DOI:
10.1039/D2QO00127F
(Research Article)
Org. Chem. Front., 2022,
9, 2164-2168
Visible-light-promoted radical amidoarylation of arylacrylamides towards amidated oxindoles†
Received
24th January 2022
, Accepted 25th February 2022
First published on 28th February 2022
Abstract
A visible-light-promoted intermolecular radical amidation/cyclization of arylacrylamides was realized by using N-aminopyridinium salts as the source of amidyl radicals. The reaction exhibits a broad scope and good functional group tolerance, and a variety of amide-tethered-oxindoles were prepared in this way in moderate to good yields.
C–N bond-forming reactions have drawn persistent interest from chemists because of the importance of nitrogen-containing compounds in materials science and in medicinal chemistry. Among the various strategies used for the construction of C–N bonds, those mediated by nitrogen-centered radicals have been gaining prominence, with accumulating studies showing that the high reactivity of N-radicals can be exploited to tackle a number of challenging synthetic problems.1 Multiple methods have been developed for the generation of N-radicals under mild conditions, which greatly enhances their usefulness in organic synthesis.2
The radical amination of alkenes constitutes a highly valuable type of reaction for the preparation of nitrogen-containing compounds.3 Significant progress has been made over the past few years, which is to a great extent attributable to the employment of visible-light photoredox catalysis.4 For instance, excellent protocols have been reported for the anti-Markovnikov hydroamination2f,5,6 and amination/difunctionalization (Scheme 1a).7–10 It is noteworthy that primary amidyl radicals, which have been sparingly employed to react with alkenes because of a lack of an effective means of generation, have begun to be used for the construction of the C–N bond.6–9 Despite this advancement, most of the investigations in this line have focused on protected amidyl radicals, and intermolecular olefin amidation with aryl and alkyl-attached primary amidyl radicals has been much less explored. In view of the great synthetic potential of amidyl radicals as well as the structural importance of amides, it would be highly desirable to broadly investigate the reactivity and efficacy of common amidyl radicals towards the addition of alkenes.
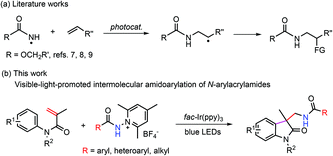 |
| Scheme 1 Photochemical protocols for the intermolecular addition of primary amidyl radicals to alkenes. | |
Oxindoles represent an important class of naturally occurring heterocycles that show highly effective biological and physiological activities.11 Oxindoles can be efficiently prepared from N-phenylacrylamides via radical addition/intramolecular aromatic substitution.12 Our previous study shows that by reacting aminium radicals with N-phenylacrylamides, aminated oxindoles can be accessed readily in good yields.13 Considering the significance of the amidyl group in medicinal chemistry, it would be desirable to incorporate the amidyl group into oxindoles by this strategy. Chang et al. reported an effective photochemical protocol for the preparation of amidated oxindoles through the reaction of benzoyl azides with N-phenylacrylamides.14 We envisioned that by reacting amidyl radicals with N-phenylacrylamides, the scope of the reaction could be expanded. N-Aminopyridinium salts have recently been demonstrated to be highly efficient precursors toward N-centered radicals (Scheme 1b);15,16 they were expected to fulfill our need to deliver the primary aryl and alkyl amidyl radicals under mild conditions. Indeed, our results verified the viability of our design, and the reaction of N-phenylacrylamides with N-aminopyridinium salts was performed under blue light irradiation with fac-Ir(ppy)3 as the photocatalyst (Scheme 1b). It is worth noting that the reaction exhibited good functional tolerance, and the alkyl-substituted amido group can be introduced into the oxindole motif as well as the aryl and heteroaryl-substituted amido group.
Initially, we selected N-phenylacrylamide 1a and N-aminopyridinium salt 2a as the model substrates to investigate the feasibility of the reaction under visible-light irradiation (40 W Kessil blue LEDs, 50% intensity) in the presence of a photocatalyst (Table 1). A systematic survey of the reaction conditions with variation in the photocatalyst, solvent and base revealed the optimum conditions to be: fac-Ir(ppy)3 as the photocatalyst, K3PO4 as the base in 1,2-dichloroethane (DCE) at room temperature, and blue-light irradiation for 36 h. Under these conditions, the desired product 3a was isolated in good yield (with 2a as the limiting substrate). The structure of 3a was confirmed by X-ray crystallographic analysis (CCDC no. 2122102†).17 Other catalysts, such as Cu(dap)2Cl and eosin Y, were ineffective under the current conditions (Table 1, entries 2 and 3). Replacing DCE with MeCN, dichloromethane (DCM), or CHCl3 resulted in lower yields (entries 4–6). Additionally, the yield was diminished when K2CO3 or Et3N was used as the base or in the absence of a base (entries 7–9). Control experiments indicate that both photocatalyst and light irradiation are necessary for the reaction to take place (entries 10 and 11).
Table 1 Optimization of the reaction conditionsa

|
Entry |
Deviation from the standard conditions |
Yieldb (%) |
Reaction conditions: 1a (0.15 mmol, 1.5 equiv.), 2a (0.1 mmol, 1.0 equiv.), K3PO4 (0.12 mmol, 1.2 equiv.), fac-Ir(ppy)3 (2.0 mol%), DCE (1.0 mL), 40 W Kessil blue LEDs (50% intensity), room temperature, 36 h, under an argon atmosphere.
Isolated yields.
The reaction was conducted at the 0.2 mmol scale.
Reaction time was 24 h. Detailed information concerning the screening of the reaction conditions is presented in Tables S1–S5 in the ESI.†
|
1 |
Standard conditions |
81 (78c) |
2 |
Eosin Y instead of Ir(ppy)3 |
Traced |
3 |
Cu(dap)2Cl instead of Ir(ppy)3 |
N.R. |
4 |
MeCN instead of DCE |
56 |
5 |
DCM instead of DCE |
74 |
6 |
CHCl3 instead of DCE |
64 |
7 |
K2CO3 instead of K3PO4 |
64 |
8 |
Et3N instead of K3PO4 |
41 |
9 |
No base |
61 |
10 |
In the dark |
N.R. |
11 |
No photocatalyst |
N.R. |
To test the generality of this reaction, the scope of arylacrylamides 1 was investigated, and the results are shown in Scheme 2. Both electron-donating and electron-withdrawing groups on the N-phenyl ring were well tolerated, and the corresponding products were obtained in moderate to good yields. Notably, a broad range of functional groups such as the halo (3g–3k) and cyano (3n) groups were well tolerated, which offers the potential for further transformations. This protocol is applicable to gram-scale preparation. As such, 3a was obtained in 61% yield upon isolation when the reaction was performed at the 4.0 mmol scale. However, the expected product 3v was not obtained when the N-unprotected acrylamide was used as the substrate. In this case, the reaction only delivered a complex mixture.
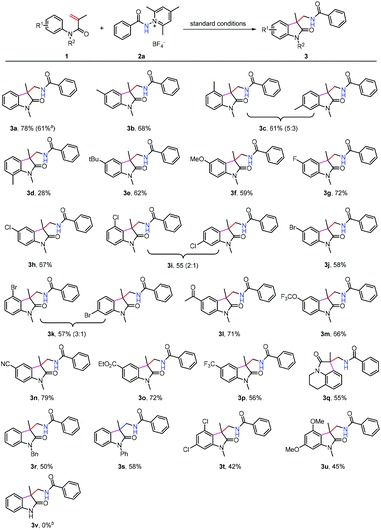 |
| Scheme 2 Scope of arylacrylamides. The reactions were conducted at the 0.2 mmol scale. Isolated yield. a 1a (6.0 mmol, 1.5 equiv.), 2a (4.0 mmol, 1.0 equiv.), base (4.8 mmol, 1.2 equiv.), fac-Ir(ppy)3 (2.0 mol%), DCE (30 mL), 40 W Kessil blue LEDs (100% intensity), room temperature, 42 h, under argon atmosphere. b A complex mixture was generated. | |
We next turned our attention to examine the scope of N-aminopyridinium salts 2 under the standard conditions (Scheme 3). It can be seen that a range of substrates bearing electron-donating and electron withdrawing groups on the phenyl ring of pyridinium salts can be converted to the expected products (3ab–3ah), and heteroaryl aminopyridinium salts such as pyridine (3ai and 3aj) and furan (3ak) were also suitable substrates for the present reaction. Moreover, alkyl and alkyloxyl-substituted amidyl groups can be introduced into the oxindole motif as well, although the yields of 3al–3ap were considerably lower than those obtained using their aryl-substituted counterparts. The lower yields of 3al–3ap might be attributed to the presence of competitive pathways (C–H abstraction from the solvent, for example) that N-alkyl amidyl radicals are liable to undergo. This method did not work for the N-disubstituted aminopyridinium salt 2r, possibly as a result of steric hindrance caused by the N-methyl group.
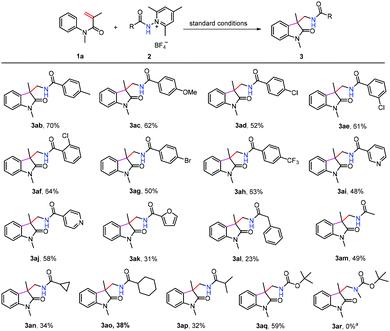 |
| Scheme 3 Scope of aminopyridinium salts. The reactions were conducted at the 0.2 mmol scale. Isolated yields. a No reaction took place. | |
The present reactions are believed to take place following the radical pathway shown in Scheme 4. Taking the reaction of 1a with 2a as an example, it can be seen that the reaction is initiated by the single electron transfer between the N-aminopyridinium salt 2a and the excited [Ir(III)]*, which generates radical A and [Ir(IV)]. Radical A then undergoes fragmentation to give the amidyl radical B, which is subsequently trapped by 1a to produce the radical intermediate C. The latter undergoes cyclization to afford radical D. Oxidation by [Ir(IV)] converts D to the carbocation E, from which 3a is finally generated by deprotonation. K3PO4 has a beneficial effect on the reaction, probably because it can enhance the basicity of the system to make the deprotonation easier. This radical mechanism was supported by the inhibition experiment with 2,2,6,6-tetramethyl-1-piperidinyloxy (TEMPO) and 2,6-di-tert-butyl-4-methylphenol (BHT) (Scheme 5). The reaction of 1a and 2a was completely inhibited in the presence of 2.0 equiv. of TEMPO, and the yield of 3a was significantly decreased when 2.0 equiv. of BHT was added into the reaction vessel. In the latter case, the BHT-trapped product 4 was also detected by HRMS. That the reaction could not take place without fac-Ir(ppy)3 and light irradiation (Table 1, entries 10 and 11) reveals the necessity of photoexcitation of the catalyst during the reaction.
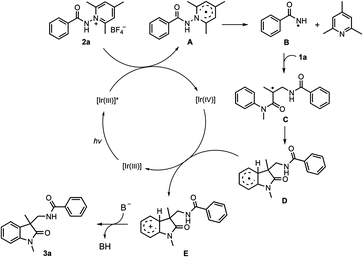 |
| Scheme 4 Proposed mechanism. | |
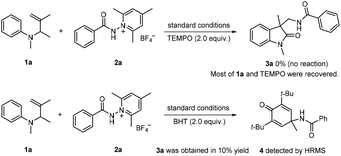 |
| Scheme 5 Inhibition experiment. | |
In summary, we have developed an effective protocol for the preparation of amidyl-attached oxindoles via photoinduced and fac-Ir(ppy)3-catalyzed amidoarylation of arylacrylamides with N-aminopyridinium salts as the amidyl radical precursors. This method allows a variety of substituted arylacrylamides and N-aminopyridinium salts to be converted to the desired products in moderate to good yields. Further attempts to expand the synthetic scope of the intermolecular radical amidation of unactivated olefins are ongoing in our laboratory.
Author contributions
Y.-Z. Wang and W. Yu contributed to the conceptualization of this study. Y.-Z. Wang carried out all the experimental work. Y.-Z. Wang and W. Yu wrote the manuscript. Y.-Z. Wang, W.-J. Lin, H.-C. Liu and W. Yu reviewed the final version of the manuscript.
Conflicts of interest
There are no conflicts to declare.
Acknowledgements
The authors thank the National Natural Science Foundation of China (no. 21772077) and the State Key Laboratory of Applied Organic Chemistry for financial support.
Notes and references
-
(a) S. Z. Zard, Recent progress in the generation and use of nitrogen-centred radicals, Chem. Soc. Rev., 2008, 37, 1603–1618 RSC;
(b) B. Quiclet-Sire and S. Z. Zard, Some aspects of radical chemistry in the assembly of complex molecular architectures, Beilstein. J. Org. Chem., 2013, 9, 557–576 CrossRef CAS PubMed;
(c) T. Xiong and Q. Zhang, New amination strategies based on nitrogen-centered radical chemistry, Chem. Soc. Rev., 2016, 45, 3069–3087 RSC.
-
(a) C.-M. Chou, J. Guin, C. Mück-Lichtenfeld, S. Grimme and A. Studer, Radical-transfer hydroamination of olefins with N-aminated dihydropyridines, Chem. – Asian J., 2011, 6, 1197–1209 CrossRef CAS PubMed;
(b) T. W. Greulich, C. G. Daniliuc and A. Studer,
N-Aminopyridinium, salts as precursors for N-centered radicals-direct amidation of arenes and heteroarenes, Org. Lett., 2015, 17, 254–257 CrossRef CAS PubMed;
(c) J. Davies, S. G. Booth, S. Essafi, R. A. W. Dryfe and D. Leonori, Visible-light-mediated generation of nitrogen-centered radicals: Metal-free hydroimination and iminohydroxylation cyclization reactions, Angew. Chem., Int. Ed., 2015, 54, 14017–14021 CrossRef CAS PubMed;
(d) Y. Xia, L. Wang and A. Studer, Site-selective remote radical C–H functionalization of unactivated C–H bonds in amides using sulfone reagents, Angew. Chem., Int. Ed., 2018, 57, 12940–12944 CrossRef CAS PubMed;
(e) S. P. Morcillo, E. M. Dauncey, J. H. Kim, J. J. Douglas, N. S. Sheikh and D. Leonori, Photoinduced remote functionalization of amides and amines using electrophilic nitrogen radicals, Angew. Chem., Int. Ed., 2018, 57, 12945–12949 CrossRef CAS PubMed;
(f) S. W. Lardy and V. A. Schmidt, Intermolecular radical mediated anti-Markovnikov alkene hydroamination using N-hydroxyphthalimide, J. Am. Chem. Soc., 2018, 140, 12318–12322 CrossRef CAS PubMed;
(g) L.-H. Li, Y. Wei and M. Shi, N-Hydroxyphthalimide imidate esters as amidyl radical precursors in the visible light photocatalyzed C–H amidation of heteroarenes, Org. Chem. Front., 2021, 8, 1935–1940 RSC;
(h) M.-J. Luo, X.-H. Ouyang, Y.-P. Zhu, Y. Li and J.-H. Li, Metal-free electrochemical [3+2] heteroannulation of anilines with pyridines enabled by dual C–H radical aminations, Green Chem., 2021, 23, 9024–9029 RSC.
-
(a) R. S. Neale, Nitrogen radicals as synthetic intermediates. N-halide rearrangements and additions to unsaturated hydrocarbons, Synthesis, 1971, 1–15 CrossRef CAS;
(b)
L. Stella, Nitrogen-centered radicals, in Radicals in Organic Synthesis, ed. P. Renaud and M. P. Sibi, John Wiley & Sons, Ltd., 2001, vol. 2, pp. 407–426 Search PubMed;
(c) H. Jiang and A. Studer, Intermolecular radical carboamination of alkenes, Chem. Soc. Rev., 2020, 49, 1790–1811 RSC.
-
(a) J.-R. Chen, X.-Q. Hu, L.-Q. Lu and W.-J. Xiao, Visible light photoredox-controlled reactions of N-radicals and radical ions, Chem. Soc. Rev., 2016, 45, 2044–2056 RSC;
(b) M. D. Kärkäs, Photochemical generation of nitrogen-centered amidyl, hydrazonyl, and imidyl radicals: Methodology developments and catalytic applications, ACS Catal., 2017, 7, 4999–5022 CrossRef;
(c) Y. Zhao and W. Xia, Recent advances in radical-based C–N bond formation via photo-/electrochemistry, Chem. Soc. Rev., 2018, 47, 2591–2608 RSC;
(d) X.-Y. Yu, Q.-Q. Zhao, J. Chen, W.-J. Xiao and J.-R. Chen, When light meets nitrogen-centered radicals: From reagents to catalysts, Acc. Chem. Res., 2020, 53, 1066–1083 CrossRef CAS PubMed.
-
(a) J. M. Andrew, C. L. Brendan, X. Zhang, G. N. Saeed, C. S. Trevor and R. R. Knowles, Catalytic intermolecular hydroaminations of unactivated olefins with secondary alkyl amines, Science, 2017, 355, 727–730 CrossRef PubMed;
(b) Q. Zhu, D. E. Graff and R. R. Knowles, Intermolecular anti-Markovnikov hydroamination of unactivated alkenes with sulfonamides enabled by proton-coupled electron transfer, J. Am. Chem. Soc., 2018, 140, 741–747 CrossRef CAS PubMed;
(c) D. C. Miller, J. M. Ganley, A. J. Musacchio, T. C. Sherwood, W. R. Ewing and R. R. Knowles, Anti-Markovnikov hydroamination of unactivated alkenes with primary alkyl amines, J. Am. Chem. Soc., 2019, 141, 16590–16594 CrossRef CAS PubMed.
- H. Jiang and A. Studer, Anti-markovnikov radical hydro- and deuteroamidation of unactivated alkenes, Chem. – Eur. J., 2019, 25, 7105–7109 CrossRef CAS PubMed.
-
(a) Q. Qin, Y.-Y. Han, Y.-Y. Jiao, Y. He and S. Yu, Photoredox-catalyzed diamidation and oxidative amidation of alkenes: Solvent-enabled synthesis of 1,2-diamides and α-amino ketones, Org. Lett., 2017, 19, 2909–2912 CrossRef CAS PubMed;
(b) X.-D. An, Y.-Y. Jiao, H. Zhang, Y. Gao and S. Yu, Photoredox-induced radical relay toward functionalized β-amino alcohol derivatives, Org. Lett., 2018, 20, 401–404 CrossRef CAS PubMed;
(c) X.-D. An, H. Zhang, Q. Xu, L. Yu and S. Yu, Stereodivergent synthesis of α-aminomethyl cinnamyl ethers via photoredox-catalyzed radical relay reaction, Chin. J. Chem., 2018, 36, 1147–1150 CrossRef CAS;
(d) X.-D. An and S. Yu, Photoredox-catalyzed radical relay reaction toward functionalized vicinal siamines, Synthesis, 2018, 50, 3387–3394 CrossRef CAS.
-
(a) H. Jiang and A. Studer, Amidyl radicals by oxidation of α-amido-oxy acids: Transition-metal-free amidofluorination of unactivated Alkenes, Angew. Chem., Int. Ed., 2018, 57, 10707–10711 CrossRef CAS PubMed;
(b) H. Jiang and A. Studer, Transition-metal-free three-component radical 1,2-amidoalkynylation of unactivated alkenes, Chem. – Eur. J., 2019, 25, 516–520 CAS;
(c) H. Jiang, G. Seidler and A. Studer, Carboamination of unactivated alkenes through three-component radical conjugate addition, Angew. Chem., Int. Ed., 2019, 58, 16528–16532 CrossRef CAS PubMed;
(d) C. You and A. Studer, Three-component 1,2-carboamination of vinyl boronic esters via amidyl radical induced 1,2-migration, Chem. Sci., 2021, 12, 15765–15769 RSC.
- X. Yi and X. Hu, Intermolecular oxidative amination of unactivated alkenes by dual photoredox and copper catalysis, Chem. Sci., 2021, 12, 1901–1906 RSC.
- J. Shen, J. Xu, L. He, Y. Ouyang, L. Huang, W. Li, Q. Zhu and P. Zhang, Photoinduced rapid multicomponent cascade reaction of aryldiazonium salts with unactivated alkenes and TMSN3, Org. Lett., 2021, 23, 1204–1208 CrossRef CAS PubMed.
-
(a) D. A. Sandham, C. Adcock, K. Bala, L. Barker, Z. Brown, G. Dubois, D. Budd, B. Cox, R. A. Fairhurst, M. Furegati, C. Leblanc, J. Manini, R. Profit, J. Reilly, R. Stringer, A. Schmidt, K. L. Turner, S. J. Watson, J. Willis, G. Williams and C. Wilson, 7-Azaindole-3-acetic acid derivatives: Potent and selective CRTh2 receptor antagonists, Bioorg. Med. Chem. Lett., 2009, 19, 4794–4798 CrossRef CAS PubMed;
(b) M. Ishikura, K. Yamada and T. Abe, Simple indole alkaloids and those with a nonrearranged monoterpenoid unit, Nat. Prod. Rep., 2010, 27, 1630–1680 RSC;
(c) A. J. Kochanowska-Karamyan and M. T. Hamann, Marine indole alkaloids: Potential new drug leads for the control of depression and anxiety, Chem. Rev., 2010, 110, 4489–4497 CrossRef CAS PubMed;
(d) K. Ding, Y. Lu, Z. Nikolovska-Coleska, G. Wang, S. Qiu, S. Shangary, W. Gao, D. Qin, J. Stuckey, K. Krajewski, P. P. Roller and S. Wang, Structure-based design of spiro-oxindoles as potent, specific small-molecule inhibitors of the MDM2–p53 Interaction, J. Med. Chem., 2006, 49, 3432–3435 CrossRef CAS PubMed.
-
(a) G. R. Humphrey and J. T. Kuethe, Practical methodologies for the synthesis of indoles, Chem. Rev., 2006, 106, 2875–2911 CrossRef CAS PubMed;
(b) J.-R. Chen, X.-Y. Yu and W.-J. Xiao, Tandem radical cyclization of N-Arylacrylamides: An emerging platform for the construction of 3,3-disubstituted oxindoles, Synthesis, 2015, 47, 604–629 CrossRef CAS;
(c) J. Singh and A. Sharma, Visible light mediated synthesis of oxindoles, Adv. Synth. Catal., 2021, 363, 4284–4308 CrossRef CAS.
- Y.-Z. Wang, W.-J. Lin, J.-Y. Zou, W. Yu and X.-Y. Liu, Preparation of oxindoles via visible-light-induced amination/cyclization of arylacrylamides with alkyl amines, Adv. Synth. Catal., 2020, 362, 3116–3120 CrossRef CAS.
- D. B. Bagal, S.-W. Park, H.-J. Song and S. Chang, Visible light sensitization of benzoyl azides: cascade cyclization toward oxindoles via a non-nitrene pathway, Chem. Commun., 2017, 53, 8798–8801 RSC.
-
(a) T. W. Greulich, C. G. Daniliuc and A. Studer, N-Aminopyridinium salts as precursors for N-centered radicals –direct amidation of arenes and heteroarenes, Org. Lett., 2015, 17, 254–257 CrossRef CAS PubMed;
(b) S. Jung, H. Lee, Y. Moon, H.-Y. Jung and S. Hong, Site-selective C–H acylation of pyridinium derivatives by photoredox catalysis, ACS Catal., 2019, 9, 9891–9896 CrossRef CAS;
(c) Y. Moon, B. Park, I. Kim, G. Kang, S. Shin, D. Kang, M.-H. Baik and S. Hong, Visible light induced alkene aminopyridylation using N-aminopyridinium salts as bifunctional reagents, Nat. Commun., 2019, 10, 4117–4125 CrossRef PubMed;
(d) D. Forster, W. Guo, Q. Wang and J. Zhu, Photoredox catalytic three-component amidoazidation of 1,3-dienes, ACS Catal., 2021, 11, 10871–10877 CrossRef CAS;
(e) W. Guo, Q. Wang and J. Zhu, Selective 1,2-aminoisothiocyanation of 1,3-dienes under visible-light photoredox catalysis, Angew. Chem., Int. Ed., 2021, 60, 4085–4089 CrossRef CAS PubMed;
(f) W.-L. Yu, H.-W. Jiang, L. Yan, Z.-T. Feng, Y.-C. Luo and P.-F. Xu, Visible-light induced generation of bifunctional nitrogen-centered radicals: a concise synthetic strategy to construct bicyclo[3.2.1] octane and azepane cores, Sci. China: Chem., 2021, 64, 274–280 CrossRef CAS;
(g) K. Miyazawa, T. Koike and M. Akita, Aminohydroxylation of olefins with iminopyridinium ylides by dual Ir photocatalysis and Sc(OTf)3 catalysis, Tetrahedron, 2016, 72, 7813–7820 CrossRef CAS.
- For reviews, see:
(a) S. L. Rössler, B. J. Jelier, E. Magnier, G. Dagousset, E. M. Carreira and A. Togni, Pyridinium salts as redox-active functional group transfer reagents, Angew. Chem., Int. Ed., 2020, 59, 9264–9280 CrossRef PubMed;
(b) F.-S. He, S. Ye and J. Wu, Recent advances in pyridinium salts as radical reservoirs in organic synthesis, ACS Catal., 2019, 9, 8943–8960 CrossRef CAS.
- CCDC 2122102† (N-((1,3-dimethyl-2-oxoindolin-3-yl)methyl)benzamide, 3a) contains the supplementary crystallographic data for this paper.
Footnote |
† Electronic supplementary information (ESI) available. CCDC 2122102. For ESI and crystallographic data in CIF or other electronic format see DOI: 10.1039/d2qo00127f |
|
This journal is © the Partner Organisations 2022 |
Click here to see how this site uses Cookies. View our privacy policy here.