DOI:
10.1039/D2QO00045H
(Research Article)
Org. Chem. Front., 2022,
9, 2169-2175
Radical hydrotrifluoromethylation of ynamides: a route toward β-CF3 enamides†
Received
12th January 2022
, Accepted 1st March 2022
First published on 2nd March 2022
Abstract
We report here a radical hydrotrifluoromethylation of ynamides to provide an alternative route toward β-CF3 enamides. By using PhICF3Cl as the CF3 reagent and DMF as the H-donor, the reaction occurred smoothly in the presence of NaH at room temperature. Further reduction of the resulting β-CF3 enamides efficiently delivered β-CF3 amines. Gram-scale synthesis was conducted to demonstrate the practicability of the method.
Introduction
Molecules containing two powerful functionals, trifluoromethyl and amino, in adjacent positions are valuable targets in drug design and synthetic chemistry.1 Consequently, great efforts have been focused on the assembly of amino and trifluoromethyl at the ortho position.2 In this field, the aminotrifluoromethylation of alkenes provides the main and step-economical route toward β-CF3 amines (Scheme 1A).2i,j On the other hand, enamines have found wide synthetic applications,3 which also encouraged investigations on the vicinal trifluoromethylated enamines. However, to date, there have been limited approaches established for preparing β-CF3 enamines. Apart from several works on utilizing the amination of pre-synthesized CF3-containing substrates,4 as well as a recent report involving a three-component reaction between trifluorodiazoethane, aldehydes, and imines,5 trifluoromethylation-based methods have been documented to deliver β-CF3 enamines, including via the aminotrifluoromethylation of alkynes (Scheme 1A)6 and direct Csp2–H trifluoromethylation of enamines (Scheme 1B).7
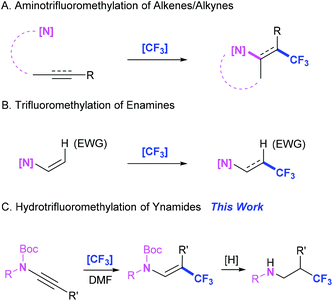 |
| Scheme 1 Trifluoromethylation-based route toward β-CF3 amines/enamines. | |
During our ongoing explorations in the field of trifluoromethylation, an externally coordinated CF3-containing λ3-iodane (PhICF3Cl)8 was synthesized to be used in a set of trifluoromethylation reactions.9 Efficient trifluoromethylative bifunctionalization reactions of alkenes have been developed by our group recently.9a–d In order to extend the synthetic applications of PhICF3Cl, the trifluoromethylation of alkynes was investigated in the follow-up work. As a result, when ynamides10 were chosen as alkyne substrates for the reaction with PhICF3Cl in DMF, unprecedented β-CF3 enamides were produced. It provides an alternative and mild route toward β-CF3 enamides. β-CF3 amines were obtained by further reduction. Herein, we report a new hydrotrifluoromethylation of ynamides (Scheme 1C).
Results and discussion
It was reported that CF3 radical addition onto N-benzoyl ynamides afforded aryltrifluoromethylative cyclization products.11 In order to avoid such possible side reactions, tert-butyl ethynyl(phenyl)carbamate 1a was chosen in our initial attempt to carry out the trifluoromethylation of ynamides using PhICF3Cl as the trifluoromethylating reagent. Notably, the hydrotrifluoromethylation product 2a was obtained in 57% NMR yield, accompanied by 3a in 36% NMR yield only by stirring a mixture of 1a and PhICF3Cl in DMF at room temperature (Table 1, entry 1). DMF was likely the H-donor in such a simple reaction mixture and a radical process was proposed for the formation of 2a. According to our previous work in which radical trifluoromethylation of alkenes with PhICF3Cl could not occur at room temperature without the assistance of a reductant,9d ynamide substrate 1a likely acted as a tertiary amine type electron donor12 for the generation of the CF3 radical from PhICF3Cl. Thus, an additional reductant was used for the above hydrotrifluoromethylation of 1a. As we hypothesized, the addition of tertiary amines, including triethylamine (TEA), 1,8-diazabicyclo[5.4.0]undec-7-ene (DBU) and N,N-diisopropylethylamine (DIPEA), could indeed help increase the yield of 2a a little bit (entries 2–4). Tetrabutylammonium iodide (TBAI) and zinc iodide showed poor performance (entries 5 and 6), and triethylsilane had no obvious influence on this reaction (entry 7). In comparison, NaH was proven to be an optimal reductant, leading to 2a in 81% NMR yield (entry 8). No 3a was detected in these cases (entries 2–6 and 8). Solvent screening showed that 2a could also be obtained in good to moderate yields in N,N-dimethylacetamide (DMAc, entry 9), tetrahydrofuran (THF, entry 10) and N-methylpyrrolidone (NMP, entry 11). However, dichloromethane (DCM, entry 12) was not suitable for the reaction. The reaction time was then controlled precisely (entries 13–15). It was found that the NMR yield of 2a was 75% within 2 h (entry 14). Finally, a gram-scale experiment was conducted and 2a was obtained in 70% isolated yield, which demonstrated the robustness of the protocol (entry 16).
Table 1 Optimization of reaction conditionsa
After establishing the optimal conditions for this reaction (Table 1, entry 14), the generality of this protocol was examined by using various kinds of ynamide substrates (Table 2).
Table 2 Substrate scope of hydrotrifluoromethylation of ynamidesa,b
Reaction conditions: 1 (0.2 mmol), PhICF3Cl (0.3 mmol, 1.5 eq.), NaH (0.3 mmol, 1.5 eq.), DMF (2.0 mL), 25 °C, N2, 2 h.
Isolated yields of E-2 unless the E/Z ratio is otherwise noted in the brackets.
Reaction conditions: 30 °C, 12 h.
|
|
First, a series of N-phenyl-substituted ynamides were selected and tested in this reaction. We were pleased to see that electronically and sterically distinct substituents at different positions were tolerated, affording the β-CF3 enamides (2b–x) in good yields. Ynamides bearing electron-withdrawing groups, such as halide (1b–e, 1q, 1t, and 1w), fluoroalkyl (1p) or carbonyl groups (1k and 1l), could all provide the desired products in good yields. Electron-rich substituents like alkyl (1f–h, 1j, 1r, 1u and 1v), aryl (1i), and alkoxyl/aryloxy (1m–o, 1s, and 1x) did not have obvious influences on the reactions. Remarkably, enamide 2w could be generated in 90% yield from the reaction of sterically hindered ynamide 1w. Secondly, the protecting groups of the ynamides were tested. Both N-Ac and N-Cbz ynamides were suitable for this reaction although the reactions afforded 2a and 2aa in reduced yields under identical conditions. However, p-toluenesulfonyl-ynamide 1ab was not suitable for this reaction. The reaction was complex and the desired product 2ab was not detected. Next, hydrotrifluoromethylations of N-benzyl ynamides were carried out under identical conditions. A series of N-benzyl ynamides substituted at different positions were selected and tested in this reaction. It was found that all the tested N-benzyl ynamides 1ac–ag were efficiently transformed into the corresponding products 2ac–ag. In addition, the enamide product 2ah could also be generated from N-phenylethyl ynamide 1ah in 70% yield.
Finally, non-terminal ynamides were synthesized to test the substrate scope. As shown in Table 2, the desired products 2 could be obtained in moderate to good yields when the reaction time was prolonged. Long-chain alkyl- (1ai–an), cycloalkyl- (1ao) and ester-ynamides (2ap) all proved to be suitable for this reaction. In comparison, phenyl substituted ynamide 1aq delivered 2aq in 28% yield, along with the generation of regiomeric isomers.13 Note that the hydrotrifluoromethylation of ynamides 1 stereoselectively afforded enamines 2. Thermodynamically stable E-isomeric enamines were obtained as the main products in most cases, which was proved by the X-ray crystal structure of 2e.14 In comparison, lower stereoselectivities were observed in some cases, such as 2am, 2an and 2ap, likely caused by the little difference in the thermodynamic stability of the products.
As described above, we have established a novel hydrotrifluoromethylation reaction of ynamides, which provides a trifluoromethylative bifunctionalization pathway to synthesize β-CF3 enamides. Further transformation of β-trifluoromethyl enamides 2 was investigated to highlight the synthetic potential of the approach. As shown in Table 3, by using silyl hydride as the reductant, reduction/deprotection products 4, β-CF3 amines,1a,b,15 could be obtained in good to excellent yields in the presence of trifluoroacetic acid.16 β-CF3 enamides generated from substituted N-phenyl ynamides (1a, 1h, 1j, 1t, 1v, 1w, and 1x), N-benzyl ynamide (1ae) and non-terminal ynamides (1ai, 1ak, 1an and1ao), were all suitable for this reductive deprotection reaction to generate the corresponding β-CF3 amines 4a–l.
Table 3 Deprotected reduction of β-CF3 enaminesa,b
Reaction conditions: 2 (0.1 mmol), Et3SiH (0.15 mmol, 1.5 eq.), TFA (1.0 mL), 0 °C, N2, 24 h.
Isolated yields of 4.
|
|
A set of control experiments were conducted to verify the possible reaction mechanism. Radical trapping experiments were carried out under the standard conditions by the addition of 2,2,6,6-tetramethylpiperidine-1-oxide (TEMPO, Scheme 2A). The desired product 2a could not be obtained in the presence or absence of NaH, which suggested the involvement of a radical process. A radical clock experiment was also conducted by using N,N-diallyl-4-methylbenzenesulfonamide under the standard reaction conditions (Scheme 2B). As a result, both chloro-9d and hydro-cyclization products17 were obtained to demonstrate a radical mechanism. To identify the hydrogen source, deuterated DMF was used in the reaction of 1a. As shown in Scheme 2C, the reactions were carried out in DMF-d7 with or without NaH, and the deuterated product 2a-d1 was obtained, demonstrating an H-abstraction process from the solvent involved in the reaction. No deuterated β-CF3 enamide was detected when D2O was added in this reaction (Scheme 2D), which excluded the formation of 2avia protonation from water; meanwhile, the deuterated chloro-enamide 3a-d1 was detected as the background product. To further verify the formation of 3a from an electrophilic addition of alkynes, we performed an additional reaction of 1a in DMF using TMSCl as the Cl-source instead of PhICF3Cl. 3a was obtained in 50% yield, suggesting that a background reaction existed in our hydrotrifluoromethylation of ynamides 1. This experimental result indicated that NaH may also help in the removal of water from the reaction mixture, which is beneficial for inhibiting the formation of byproducts 3. Further investigations on the role of NaH were carried out by the reaction of 1a and PhICF3Cl in the presence of desiccants without NaH (Scheme 2F). As a result, the formation of 3a was inhibited, but the yield of 2a was still moderate. These experimental results showed that NaH not only acted as a water removal reagent but also assisted in the radical hydrotrifluoromethylation of ynamides.
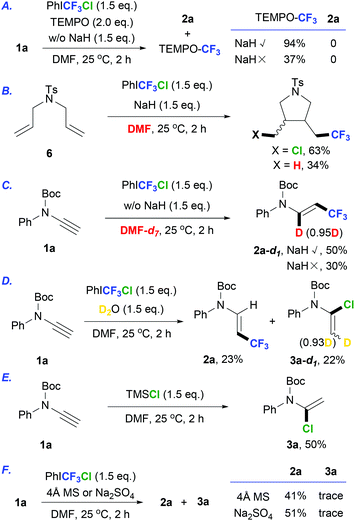 |
| Scheme 2 Control experiments. | |
According to the literature12c,18 and experiment results, a plausible mechanism is proposed as described in Scheme 3. PhICF3Cl is reduced by NaH to release the trifluoromethyl radical, which is added to substrate 1 immediately to generate alkenyl radical intermediate I. Meanwhile, iodobenzene and hydrogen19 are generated, respectively, suggesting that NaH acts as a reductant of PhICF3Cl through an electron transfer pathway. An H-atom-abstraction of I from the solvent DMF subsequently occurred and gave the hydrotrifluoromethylation product 2. In this case, it is proposed that the N-methyl-H of DMF is abstracted20 by the electrophilic trifluoromethylated radical I, which is supported by the fact that good transformations could also be obtained in either DMAc or NMP (Table 1, entries 9 and 10). The generated DMF-based carbon radical would further undergo dimerization, oxidation, hydrolysis, or other processes, resulting in the formation of mixed products.21 Note that although this proposed mechanism is preferred, other plausible H-abstraction processes or reaction mechanisms cannot be ruled out at this stage.
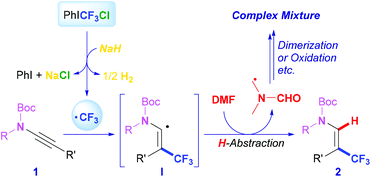 |
| Scheme 3 A plausible radical mechanism. | |
Conclusions
In conclusion, by using PhICF3Cl as the trifluoromethyl source, the hydrotrifluoromethylation of ynamides was achieved successfully, in which a series of β-CF3 enamides were synthesized efficiently. The mild and transition-metal free reaction conditions, the good functional group tolerance and the capability to be performed at the gram scale make this method valuable and practical. Control experiments proved that the reaction occurred via a radical process, which is a new achievement in the field of underexplored free radical reactions of ynamides.
Author contributions
W. Huang and R. Z. Zhang performed the experiments and co-wrote the ESI.† W. Huang wrote the initial draft of this manuscript. R. X. Zhang and J. Yu helped in preparing several substrates. Prof. M. Wang conceived the project and concept reviewed the manuscript. All authors read and contributed to the revisions and approved the final version to be published.
Conflicts of interest
There are no conflicts to declare.
Acknowledgements
We thank the National Natural Science Foundation of China (21672032) and the Department of Science and Technology of Jilin Province (20200201067JC) for funding this work.
Notes and references
-
(a) Q. Li, W. Wang, K. B. Berst, A. Claiborne, L. Hasvold, K. Raye, M. Tufano, A. Nilius, L. L. Shen, R. Flamm, J. Alder, K. Marsh, D. Crowell, D. T. W. Chu and J. J. Plattner, Synthesis and Structure-Activity Relationships of 2-Pyridones: II. 8-(Fluoro-Substituted Pyrrolidinyl)-2-Pyridones as Antibacterial Agents, Bioorg. Med. Chem. Lett., 1998, 8, 1953–1958 CrossRef CAS PubMed;
(b) J. A. Sikorski, Oral Cholesteryl Ester Transfer Protein (CETP) Inhibitors: A Potential New Approach for Treating Coronary Artery Disease, J. Med. Chem., 2006, 49, 1–22 CrossRef CAS PubMed;
(c) I. I. Gerus, R. V. Mironets, E. N. Shaitanova and V. P. Kukhar, Synthesis of New β-Trifluoromethyl Containing GABA and β-Fluoromethyl Containing N-Benzylpyrrolidinones, J. Fluorine Chem., 2010, 131, 224–228 CrossRef CAS;
(d) T. Borisova, N. Pozdnyakova, E. Shaitanova, I. Gerus, M. Dudarenko, R. Mironets, G. Haufe and V. Kukhar, Synthesis of New Fluorinated Analogs of GABA, Pregabalin Bioisosteres, and Their Effects on [3H]GABA Uptake by Rat Brain Nerve Terminals, Bioorg. Med. Chem., 2015, 23, 4316–4323 CrossRef CAS PubMed.
- For selected reviews, see:
(a) H. Egami and M. Sodeoka, Trifluoromethylation of Alkenes with Concomitant Introduction of Additional Functional Groups, Angew. Chem., Int. Ed., 2014, 53, 8294–8308 CrossRef CAS PubMed;
(b) E. Merino and C. Nevado, Addition of CF3 Across Unsaturated Moieties: A Powerful Functionalization Tool, Chem. Soc. Rev., 2014, 43, 6598–6608 RSC;
(c) C. Alonso, E. M. de Marigorta, G. Rubiales and F. Palacios, Carbon Trifluoromethylation Reactions of Hydrocarbon Derivatives and Heteroarenes, Chem. Rev., 2015, 115, 1847–7935 CrossRef CAS PubMed;
(d) P. Gao, X.-R. Song, X.-Y. Liu and Y.-M. Liang, Recent Developments in the Trifluoromethylation of Alkynes, Chem. – Eur. J., 2015, 21, 7648–7661 CrossRef CAS PubMed;
(e) T. Koike and M. Akita, A Versatile Strategy for Difunctionalization of Carbon-Carbon Multiple Bonds by Photoredox Catalysis, Org. Chem. Front., 2016, 3, 1345–1349 RSC;
(f) T. Koike and M. Akita, Fine Design of Photoredox Systems for Catalytic Fluoromethylation of Carbon-Carbon Multiple Bonds, Acc. Chem. Res., 2016, 49, 1937–1945 CrossRef CAS PubMed;
(g) E. H. Oh, H. J. Kim and S. B. Han, Recent Developments in Visible-Light-Catalyzed Multicomponent Trifluoromethylation of Unsaturated Carbon-Carbon Bonds, Synthesis, 2018, 50, 3346–3358 CrossRef CAS;
(h) Y. Tian, S. Chen, Q.-S. Gu, J.-S. Lin and X.-Y. Liu, Amino- and Azidotrifluoromethylation of Alkenes, Tetrahedron Lett., 2018, 59, 203–215 CrossRef CAS;
(i) H. Jiang and A. Studer, Intermolecular Radical Carboamination of Alkenes, Chem. Soc. Rev., 2020, 49, 1790–1811 RSC;
(j) S. Barata-Vallejo and A. Postigo, New Visible-Light-Triggered Photocatalytic Trifluoromethylation Reactions of Carbon-Carbon Multiple Bonds and (Hetero)Aromatic Compounds, Chem. – Eur. J., 2020, 26, 11065–11084 CrossRef CAS PubMed;
(k) K. Keerthika, S. Nath and K. Geetharani, Transition-Metal-Free Trifluoromethylative Difunctionalization of Olefins and Alkynes: Approaches and Challenges Ahead, Catal. Sci. Technol., 2020, 10, 7142–7159 RSC.
-
(a) H. Hayashi, A. Kaga and S. Chiba, Application of Vinyl Azides in Chemical Synthesis: A Recent Update, J. Org. Chem., 2017, 82, 11981–11989 CrossRef CAS PubMed;
(b) V. A. Bakulev, T. Beryozkina, J. Thomas and W. Dehaen, The Rich Chemistry Resulting from the 1,3-Dipolar Cycloaddition Reactions of Enamines and Azides, Eur. J. Org. Chem., 2018, 262–294 CrossRef CAS;
(c) Y.-Q. Zou, F. M. Hörmann and T. Bach, Iminium and Enamine Catalysis in Enantioselective Photochemical Reactions, Chem. Soc. Rev., 2018, 47, 278–290 RSC;
(d) L. Zhu, D. Wang, Z. Jia, Q. Lin, M. Huang and S. Luo, Catalytic Asymmetric Oxidative Enamine Transformations, ACS Catal., 2018, 8, 5466–5484 CrossRef CAS.
-
(a) V. M. Muzalevskiy, V. G. Nenajdenko, A. Y. Rulev, I. A. Ushakov, G. V. Romanenko, A. V. Shastin, E. S. Balenkova and G. Haufe, Selective Synthesis of α-Trifluoromethyl-β-Aryl Enamines or Vinylogous Guanidinium Salts by Treatment of β-Halo-β-Trifluoromethylstyrenes with Secondary Amines under Different Conditions, Tetrahedron, 2009, 65, 6991–7000 CrossRef CAS;
(b) A. Y. Rulev, I. A. Ushakov, E. V. Kondrashov, V. M. Muzalevskiy, A. V. Shastin and V. G. Nenajdenko, Mechanistic Study of Multi-Step Nucleophilic Substitution for Trifluoromethylated Styrenes, J. Fluorine Chem., 2011, 132, 945–950 CrossRef CAS;
(c) I. W. Davies, D. M. Tellers, C. S. Shultz, F. J. Fleitz, D. Cai and Y. Sun, A [2+2] Cycloaddition Route to Dimethylaminomethylene Vinamidinium Salts, Org. Lett., 2002, 4, 2969–2972 CrossRef CAS PubMed;
(d) H. Yamanaka, K. Shiomi and T. Ishihara, Reactions of Quaternary Ammonium Salt Having a Polyfluoroalkenyl Group with Secondary Amines. A New Convenient and Stereoselective Synthesis of β-Trifluoromethylated Enamines, Tetrahedron Lett., 1995, 36, 7267–7270 CrossRef CAS.
- A. Kumar, A. Dhami, J. Fairoosa, R. Kant and K. Mohanan, Silver-Catalyzed Direct Synthesis of Trifluoromethylated Enaminopyridines and Isoquinolinones Employing Trifluorodiazoethane, Org. Lett., 2021, 23, 5815–5820 CrossRef CAS PubMed.
-
(a) G.-C. Ge, X.-J. Huang, C.-H. Ding, S.-L. Wan, L.-X. Dai and X.-L. Hou, A New Strategy to Construct a C=C-CF3 Subunit via CuBr-Catalyzed Domino Reaction of Homopropargyl Amines: An Efficient Synthesis of Trifluoromethyl Containing Building Blocks 4-Trifluoromethyl-2,3-Dihydro-Pyrroliums, Chem. Commun., 2014, 50, 3048–3051 RSC;
(b) G. C. Ge, X. J. Huang, C. H. Ding, H. Li, S. Wan and X. Hou, CuBr-Promoted Tandem Cyclization/Trifluoromethylation of 2-Alkynylanilines: Efficient Synthesis of 3-Trifluoromethylindoles, Chin. J. Chem., 2014, 32, 727–733 CrossRef CAS;
(c) F. Wang, N. Zhu, P. Chen, J. Ye and G. Liu, Copper-Catalyzed Trifluoromethylazidation of Alkynes: Efficient Access to CF3-Substituted Azirines and Aziridines, Angew. Chem., Int. Ed., 2015, 54, 9356–9360 CrossRef CAS PubMed;
(d) Y.-T. He, Q. Wang, J. Zhao, X.-Y. Liu, P.-F. Xu and Y.-M. Liang, The Copper-Catalyzed Synthesis of β-Trifluoromethylated Acrylonitriles and Trifluoromethyl-Substituted 2H-Azirines, Chem. Commun., 2015, 51, 13209–13212 RSC;
(e) Y. Xiang, Y. Kuang and J. Wu, Generation of Benzosultams via Trifluoromethylation of 2-Ethynylbenzenesulfonamide under Visible Light, Org. Chem. Front., 2016, 3, 901–905 RSC;
(f) Y. Ye, K. P. S. Cheung, L. He and G. C. Tsui, Domino Cyclization/Trifluoromethylation of 2-Alkynylanilines Using Fluoroform-Derived CuCF3: Synthesis of 3-(Trifluoromethyl)indoles, Org. Chem. Front., 2018, 5, 1511–1515 RSC;
(g) H. S. Han, E. H. Oh, Y.-S. Jung and S. B. Han, Photoredox-Catalyzed Trifluoromethylative Intramolecular Cyclization: Synthesis of CF3-Containing Heterocyclic Compounds, Org. Lett., 2018, 20, 1698–1702 CrossRef CAS PubMed;
(h) J. Ge, Q. Ding, X. Wang and Y. Peng, Three-Component Cascade Synthesis of Fully Substituted Trifluoromethyl Pyrroles via A Cu(II)/Rh(III)-Promoted Aza-Michael Addition/Trifluoromethylation Cyclization/Oxidation Reaction, J. Org. Chem., 2020, 85, 7658–7665 CrossRef CAS PubMed.
-
(a) C. Feng and T.-P. Loh, Copper-Catalyzed Olefinic Trifluoromethylation of Enamides at Room Temperature, Chem. Sci., 2012, 3, 3458–3462 RSC;
(b) Y. Ohtsuka, D. Uraguchi, K. Yamamoto, K. Tokuhisa and T. Yamakawa, Fenton Reagent-Catalyzed Trifluoromethylation of Enamines of 3-Oxocarboxylates with CF3I, J. Fluorine Chem., 2016, 181, 1–6 CrossRef CAS;
(c) H. Jiang, W. Huang, Y. Yu, S. Yi, J. Li and W. Wu, Transition-Metal-Free Synthesis of β-Trifluoromethylated Enamines with Trifluoromethanesulfinate, Chem. Commun., 2017, 53, 7473–7476 RSC.
- C. Xu, X. Song, J. Guo, S. Chen, J. Gao, J. Jiang, F. Gao, Y. Li and M. Wang, Synthesis of Chloro(phenyl)trifluoromethyliodane and Catalyst-Free Electrophilic Trifluoromethylations, Org. Lett., 2018, 20, 3933–3937 CrossRef CAS PubMed.
-
(a) J. Guo, C. Xu, X. Liu and M. Wang, Aryltrifluoromethylative Cyclization of Unactivated Alkenes by the Use of PhICF3Cl under Catalyst-Free Conditions, Org. Biomol. Chem., 2019, 17, 2162–2168 RSC;
(b) J. Guo, C. Xu, L. Wang, W. Huang and M. Wang, Catalyst-Free and Selective Trifluoromethylative Cyclization of Acryloanilides Using PhICF3Cl, Org. Biomol. Chem., 2019, 17, 4593–4599 RSC;
(c) C. Xu, W. Huang, R. Zhang, C. Gao, Y. Li and M. Wang, Trifluoromethylations of Alkenes Using PhICF3Cl as Bifunctional Reagent, J. Org. Chem., 2019, 84, 14209–14216 CrossRef CAS PubMed;
(d) W. Huang, C. Xu, J. Yu and M. Wang, ZnI2-Catalyzed Aminotrifluoromethylation Cyclization of Alkenes Using PhICF3Cl, J. Org. Chem., 2021, 86, 1987–1999 CrossRef CAS PubMed;
(e) R. Z. Zhang, R. X. Zhang, S. Wang, C. Xu, W. Guan and M. Wang, An N-Trifluoromethylation/Cyclization Strategy for Accessing Diverse N-Trifluoromethyl Azoles from Nitriles and 1,3-Dipoles, Angew. Chem., Int. Ed., 2022, 61, e202110749 CAS.
- For selected reviews, see:
(a) C. C. Lynch, A. Sripada and C. Wolf, Asymmetric Synthesis with Ynamides: Unique Reaction Control, Chemical Diversity and Applications, Chem. Soc. Rev., 2020, 49, 8543–8583 RSC;
(b) Y.-B. Chen, P.-C. Qian and L.-W. Ye, Brønsted Acid-Mediated Reactions of Ynamides, Chem. Soc. Rev., 2020, 49, 8897–8909 RSC;
(c) F.-L. Hong and L.-W. Ye, Transition Metal-Catalyzed Tandem Reactions of Ynamides for Divergent N-Heterocycle Synthesis, Acc. Chem. Res., 2020, 53, 2003–2019 CrossRef CAS PubMed;
(d) C. Mahe and K. Cariou, Ynamides in Free Radical Reactions, Adv. Synth. Catal., 2020, 362, 4820–4832 CrossRef CAS;
(e) J. Luo, G.-S. Chen, S.-J. Chen, J.-S. Yu, Z.-D. Li and Y.-L. Liu, Exploiting Remarkable Reactivities of Ynamides: Opportunities in Designing Catalytic Enantioselective Reactions, ACS Catal., 2020, 10, 13978–13992 CrossRef CAS.
- M. Cassé, C. Nisole, H. Dossmann, Y. Gimbert, J.-M. Fourquez, L. Haberkorn, C. Ollivier and L. Fensterbank, Trifluoromethyl Radical Triggered Radical Cyclization of N-benzoyl Ynamides Leading to Isoindolinones, Sci. China: Chem., 2019, 62, 1542–1546 CrossRef.
-
(a) Y. Cheng and S. Yu, Hydrotrifluoromethylation of Unactivated Alkenes and Alkynes Enabled by an Electron-Donor-Acceptor Complex of Togni's Reagent with a Tertiary Amine, Org. Lett., 2016, 18, 2962–2965 CrossRef CAS PubMed;
(b) G. M. F. Batista, P. P. de Castro, H. F. Dos Santos, K. T. de Oliveira and G. W. Amarante, Electron-Donor-Acceptor Complex-Enabled Flow Methodology for the Hydrotrifluoromethylation of Unsaturated β-Keto Esters, Org. Lett., 2020, 22, 8598–8602 CrossRef CAS PubMed;
(c) D. Wei, T. Liu, Y. He, B. Wei, J. Pan, J. Zhang, N. Jiao and B. Han, Radical 1,4/5-Amino Shift Enables Access to Fluoroalkyl-Containing Primary β(γ)-Aminoketones under Metal-Free Conditions, Angew. Chem., Int. Ed., 2021, 60, 26308–26313 CrossRef CAS PubMed.
- A mixture of regiomeric products E/Z-2aq′ was obtained in 35% yield. See the
ESI for details of the characterization data and NMR spectra.†.
- For the crystal data for 2e, please see the ESI. Deposition number 2141492 (2e) contains the supplementary crystallographic data for this paper.†.
-
(a) Y. Yasu, T. Koike and M. Akita, Intermolecular Aminotrifluoromethylation of Alkenes by Visible-Light-Driven Photoredox Catalysis, Org. Lett., 2013, 15, 2136–2139 CrossRef CAS PubMed;
(b) A. Carboni, G. Dagousset, E. Magnier and G. Masson, Photoredox-Induced Three-Component Oxy-, Amino-, and Carbotrifluoromethylation of Enecarbamates, Org. Lett., 2014, 16, 1240–1243 CrossRef CAS PubMed;
(c) J.-S. Lin, F.-L. Wang, X.-Y. Dong, W.-W. He, Y. Yuan, S. Chen and X.-Y. Liu, Catalytic Asymmetric Radical Aminoperfluoroalkylation and Aminodifluoromethylation of Alkenes to Versatile Enantioenriched-Fluoroalkyl Amines, Nat. Commun., 2017, 8, 14841–14851 CrossRef PubMed;
(d) C.-L. Zhu, C. Wang, Q.-X. Qin, S. Yruegas, C. D. Martin and H. Xu, Iron(II)-Catalyzed Azidotrifluoromethylation of Olefins and N-Heterocycles for Expedient Vicinal Trifluoromethyl Amine Synthesis, ACS Catal., 2018, 8, 5032–5037 CrossRef CAS PubMed;
(e) H. Xiao, H. Shen, L. Zhu and C. Li, Copper-Catalyzed Radical Aminotrifluoromethylation of Alkenes, J. Am. Chem. Soc., 2019, 141, 11440–11445 CrossRef CAS PubMed;
(f) P. Wang, S. Zhu, D. Lu and Y. Gong, Intermolecular Trifluoromethyl-Hydrazination of Alkenes Enabled by Organic Photoredox Catalysis, Org. Lett., 2020, 22, 1924–1928 CrossRef CAS PubMed.
- J. Yao, C.-L. Li, X. Fan, Z. Wang, Z.-X. Yu and J. Zhao, Ynamide Protonation-Initiated Cis-Selective Polyene Cyclization and Reaction Mechanism, CCS Chem., 2021, 3, 3133–3143 CrossRef.
- J.-J. Ma, W.-B. Yi, G.-P. Lu and C. Cai, Decarboxylative and Denitrative Trifluoromethylation for the Synthesis of Cvinyl-CF3 Compounds with Togni (II) Reagent, Adv. Synth. Catal., 2015, 357, 3447–3452 CrossRef CAS.
-
(a) B. Zhang and A. Studer, 2-Trifluoromethylated Indoles via Radical Trifluoromethylation of Isonitriles, Org. Lett., 2014, 16, 1216–1219 CrossRef CAS PubMed;
(b) R. Beniazza, M. Douarre, D. Lastécouères and J.-M. Vincent, Metal-Free and Light-Promoted Radical Iodotrifluoromethylation of Alkenes with Togni Reagent as the Source of CF3 and Iodine, Chem. Commun., 2017, 53, 3547–3550 RSC;
(c) J. Huang, Y. Jia, X. Li, J. Duan, Z.-X. Jiang and Z. Yang, Halotrifluoromethylation of 1,3-Enynes: Access to Tetrasubstituted Allenes, Org. Lett., 2021, 23, 2314–2319 CrossRef CAS PubMed.
- Hydrogen generated during the model reaction was detected by gas chromatography. See the ESI for details.†.
- For selected works where DMF was used as an H-donor, see:
(a) F. W. Wassmundt and W. F. Kiesman, Efficient Catalysis of Hydrodediazoniations in Dimethylformamide, J. Org. Chem., 1995, 60, 1713–1719 CrossRef CAS;
(b) C. M. Tam, C. T. To and K. S. Chan, Carbon-Carbon σ-Bond Transfer Hydrogenation with DMF Catalyzed by Cobalt Porphyrins, Organometallics, 2016, 35, 2174–2177 CrossRef CAS.
- F. W. Wassmundt and W. F. Kiesman, Detection of Aryl Radicals in Hydrodediazoniations, J. Org. Chem., 1997, 62, 8304–8308 CrossRef CAS PubMed.
Footnotes |
† Electronic supplementary information (ESI) available: 1H, 13C, 19F and NOE NMR spectra and crystallographic data. CCDC 2141492. For ESI and crystallographic data in CIF or other electronic format see DOI: 10.1039/d2qo00045h |
‡ These authors contributed equally to this work. |
|
This journal is © the Partner Organisations 2022 |
Click here to see how this site uses Cookies. View our privacy policy here.