DOI:
10.1039/D0QI00511H
(Highlight)
Inorg. Chem. Front., 2020,
7, 2556-2559
Journey to the Holy Grail of a coordination saturated buckyball
Received
7th May 2020
, Accepted 3rd June 2020
First published on 3rd June 2020
Abstract
Exohedral metallofullerenes are increasingly gaining attention from both academia and industry due to their unique structural and electronic properties. C60 provides an incredible opportunity to bind up to 30 metals on the surface to realize an icosidodecahedral C60@M30 supramolecular complex, the Holy Grail of a coordination saturated buckyball. Recently, the Li and Zhan groups reported a rhombicuboctahedral C60@Cu24 core–shell structure, a giant leap on their journey toward the Holy Grail.
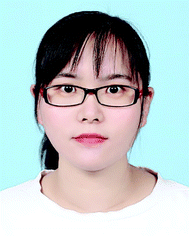 Dandan Chu | Dandan Chu was born in Anhui province, China. She received her BS degree from Anhui Normal University in 2014. Then, she joined the research group of Prof. Yong Cui at Shanghai Jiao Tong University. Her research interest focuses on chiral metal–organic cages. |
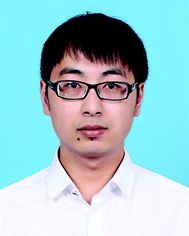 Yuhao Liu | Yuhao Liu obtained his MS degree from Fuzhou University in 2016, where he worked on the synthesis of 2D nanosheets under the supervision of Dr Ling Wu. Then, he joined the research group of Prof. Yong Cui at Shanghai Jiao Tong University. His research interest is focused on the synthesis, properties, and applications of supramolecular materials. |
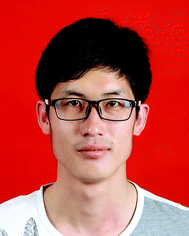 Yingguo Li | Yingguo Li was born in Henan province, China. He received his BS degree from Xuchang University in 2013 and MS degree in 2016 at Lanzhou University. Then, he joined the research group of Prof. Yong Cui group at Shanghai Jiao Tong University. His research interest focuses on metal–organic cages for catalysis and separation. |
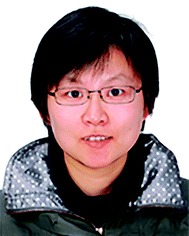 Yan Liu | Yan Liu received her BS degree in applied chemistry in 2006 and PhD in chemistry in 2011 from Shanghai Jiao Tong University. She conducted her postdoctoral research at the University of North Carolina (2011–2013). She joined Shanghai Jiao Tong University in 2014, where she is now a professor of chemistry. Her research interest focuses on supramolecular chemistry and coordination chemistry. |
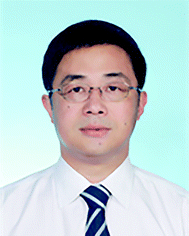 Yong Cui | Yong Cui received his PhD in physical chemistry in 1999 from Fujian Institute of Research on the Structure of Matter, CAS. He conducted his postdoctoral research at the University of Science and Technology of China, the University of North Carolina and the University of Chicago from 1999 to 2005. He joined Shanghai Jiao Tong University in 2005, where he is now a chair professor of chemistry. His research interest focuses on the fields of molecular assembly, porous materials and chiral materials. |
Since the discovery in 1985,1 fullerene-C60 (C60, buckyball) and its derivatives have always been a research hotspot because of their unique structures and physicochemical properties.2–17 One particular direction is to exploit the exohedral metallofullerenes,7–9,18,19 coordination complexes of transition metals (M) spreading over the surface of C60 binding to its C
C bonds in η2 fashion, resulting in various C60 derivatives with intriguing photofunctional/electronic properties.4 As a spherical polyene system, the 30 C
C bonds of C60 provide an incredible opportunity to bind up to 30 metal atoms in a μ30-(η2)30 fashion and realize the C60@M30 core–shell structure, an icosidodecahedral supramolecular complex featuring 20 vertex-shared M3-trigons, the Holy Grail of a coordination saturated buckyball (Scheme 1).
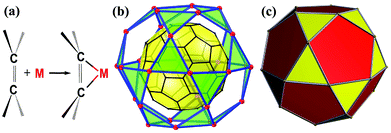 |
| Scheme 1 Formation of η2-fashion metal-olefin complex (a), the proposed icosidodecahedral C60@M30 structure in a μ30-(η2)30 coordination mode (b), and the icosidodecahedron polyhedron (c). For clarity, the coordination interactions between M and C C are omitted in (b). | |
Simple as it may seem, the highest nuclearity of the reported exohedral metallofullerenes to date is merely 6,8,9 including C60@M6 structures with six mononuclear clusters in η2 fashion (octahedron geometry)20 and two trinuclear clusters in (μ3–η2:η2:η2) fashion.21 Intuitively, metallofullerenes with nuclearity of more than 6 have been, until now, difficult to synthesize, most likely due to the steric crowding between bulky stabilizing ligands.
One of the key requisites to achieve a coordination saturated buckyball is adopting a vertex-shared M3-trigon coordination configuration. However, it seems like an impossible mission as the multiple auxiliary ligands surrounding the M3 unit prevent other M3 units from hovering over the neighbor hexagons and thus restrict the total number of M3 units on the C60 surface, particularly for these open-shell transition metals with a coordination number no less than 4.
The studies of metallofullerenes can be dated back to the early 1990s, when several metal-coated fulleride clusters with high nuclearity such as C60@Li12,22,23 C60@Ba32, C60@Ca32,24 and others25,26 were speculated to exist under specific conditions. Ab initio molecular dynamics calculation revealed that C60@Li12 could be a stable cluster. However, their exact structures were not confirmed due to the lack of analytical techniques, preventing further investigation of their functions.
Recently, Li's group at Jinan University and Zhan's group at Shantou University jointly reported a rhombicuboctahedral C60@Cu24, a metallofullerene of unprecedented high nuclearity.27 It is a giant leap toward complete coordination on the C60 surface. Overall, the ingenious strategies in their endeavor toward high nuclearity include: (i) adopting closed-shell Cu(I) with a lower coordination number instead of open-shell metals and (ii) adopting anionic bridge ligands instead of terminal ligands. Both of these strategies were intended to minimize the steric crowd of the M3 coordination sphere. As a continuous effort of the two research groups to explore metallofullerene complexes, four exohedral Cu(I)–C60 (cuprofullerene) carboxylate complexes with nuclearities of 6, 12, and 24 were successfully synthesized by solvothermal reactions of fluorocarboxylic/bicarboxylic acid, Cu2O, and C60 in aromatic solvents. From a structure perspective, all of the cuprofullerene complexes embody the same coordination mode of [μ3–η2:η2:η2)-C60]-Cu3-[(μ2–κ1:κ1)-COO]3 (Fig. 1a) with a Cu3
:
C60 ratio of 2
:
1, 4
:
1, and 8
:
1 (Fig. 1b–d). Particularly, the 24-nuclear cuprofullerene represents a rhombicuboctahedral C60@Cu24 core–shell structure with 8 Cu3 units canopying on the 8 independent hexagons of C60 (Fig. 1d), paving the road toward the completely coordinated C60. For detailed structural analysis, please refer to their recently published paper.27
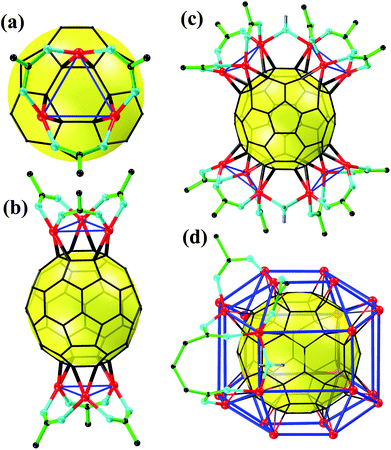 |
| Fig. 1 The simplified structures of the synthesized cuprofullerenes. (a) Cu3 unit, (b) C60@Cu6, (c) C60@Cu12 and (d) C60@Cu24. Color code: red, Cu; cyan, O; black, C; green, F; gray, H. | |
As opposed to the reported exohedral M3-metallofullerenes, in which the monodentate auxiliary ligands such as phosphine, CO, and isocyanide were frequently used,7–9 the synergistic associations of (μ2–κ1:κ1)-carboxylate and (μ3–η2:η2:η2)-hexagon could significantly enhance the stability of the Cu3 units. Furthermore, the low coordination of Cu(I) and the outward orientation of carboxylates far away from the buckyball could endow the Cu3 unit with much relieved steric crowding on the surface of C60, which provides an opportunity to squeeze in more Cu3 units on the adjacent hexagons to increase the overall nuclearity.
Besides adopting low coordination Cu(I) and carboxylate as auxiliary ligands, the use of fluorocarboxylate as ligands might also play an essential role in the formation of cuprofullerene complexes of high nuclearity. Compared with the non-fluorinated carboxylate, the poorly coordinated fluorocarboxylate facilitates relatively strong association of the weak basic olefin C60 with Cu(I), as reported in the tris(η2-ethene)-Cu(I) cationic complexes.28 As a matter of fact, using non-fluorinated n-butylcarboxylate under similar conditions did not produce a complex of similar nuclearity. Inspired by the successful evolution of nuclearity from C60@Cu6 to C60@Cu12 through increasing the concentration of heptafluorobutyrate auxiliary ligands, the C60@Cu24 structure was eventually realized by using dicarboxylate, hexafluoroglutarate and glutarate, as bridging ligands, which is vital to further increase the local concentration of carboxylates around the surface of the buckyball. As a result, the bridging dicarboxylate provides extra bridging interactions beyond μ2-H2O associating eight Cu3 units, further stabilizing the rhombicuboctahedral C60@Cu24 core–shell structure. It is worth pointing out that the authors were also able to obtain the extremely high-nuclear structure with non-fluorinated glutarate in 1-chloronaphthalene, in which the high solubility of C60 might have played an important role. It exemplifies the intricacy and complexity of the crystallization process, which leads us to believe that the final mission, the complete coordination of C60, can be accomplished by the fine tuning of the reaction systems. In addition, by closely looking into the geometry of the obtained C60@Cu24 molecule, it appears there is enough space above the 6 vacant C
C bonds to allow additional metal atoms and complete the proposed C60@Cu30 icosidodecahedron, provided the suitable auxiliary ligands are used. We look forward to the realization of the great Holy Grail by the authors as soon as possible.
Note that solvothermal conditions were utilized for the first time to prepare exohedral metallofullerene in this work. The high-boiling-point aromatic solvents make it possible to run the solvothermal reactions at rather high temperatures, and thus to overcome the energy barriers toward these high-nuclear cuprofullerenes.
The initial photophysical/photochemical properties were studied for the above-obtained Cu3-metallofullerenes. Their solid-state UV-Vis diffuse reflectance spectra exhibit absorption of the whole UV-visible area, indicating more efficient absorption of visible light than the pristine C60 due to the complexation of Cu(I). Theoretical calculations confirmed that the complexation could reduce the symmetry and disturb the π-system of C60, resulting in the symmetry-allowed excitation in the visible-light region. With the increase of nuclearity, the lowest absorptions of the four complexes show a hypsochromic shift, suggesting the potential influence of nuclearity on their electronic structures. Compared with the pristine C60 material, the cuprofullerene complexes show not only much higher efficient absorption of visible light, but also more controllable HOMO–LUMO gap, which implies an attractive prospect in utilizing solar energy.
As organocopper reagents, we could also expect these cuprofullerene complexes to function as novel catalysts in preparing organic fullerene derivatives,29 opening a new door for organic fullerene chemistry.
Conclusions
In short, the Li and Zhan groups have successfully prepared a series of exohedral cuprofullerene complexes, featuring Cu3 units canopying on the C60 surface in a (μ3–η2:η2:η2) fashion, by judiciously selecting fluorocarboxylate/dicarboxylate as auxiliary ligands and solvothermal reaction conditions. The ratio of M3
:
C60 has been expanded from 2
:
1 to 4
:
1 and 8
:
1, forming the unprecedented 12- and 24-nuclear cuprofullerenes. Particularly, the 24-nuclear complex, a rhombicuboctahedral C60@Cu24 core–shell structure, represents an exohedral metallofullerene of the most nuclearity, a giant leap toward the coordination saturated C60@M30. This work greatly enriches the structural diversity of exohedral metallofullerenes and is expected to bring advanced applications such as in the photoelectric conversion and catalytic synthesis of organic fullerene derivatives. We look forward to the realization of C60@M30 icosidodecahedron and its intriguing applications in the near future.
Conflicts of interest
There are no conflicts to declare.
Acknowledgements
This work was financially supported by the National Key Basic Research Program of China (2016YFA0203400), the Key Project of Basic Research of Shanghai (17JC1403100 and 18JC1413200) and the Shanghai Rising-Star Program (19QA1404300).
References
- H. W. Kroto, J. R. Health, S. C. O'Brein, R. F. Curl and R. E. Smalley, C60: Buckminsterfullerene, Nature, 1985, 318, 162–163 CrossRef CAS.
- D. M. Guldi and M. Prato, Excited-State Properties of C60 Fullerene Derivatives, Acc. Chem. Res., 2000, 33, 695–703 CrossRef CAS PubMed.
- H. Kang, W. Lee, J. Oh, T. Kim, C. Lee and B. J. Kim, From Fullerene−Polymer to All-Polymer Solar Cells: The Importance of Molecular Packing, Orientation, and Morphology Control, Acc. Chem. Res., 2016, 49, 2424–2434 CrossRef CAS PubMed.
- K. Lee, H. Song and J. T. Park, [60]Fullerene-Metal Cluster Complexes: Novel Bonding Modes and Electronic Communication, Acc. Chem. Res., 2003, 36, 78–86 CrossRef CAS PubMed.
- T. Wang and C. Wang, Endohedral Metallofullerenes Based on Spherical Ih-C80 Cage: Molecular Structures and Paramagnetic Properties, Acc. Chem. Res., 2014, 47, 450–458 CrossRef CAS PubMed.
- J. Zhang, S. Stevenson and H. C. Dorn, Trimetallic Nitride Template Endohedral Metallofullerenes: Discovery, Structural Characterization, Reactivity, and Applications, Acc. Chem. Res., 2013, 46, 1548–1557 CrossRef CAS PubMed.
- A. L. Balch and M. M. Olmstead, Reactions of Transition Metal Complexes with Fullerenes (C60,C70, etc.) and Related Materials, Chem. Rev., 1998, 98, 2123–2165 CrossRef CAS PubMed.
- A. L. Balch and K. Winkler, Two-Component Polymeric Materials of Fullerenes and the Transition Metal Complexes: A Bridge between Metal-Organic Frameworks and Conducting Polymers, Chem. Rev., 2016, 116, 3812–3882 CrossRef CAS PubMed.
- M. A. Lebedeva, T. W. Chamberlain and A. N. Khlobystov, Harnessing the Synergistic and Complementary Properties of Fullerene and Transition-Metal Compounds for Nanomaterial Applications, Chem. Rev., 2015, 115, 11301–11351 CrossRef CAS PubMed.
- O. Ostroverkhova, Organic Optoelectronic Materials: Mechanisms and Applications, Chem. Rev., 2016, 116, 13279–13412 CrossRef CAS PubMed.
- S. S. Babu, H. Mohwald and T. Nakanishi, Recent progress in morphology control of supramolecular fullerene assemblies and its applications, Chem. Soc. Rev., 2010, 39, 4021–4035 RSC.
- C. García-Simón, M. Costas and X. Ribas, Metallosupramolecular receptors for fullerene binding and release, Chem. Soc. Rev., 2016, 45, 40–62 RSC.
- S.-Z. Zhan, J.-H. Li, G.-H. Zhang, M.-D. Li, S. Sun, J. Zheng, G.-H. Ning, M. Li, D.-B. Kuang, X.-D. Wang and D. Li, Coordination disk-type nano-Saturn complexes, Chem. Commun., 2020, 56, 3325–3328 RSC.
- H. Cong, B. Yua, T. Akasak and X. Lu, Endohedral metallofullerenes: An unconventional core-shell coordination union, Coord. Chem. Rev., 2013, 257, 2880–2898 CrossRef CAS.
-
A. Hirsch and M. Brettreich, Fullerenes: Chemistry and Reactions, Wiley-VCH, Weinheim, 2005 Search PubMed.
- Y. Zhang, M. Yao, M. Du, Z. Yao, Y. Wang, J. Dong, Z. Yang, B. Sundqvist, E. Kovats, S. Pekker and B. Liu, Negative Volume Compressibility in Sc3N@C80-Cubane Cocrystal with Charge Transfer, J. Am. Chem. Soc., 2020, 142, 7584–7590 CrossRef CAS PubMed.
- Y. Chai, X. Liu, B. Wu, L. Liu, Z. Wang, Y. Weng and C. Wang, In Situ Switching of Photoinduced Electron Transfer Direction by Regulating the Redox State in Fullerene-Based Dyads, J. Am. Chem. Soc., 2020, 142, 4411–4418 CrossRef CAS PubMed.
- P. J. Fagan, J. C. Calabrese and B. Malone, The Chemical Nature of Buckminsterfullerene (C60) and the Characterization of a Platinum Derivative, Science, 1991, 252, 1160–1161 CrossRef CAS.
- J. M. Hawkins, A. Meyer, T. A. Lewis, S. Loren and F. J. Hollander, Crystal Structure of Osmylated C60: Confirmation of the Soccer Ball Framework, Science, 1991, 252, 312–313 CrossRef CAS PubMed.
- P. J. Fagan, J. C. Calabrese and B. Malone, A multiply-substituted buckminsterfullerene (C60) with an octahedral array of platinum atoms, J. Am. Chem. Soc., 1991, 113, 9408–9409 CrossRef CAS.
- B. K. Park, C. Y. Lee, J. Jung, J. H. Lim, Y.-K. Han, C. S. Hong and J. T. Park, [Os3(CO)6(PMe3)3](μ3-η2:η2:η2-C60)[Re3(μ-H)3(CO)9]: A Fullerene[60] Coordinated to Two Different Trinuclear Clusters, Angew. Chem., Int. Ed., 2007, 46, 1436–1439 CrossRef CAS PubMed.
- Y. Chabre, D. Djurado, M. Armand, W. R. Romanow, N. Coustel, J. John, P. McCauley, J. E. Fischer and A. B. Smith, Electrochemical Intercalation of Lithium into Solid C60, J. Am. Chem. Soc., 1992, 114, 764–766 CrossRef CAS.
- J. Kohanoff, W. Andreoni and M. Parrinello, A possible new highly stable fulleride cluster: Li12C60, Chem. Phys. Lett., 1992, 198, 472–477 CrossRef CAS.
- U. Zimmermann, N. Malinowski, U. Näher, S. Frank and T. P. Martin, Multilayer metal coverage of fullerene molecules, Phys. Rev. Lett., 1994, 72, 3542–3545 CrossRef CAS PubMed.
- F. Tast, N. Malinowski, S. Frank, M. Heinebrodt, I. M. L. Billas and T. P. Martin, Cage Destruction in Metal-Fullerene Clusters, Phys. Rev. Lett., 1996, 77, 3529–3532 CrossRef CAS PubMed.
- F. Tast, N. Malinowski, S. Frank, M. Heinebrodt, I. M. L. Billas and T. P. Martin, Transition metal coated fullerenes, Z. Phys. D, 1997, 40, 351–354 CrossRef CAS.
- S.-Z. Zhan, G.-H. Zhang, J.-H. Li, J.-L. Liu, S.-H. Zhu, W. Lu, J. Zheng, S. W. Ng and D. Li, Exohedral Cuprofullerene: Sequentially Expanding Metal Olefin Up to a C60@Cu24 Rhombicuboctahedron, J. Am. Chem. Soc., 2020, 142, 5943–5947 CrossRef CAS PubMed.
- G. Santiso-Quinñones, A. Reisinger, J. Slattery and I. Krossing, Homoleptic Cu-phosphorus and Cu-ethene complexes, Chem. Commun., 2007, 47, 5046–5048 RSC.
- Y. Matsuo and E. Nakamura, Selective Multiaddition of Organocopper Reagents to Fullerenes, Chem. Rev., 2008, 108, 3016–3028 CrossRef CAS PubMed.
|
This journal is © the Partner Organisations 2020 |
Click here to see how this site uses Cookies. View our privacy policy here.