DOI:
10.1039/D2QM00569G
(Research Article)
Mater. Chem. Front., 2022,
6, 2741-2749
Achievement of high efficiency and thermally stable near-infrared phosphors by designing a chromium crystallographic environment for nondestructive testing and night vision†
Received
14th June 2022
, Accepted 3rd August 2022
First published on 4th August 2022
Abstract
Clarification of the effect of the crystallographic environment of Cr3+ ions on its luminescence properties is essential for the construction of novel stable and efficient near-infrared (NIR) phosphors. To this end, we investigated a series of XTaO4:Cr3+ (X = In, Sc, and Ga) phosphors with Cr3+ ions occupying the [XO6] crystallographic sites. And the thermal stability increased from 8% to 73% and the IQE increased from 36% to 82.6% when X changed from In to Ga. An analysis of the internal reasons for the dramatic changes in the luminescence properties caused by Cr3+ ions occupying different crystallographic site environments has been performed in detail. With the order of X = In, Sc, and Ga, the Debye temperature of XTaO4:Cr3+ was significantly enhanced and the electron–phonon coupling effect was gradually reduced, and as a result, GaTaO4:Cr3+ with desirable structural rigidity has a high internal/external quantum efficiency (IQE/EQE: 82.6%/43.5%) and good thermal stability (I423K = 73%). Finally, NIR phosphor-converted light-emitting diodes (pc-LEDs) were fabricated by combining XTaO4:Cr3+ (X = In, Sc, and Ga) with blue LED chips and demonstrated for applications in nondestructive testing and night vision. The results provide a novel pointcut for the design of NIR light-emitting materials with desirable luminescence properties.
1 Introduction
NIR luminescent materials have generated significant interest in the biomedical field, nondestructive food testing, night vision, and bioimaging technologies.1–12 Compared to traditional NIR light sources such as halogen lamps and tungsten–halogen lamps, NIR pc-LEDs exhibit prominent advantages because of their low cost, high efficiency, and portability, which can be employed in a smart device.5,13–16 The Cr3+ ions with a 3d3 structure are the main protagonists of current NIR phosphor materials. First of all, their unique absorption band can be well adapted to the current blue-light and red-light chips. More importantly, Cr3+ doped NIR phosphors tend to exhibit high IQE and a relatively broad spectrum. Therefore, they have been more widely researched compared with other lanthanides (Nd3+, Pr3+, Yb3+, etc.)17,18 and transition metal elements (Mn2+, Ni2+, etc.).19–21
Recently, crystal field engineering and co-doped rare-earth ions for energy transfer have been explored to optimize the luminescence performance of Cr3+-doped broadband NIR phosphors. In particular, modulating the crystal field environment by substitution of single cations or chemical units is an effective way to improve the luminescence performance, such as Sr(Al12−xGax)O19:Cr3+, Gd3(Sc2−xAlx)Ga3O12:Cr3+, Ca3Hf2(LuxAl2−x)SiO12:Cr3+, and (Li1−xIn2−xZn2x)SbO6:Cr3+.3,22–24 An enhancement of the luminescence properties can be achieved fundamentally by tuning the environment of the crystallographic site occupied by Cr3+ ions. However, currently, the research on the influence of the crystallographic sites occupied by Cr3+ ions on the luminescence properties (FWHM, thermal stability, and IQE) is still not comprehensive and remains a valuable and promising study.1,21–23,25–27 XTaO4 (X = In, Sc, and Ga) is a layered structure with [XO6] and [TaO6] layers arranged in phase, which provides a relatively sensitive crystallographic sites environment for Cr3+ ions and can be used as a good host to study the effect of crystallographic sites environment on the luminescence properties of NIR phosphors. Recently, Zhang et al. reported a series of ABO4:Cr3+ (A3+ = Ga, Sc, In, B5+ = Ta, Nb) NIR phosphors based on crystal site engineering and crystal field tuning, to achieve tunable photoluminescence.28 From a different perspective, when we investigated the luminescence properties of XTaO4:Cr3+ (X = In, Sc, and Ga) phosphors, we found a phenomenon well worth exploring: the thermal stability increased from 8% to 73% and IQE increased from 36% to 82.6% when X changed from In to Ga. Such a large difference in luminescence properties may be closely related to the different crystallographic site environments for Cr3+ in XTaO4:Cr3+. Therefore, a detailed analysis of the internal reasons for the dramatic changes in luminescence properties caused by Cr3+ ions occupying different crystallographic site environments has been implemented, which will be helpful in exploring some fascinating properties of the matrix of NIR materials with excellent luminescence properties. The results showed that when X = Ga, that is GaTaO4:Cr3+, exhibited superior luminescence properties (IQE = 82.6%, EQE = 43.5%, I423K = 73%) due to its higher Debye temperature and lower electron–phonon coupling effect. And it was demonstrated that excellent structural rigidity and lower electron–phonon coupling effects are beneficial to enhancing the IQE/EQE and thermal stability of NIR phosphors, respectively. Finally, the NIR pc-LEDs were fabricated by combining commercial blue LED chips emitting at 450 nm with the prepared XTaO4:Cr3+ (X = In, Sc, and Ga) phosphors and demonstrated their promising applications in nondestructive testing and night vision.
2 Experimental sections
2.1 Materials and synthesis
Despite the inherent shortcomings of the high-temperature solid-state method (SSD), this method has the advantages of low cost, large yield, and a simple preparation process, and is widely used in commercial production of phosphor materials. And the high-temperature environment provided helps to improve the doping activator ions distributed homogeneously in the host lattice. In general, the emission intensity for a phosphor by SSD is higher than that by other methods. Furthermore, the composition of the host material XTaO4 (X = Ga, Sc, and In) contains four kinds of cations, and the precursors cannot be selected easily for the sol–gel and precipitation processes, etc., and the SSD seems to be the best choice. Therefore, all investigated phosphors were prepared via the SSD. The starting reagents Sc2O3 (99.99%), Ga2O3 (99.99%), In2O3 (99.99%), Ta2O5 (99.95%), and Cr2O3 (99.95%) were used as received without further purification. Stoichiometric amounts of raw materials weighed according to the nominal composition of X1−xTaO4:xCr3+ (0 < x < 0.02) were homogeneously mixed in an agate mortar and ground for 20 min. The obtained mixtures were then transferred into aluminum oxide crucibles and then sintered at 1500 °C for 10 h in air. After cooling, the samples were ground into powders for further measurement.
2.2 Characterization
The X-ray diffraction (XRD) patterns were tested by a Bruker D8 Advance X-ray diffractometer at 40 kV and 40 mA with Cu-α (λ = 1.54056 Å) irradiation. Rietveld refinement of the measured XRD data was performed using GSAS software. X-Ray photoelectron spectra (XPS) were obtained using an X-ray photoelectron spectrometer, Thermosphere ESCALAB 250Xi. The morphological characteristics of the prepared phosphors were recorded by field emission scanning electron microscopy (SEM, Nova Nano 450). The elemental composition and distribution were determined using an energy dispersive X-ray spectroscope attached to a SEM. The photoluminescence excitation (PLE) and photoluminescence emission (PL) spectra were recorded using a HORIBA FLuorolog3 fluorescence spectrometer with a 450 W Xe lamp as the excitation source low-temperature PL spectra were tested with an external device. The diffuse reflectance spectra (DRs) were measured on a Hitachi U4100, using BaSO4 as a standard reference. The spectra TL were tested by an FJ-427A1 heat release meter to test the samples for heat release spectroscopy with a temperature rise rate of 1 K s−1 and a test range of 300–600 K.
2.3 Performance of LEDs
The NIR LED device is made of a synthetic NIR phosphor and a blue light chip with a power of 1 W and emission at 450 nm. The mass ratio of AB glue to NIR phosphor was set to 1
:
1 and mixed uniformly. The taken night vision pictures were obtained with the help of a NIR camera.
2.4 Density functional theory calculations (DFT)
DFT calculations were performed by Vienna Ab Initio Simulation Package (VASP). The projector augmented wave potential was implemented to describe the core electrons. The valence electrons were described by plane waves with a cutoff kinetic energy of 500 eV. The Brillouin zone was sampled in the Monkhorst–Pack scheme with a K-point The convergence criteria of energy and forces were set as 10–5 eV and 0.05 eV Å−1, respectively. The electronica change-correlation energy was described by the generalized-gradient approximation of the Perdew–Burke–Ernzerhof (GGA-PBE) form.
3 Results and discussion
3.1 Structure and phase identification
Compounds with the general chemical formula XTaO4 (X = In, Sc, and Ga) have been reported to adopt the monoclinic-type (P2/a). As displayed in Fig. 1a, the 2 × 2 × 2 supercell of the XTaO4 crystal structure is observed. The independent distribution of [XO6] and [TaO6] layers make these two octahedral environments very sensitive, and changes in their average bond length or cell volume can directly affect the rigidity of the structure. The phase purity of XTaO4:Cr3+ (X = In, Sc, and Ga) was checked by XRD (Fig. 1b). All the diffraction peaks can be well-matched with the standard card (GaTaO4: ICSD-#8128, ScTaO4: ICSD-#109192, GaTaO4: ICSD-#72569), indicating the successful synthesis of high-purity target phosphors. The XPS of XTaO4 (X = In, Sc, and Ga) are presented in Fig. 1c. Binding energies corresponding to Ga-3s, Sc-2p, In-3d, Ta-4d, Cr-2p3/2, and O-1s were detected in the XTaO4 (X = In, Sc, and Ga), and the binding energy peak of Cr-2p was weak due to the very low doping of Cr3+ relative to the host (the binding energy peak of 576.4 eV is magnified in the inset and corresponds to 2p3/2 of Cr3+). To further determine the crystal structure, the Rietveld refinement of the XRD data for the undoped and Cr3+-doped XTaO4 (X = In, Sc, and Ga) sample is performed (Fig. 1d and e, detailed refinement data is placed in Tables S1 and S3, ESI†). The lattice parameters (a, b, and c) and volume (V) of the unit cell decrease with increasing Cr3+ concentration, while the refinement parameters remain essentially constant (Fig. S1, ESI†), which could prove that Cr3+ was effectively doped. The SEM images of XTaO4 (X = In, Sc, and Ga) reveal that the size and morphology of the three sample particles are essentially uniforms (Fig. 1f). The results of elemental mapping of XTaO4:Cr3+ (X = In, Sc, and Ga) show that the elements Ga/Sc/In, Ta, Cr, and O are uniformly presented in the phosphor particles (Fig. 1g and Fig. S2 and S3, ESI†). The EDS results for XTaO4:Cr3+ (X = In, Sc, and Ga) demonstrate the same elemental proportions as the molecular formula (Tables S4–S6, ESI†).
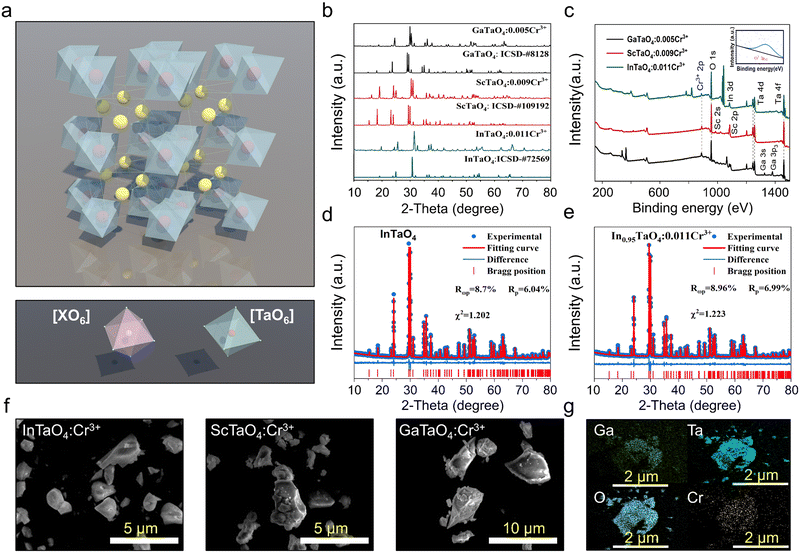 |
| Fig. 1 (a) Crystal structure and the coordination environments of XTaO4 (X = In, Sc, and Ga); (b) XRD patterns of XTaO4 (X = In, Sc, and Ga); (c) XPS results of XTaO4 (X = In, Sc, and Ga). The inset shows the binding energy peak of Cr3+–2p3/2; (d and e) Rietveld refinement of XRD results of InTaO4 and In0.989TaO4 : 0.011Cr3+; (f) SEM of XTaO4:Cr3+ (X = In, Sc, and Ga); (g) mapping of GaTaO4:Cr3+. | |
3.2 Luminescence properties of XTaO4:Cr3+ (X = In, Sc, Ga)
The PLE spectra of XTaO4:Cr3+ (X = In, Sc, and Ga) all have similar three excitation bands corresponding to the spin-allowed transitions of Cr3+ (4A2 → 4T1 (4P), 4A2 → 4T1 (4F), and 4A2 → 4T2 (4F) transition). The three absorption bands of the diffuse reflectance spectra (DRs) of XTaO4:Cr3+ (X = In, Sc, and Ga) can correspond well to the excitation spectra and have strong absorption bands in the range of 400–550 nm and 600–750 nm, which can match well with the emission bands of the blue and red chips (Fig. S4, ESI†). The XTaO4:Cr3+ (X = In, Sc, and Ga) phosphors exhibit broadband NIR luminescence, which can be attributed to the spin-allowed 4T2(4F) → 4A2 transition of the octahedra occupied by the Cr3+ ion (Fig. 2a). For the lifetime tests of GaTaO4:Cr3+ at different wavelengths at a low temperature of 4 K, there are only weak differences and it is difficult to distinguish multiple luminescence centers, so we tend to think that this is a single luminescence center formed by the Cr3+ ion occupying the [XO6] octahedron (Fig. 2b and c). Furthermore, if the Cr3+ ion replaces the Ta5+ ion, it will lead to the substitution of charge imbalance and may form defects, but no defects were found by testing the thermoluminescence spectra (Fig. S5, ESI†). The use of DFT calculations in the GaTaO4:Cr3+ system, as reported by Zhao et al. suggests that Cr3+ ions prefer to replace Ga3+ ions for luminescence.29 To better illustrate this point, we added the relevant DFT calculation that the relative formation energy of the GaTaO4 host is much smaller than that of the Ta5+ ion after its substitution by the Cr3+ ion (Table S7, ESI†). This result suggests that Cr3+ is very much less inclined to occupy the Ta5+ ion. Meanwhile, the XRD of X3+ ions and Ta5+ ions substituted by Cr3+ ions were. The refinement parameters are smaller when X3+ ions are substituted by Cr3+ ions, which can be a good indication that Cr3+ ions prefer to substitute X3+ ions rather than Ta5+ ions (Fig. S6, ESI†). Having such a large difference in the luminescence properties is necessarily related to the sensitive [XO6] octahedral environment occupied by Cr3+ ions. As the average X3+–O2− distance d(x–o), octahedral volume V[XO6] and luminescence peak positions in XTaO4 (X = In, Sc, and Ga) generate a large variation ratio with the change of X3+ ion radius r(x) (Table 1). In contrast to [MO6] in Sr9M(PO4)7 (M = Ga, Sc, In, and Lu) (where the X3+–O2− average distance d(x–o), octahedral volume V[XO6], and luminescence peak position remain essentially constant as the M3+ ion radius changes),25 [XO6] in XTaO4 (X = In, Sc, and Ga) is a sensitive octahedron.
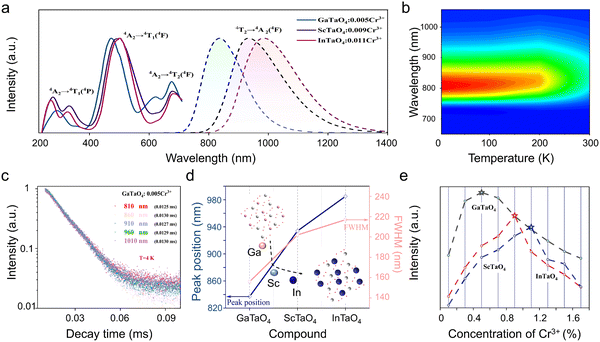 |
| Fig. 2 (a) PLE and PL spectrum of XTaO4:Cr3+ (X = In, Sc, and Ga); (b) the low-temperature emission spectra of GaTaO4:Cr3+; (c) decay curves of GaTaO4:Cr3+ were monitored at 810 nm, 860 nm, 910 nm, 960 nm, and 1010 nm; (d) variation trend of the PL intensity of XTaO4:Cr3+(X = In, Sc, and Ga) with Cr3+-ion concentration; (e) the peaking position and FWHM of XTaO4:Cr3+ (X = In, Sc, and Ga). | |
Table 1 The ionic radii of X3+ ions, average X3+-O2− distances, octahedral volumes V[XO6], PL peak positions, and variation ratio for XTaO4:Cr3+ (X = Ga, Sc, and In)
Phosphor |
r
(X) (nm) |
d
(X–O) (Å) |
V
[XO6] (Å) |
Peak (nm) |
GaTaO4 |
0.062 |
2.017 |
10.724 |
837 |
ScTaO4 |
0.075 |
2.133 |
12.527 |
934 |
InTaO4 |
0.08 |
2.151 |
12.861 |
985 |
Variation ratio (%) |
29.03 |
6.64 |
19.93 |
17.7 |
The lattice vibrational energy in the crystal is considered to be closely related to its structural rigidity, and to further illustrate the variation of structural rigidity; it can be evaluated theoretically by calculating the Debye temperature of XTaO4 (X = In, Sc and Ga) using DFT with calculated values of 420.1 K, 430.801 K, and 488.329 K.6,30–32 As the structural rigidity of the crystallographic lattice environment occupied by Cr3+ ions changes, it makes XTaO4 (X = In, Sc and Ga) show great differences in its luminescence properties.
In NIR phosphors with Cr3+-doped octahedra, the emission wavelength depends mainly on the crystal field intensity of the phosphor. When the X3+ ions are Ga3+, Sc3+, and In3+ in that order, respectively, the crystal field intensity decreases from 2.29 to 2.03 as calculated from the crystal field equation, which indicates that XTaO4:Cr3+ (X = Ga, Sc, and In) is located in the weak crystal field. When the Dq/B value decreases, it leads to a gradual decrease in the energy level difference between the 4T2 and 4A2 levels, (Fig. S7, ESI†), which is the main reason for the redshift of the peak position from 837 nm to 985 nm.27 The FWHM broadens from Ga3+ (156 nm) to In3+ (217 nm), which is determined by the electron–phonon coupling effect (Fig. 2d).27,33 When the X3+ ions are Ga3+, Sc3+, and In3+ in order, the X3+–O2− bond stretching increases, the [XO6] octahedral lattice expands, and the gradually expanding coordination environment leads to a larger Stokes shift, because the Stokes shift is strongly influenced by the rigidity of the crystal structure. Therefore, the non-radiative transitions generated when the X3+ ion is Ga3+ will be weaker than that for Sc3+ and In3+, and the emission intensity of GaTaO4:Cr3+ will be stronger (Fig. 2e and Fig. S8, ESI†).26,33,34
Meanwhile, the IQE of the phosphor is an important parameter to evaluate whether it can be used as an efficient phosphor for pc-LED. The IQE values of XTaO4:Cr3+ (X = In, Sc, and Ga) were 36%, 54%, and 82.6%, respectively (Fig. S9, ESI†), where GaTaO4:Cr3+ was superior to most of the previously reported Cr3+-doped NIR phosphors (Table 2). Excellent structural rigidity has been reported to be effective in enhancing photon emission and enhancing IQE.35,36 Since [XO6] is a sensitive octahedral structure, the change of X3+ ions will have a great impact on the structural rigidity. When the X3+ ion changes from the Ga3+ ion to the In3+ ion, the structure will become loose, allowing the soft phonon mode of nonradiative relaxation to be enhanced, thus increasing the tendency for photon emission and causing a substantial decrease in IQE.36
3.3 Temperature-dependent spectra analysis
To analyze the thermal stability of XTaO4 (X = In, Sc, and Ga), PL spectra in the temperature range of 273–473 K were tested (Fig. 3a and Fig. S10, ESI†). The temperature-dependent normalized spectrum of GaTaO4:Cr3+ is redshifted by about 19 nm and the FWHM broadens from 155 nm to 174 nm. The redshift at the peak position comes from the temperature-induced weakening of the crystal field environment, while the broadening of the emission peak is due to the enhancement of the electron–phonon coupling effect (Fig. 3b). Here the enhancement of the electron–phonon coupling effect is reflected by the Huang–Rhys factor (S), the resulting S and ℏω of XTaO4:Cr3+ (X = Ga, Sc, and In) are calculated to be 1.4 and 50.45 meV, 3.11 and 33 meV, 5.26 and 12.45 meV (the detailed calculation procedure is placed in the ESI† ‘Measurements of Huang–Rhys factor’ and Fig. S11, ESI†). In general, the Stokes displacement and FWHM increase as the S value increases, which will lead to weaker thermal stability. The integrated emission intensity of XTaO4:Cr3+ (X = In, Sc, and Ga) at 423 K remains around 8%, 12%, and 73% of the room temperature value, respectively (Fig. 3c). Compared with the other reported broadband NIR phosphors listed in Table 2, the thermal stability of GaTaO4:Cr3+ is competitive.
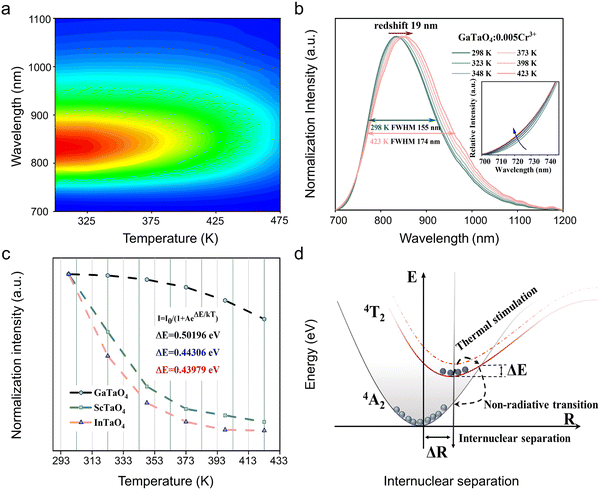 |
| Fig. 3 (a) Temperature-dependent PL spectra of samples GaTaO4 : 0.005Cr3+; (b) temperature-dependent PL spectra of the normalized intensities for the GaTaO4 : 0.005Cr3+ sample, and the inset represents the emission spectra wavelength from 700 nm to 750 nm; (c) The integrated intensities of temperature-dependent NIR emission of XTaO4:Cr3+ (X = In, Sc, and Ga); the values are the fitting results for the Arrhenius equation; (d) configuration coordinate diagram of Cr3+-ions in the XTaO4:Cr3+ (X = In, Sc, and Ga) host. | |
Table 2 Summary of some of the reported luminescent properties of NIR phosphors
Phosphor |
λ
em (nm) |
FWHM (nm) |
IQE/EQE (%) |
I
423K (%) |
Ref. |
La2MgZrO6:Cr3+ |
825 |
210 |
58/– |
40 |
14
|
Ca2LuZr2Al3O12:Cr3+ |
752 |
117 |
69/– |
60 |
37
|
La3Sc2Ga3O12:Cr3+ |
818 |
145 |
35/– |
60 |
33
|
ScBO3:Cr3+ |
800 |
120 |
65/– |
51 |
38
|
Na3In2Li3F12:Cr3+ |
778 |
121 |
87.2/20.05 |
58 |
18
|
ScF3:Cr3+ |
853 |
140 |
45/– |
85.5 |
4
|
MgAl2O4:Cr3+ |
740 |
140 |
15/– |
59 |
5
|
LiScP2O7:Cr3+ |
880 |
170 |
53/– |
18 |
39
|
GaTaO4:Cr3+ |
850 |
266 |
82.6/43.5 |
73 |
This work |
To better understand the luminescence thermal stability, the activation energy (Ea) can be calculated by the Arrhenius equation:19,33,37
| 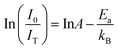 | (1) |
where
I0 is the initial emission intensity at 273 K,
IT is the emission intensity at a given temperature T,
A is constant, and
kB is the Boltzmann constant (8.617 × 10
−5 eV K
−1). The energy difference between the lowest excited state and intersection point is defined as the thermal activation energy
Ea, which can be estimated to be 0.440 eV, 0.443 eV, and 0.502 eV for the composition of XTaO
4:Cr
3+ (X = In, Sc, and Ga), respectively. The thermal quenching process can be described in
Fig. 3dvia a configurational coordinate diagram. When the activation energy Δ
E increases, it is more difficult for the activated electrons to overcome the energy barrier Δ
E, so the nonradiative transition decreases, leading to an increase in thermal stability. Such a huge difference in thermal stability performance is triggered by two main points: first, the X
3+ ions in the sequence of Ga
3+, Sc
3+, and In
3+ provide a gradually relaxing luminescent environment for the Cr
3+ ions, leading to an enhanced electron–phonon coupling effect, which makes the cross-relaxation between the phonon-assisted excited and ground states easier. Second, the doping of Cr
3+ ions in the sensitive [XO
6] octahedral lattice sites, with the X
3+ ions being Ga
3+, Sc
3+, and In
3+ in sequence, the progressively relaxed environment of the octahedra leads to an increase in the Stokes shift as well as a decrease in the activation energy Δ
E, allowing the probability of the nonradiative transition to be increased, resulting in a lower thermal quenching resistance.
3.4 NIR pc-LED application
Given the excellent luminescence properties of XTaO4:Cr3+ (X = In, Sc, Ga) phosphors, NIR pc-LED were prepared by combining commercial InGaN chips. The NIR output power of XTaO4:Cr3+ (X = In, Sc, and Ga) was 282 mW, 283 mW, and 287 mW, respectively, while the efficiency was 0.7%, 1.9%, and 11.5%, respectively, when the driving current was 100 mA (Fig. 4a). Among them, GaTaO4:Cr3+ has better luminescence properties, and its photoelectric conversion efficiency decreases from 11.5% to 3.9% (as the current thermal effect, with the increase of current, causes a certain amount of energy to be dissipated as a thermal energy situation, resulting in the decrease of photoelectric conversion efficiency) and output power increases from 287 mW to 4090 mW when the driving current is from 100 mA to 1000 mA (Fig. 4b). It is worth noting that the actual output power should be higher since the detector can only effectively capture the energy that is certain to pass under the NIR emission range (650–1000 nm). In addition, the operating temperature of the high-power pc-LED increases from about 300 K to about 420 K when the drive current increases from 0 mA to 800 mA (Fig. 4c), demonstrating that the fabricated XTaO4:Cr3+ (X = In, Sc, and Ga) NIR pc-LEDs still exhibit good luminescence performance in practical applications.
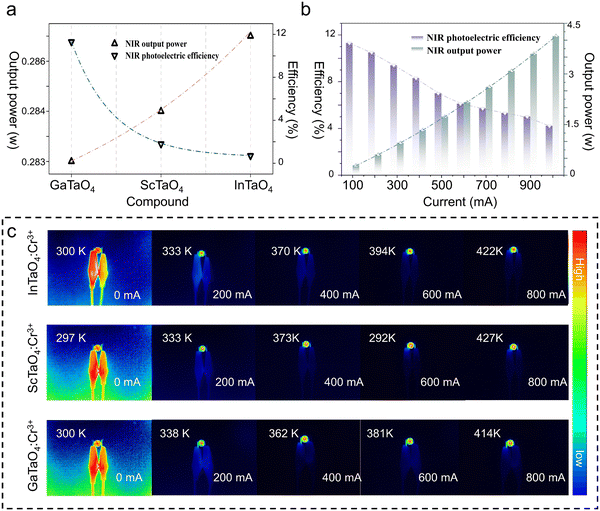 |
| Fig. 4 (a) Output optical powers, and conversion efficiencies of the NIR-LED of XTaO4:Cr3+ (X = In, Sc, and Ga) depending on the 100 mA driving current; (b) output optical powers, and conversion efficiencies of the NIR-LED of GaTaO4:Cr3+ depending on the driving current from 100 mA to 1000 mA; (c) thermal images of the pc-LED of XTaO4:Cr3+ (X = In, Sc, and Ga) at different operating currents over 0–800 mA. | |
The application of the NIR pc-LED of GaTaO4:Cr3+ in night vision is demonstrated in Fig. 5a. For NIR spectroscopy applications, the ideal light source should have a broad spectrum to cover more information about the functional groups. Herein, the spectral luminescence spectra of XTaO4:Cr3+ (X = In, Sc, and Ga) in the bands 700–1200, 800–1300, 850–1400 nm respectively can be combined to detect more information in the ultra-wide range. The PL spectra of five solutions (water, glucose, tea, milk, and coffee) after penetrating them with three LEDs respectively are shown in Fig. S12 (ESI†). Using the spectrum without penetrating any substance as the normal line, the difference spectrum can distinguish the five solutions more clearly (Fig. 5b). It was found that the solution has different absorption in the 700–1400 nm range and the light sources with different peak wavelengths show different detection sensitivity to the absorption signal, which is very obvious in the case of milk. The combination of the three LEDs gives more complete information on the absorption of each sample, which can improve the accuracy of monitoring, demonstrating their potential for non-destructive testing applications. Finally, the ability to compare images of eggs, access cards, and palms took in both natural and NIR light demonstrates the viability of nondestructive inspection using GaTaO4:Cr3+ manufactured NIR pc-LED in a variety of working circumstances (Fig. 5c). In summary, NIR pc LED-based non-destructive testing may become an effective and convenient alternative to traditional radiography.
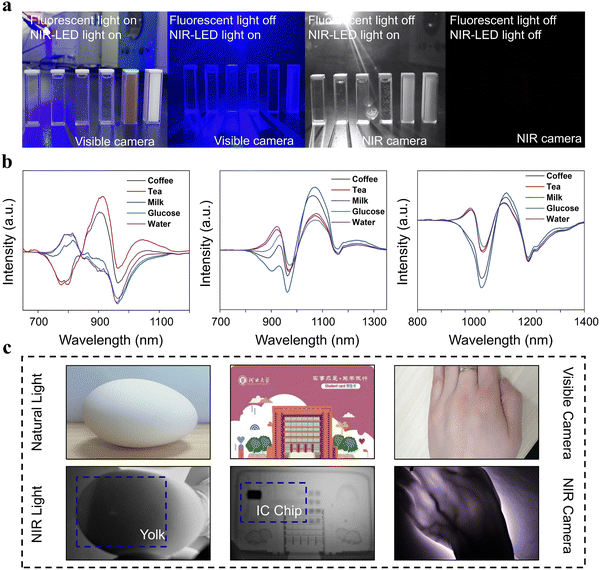 |
| Fig. 5 A multitude of applications of XTaO4:Cr3+ (X = In, Sc, and Ga) are demonstrated; (a) night vision (visual and NIR images of water, glucose, tea, milk, and coffee); (b) nondestructive food detection (NIR spectra after penetrating water, glucose, tea, milk, and coffee); (c) NIR non-destructive testing applications (photos of eggs, access cards and palms in natural and NIR light). | |
4 Conclusions
In summary, it was demonstrated via Debye temperature, electron–phonon coupling effects, and crystal field theory that the structural rigidity of XTaO4:Cr3+ (X = In, Sc, and Ga) becomes progressively stronger when the X3+ ions are In3+, Sc3+ and Ga3+ ions in sequence, leading to a significant improvement in the luminescence properties (thermal stability: In3+ (8%) → Ga3+ (73%), IQE: In3+ ((36%) → Ga3+ (82.6%)). The rigid structure will effectively enhance the luminescence properties of Cr3+ ions, and the detailed mechanism explanation provides an effective idea to continue the exploration of novel stable and efficient NIR phosphors in the future. Due to its excellent rigidity, GaTaO4:Cr3+ has excellent IQE/EQE (82.6%/43.5%) and good thermal stability (I423K = 73%), which meets the current requirements for high-performance NIR phosphors. Ultimately, a series of XTaO4:Cr3+ (X = In, Sc, and Ga) NIR pc-LED devices were fabricated, proving their promising applications in night vision, food composition analysis, and non-destructive testing.
Conflicts of interest
The authors declare no conflict of interest.
Acknowledgements
The work is supported by the National Natural Science Foundation of China (No. 51902080), the Natural Science Foundation of Hebei Province, China (No. E2019201223), the personnel training project of Hebei Province, China (No. A201902005), and the central government to guide local scientific and Technological Development (No. 206Z1102G and 216Z1101G).
References
- Z. Wu, X. Han, Y. Zhou, K. Xing, S. Cao, L. Chen, R. Zeng, J. Zhao and B. Zou, Efficient broadband near-infrared luminescence of Cr3+ doped fluoride K2NaInF6 and its NIR-LED application toward veins imaging, Chem. Eng. J., 2022, 427, 131740 CrossRef CAS
.
- H. Yu, J. Chen, R. Mi, J. Yang and Yg Liu, Broadband near-infrared emission of K3ScF6:Cr3+ phosphors for night vision imaging system sources, Chem. Eng. J., 2021, 417, 129271 CrossRef CAS
.
- Y. Wei, P. Dang, Z. Dai, G. Li and J. Lin, Advances in Near-Infrared Luminescent Materials without Cr3+: Crystal Structure Design, Luminescence Properties, and Applications, Chem. Mater., 2021, 33(14), 5496–5526 CrossRef CAS
.
- Q. Lin, Q. Wang, M. Liao, M. Xiong, X. Feng, X. Zhang, H. Dong, D. Zhu, F. Wu and Z. Mu, Trivalent Chromium Ions Doped Fluorides with Both Broad Emission Bandwidth and Excellent Luminescence Thermal Stability, ACS Appl. Mater. Interfaces, 2021, 13(15), 18274–18282 CrossRef CAS
.
- J. Qiao, G. Zhou, Y. Zhou, Q. Zhang and Z. Xia, Divalent europium-doped near-infrared-emitting phosphor for light-emitting diodes, Nat. Commun., 2019, 10(1), 5267 CrossRef PubMed
.
- C. Yuan, R. Li, Y. Liu, L. Zhang, J. Zhang, G. Leniec, P. Sun, Z. Liu, Z. Luo, R. Dong and J. Jiang, Efficient and Broadband LiGaP2O7:Cr3+ Phosphors for Smart Near-Infrared Light-Emitting Diodes, Laser Photonics Rev., 2021, 2100227 CrossRef CAS
.
- F. He, E. Song, Y. Zhou, H. Ming, Z. Chen, J. Wu, P. Shao, X. Yang, Z. Xia and Q. Zhang, A General Ammonium Salt Assisted Synthesis Strategy for Cr3+-Doped Hexafluorides with Highly Efficient Near-Infrared Emissions, Adv. Funct. Mater., 2021, 31(36), 2103743 CrossRef CAS
.
- D. Liu, G. Li, P. Dang, Q. Zhang, Y. Wei, H. Lian, M. Shang, C. C. Lin and J. Lin, Simultaneous Broadening and Enhancement of Cr(3+) Photoluminescence in LiIn2SbO6 by Chemical Unit Co substitution: Night-Vision and Near-Infrared Spectroscopy Detection Applications, Angew. Chem., Int. Ed., 2021, 60(26), 14644–14649 CrossRef CAS PubMed
.
- C. Dincer, R. Bruch, E. Costa-Rama, M. T. Fernandez-Abedul, A. Merkoci, A. Manz, G. A. Urban and F. Guder, Disposable Sensors in Diagnostics, Food, and Environmental Monitoring, Adv. Mater., 2019, 31(30), e1806739 CrossRef PubMed
.
- Y. J. Liang, F. Liu, Y. F. Chen, X. J. Wang, K. N. Sun and Z. Pan, New function of the Yb(3+) ion as an efficient emitter of persistent luminescence in the short-wave infrared, Light: Sci. Appl., 2016, 5(7), e16124 CrossRef CAS PubMed
.
- K. Tuong Ly, R.-W. Chen-Cheng, H.-W. Lin, Y.-J. Shiau, S.-H. Liu, P.-T. Chou, C.-S. Tsao, Y.-C. Huang and Y. Chi, Near-infrared organic light-emitting diodes with very high external quantum efficiency and radiance, Nat. Photonics, 2016, 11(1), 63–68 CrossRef
.
- Y. Gu, Z. Guo, W. Yuan, M. Kong, Y. Liu, Y. Liu, Y. Gao, W. Feng, F. Wang, J. Zhou, D. Jin and F. Li, High-sensitivity imaging of time-domain near-infrared light transducer, Nat. Photonics, 2019, 13(8), 525–531 CrossRef CAS
.
- W. T. Huang, C. L. Cheng, Z. Bao, C. W. Yang, K. M. Lu, C. Y. Kang, C. M. Lin and R. S. Liu, Broadband Cr(3+), Sn(4+)-Doped Oxide Nanophosphors for Infrared Mini Light-Emitting Diodes, Angew. Chem., Int. Ed., 2019, 58(7), 2069–2072 CrossRef CAS PubMed
.
- H. Zeng, T. Zhou, L. Wang and R.-J. Xie, Two-Site Occupation for Exploring Ultra-Broadband Near-Infrared Phosphor-Double-Perovskite La2MgZrO6:Cr3+, Chem. Mater., 2019, 31(14), 5245–5253 CrossRef CAS
.
- P. Xiong, M. Peng, K. Qin, F. Xu and X. Xu, Visible to Near-Infrared Persistent Luminescence and Mechanoluminescence from Pr3+-Doped LiGa5O8 for Energy Storage and Bioimaging, Adv. Opt. Mater., 2019, 7(24), 1901107 CrossRef CAS
.
- J. Cao, J. Peng, L. Wang, H. Luo, X. Wang, P. Xiong, Y. Wang and M. Peng, Broadband NIR emission from multiple Bi centers in nitridated borogermanate glasses via tailoring local glass structure, J. Mater. Chem. C, 2019, 7(7), 2076–2084 RSC
.
- X. Zhou, W. Geng, J. Li, Y. Wang, J. Ding and Y. Wang, An Ultraviolet-Visible and Near-Infrared-Responded Broadband NIR Phosphor and Its NIR Spectroscopy Application, Adv. Opt. Mater., 2020, 8(8), 1902003 CrossRef CAS
.
- S. He, L. Zhang, H. Wu, H. Wu, G. Pan, Z. Hao, X. Zhang, L. Zhang, H. Zhang and J. Zhang, Efficient Super Broadband NIR Ca2LuZr2Al3O12:Cr3+, Yb3+ Garnet Phosphor for pc-LED Light Source toward NIR Spectroscopy Applications, Adv. Opt. Mater., 2020, 8(6), 1901684 CrossRef CAS
.
- M. Northey and J. Mckibbin, Optical Spectroscopy of Inorganic Solids, Monogr. Phys. Chem. Mater., 2006, 45–59 Search PubMed
.
- T. Maldiney, A. Bessière, J. Seguin, E. Teston, S. K. Sharma, B. Viana, A. J. J. Bos, P. Dorenbos, M. Besides, D. Gourier, D. Scherman and C. Richard, The in vivo activation of persistent nanophosphors for optical imaging of vascularization, tumors, and grafted cells, Nat. Mater., 2014, 13(4), 418–426 CrossRef CAS PubMed
.
- Z. Pan, Y. Y. Lu and F. Liu, Sunlight-activated long-persistent luminescence in the near-infrared from Cr(3+)-doped zinc gallogermanates, Nat. Mater., 2011, 11(1), 58–63 CrossRef PubMed
.
- V. Rajendran, M. H. Fang, W. T. Huang, N. Majewska, T. Lesniewski, S. Mahlik, G. Lennie, S. M. Kaczmarek, W. K. Pang, V. K. Peterson, K. M. Lu, H. Chang and R. S. Liu, Chromium Ion Pair Luminescence: A Strategy in Broadband Near-Infrared Light-Emitting Diode Design, J. Am. Chem. Soc., 2021, 143(45), 19058–19066 CrossRef CAS PubMed
.
- E. T. Basore, W. Xiao, X. Liu, J. Wu and J. Qiu, Broadband Near-Infrared Garnet Phosphors with Near-Unity Internal Quantum Efficiency, Adv. Opt. Mater., 2020, 8(12), 2000296 CrossRef CAS
.
- L. Zhang, D. Wang, Z. Hao, X. Zhang, G. H. Pan, H. Wu and J. Zhang, Cr3+-Doped Broadband NIR Garnet Phosphor with Enhanced Luminescence and its Application in NIR Spectroscopy, Adv. Opt. Mater., 2019, 7(12), 1900185 CrossRef
.
- F. Zhao, H. Cai, Z. Song and Q. Liu, Structural Confinement for Cr3+ Activators toward Efficient Near-Infrared Phosphors with Suppressed Concentration Quenching, Chem. Mater., 2021, 33(10), 3621–3630 CrossRef CAS
.
- F. Y. Zhao, H. Cai, S. Y. Zhang, Z. Song and Q. L. Liu, Octahedron-dependent near-infrared luminescence in Cr3+-activated phosphors, Mater. Today Chem., 2022, 23, 100704 CrossRef CAS
.
- W. Nie, Y. Li, J. Zuo, Y. Kong, W. Zou, G. Chen, J. Peng, F. Du, L. Han and X. Ye, Cr3+-activated Na3X2Li3F12 (X = Al, Ga, or In) garnet phosphors with broadband NIR emission and high luminescence efficiency for potential biomedical application, J. Mater. Chem. C, 2021, 9(42), 15230–15241 RSC
.
- Q. Zhang, D. Liu, P. Dang, H. Lian, G. Li and J. Lin, Two Selective Sites Control of Cr3+-Doped ABO4 Phosphors for Tuning Ultra-Broadband Near-Infrared Photoluminescence and Multi-Applications, Laser Photonics Rev., 2021, 16(2), 2100459 CrossRef
.
- J. Zhong, Y. Zhuo, F. Du, H. Zhang, W. Zhao, S. You and J. Brgoch, Efficient Broadband Near-Infrared Emission in the GaTaO4:Cr3+ Phosphor, Adv. Opt. Mater., 2021, 10(2), 2101800 CrossRef
.
- X. Xu, Q. Shao, L. Yao, Y. Dong and J. Jiang, Highly efficient and thermally stable Cr3+-activated silicate phosphors for broadband near-infrared LED applications, Chem. Eng. J., 2020, 383, 123108 CrossRef CAS
.
- M.-H. Fang, G. N. A. De Guzman, Z. Bao, N. Majewska, S. Mahlik, M. Grinberg, G. Leniec, S. M. Kaczmarek, C.-W. Yang, K.-M. Lu, H.-S. Sheu, S.-F. Hu and R.-S. Liu, Ultra-high-efficiency near-infrared Ga2O3:Cr3+ phosphor and controlling of phytochrome, J. Mater. Chem. C, 2020, 8(32), 11013–11017 RSC
.
- L. You, R. Tian, T. Zhou and R.-J. Xie, Broadband near-infrared phosphor BaMgAl10O17:Cr3+ realized by crystallographic site engineering, Chem. Eng. J., 2021, 417, 129224 CrossRef CAS
.
- B. Malysa, A. Meijerink and T. Jüstel, Temperature-dependent Cr3+ photoluminescence in garnets of the type X3Sc2Ga3O12 (X = Lu, Y, Gd, La), J. Lumin., 2018, 202, 523–531 CrossRef CAS
.
- L. He, Z. Song, X. Jia, Z. Xia and Q. Liu, Consequence of Optimal Bonding on Disordered Structure and Improved Luminescence Properties in T-Phase (Ba, Ca)2SiO4:Eu(2+) Phosphor, Inorg. Chem., 2018, 57(7), 4146–4154 CrossRef CAS PubMed
.
- J. Brgoch, S. P. DenBaars and R. Seshadri, Proxies from Ab Initio Calculations for Screening Efficient Ce3+ Phosphor Hosts, J. Phys. Chem. C, 2013, 117(35), 17955–17959 CrossRef CAS
.
- Y. Zhuo, A. Mansouri Tehrani, A. O. Oliynyk, A. C. Duke and J. Brgoch, Identifying an efficient, thermally robust inorganic phosphor host via machine learning, Nat. Commun., 2018, 9(1), 4377 CrossRef PubMed
.
- L. Zhang, S. Zhang, Z. Hao, X. Zhang, G.-H. Pan, Y. Luo, H. Wu and J. Zhang, A high-efficiency broadband near-infrared Ca2LuZr2Al3O12:Cr3+ garnet phosphor for blue LED chips, J. Mater. Chem. C, 2018, 6(18), 4967–4976 RSC
.
- M.-H. Fang, P.-Y. Huang, Z. Bao, N. Majewska, T. Leśniewski, S. Mahlik, M. Grinberg, G. Leniec, S. M. Kaczmarek, C.-W. Yang, K.-M. Lu, H.-S. Sheu and R.-S. Liu, Penetrating Biological Tissue Using Light-Emitting Diodes with a Highly Efficient Near-Infrared ScBO3:Cr3+ Phosphor, Chem. Mater., 2020, 32(5), 2166–2171 CrossRef CAS
.
- L. Yao, Q. Shao, S. Han, C. Liang, J. He and J. Jiang, Enhancing Near-Infrared Photoluminescence Intensity and Spectral Properties in Yb3+ Codoped LiScP2O7:Cr3+, Chem. Mater., 2020, 32(6), 2430–2439 CrossRef CAS
.
|
This journal is © the Partner Organisations 2022 |