtain better sensitivity and a higher T/C value for the biosensor, some conditions need to be optimized. The antibodies that had been successfully paired were tested and the one that had the strongest T-line color rendering was chosen for condition optimization.
2.5.1 Optimization of pH.
The mAb was labeled with colloidal gold according to the above steps, where the pH was adjusted to 5, 5.5, 6, 6.5, 7, 7.5 or 8 with 0.1 M K2CO3. The test strips were assembled, then 100 μL of S100B protein diluted to 10 ng mL−1 was added to the sample wells, and the T/C signal value was read by a colloidal gold optical reader after 15 min of reaction.
2.5.2 Optimization of antibody labeling amount.
At the optimal labeling pH, mAbs were labeled with colloidal gold in accordance with step 2.2.2, and the antibodies were diluted to concentrations of 2, 5, 10, 15, 20 and 25 μg mL−1. The T/C signal value was read 15 min after 10 ng mL−1 of S100B protein was added.
2.5.3 Optimization of capture antibody concentration.
During preparation of the colloidal gold test strips, the amount of antibody coating on the T-line will affect the sensitivity of the test strips. If the coating concentration is too low, the strips will show unclear color or hollow phenomenon, and the stability will be poor, causing false negative results; if the coating concentration is too high, it will unnecessarily waste the antibody, and also affect labeling activity. Therefore, the amount of antibody to be coated needs to be optimized. The anti-S100B mAb was diluted to 0.2, 0.5, 1, 1.5, 2 and 2.5 mg mL−1 and sprayed onto the NC membrane as the T-line. The colloidal gold-labeled mAb under optimized conditions was sprayed onto the conjugate pad and assembled into test strips, 100 μL of diluted S100B was spiked, and the signal intensity of T/C was recorded after 15 min of reaction.34
2.5.4 Study of reaction kinetics.
The assay process of the test strip is a dynamic immunological reaction, and analysis of the dynamic reaction during the test strip assay creates some theoretical guidance for the test strip quantitative assay method. In this experiment, changes of the T and C line values of the test strips were read with the aid of a colloidal gold optical reader, and the kinetic curves were plotted. The color change curves of the T and C lines indirectly reflects the immunokinetic process of antigen and antibody binding on the T and C lines. To perform the analysis, 100 μL of diluted S100B at 10 ng mL−1 was added and the T and C line signal intensities and T/C values were recorded once per min for 20 min, starting 2 min after the reaction was started.
2.6. Evaluation of the quantitative colloidal gold immunochromatographic biosensor
2.6.1 Sensitivity of the colloidal gold immunochromatographic biosensor.
S100B standards were prepared using negative sera in a series of concentrations of 0, 0.01, 0.05, 0.1, 0.5, 1, 10, and 100 ng mL−1, and assayed using the immunochromatographic biosensor. The assay was repeated three times for each concentration and the T/C signal values of the test strips were recorded at the optimal equilibrium time using a colloidal gold optical reader. A standard curve was constructed by fitting the logarithm of S100B concentration against the T/C value, and the sensitivity of the biosensor was expressed by the limit of detection (LOD), which was calculated by the IUPAC formula (LOD = yblank + 3 × SDblank, where yblank and SDblank are the average T/C value and the standard deviation of the blank control (n = 20), respectively).35–37
2.6.2 Specificity of the colloidal gold immunochromatographic biosensor.
The specificity of the immunochromatographic biosensor was evaluated by analyzing the three biomarkers NSE, GFAP, PGP9.5,38 as well as S100A, which belongs to the same family of calcium-binding proteins, in the serum sample. These proteins were diluted to 1000 ng mL−1, added dropwise to prepared test strips, and assayed at optimal equilibration times.
2.6.3 Accuracy of the colloidal gold immunochromatographic biosensor.
To further evaluate the accuracy of the method, high-value serum samples were diluted with negative serum to generate three different concentrations of serum samples (100, 10 and 1 ng mL−1) and assayed with immunochromatographic biosensor, and the experiment was repeated three times. Finally, the corresponding recoveries and CVs were calculated.
2.6.4 Validation of the strip in real serum samples.
To validate the reliability of the established S100B colloidal gold quantitative assay, 40 clinical serum samples were tested. All serum samples were obtained from Yixing People's Hospital (Wuxi, China), and positive samples were calibrated using a chemiluminescence immunoassay kit (Roche, Basel, Switzerland).
3. Results and discussion
3.1. Identification of S100B protein and mAbs
The designed plasmids were tagged with His tags, so nickel columns were first selected to enrich the S100B protein. Using elution buffers containing different concentrations of imidazole, the S100B protein was eluted and the obtained eluate was subjected to the next step of purification. To improve the purity of the S100B protein, ultrafiltration tubes were selected for concentration of the S100B protein, based on which a gel filtration chromatography column was used to separate the S100B protein from other proteins. The results (Fig. 2(A)) showed that the molecular weight of the S100B protein was about 14 kD, as expected, and the purity after secondary purification reached 95%, so it could be used for the subsequent preparation of mAbs. In this study, 30 monoclonal cell lines capable of secreting antibodies were successfully prepared by immunizing mice with the expressed S100B protein. Among the 30 cell lines screened, ten cell lines with high affinity were selected for preparation of monoclonal antibody ascites, and a total of 10 anti-S100B mAbs were obtained. Fig. 2(B) and Table 1 show the SDS-PAGE characterization and isotypes of these antibodies, respectively.
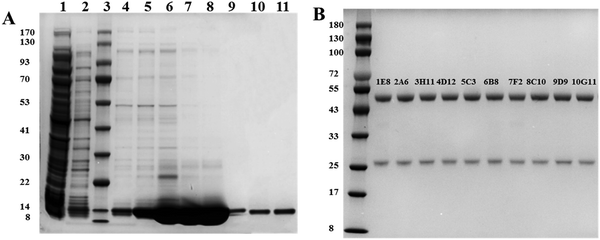 |
| Fig. 2 SDS-PAGE of (A) S100B protein: lanes 1: cell supernatants; lanes 2: flow-through; lane 3: protein molecular weight marker; lane 4–8: S100B protein purified using Ni-NTA; lane 9–11: S100B protein purified using Ni-NTA and gel filtration; and (B) anti-S100B mAbs. | |
Table 1 The isotypes of ten anti-S100B mAbs
mAbs |
Isotype |
mAbs |
Isotype |
1E8 |
IgG2b |
6B8 |
IgG2b |
2A6 |
IgG1 |
7F2 |
IgG1 |
3H11 |
IgG1 |
8C10 |
IgG2a |
4D12 |
IgG1 |
9D9 |
IgG2a |
5C3 |
IgG2b |
10G11 |
IgG1 |
3.2. Characterization of colloidal gold
The color of the prepared colloidal gold solution was burgundy; the solution was uniform, clarified and translucent, with no precipitation or impurities. UV-Vis spectroscopy showed that its maximum absorption peak was 525 nm (Fig. S1A, ESI†), which corresponds to the particle size of colloidal gold of about 40 nm. TEM images (Fig. S1B, ESI†) also showed that the colloidal gold particles had a uniform particle size and good dispersion, and thus could be used for the establishment of an immunoassay.
3.3. MAb pairing
Ten purified anti-S100B mAbs were selected and assembled in pairs on the test strips. The results of the selected mAbs and their paired color development are shown in Table 2. For the subsequent preparation of S100B double antibody sandwich immunochromatographic strips, 8C10-6B8 were selected in terms of the strength of paired color development, where the capture antibody was used to create the T-line and the detection antibody was used to label colloidal gold.
Table 2 The screening of the matched mAbs against S100B protein by the checkerboard method
Detection antibodies |
Capture antibodies |
1E8 |
2A6 |
3H11 |
4D12 |
5C3 |
6B8 |
7F2 |
8C10 |
9D9 |
10G11 |
1E8 |
− |
− |
+ |
− |
+ |
− |
− |
+ |
+ |
+ |
2A6 |
− |
− |
+ |
− |
+ |
+ |
+ |
+ |
− |
+ |
3H11 |
+ |
− |
+ |
+ |
− |
− |
− |
+ |
− |
+ |
4D12 |
+ |
− |
− |
− |
− |
− |
+ |
+ |
− |
+ |
5C3 |
− |
− |
− |
− |
+ |
− |
− |
+ |
− |
+ |
6B8 |
+ |
− |
+ |
− |
− |
+ |
+ |
+++ |
+ |
++ |
7F2 |
− |
+ |
+ |
+ |
− |
− |
− |
++ |
− |
+ |
8C10 |
− |
+ |
+ |
+ |
− |
− |
− |
+ |
− |
+ |
9D9 |
− |
− |
− |
− |
− |
− |
− |
+ |
− |
+ |
10G11 |
++ |
++ |
− |
− |
− |
+ |
− |
+ |
− |
+ |
3.4. Optimization of the process
The labeling pH can affect the activity of the antibody and the coupling efficiency of the antibody to colloidal gold, which further affects the sensitivity of the assay; therefore, the process of optimizing the antibody-labeling pH is important. In the antibody-labeling pH optimization process, it can be seen from Fig. S2A (ESI†) that when the pH of the colloidal gold solution was below 6.5, the colloidal gold turned purple and precipitated after the addition of antibody 6B8. The labeled antibodies were applied to the test strips and the prepared test strips were used to detect S100B. As can be seen in Fig. 3(A), the strongest T/C value was obtained when the pH was 7.5, indicating that the effective amount of antibody labeling was greatest at pH 7.5. Therefore, the optimal labeling pH was 7.5.
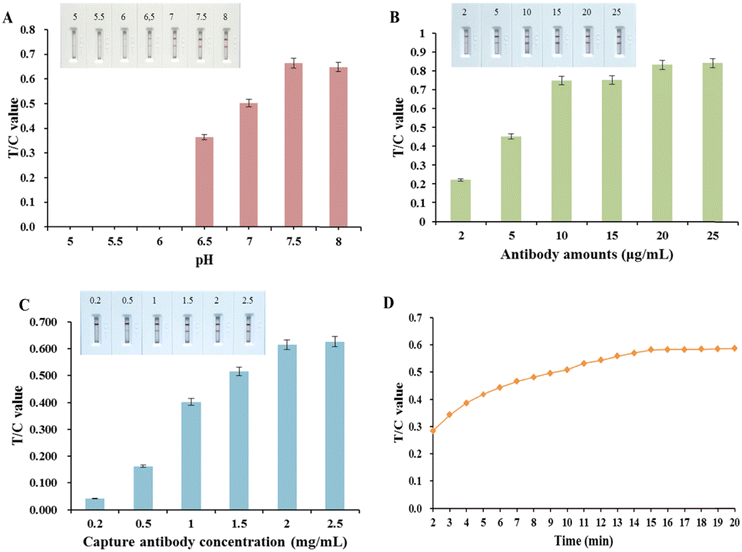 |
| Fig. 3 Optimization of conditions for colloidal gold test strips. (A) Labeling pH value; (B) amount of antibody labeling; (C) concentration of capture antibody on T-line; (D) determination of reaction time. | |
The effect of the amount of colloidal gold used to label the antibody on the intensity of the chromogenic signal of the test strips is shown in Fig. 3(B). As can be seen from the figure, the T/C value of the test strip gradually increased with the labeling amount of antibody 6B8 from 2 to 25 μg and decreased thereafter, reaching a peak at 20 μg, indicating that the amount of antibody input was not positively correlated with the labeling efficiency of the antibody. Finally, the labeling amount at the highest T/C value, i.e. 20 μg, was selected as the optimal labeling amount.
As shown in Fig. 3(C), the T/C value was gradually enhanced when the capture antibody on the T-line was at a concentration of 0.2 to 2.5 mg mL−1. The T/C values were strongest and leveled off at T-line antibody concentrations of 2.0 to 2.5 mg mL−1. Consequently, 2.0 mg mL−1 was selected as the optimal T-line antibody concentration for maximum T-line signal strength combined with antibody economy.
In colloidal gold immunochromatographic assays, the antigen and antibody are reacting dynamically during the chromatographic process. However, over time, the detection signal based on the T/C signal value eventually tends to equilibrium, and in order to achieve the purpose of rapid detection, the time when the dynamic detection signal basically reaches equilibrium can be set as the detection time. The experimental results (Fig. 3(D)) showed that the signal intensity of T and C lines increased rapidly and then increased slowly and stabilized within 15 min after the sample was added to the test strip, and the T/C value reached equilibrium at 15 min, so the test strip detection time was set at 15 min.
3.5. Construction of the standard curve for S100B quantitative detection
S100B standard solutions were prepared using negative sera at concentrations ranging from 0 to 100 ng mL−1 and spiked onto test strips for detection. Fig. 4(A) presented the changes in color of the T and C lines. The color intensity of the T line increased with increasing S100B protein concentration. T/C values were read at the optimized reaction equilibrium time points, and three parallel experiments were performed for each sample concentration. Fig. 4(B) presented the calibration curve as Y = 0.729 − 0.726/[1 + (X/2.456)0.933] with a reliable correlation coefficient R2 = 0.997, where Y is the T/C value and X is the S100B concentration. The sensitivity of the strips was expressed by the LOD, which was calculated to be 4.6 pg mL−1 with a linear range of 0.01–2 ng mL−1.
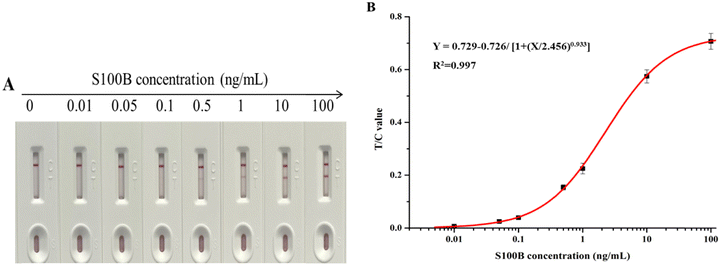 |
| Fig. 4 (A) Photograph of colloidal gold-based strips for different concentrations of S100B antigen detection (0–100 ng mL−1); (B) corresponding calibration curve of S100B antigen detection. | |
3.6. Evaluation of colloidal gold immunochromatographic biosensor
Solutions of NSE, GFAP, PGP9.5, and S100A at 1000 ng mL−1 were analyzed by our proposed method (Fig. 5(A)). When S100A was detected a faint band could be seen on the T line, whereas the other three biomarkers did not show any color. This result demonstrated that the immunochromatographic biosensor only weakly cross-reacted with S100A at very high concentrations, indicating very good specificity.
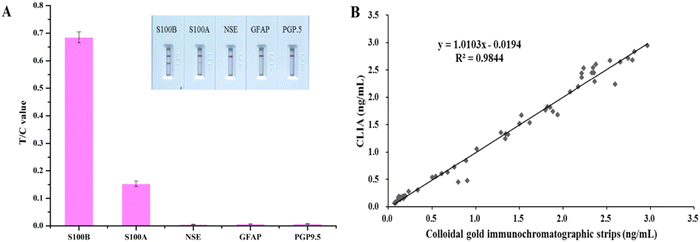 |
| Fig. 5 Evaluation of colloidal gold immunochromatographic biosensor. (A) Assessment of specificity in the detection of S100B; (B) correlation of CLIA and colloidal gold immunochromatographic biosensor analysis results determined using 40 samples. | |
Three sera were used for the recovery assay. The results are shown in Table S1 (ESI†), and the recoveries ranged from 96.0% to 110.0%, with a relatively low CV ranging from 3.7% to 4.7%. This result demonstrated the reliable accuracy of our established S100B quantitative assay.
The results of 40 clinical sera tested simultaneously using a commercially-available chemiluminescence immunoassay kit alongside the S100B colloidal gold quantitative assay established in this study are shown in Fig. 5(B). The two were well correlated, with the regression equation: y = 1.0103x − 0.019 (R2 = 0.984). This result confirmed the good reliability of the quantitative S100B detection established in this study when analyzing actual clinical serum samples.
4. Discussion
The quantitative determination of S100B protein in human serum by colloidal gold immunochromatography has the following advantages in application: it is highly sensitive and specific for TBI, and the changes in its content are closely related to clinical symptoms, signs and imaging changes.39 Significant changes in blood within 24 h of the appearance of cerebral ischemic symptoms can assist in early diagnosis and can be used as a complement to imaging.40 Moreover, the in vitro diagnostic reagents cause no secondary harm to patients, and the bedside testing mode is suitable for patients with low cooperation, which would make it inconvenient to implement imaging observation. Of course, the results of S100B protein testing should be combined with other clinical information and should not be used as the only criterion for judgment.
In this study, a method was established for the quantitative detection of S100B using colloidal gold immunochromatographic biosensor. The best detection and capture antibodies for use in the test strips were determined to be 6B8 and 8C10, respectively, by antibody pairing experiments. The colloidal gold immunochromatographic biosensor exhibited good sensitivity in real serum samples with a LOD of 4.6 pg mL−1. Further, the results of specificity tests showed that the biosensor cross-reacted weakly with S100A at a very high concentration, while there was no cross-reactivity with other serum markers of TBI. In addition, the accuracy and real sample detection of the biosensor were fully validated. However, due to time constraints, there is still some work to be done for a further in-depth study, including evaluation of the stability of the biosensor and the validation of more clinical samples. Overall, this method enables rapid determination of S100B, which is useful for clinicians to reach an early diagnosis, and to perform assessment of disease progression and prognosis of TBI.
Ethical approval
This article does not contain any studies with human subjects. All animal studies were carried out under the guidance of the animal welfare committee of Jiangnan University.
Conflicts of interest
No potential conflict of interest was reported by the authors.
Acknowledgements
This work was funded by the Key Programs from MOST (2022YFA1207300).
References
- A. I. R. Maas, D. K. Menon and P. D. Adelson,
et al., Traumatic brain injury:integrated approaches to improve prevention, clinical care, and research, Lancet Neurol., 2017, 16(12), 987–1048 CrossRef PubMed.
- H. Zeina, K. Firas and L. K. Massoud,
et al., Advances in point-of-care platforms for traumatic brain injury: recent developments in diagnostics, Rev. Neurosci., 2022, 33(3), 327–345 CrossRef PubMed.
- E. L. Dennis, K. Caeyenberghs and R. F. Asarnow,
et al., Challenges and opportunities for neuroimaging in young patients with traumatic brain injury: a coordinated effort towards advancing discovery from the ENIGMA pediatric moderate/severe TBI group, Brain Imaging Behav., 2021, 15(2), 555–575 CrossRef PubMed.
- Y. G. Bodien, A. Barra and N. R. Temkin,
et al., Diagnosing level of consciousness: the limits of the glasgow coma scale total score, J. Neurotrauma, 2021, 38(23), 3295–3305 CrossRef PubMed.
- M. E. Huibregtse, J. J. Bazarian and S. R. Shultz,
et al., The biological significance and clinical utility of emerging blood biomarkers for traumatic brain injury, Neurosci. Biobehav. Rev., 2021, 130, 433–447 CrossRef CAS PubMed.
- E. Thelin, F. A. Nimer and A. Frostell,
et al., A serum protein biomarker panel improves outcome prediction in human traumatic brain injury, J. Neurotrauma, 2019, 36(20), 2850–2862 CrossRef PubMed.
- E. Mercier, P. A. Tardif and P. A. Cameron,
et al., Prognostic value of S-100β protein for prediction of post-concussion symptoms after a mild traumatic brain injury: systematic review and meta-analysis, J. Neurotrauma, 2018, 35(4), 609–622 CrossRef PubMed.
- S. Schulte, L. W. Podlog and J. J. Hamson-Utley,
et al., A systematic review of the biomarker S100B: implications for sport-related concussion management, J. Athl. Train., 2014, 49(6), 830–850 CrossRef PubMed.
- K. K. Wang, Z. Yang and T. Zhu,
et al., An update on diagnostic and prognostic biomarkers for traumatic brain injury., Expert Rev. Mol. Diagn., 2018, 18(2), 165–180 CrossRef CAS PubMed.
- K. Kawata, C. Y. Liu and S. F. Merkel,
et al., Blood biomarkers for brain injury: what are we measuring?, Neurosci. Biobehav. Rev., 2016, 68, 460–473 CrossRef CAS PubMed.
- A. Celikbilek, L. Akyol and S. Sabah,
et al., S100B as a glial cell marker in diabetic peripheral neuropathy, Neurosci. Lett., 2014, 13(558), 53–57 CrossRef PubMed.
- L. H. M. Smit, C. M. Korse and J. M. G. Bonfrer, Comparison of four different assays for determination of serum S100B, Int. J. Biol. Markers, 2005, 20(1), 34–42 CrossRef CAS PubMed.
- F. Di Nardo, M. Chiarello and S. Cavalera,
et al., Ten years of lateral flow immunoassay technique applications: trends, challenges and future perspectives, Sensors, 2021, 21(15), 5185 CrossRef CAS PubMed.
- L. Anfossi, F. Di Nardo and S. Cavalera,
et al., Multiplex lateral flow immunoassay: an overview of strategies towards high-throughput point-of-need testing, Biosensors, 2019, 9(1), 2 CrossRef CAS PubMed.
- L. Fei, B. Mya and D. Slc,
et al., Paper-based point-of-care immunoassays: Recent advances and emerging trends, Biotechnol. Adv., 2020, 39, 107442 CrossRef PubMed.
- J. Guo, S. Chen and J. Guo,
et al., Nanomaterial labels in lateral flow immunoassays for point-of-care-testing, J. Mater. Sci. Technol., 2021, 60, 90–104 CrossRef CAS.
- N. Jiang, R. Ahmed and M. Damayantharan,
et al., Lateral and vertical flow assays for point-of-care diagnostics. Advanced Healthcare, Materials, 2019, 8(14), 1900244 Search PubMed.
- B. N. Khlebtsov, R. S. Tumskiy and A. M. Burov,
et al., Quantifying the numbers of gold nanoparticles in the test zone of lateral flow immunoassay strips, ACS Appl. Nano Mater., 2019, 2(8), 5020–5028 CrossRef CAS.
- W. Xiao, C. Huang and F. Xu,
et al., A simple and compact smartphone-based device for the quantitative readout of colloidal gold lateral flow immunoassay strips, Sens. Actuators, B, 2018, 266, 63–70 CrossRef CAS PubMed.
- L. Yu and A. Andriola, Quantitative gold nanoparticle analysis methods: A review, Talanta, 2010, 82(3), 869–875 CrossRef CAS PubMed.
- C. Parolo, A. Sena-Torralba and J. F. Bergua,
et al., Tutorial: design and fabrication of nanoparticle-based lateral-flow immunoassays, Nat. Protoc., 2020, 15, 3788–3816 CrossRef CAS PubMed.
- V. Shirshahi and G. Liu, Enhancing the analytical performance of paper lateral flow assays: from chemistry to engineering, TrAC, Trends Anal. Chem., 2021, 136, 116200 CrossRef CAS.
- T. Siriwan, W. Uraiwan and P. Pongsathon, Development of qualitative and quantitative immunochromatographic strip test assay for rapid and simple detection of leucomalachite green residual in aquatic animals, Food Chem., 2020, 320, 126613 CrossRef PubMed.
- X. X. Xu, T. Y. Xiang and S. S. Song,
et al., Secretory expression and purification of recombinant PLA2R epitopes for the detection of anti-PLA2R autoantibody in serum, Analyst, 2022, 147(5), 965–974 RSC.
- L. Y. Ye, X. X. Xu and S. S. Song,
et al., Rapid colloidal gold immunochromatographic assay for the detection of SARS-CoV-2 total antibodies after vaccination, J. Mater. Chem. B, 2022, 10, 1786–1794 RSC.
- J. Liu, L. L. Guo and A. H. Wu,
et al., Immunochromatographic assay for the analysis of methomyl in cabbage and tomato, Food Chem., 2023, 409, 135273 CrossRef CAS PubMed.
- Y. Liu, X. X. Xu and L. Q. Liu,
et al., Development of a GNP-based lateral flow immunoassay for the detection of isoprothiolane in rice samples, Food Chem., 2023, 404, 134483 CrossRef CAS PubMed.
- L. Y. Ye, X. L. Lei and X. X. Xu,
et al., Gold-based paper for antigen detection of monkeypox virus, Analyst, 2023, 148(5), 985–994 RSC.
- L. L. Guo, X. X. Xu and J. Zhao,
et al., Multiple detection of 15 triazine herbicides by gold nanoparticle based-paper sensor, Nano Res., 2022, 15, 5483–5491 CrossRef CAS PubMed.
- G. Frens, Controlled nucleation for regulation of particle size inmonodisperse gold suspensions, Nat. Phys. Sci., 1973, 241, 20–22 CrossRef CAS.
- L. Zeng, X. X. Xu and S. S. Song,
et al., Synthesis of haptens and gold-based immunochromatographic paper sensor for vitamin B6 in energy drinks and dietary supplements, Nano Res., 2022, 15, 2479–2488 CrossRef CAS.
- Z. X. Wang, J. Zhao and X. X. Xu,
et al., An overview for the nanoparticles-based quantitative lateral flow assay, Small Methods, 2022, 6(1), 2101143 CrossRef CAS PubMed.
- W. Xiao, C. H. Huang and F. Xu,
et al., A simple and compact smartphone-based device for the quantitative readout of colloidal gold lateral flow immunoassay strips, Sens. Actuators, B, 2018, 266, 63–70 CrossRef CAS PubMed.
- G. Li, A. P. Wang and Y. M. Chen,
et al., Development of a colloidal gold-based immunochromatographic strip for rapid detection of severe acute respiratory syndrome coronavirus 2 spike protein, Front. Immunol., 2021, 12, 635677 CrossRef CAS PubMed.
- X. L. Huang, Z. P. Aguilar and H. Y. Xu,
et al., Membrane-based lateral flow immunochromatographic strip with nanoparticles as reporters for detection: A review, Biosens. Bioelectron., 2016, 75, 166–180 CrossRef CAS PubMed.
- T. Putnin, A. Ngamaroonchote and N. Wiriyakun,
et al., Dually functional polyethylenimine-coated gold nanoparticles: a versatile material for electrode modification and highly sensitive simultaneous determination of four tumor markers, Microchim. Acta, 2019, 186, 305 CrossRef PubMed.
- J. Hu, Y. Z. Jiang and L. L. Wu,
et al., Dual-signal readout nanospheres for rapid point-of-care detection of ebola virus glycoprotein, Anal. Chem., 2017, 89(24), 13105–13111 CrossRef CAS PubMed.
- Z. S. Gan, S. C. Stein and R. Swanson,
et al., Blood biomarkers for traumatic brain injury: a quantitative assessment of diagnostic and prognostic accuracy, Front. Neurol., 2019, 10, 00446 CrossRef PubMed.
- S. Mondello, A. Sorinola and E. Czeiter,
et al., Blood-based protein biomarkers for the management of traumatic brain injuries in adults presenting to emergency departments with mild brain injury: a living systematic review and meta-analysis., J. Neurotrauma, 2021, 38(8), 1086–1106 CrossRef PubMed.
- E. P. Thelin, L. Johannesson and D. Nelson,
et al., S100B is an important outcome predictor in traumatic brain injury., J. Neurotrauma, 2013, 30(7), 519–528 CrossRef PubMed.
|
This journal is © The Royal Society of Chemistry 2023 |
Click here to see how this site uses Cookies. View our privacy policy here.