DOI:
10.1039/C9CC05238K
(Feature Article)
Chem. Commun., 2019,
55, 11979-11991
Stereochemical diversity in pyrrolidine synthesis by catalytic asymmetric 1,3-dipolar cycloaddition of azomethine ylides
Received
8th July 2019
, Accepted 13th September 2019
First published on 13th September 2019
Abstract
The pyrrolidine ring is a privileged structural motif in synthetic and medicinal chemisty. This review aims to highlight the high versatility of the catalytic asymmetric 1,3-dipolar cycloaddition of azomethine ylides for access to different types of stereochemical patterns in enantioselective pyrrolidine synthesis. Special attention will be paid to stereodivergent procedures giving rise to different stereoisomers from the same starting materials.
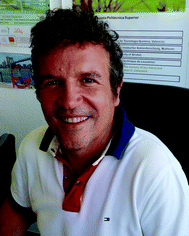
Javier Adrio
| Javier Adrio completed his PhD thesis at the Department of Organic Chemistry (UAM), under the supervision of Prof. Juan Carlos Carretero. From 2001 to 2003 he worked on PharmaMar as a senior scientist, during this period he undertook postdoctoral studies at the University of Pennsylvania with Prof. Madeleine Joullié. He returned to the UAM as a Ramon y Cajal researcher until he was appointed Associate Professor. He has spent several short stays as a Visiting Scholar at the University of Pennsylvania working in Prof. Patrick Walsh's lab His research interest includes the development of catalytic asymmetric transition metal catalyzed methodologies. |
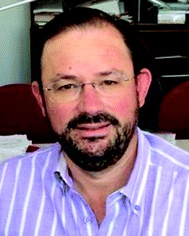
Juan C. Carretero
| Juan Carlos Carretero completed his PhD at the University Autonomous of Madrid (UAM) in 1985 under the direction of Prof. José L. García Ruano. From 1985 to 1988 he was a postdoctoral fellow at the Université Catholique de Louvain (Belgium) with Prof. Léon Ghosez. He subsequently joined the Department of Organic Chemistry of the UAM, where he became Associate Professor in 1988 and Professor of Organic Chemistry in 2000. He received the Janssen-Cilag Award in Organic Chemistry from the Spanish Royal Society of Chemistry in 2010. His current research interests are mainly focused on metal-catalyzed reactions, C–H functionalization processes and catalytic asymmetric cycloadditions. |
1. Introduction
The pyrrolidine ring has become a privileged structure in synthetic and medicinal chemistry. The great interest in this heterocycle is based on several aspects: (a) pyrrolidines constitute the central structure of the proline amino acid and are present in a plethora of natural products;1 (b) in the last few years, chiral pyrrolidines have become leading scaffolds in ligand design either in transition metal mediated or organocatalytic processes;2 (c) many substituted pyrrolidine derivatives are acknowledged to possess a wide range of bioactivities, such as antibiotic, antibacterial, antifungal and cytotoxic effects, and consequently offer an excellent opportunity for the discovery of new pharmaceuticals agents.3 Indeed, there are more than 20 marketed drugs containing the pyrrolidine scaffold in theirstructure.4
Over the past fifteen years, the catalytic asymmetric 1,3-dipolar cycloaddition of azomethine ylides has emerged as a powerful methodology for the enantioselective convergent preparation of pyrrolidines with an increasing variety of substitution patterns. During this period of time the effort of numerous research groups has been focused on the development of a wide variety of chiral catalysts, both transition metal complexes and small organic molecules (organocatalysts). This pool of new catalysts has also enabled the improvement of the structural scope of this cycloaddition, originally limited to the use of highly activated olefins and α-iminoester derivatives as azomethine ylide precursors. Thus, less activated dipolarophiles and more challenging 1,3-dipoles have been incorporated into the arsenal of suitable substrates for this transformation. The growing progress in the synthetic interest of this catalytic asymmetric reaction with outstanding results has been summarized in several reviews.5 In addition, the first examples of higher order cycloadditions of azomethine ylides (e.g. [3+3] and [6+3] cycloadditions) have been reported in recent years.6
1.1 Stereochemical issues
With four sp3-hybridized carbon atoms in its structure the pyrrolidine ring can present up to four stereocentres, and consequently up to 16 different stereoisomers. Since every stereoisomer can display different biological properties, the development of selective reactions that allow the preparation of pyrrolidines with several stereocenters and complete control of both absolute and relative configuration is crucial in drug discovery.
The catalytic asymmetric 1,3-dipolar cycloaddition can occur either through a concerted (most common) or a stepwise mechanism. Both pathways usually take place with complete or very high stereospecificity. Consequently the 3,4 configuration of the pyrrolidine depends exclusively on the stereochemistry of the dipolarophile (Scheme 1A).
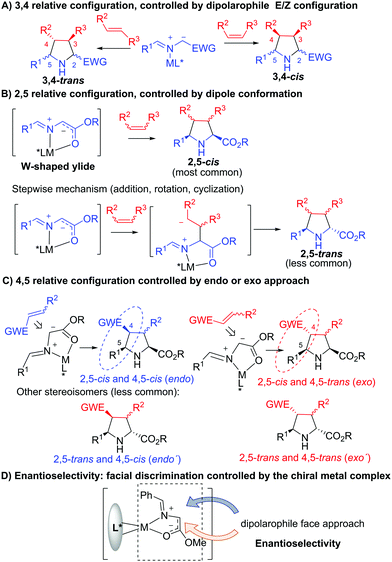 |
| Scheme 1 Controlling factors on the stereochemical outcome of metal-catalyzed asymmetric 1,3-dipolar cycloaddition of stabilized azomethine ylides. | |
Typically, the 2,5-cis diastereoselectivity obtained in this catalyzed cycloaddition is based on the participation of a metal complex by bidentate coordination of the azomethine ylide precursor with a chiral Lewis acid. The formation of this complex facilitates the deprotonation of the iminoester and the transmission of the chiral information from the chiral ligand. The coordination with the nitrogen and oxygen atoms of the azomethine precursor also fixes the W-conformation of the 1,3-dipole, which determines the usual 2,5-cis configuration of the final pyrrolidine (Scheme 1B). However, less common 2,5-trans pyrrolidines have also been prepared. This stereochemical result has been justified by a stepwise mechanism involving a Michael addition, a rotation of the C–N bond, and a Mannich reaction to afford the 2,5-trans adduct (see Section 2.4).
The 4,5-configuration of the pyrrolidine depends on the exo or endo approach of the dipolarophile to the dipole (Scheme 1C), whereas the control of the enantioselectivity is based on the ability of the chiral ligand to promote a good discrimination between both faces of the metallo-dipole (Scheme 1D).
Finally, with the goal of developing more stereochemically efficient methodologies, catalytic stereodivergent procedures are extremely appealing. In these processes the judicious choice of the catalytic system could enable a controllable access to more than one diastereoisomer from the same starting materials.7 In the last few years, several examples of catalytic asymmetric stereodivergent 1,3-dipolar cycloadditions of azomethine ylides have been developed, facilitating the preparation of structurally diverse small-molecule libraries.
1.2 Organization of the review
Due to the ongoing progress in the stereochemical control of the metal catalyzed asymmetric 1,3-dipolar cycloaddition of azomethine ylides, the aim of this review is focused on the stereochemical diversity achievable by this reaction rather than its structural scope, summarized in other reviews.5 Our goal is to provide the readers with an overview on the impact of the structure of the catalyst, starting materials and reaction conditions on the stereoselectivity of the cycloaddition, with main focus on recent developments. A selection of representative examples to illustrate this stereochemical diversity will be presented, starting with a selected historic compilation of the most frequent and well developed pyrrolidine configurations. In the second part special attention will be devoted to stereodivergent procedures.7 In addition, some mechanistic aspects will be presented highlighting the relationships between catalyst structure and diastereoselectivity.
2.
endo/exo selectivity
As it is shown in Scheme 1, in the 1,3 dipolar cycloaddition with azomethine ylides two possible diastereomeric cycloadducts can be formed as result of the endo or exo approach of the 1,3-dipole and dipolarophile. In principle, the endo approach would be favoured by secondary orbital interaction, in a similar way as in the Diels–Alder reaction. However, in 1,3-dipolar cycloadditions this stabilizing orbital interaction is usually much smaller and the unfavourable steric effects can play a major role in the observed diastereoselectivity. Therefore, the endo/exo selectivity of the reaction depends frequently on the steric hindrance and electronic nature of the substitution at both reaction partners, as well as the metal complex used as catalyst. As a general trend, It is worth mentioning that the silver-catalyzed 1,3-dipolar cycloadditions are typically more endo selective than the corresponding copper-catalyzed processes. In the latter case the endo/exo highly depends on the ligand and dipolarophile.
2.1 Silver-catalyzed cycloadditions
2.1.1.
endo-Selective cycloadditions (4,5-cis pyrrolidines).
The first examples of transition metal catalyzed 1,3-dipolar cycloadditions of azomethine ylides were reported by the groups of Zhang8 and Jorgensen9 in 2002, and both procedures afforded the corresponding endo pyrrolidines in the reaction of α,β-unsaturated esters with excellent enantioselectivity. From these findings, a set of asymmetric procedures were described for the endo selective cycloaddition of α,β-unsaturated carboxylic acid derivatives using different transition metal complexes and chiral ligands. In this arena, chiral silver complexes have afforded excellent results, becoming the most used catalysts for the preparation of endo-pyrrolidines under different reaction conditions.10 A highly stabilizing bonding interaction between the carbonyl group of the dipolarophile and the silver centre has been proposed to explain the excellent observed endo diastereoselectivity.11 In Scheme 2 the most relevant results obtained in silver catalyzed cycloaddition are summarized using maleates, fumarates, acrylates and maleimides as dipolarophiles. In 2002, Zhang and co-workers7 reported the endo selective cycloaddition between iminoesters and dimethyl fumarate using the complex AgI/xilyl-FAP (1) as a catalyst (Scheme 2, Aa). The AgI/Quinap (9) catalytic system developed by Schereiber and co-workers enlarged the substrate scope of the reaction by the use of other iminoesters different from glycinates (R3 ≠ H).10a The endo pyrrolidines were obtained with excellent diastereo and enantioselectivity in the reaction with acrylates (Scheme 2, Ca). Ferrocenyloxazoline 210b and Ugi-amine derived ferrocenyl ligands such as 3,10c4,10f810i and 1210k afforded the endo adducts with high enantiocontrol in the silver catalyzed cycloaddition with maleates (Scheme 2, Ab, Ac, Ad, Ag),10b,c,f,i fumarates (Ba, Bc),10b,i acrylates (Cb, Ce)10b,i and maleimides (Da, Db, De, Dh).10b,c,i,k Ligands with axial chirality such as BINAP (11)10d and BiphamPhos (5)10g have been also successfully applied in this transformation (Scheme 2, Ae, Cc, Dc, Dg). In particular, the BiphamPhos family of ligands developed by Wang and co-workers showed a wide scope regarding both the dipole and dipolarophile partner.10g In 2008 Najera and co-workers10e described that the combination of the phosphoramidite monodentate ligand 7 and a silver salt was an excellent catalytic system for the endo selective cycloaddition of azomethine ylides with acrylates and fumarates (Scheme 2, Bb, Cd, Dd). A sulfinamide derived non-biaryl atropoisomer ligand 10 was a very efficient ligand in the silver catalyzed cycloaddition with malemides (Scheme 2, Df).10j Finally, the chiral secondary amine–amidophosphane 6 in combination with Ag2CO3 served as an efficient catalyst in the reaction with diethyl maleate (Scheme 2, Af).10h
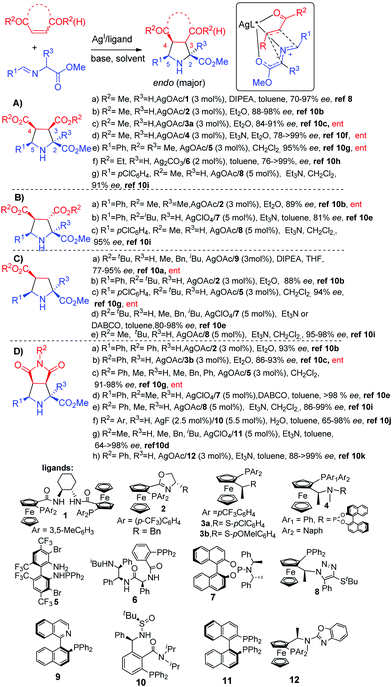 |
| Scheme 2 Silver-catalyzed endo selective cycloaddition of azomethine ylides with α,β-unsaturated carboxylic acid derivatives. | |
Since 2005 enones, nitroalkenes and vinylsulfones have been incorporated to the arsenal of suitable dipolarophiles (Scheme 3). In 2009, Najera and co-workers reported the first examples of silver catalyzed endo selective cycloaddition of azomethine ylides with acyclic and cyclic enones using phosphoramidite 7 as a ligand (Scheme 3, Aa).11 Later the Fukuzawa12 and Zheng13 groups developed a couple of catalytic systems for this transformation based on the ferrocenyl ligands 8 and 13 (Scheme 3, Ab, Ac). An efficient silver/TF biphamPhos 5 catalyzed asymmetric desymmetrization of prochiral cyclopentenediones was also reported by Wang and co-workers (Scheme 3, Ad).14
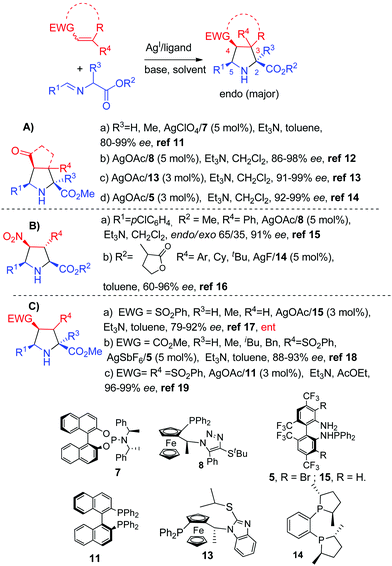 |
| Scheme 3 Silver-catalyzed endo-selective cycloaddition of azomethine ylides with enones, nitroalkenes and vinyl sulfones. | |
Regarding the use of nitroalkenes (Scheme 3, B), in 2010 Fukuzawa and co-workers15 reported that with the system AgOAc/ClickFerrophos 8, the reaction with p-chlorobenzylidene methyl glycinate occurred with high enantioselectivity (91% ee) but in a low yield and diastereoselectivity (Scheme 3, Ba). The synthesis of spiro-prolinates was described by Sansano, Cossío and co-workers by the AgF/Me-DuPhos·14 catalyzed cycloaddition of α-imino-γ-lactones and nitroalkenes (Scheme 3, Bb).16 In 2008 Wang and co-workers reported the first examples of endo selective silver catalyzed cycloaddition of vinyl phenyl sulfone using TF-BiphamPhos 15 as a ligand (Scheme 3, Ca).17 The scope of the sulfonyl dipolarophile was later expanded to β-sulfonylacrylates (Scheme 3, Cb)18 and 1,2-bis-phenylsulfonyl ethylene (Scheme 3, Cc).19
Although α-iminoglycinates are the standard, typically used, azomethine precursors (R = H), substituted α iminoesters derived from other aminoacids, such as alanine, phenylalanine, valine, etc. have also been successfully applied in some cases.10a,d,e,11,17,18
2.1.2.
exo-Selective silver-catalyzed cycloadditions (4,5-trans pyrrolidines).
As previously mentioned the examples of silver catalyzed exo selective cycloadditions are very rare: in 2011, Kobayashi and co-workers20 reported the first examples of 1,3-dipolar cycloaddition of azomethine ylides and diverse olefins, catalyzed by an AgHMDS/DTBM-segphos ligand (16) leading to the corresponding pyrrolidines with excellent exo diasteroselectivity and enantioselectivity (Scheme 4). As it will be emphasized throughout this review the very bulky DTBM-segphos ligand typically leads to the formation of the exo adducts with high selectivity due to the destabilizing steric interaction between the substituents of the phosphine and the dipolarophile in the endo approach.
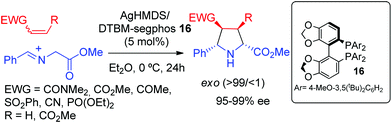 |
| Scheme 4 AgHMDS/DTBM-segphos catalyzed exo-selective asymmetric 1,3-dipolar cycloaddition of azomethine ylides. | |
An unusual exo-selective 1,3-dipolar cycloaddition between iminoesters and nitroalkenes using silver triflate or silver benzoate phosphoramidite 7 complexes was reported by Nájera, Sansano, Cossío and co-workers.21 DFT calculations demonstrated the crucial role of the counteranion: the OTf anion remained coordinated to the silver atom along the reaction pathway avoiding the stabilizing endo interaction between the metal and the nitro group of the dipolarophile (Scheme 5).
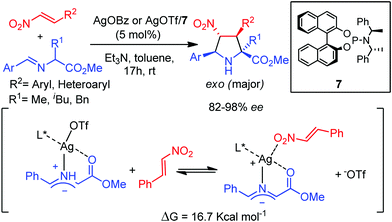 |
| Scheme 5 Ag/phosphoramidite catalyzed exo selective asymmetric 1,3-dipolar cycloaddition of azomethine ylides with nitroalkenes. | |
In 2005, Xia, Xu and co-workers described the synthesis of a new family of non-biaryl atropoisomer chiral ligands. These sulfinyl phosphine ligands afforded excellent levels of exo diastereoselectivity and enantioselectivity in the silver catalyzed exo-selective 1,3-dipolar cycloaddition of iminoesters and nitroalkenes (Scheme 6).22
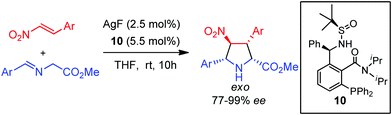 |
| Scheme 6
exo-Selective cycloaddition of azomethine ylides with nitroalkenes catalyzed by Xing-Phos/Ag complex. | |
The applicability of a Xing-Phos ligand (10) was efficiently extended to other dipolarophiles such as chalcones and the less reactive methyl cinnamates (Scheme 7).23
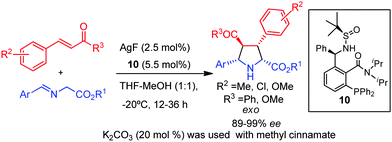 |
| Scheme 7 Xing-Phos/Ag exo-selective cycloaddition of azomethine ylides with chalcones. | |
2.2 Copper-catalyzed cycloadditions
Together with a silver based catalyst, copper(I) complexes are the most widely employed catalysts in asymmetric 1,3-dipolar cycloadditions of azomethine ylides.
The first examples of chiral copper complexes were reported by Komatsu and co-workers in 2003.24 The reaction with maleimide or fumarate, catalyzed by BINAP (11)/Cu(OTf)2 complexes, afforded mainly the exo adducts albeit with modest enantioselectivities (Scheme 8, Ca, Da). Two years later, the first copper catalyzed highly exo-selective cycloadditions were reported by Zhang and co-workers:25 the reaction of azomethine ylides with acrylates in the presence of CuI/ferrocenyl P,N-ligand 17 resulted in the exo pyrrolidines in up to 98% ee (Scheme 8, Aa). The same year, our research group26 described a general protocol involving CuI/Fesulphos 18 as a catalyst, providing high levels of diastereo and enantioselectivity with a wide variety of iminoesters and dipolarophiles (Scheme 8, Ab, Ba, Cb, Db). The endo/exo selectivity proved to be highly dependent on the dipolarophile. Thus, whereas the endo adduct was exclusively obtained from maleimide the diatereoselectivity was much poorer with fumarate, maleate and acrylates (endo/exo: 90/10, 67/33 and 75/25, respectively). Similarly, the CuOAc/ClickFerrophos 19 complex developed by Fukuzawa and co-workers provided good results in the reaction with acrylates, maleates, fumarates and maleimides. Except in the case of the fumarate (Scheme 8, Cc), the exo adducts were predominantly formed with high enantioselectivity (Scheme 8, Ac, Bb, Dc).27 Alternatively, the endo adducts were selectively prepared using the CuI/TF-BiphamPhos 5 complex.28 Excellent enantioselectivity was also observed in the reaction with acrylates and maleates (Scheme 8, Ad, Bc), and different types of iminoesters.
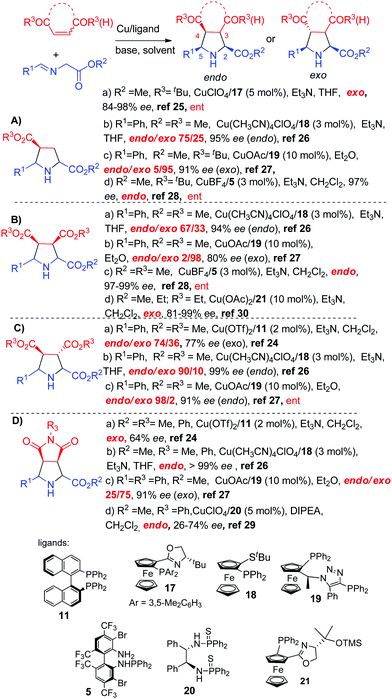 |
| Scheme 8 Copper catalyzed 1,3-dipolar cycloaddition of azomethine ylides with α,β-unsaturated carboxylic acid derivatives. | |
Shi and co-workers reported a new thiophosphoramide ligand (20) for the CuI-promoted cycloaddition with maleimides, leading to the endo adducts with moderate enantioselectivities (Scheme 8, Dd).29 The P,N-ferrocenyl ligand (21) developed by Bdiri30 and co-workers afforded excellent results in the exo-selective CuII-catalyzed cycloaddition with maleates (Scheme 8, Bd).
Copper catalysts have been also widely employed in the reaction with enones, nitroalkenes, and vinylsulfones. The first procedure for the catalytic enantioselective cycloaddition of azomethine ylides with enones was reported by our research group in 2009 using the CuI/Fesulphos 18 complex (Scheme 9, Aa). The endo/exo diastereoselectivity proved to be highly dependent on the E/Z configuration of the enone. Thus, acyclic trans-enones led to the formation of the exo adducts, whereas cyclopentenone provided the endo pyrrolidines.31 We have recently reported that the same catalytic system afforded excellent results in the reaction with cyclobutenones.32 In this case, taking advantage of the ring strain of the dipolarophile sterically demanding substrates including β-substitution at the cyclobutenone unit, provided the endo adducts with excellent diastereo and enantioselectivity (Scheme 9, Ab).32
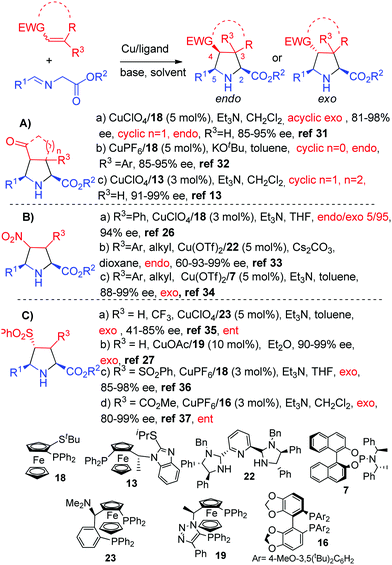 |
| Scheme 9 Copper-catalyzed 1,3-dipolar cycloaddition of azomethine ylides with enones, nitroalkenes and vinylsulfones. | |
Zhang and co-workers extended the scope of the reaction to cyclohexenones with ImiFerroS 13 as a ligand (up to 99% ee, Scheme 9, Ac).13
In 2005, our research group reported the catalytic asymmetric cycloaddition of methyl glycinate with nitrostyrene. The use of CuI/Fesulphos 18 as a catalytic system enabled the formation of the exo pyrrolidine with excellent diastereo and enantioselectivity (Scheme 9, Ba).26 In 2010 Arai and co-workers described the copper(II)/PyBidine 22 catalyzed endo-selective version of this reaction (Scheme 9, Bb).33 Later, a CuIIexo-selective cycloaddition was reported by Sansano and co-workers34 with the phosphoramidite ligand 7 (Scheme 9, Bc).
The first catalytic asymmetric cycloaddition of vinyl sulphones was described by our research group.35 Almost complete exo selectivity and excellent enantioselectivities were obtained using CuClO4/Taniaphos 23 as the catalyst system (Scheme 9, Ca). The CuI/ClickFerrophos 19 combination also provided excellent results in this cycloaddition (Scheme 9, Cb).27 Later, our research group extended the scope of the reaction to novel sulfonyl dipolarophiles such as bis-sulfonyl ethylenes36 (Scheme 9, Cc, ligand 18), and sulfonylacrylates (Scheme 9, Cd, ligand 16).37
2.3 Other metal-catalyzed cycloadditions
As previously mentioned,9 in 2002 Jorgensen and co-workers described that the combination of Zn(OTf)2 with a BOX ligand 24 was very effective for the endo selective cycloaddition of azomethine ylides with acrylate and fumarate (Scheme 10, Aa, Ca). Later, Dogan, Garner and co-workers developed a chiral ferrocenyl aziridino ligand 25 for Zn-catalyzed cycloadditions: the endo adducts were obtained with high ee's in the reaction with acrylates, maleates or maleimides38 (Scheme 10, Ab, Ba, Cb). In 2008 Shi and co-workers39 demonstrated the feasibility of Ni complexes, and excellent endo and enantioselectivities were obtained in the reaction with maleimides catalysed by Ni(ClO4)2/BINIM 26 (Scheme 10, Db). On the other hand, chiral calcium complexes were reported by Kobayashi and co-workers in 2008.40 The catalytic system prepared from Ca(iPrO)2 and bisoxazoline 27 afforded the endo adducts with very high diastereo and enantioselectivities in the reaction with crotonates and alkenyl amides (Scheme 10, Ac).
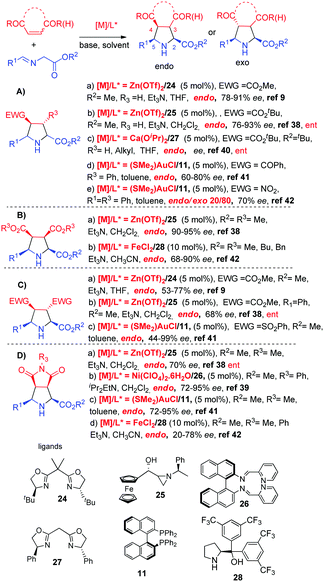 |
| Scheme 10 Zn-, Ni-, Ca-, Au- and Fe-catalyzed 1,3-dipolar cycloadditions of azomethine ylides. | |
The endo selective cycloadditions of iminoesters with maleimides, bis-sulfonylethylene, chalcone and nitrostyrene were also efficiently catalyzed by the Au-BINAP 11 complex (Scheme 10, Cc, Dc).41
In 2011 Wang and co-workers reported the FeII/diaryl prolinol 28 catalyzed asymmetric 1,3-dipolar cycloaddition of azomethine ylides with maleates and maleimides providing the endo adducts with modest enantioselectivities (Scheme 10, Bb, Dd).42 Interestingly, except for the case of gold catalyzed reactions with nitrostyrene (Scheme 10, Ae), all the reactions summarized in Scheme 10 led to the formation of the endo isomers.
2.4
exo′/endo′ isomers: pyrrolidines with 2,5-trans configuration
Unlike pyrrolidines with 2,5-cis configuration, the enantioselective access to the 2,5-trans diastereomers has been described much later and it is still underdeveloped. In 2010 Arai and co-workers reported the first metal-catalyzed exo′-selective (2,5-trans configuration) cycloaddition of azomethine ylides,43 which differed from all the precedents described to that date via W-shaped azomethine metal complexes and consequent formation of 2,5-cis substituted pyrrolidines. This unusual stereochemistry was obtained in the Ni/imidazoline–aminophenol 29 catalyzed reaction of iminoesters with nitroalkenes. This stereochemical outcome suggested that the cycloaddition takes place through a stepwise mechanism.44 Thus, the proposed mechanism would involve an initial anti selective Michael addition of the metallo-dipole to the corresponding nitroalkene controlled by the interaction of the nitro group with the nickel centre. The subsequent coordination of a Ni atom to the nitronate would allow the rotation of the C–N bond before the Mannich reaction, which would afford the exo′ adduct. DFT calculations confirmed that the exo′-cycloadduct is the most stable of the four possible pyrrolidines (Scheme 11).
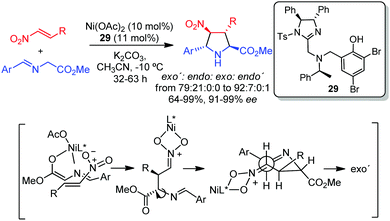 |
| Scheme 11 Ni-Catalyzed exo′-selective 1,3-dipolar cycloaddition of azomethine ylides with nitroalkenes. | |
More recently, the Arai group has extended this methodology to the use of 3-nitroindoles as dipolarophiles using copper(II) as a metal.45 The CuII/PyBidine 22 complex enabled the dearomative cycloaddition process leading to the corresponding exo′ pyrroloindoline compounds with high diastereo and enantioselectivity (Scheme 12).
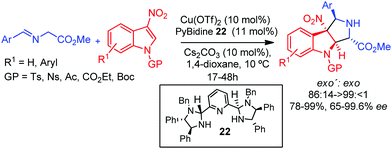 |
| Scheme 12 CuII-Catalyzed exo′ selective 1,3-dipolar cycloaddition of azomethine ylides with 3-nitroindoles. | |
3. Stereodivergent reactions
As pointed out above, the metal catalyzed asymmetric 1,3-dipolar cycloaddition of azomethine ylides has experienced outstanding progress and a diversity of highly diastereo and enantioselective procedures have been developed.5 However, most of these methods are oriented to the selective synthesis of either the endo or the exo diastereoisomer. Only in recent years has special focus been applied to the stereodivergent preparation of several diastereomers from the same starting materials, which significantly improves the synthetic efficiency of this reaction.7 As described below this stereodivergence can be addressed using either ligand-controlled or metal-controlled cycloadditions.
3.1 Ligand-controlled diastereodivergent reactions
The first example of catalytic asymmetric diastereodivergent 1,3-dipolar cycloaddition of iminoesters with nitroalkenes was reported by Hou and co-workers in 2006.46 By proper choice of electron rich or electron deficient aryl groups on the phosphorous atom of the ferrocenyl phophine oxazoline ligand a reversal in the exo/endo diastereoselectivity was observed. Thus, the cycloaddition in the presence of ligand 31 provided the exo adduct as a major isomer, whereas with ligand 32 the endo-diastereomers were selectively obtained. Computational studies suggested that the transition state models A and B were the most favourable approaches for the formation of exo and endo adducts, respectively (Scheme 13). In structure A the nitro group is far from the aromatic groups of the ligand, while in structure B it lies between the two aromatic substituents of the phosphine. Thus, the transition structure A which would lead to the exo product is expected to be more stable with electron rich aryl groups. Conversely, the approach B is stabilized by electrostatic interactions when the aryl groups are electron deficient.
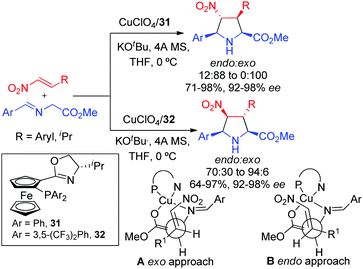 |
| Scheme 13 Diastereodivergent cycloaddition of α-iminoesters with nitroalkenes. | |
In 2010 our research group described the catalytic asymmetric 1,3-dipolar cycloaddition of azomethine ylides with β-sulfonylenones.47 The regioselectivity of the reaction catalyzed by CuI/Segphos-type ligands was mainly controlled by the carbonyl group, leading to 4-acetyl pyrrolidines as the major products. Interestingly, the endo/exo selectivity could be controlled by the substitution on the phosphorous atoms of the segphos-type ligands. Thus, the use of the segphos ligand 33 (Ar = Ph) afforded the endo isomers while the sterically hindered and electron rich DTBM-segphos 16 led to the exo isomers with high selectivity (Scheme 14).
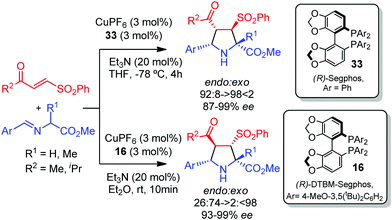 |
| Scheme 14 Catalytic asymmetric 1,3-dipolar cycloaddition of azomethine ylides with β-sulfonylenones. | |
In 2011 Wang and co-workers developed a new methodology for the enantioselective synthesis of 3-trifluoromethylated pyrrolidines from (Z) or (E)-4,4,4-trifluorocrotonates.48 The catalyst CuI/(S)-TF-BiphamPhos 5 led to the endo isomers with high enantioselectivity regardless of the (Z) or (E) configuration of the trifluorocrotonate. The exo-selective cycloaddition was later developed using the bulky electron rich biphosphine ligand DTBM-Biphep 34 (Scheme 15).49
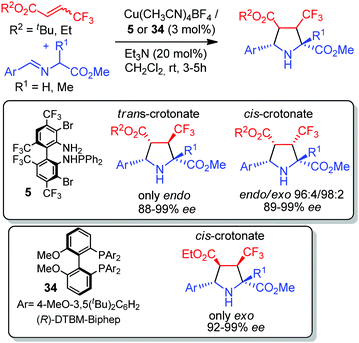 |
| Scheme 15 CuI/(S)-TF-BiphamPhos catalyzed cycloaddition with trifluorocrotonates. | |
A new family of hybrid pyrrolidine ferrocene ligands, developed by Cossío and co-workers, enabled the diastereodivergent cycloaddition of azomethine ylides with nitroolefins.50 The endo cycloadducts were obtained in high yield and enantioselectivity using a CuI/ligand 35 complex, whereas the combination of CuI and ligand 36 afforded selectively the endo pyrrolidines. DFT calculations indicated that in the endo transition state the metallated azomethine ylide is only coordinated to the phosphorous atom of the ligand and, consequently, the Cu atom has a vacant orbital to coordinate the nitro group. However, ligand 36 showed a bidentate coordination, where the copper atom was bound to the phosphorous and nitrogen atoms. Therefore, the nitro group of the dipolarophile was not available to coordinate the metal and the exo pyrrolidines were formed (Scheme 16).
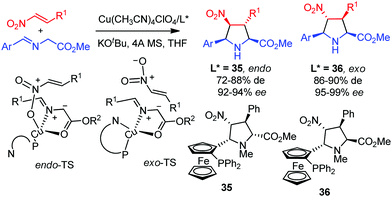 |
| Scheme 16 Cu(I)-Catalyzed diastereodivergent cycloaddition of azomethine ylides and nitroalkenes. | |
In 2015 our research group reported the catalytic asymmetric 1,3-dipolar cycloaddition of azomethine ylides with acyclic electron-poor 1,3-dienes and 1,3-enynes.51 The combination of a CuI salt and a bidentate axial chiral phosphine allowed the control of the regio, diastereo and enantioselectivity of the process. The reaction occurred regioselectively in the γ,δ-double bond of the dipolarophile. The exo isomers were obtained with excellent diastereo and enantioselectivities using CuI/DTBM-Segphos 16 as catalyst and KtBuO as base. A complete reversal of the diastereoselectivity was achieved in the presence of the ligand BTFM-Garphos 37 (Scheme 17).
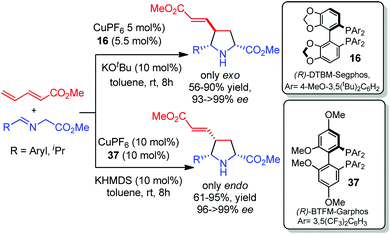 |
| Scheme 17 CuI/DTBM-Segphos catalyzed 1,3-dipolar cycloaddition of azomethine ylides and 1,3-dienes. | |
Shortly after, Wang and co-workers developed an AgI-catalyzed diastereodivergent 1,3-dipolar cycloaddition of azomethine ylides with 3-methyl-4-nitro-5-styrylisoxazoles.52 The reaction using Phosferrox ligand 38 afforded the expected endo adduct with almost complete diastereoselectivity and good enantioselectivity. Interestingly, the monodentate phosphoramidite ligand 7 led selectively to the exo adducts also with high enantioselectivity (Scheme 18).
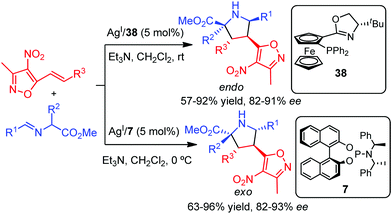 |
| Scheme 18 Diastereodivergent cycloaddition with methyl-4-nitro-5-styrylisoxazoles. | |
In 2016, Zhang and co-workers described the enantioselective preparation of pyrrolidines bearing a quaternary stereocenter at C-3 by a CuI-catalyzed [3+2] cycloaddition of azomethine ylides with β-CF3-β,β-disubstituted enones. The sulfonamide monophosphine (Ming-Phos) ligand 39 afforded the endo pyrrolidines with high diastereoselectivity and enantioselectivity.53 This research group later described the exo selective version of the cycloaddition using (S)-MeO-DTBM-Biphep 34 as the ligand (Scheme 19).54
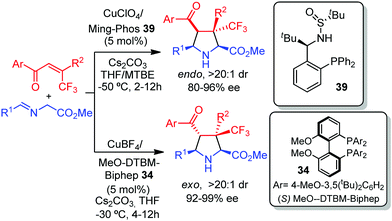 |
| Scheme 19 [3+2] cycloaddition of azomethine ylides with β-CF3-β,β-substituted enones. | |
In 2012 Antonchick and Waldmann55 reported the enantioselective Cu-catalyzed [6+3] cycloaddition of iminoesters with fulvenes. The best results were obtained using the catalyst combination of Cu(CH3CN)4BF4 and Fesulphos ligand 18. Due to the instability of the products a subsequent Diels–Alder reaction with maleimide was performed, affording the endo adducts with high enantioselectivity (Scheme 20).
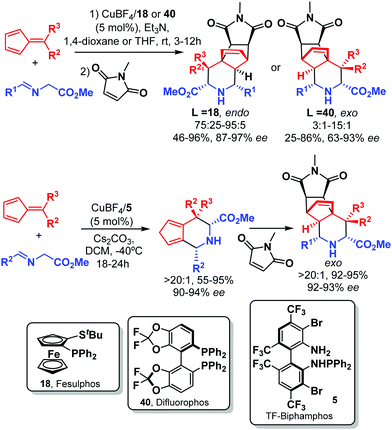 |
| Scheme 20 [6+3] cycloaddition of iminoesters with fulvenes. | |
In a later work the diastereodivergent version, which provided the exo-adducts, was performed using difluorophos ligand 40.56 Almost simultaneously, a similar cycloaddition catalyzed by CuI/TF-Biphamphos 5 was reported.57
Very recently, Xu, Deng and co-workers have described a catalytic asymmetric cycloaddition with four membered rings decorated with an exocyclic double bond.58 The endo or exo spirocyclic pyrrolidines were selectively prepared depending exclusively on the ligand. Thus, the CuI/Phosferrox 38 complex afforded the exo adduct whereas the CuI/N,O-ligand 41 complex gave rise to the endo pyrrolidine, in both cases with excellent diastereo and enantioselectivities (Scheme 21).
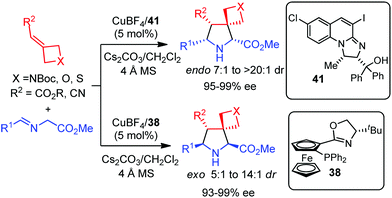 |
| Scheme 21 Diastereodivergent synthesis of spirocyclic pyrrolidines. | |
3.2 Metal-controlled diastereodivergent reactions
In 2009 Martín and co-workers reported the first catalytic asymmetric methodology for obtaining pyrrolidino fullerenes. The 1,3-dipolar cycloaddition of azomethine ylides derived from iminoesters using CuII/Fesulphos 18 or Ag/BPE 42 as catalysts enabled the preparation of cis C60/pyrrolidine adducts in high yield and enantioselectivity.59 The trans adducts were selectively obtained using the combination of Cu(OTf)2 and DTBM-segphos 16.60 This diastereodivergent methodology was also successfully applied to the preparation of C7061 and endohedral H2@C60 derivatives.62 These catalytic systems provided excellent diastereocontrol in the cycloaddition of C60 with varied azomethine ylides including steroid substitution: the catalytic combinations CuII/Fesulphos 18 and AgI/BPE afforded the cis adducts, while the Cu(OTf)2/DTBM-segphos complex provided trans-pyrrolidino fullerenes (Scheme 22).63
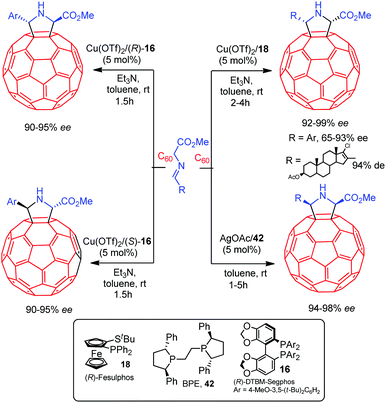 |
| Scheme 22 Fullerenes as dipolarophiles. | |
This diastereodivergent methodology was extended to activated olefins such as acrylates and acrylonitriles. In this case only the formation of pyrrolidines with 2,5-cis configuration was observed. The combination of Cu(OTf)2 and DTBM-segphos 16 afforded the exo adducts, whereas the AgOAc/BPE 42 complex gave rise to the endo pyrrolidines. Excellent diastereo and enantioselectivities were obtained for both diastereomers (Scheme 23).60
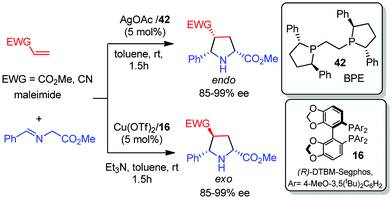 |
| Scheme 23 Diastereodivergent 1,3-dipolar cycloadditions of azomethine ylides with acrylates and acrylonitriles. | |
In 2010, Waldmann and co-workers reported the preparation of 3,3-pyrrolidinyl spirooxoindoles via CuI-catalyzed 1,3-dipolar cycloaddition of azomethine ylides with 3-methylen-2-oxoindoles.64 The combination of Cu(CH3CN)4PF6 and a ferrocenyl ligand 30 afforded the endo cycloadducts with excellent diastereo and enantioselectivities. Shortly after, Wang and co-workers described the silver catalyzed version of this reaction.65 As a stereochemical complementary protocol, Arai, and co-workers published the exo′ selective cycloaddition by using the Ni–imidazoline–aminophenol 29 complex, providing the 2,5-trans configured spirooxoindoles with high enantioselectivity (Scheme 24).66
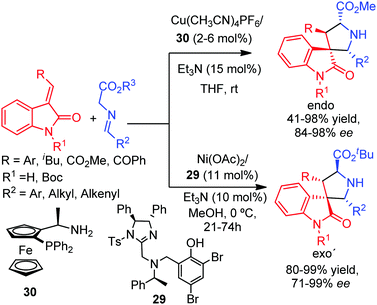 |
| Scheme 24 Synthesis of endo and exo′ 3,3-pyrrolidinyl spirooxoindoles. | |
In 2016, our research group reported the feasibility of vinylarenes as dipolarophiles in catalytic asymmetric [3+2] cycloaddition of azomethine ylides.67 The reaction catalyzed by CuI/DTBM-Segphos 16 led to the formation of exo 4-arylpyrrolidines with high enantioselectivity. Interestingly, the complementary endo adducts were enantioselectively prepared using silver catalysis (AgOAc/Fesulphos 18). The presence of an electron-withdrawing substitution at the aryl group of the alkenyl arene was crucial for the reaction to occur. Vinyl heteroarenes bearing pyridyl, quinolyl or thiazolyl moieties were also suitable dipolarophiles in this cycloaddition. DTF calculations on the reactions of styrene and p-nitrostyrene catalysed by CuI/DBTM-Segphos 16 were performed: the cycloaddition with styrene suggested a concerted pathway with a high activation barrier (25 kcal mol−1), whereas the reaction with p-nitrostyrene was stepwise and with significantly lower activation energy (Scheme 25).
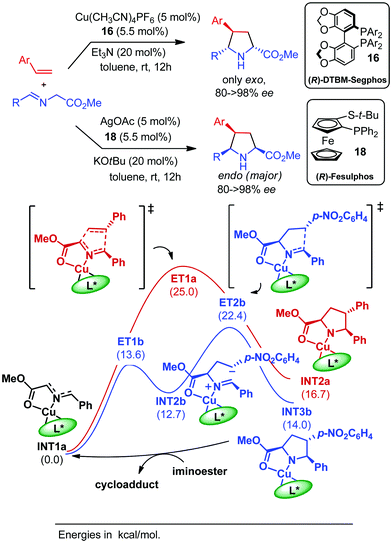 |
| Scheme 25 1,3-Dipolar cycloaddition of azomethine ylides with vinylarenes. | |
3.3 Ligand-controlled enantiodivergent reactions
A ligand controlled enantiodivergent cycloaddition of iminoesters with dimethyl maleate was reported by Li and co-workers in 2007.68 The use of AgOAc and the Ugi amine derived ligand 43 containing a primary amine afforded exclusively the endo cycloadducts with high ee's (Scheme 26). A complete inversion of the enantioselectivity was achieved varying the substitution on the nitrogen atom of the ligand. Thus, using dimethyl amino derived ligand 44 the endo enantiomer was obtained with similar enantioselectivity. DFT calculations were carried out to shed some light on this unusual enantiodivergent process: with ligand 43 the two carbonyl groups of the dipolarophile could form two hydrogen bonds with the NH2 of the ligand, which favours the approach from the top face. However, from ligand 44, having a NMe2 group (which cannot form this hydrogen bonding), the maleate was coordinated to the silver atom and would approach from the less hindered bottom face, leading to the formation of the opposite enantiomer (Scheme 26).
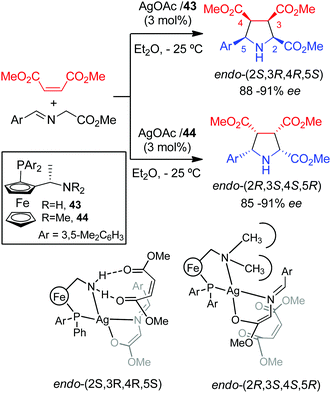 |
| Scheme 26 Ligand-controlled enantiodivergent cycloaddition of iminoesters with dimethyl maleate. | |
3.4 Metal-controlled enantiodivergent reactions
In 2009, Oh and co-workers69 described a metal-controlled enantiodivergent cycloaddition between azomethine ylides and acrylates by using a brucine derived amino alcohol ligand 45 in combination with silver or copper salts. The inversion on the enantioselectivity was based on the different coordination modes of CuI and AgI with the ligand. Both catalytic systems afforded excellent results with a variety of iminoesters, allowing the preparation of pyrrolidines with quaternary stereocentres in C-2 or C-4 positions (Scheme 27). Later, this enantiodivergent methodology was extended to chalcones.70
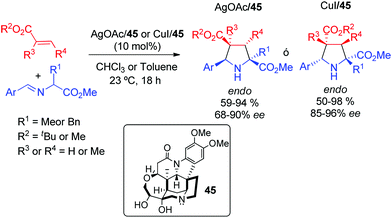 |
| Scheme 27 Metal controlled enantiodivergent 1,3-dipolar cycloaddition of azomethine ylides with acrylates. | |
3.5 Regiodivergent reactions
The usual regioselectivity found in the 1,3-dipolar cycloaddition of iminoesters is controlled by the higher stability of resonance form A where the negative charge was at the α-position of the ester group. The first example of regioreversed catalytic asymmetric [3+2] cycloaddition of iminoesters, involving an umpolung of the azomethine ylide, was developed using organocatalytic approaches.71 Very recently, Liu, Zhang and co-workers72 have developed a CuI-catalyzed asymmetric regiodivergent 1,3-dipolar cycloaddition of iminoesters with β-trifluoromethyl enones (Scheme 28). The regioselectivity of the cycloaddition was controlled by the chiral ligand. DFT calculations revealed that using a Phosferrox ligand 31, the usual catalytic pathway where the P and N atoms of the ligand were coordinated to the copper atom led to the expected regioisomer with excellent diastereo and enantioselectivity. A switch in the regioselectivity was observed with PPFA 46 that acted as a pseudobidentate ligand. The dissociation of the nitrogen from copper and subsequent formation of a Cu–O bond with the carbonyl oxygen atom of the dipolarophile would explain the formation of the opposite regioisomer.
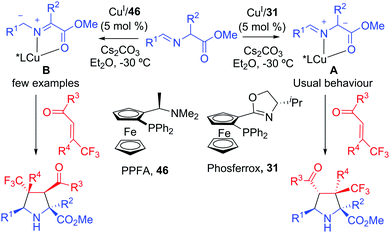 |
| Scheme 28 CuI-Catalyzed regiodivergent 1,3-dipolar cycloaddition of iminoesters with β-trifluoromethyl enones. | |
Very recently, Wang and co-workers73 have reported a very efficient kinetic resolution of alkylidene norcamphors via Cu-catalyzed 1,3-dipolar cycloaddition of azomethine ylides, providing spiro-pyrrolidines with high levels of diastereo and enantioselectivity. The regiochemistry of the reaction could be controlled by the chiral ligand: the BiphamPhos ligand 47 afforded the expected regioisomer while the unconventional regioisomer was effectively obtained using ligand 5 (Scheme 29).
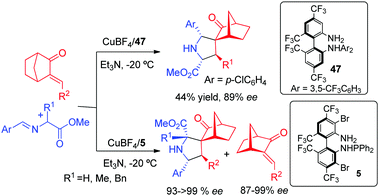 |
| Scheme 29 Cu-Catalyzed 1,3-dipolar cycloaddition of azomethine ylides with alkylidene norcamphors. | |
4. Conclusions
In conclusion this feature article summarizes the great progress achieved in the diastereoselective and enantioselective synthesis of varied stereochemical patterns in substituted pyrrolidines by metal-catalyzed asymmetric 1,3-dipolar cycloaddition of azomethine ylides. Not only have a wide variety of highly enantioselective endo or exo [3+2] cycloadditions been developed, but also access to less common configurations such as 2,5-trans pyrrolidines was achieved (endo′ and exo′ isomers).
Interestingly, significant effort has been recently made to develop stereodivergent versions of this process, providing the enantioselective preparation of two different pyrrolidine stereoisomers from the same reaction partners. In this regard efficient procedures have been recently described using either ligand-controlled or metal controlled cycloadditions. All these advances have substantially improved the state of the art in the enantioselective stereocontrolled access to pyrrolidines on demand.
Conflicts of interest
There are no conflicts to declare.
Acknowledgements
We acknowledge the Spanish Ministerio de Economía, Industria y Competitividad (Grant CTQ2015-66954-P, MINECO/FEDER, UE) and FEDER/Ministerio de Ciencia, Innovación y Universidades – Agencia Estatal de Investigación (Grant PGC2018-098660-B-I00) for financial support.
Notes and references
-
(a) C. Bhat and S. G. Tilve, RSC Adv., 2014, 4, 5405 RSC;
(b) J. P. Michael, Nat. Prod. Rep., 2008, 25, 139 RSC;
(c) J. W. Daly, T. F. Spande and H. M. Garraffo, J. Nat. Prod., 2005, 68, 1556 CrossRef CAS PubMed;
(d) S. G. Pyne, A. S. Davis, N. J. Gates, K. B. Lindsay, T. Machan and M. Tang, Synlett, 2004, 2670 CrossRef CAS;
(e) Y. Cheng, Z.-T. Huang and M.-X. Wang, Curr. Org. Chem., 2004, 8, 325 CrossRef CAS.
-
(a)
T. Mukaiyama and M. Asami, Chiral pyrrolidine diamines as efficient ligands in asymmetric synthesis, in Organic Chemistry Topics in Current Chemistry, Springer, Berlin, Heidelberg, vol. 127, 1985 Search PubMed;
(b) D. W. C. MacMillan, Nature, 2008, 455, 304 CrossRef CAS PubMed;
(c) S. K. Panday, Tetrahedron: Asymmetry, 2011, 22, 1817 CrossRef CAS.
-
(a) M. Kuhnert, A. Blum, H. Steuber and W. E. Diederich, J. Med. Chem., 2015, 58, 4845 CrossRef CAS;
(b) R. D. Taylor, M. MacCoss and A. D. G. Lawson, J. Med. Chem., 2014, 57, 5845 CrossRef CAS PubMed;
(c) S. D. Roughley and A. M. Jordan, J. Med. Chem., 2011, 54, 3451 CrossRef CAS PubMed;
(d) M. Baumann, I. R. Baxendale, S. V. Ley and N. Nikbin, Beilstein J. Org. Chem., 2011, 7, 442 CrossRef CAS PubMed;
(e) P. Garner, P. B. Cox, U. Rathnayake, N. Holloran and P. Erdman, ACS Med. Chem. Lett., 2019, 10, 811 CrossRef CAS PubMed.
- E. Vitaku, D. T. Smith and J. T. Njardarson, J. Med. Chem., 2014, 57, 10257 CrossRef CAS PubMed.
- For reviews, see:
(a) I. Arrastia, A. Arrieta and F. P. Cossio, Eur. J. Org. Chem., 2018, 5889 CrossRef CAS;
(b) X. Fang and C.-J. Wang, Org. Biomol. Chem., 2018, 16, 2591 RSC;
(c) B. Bdiri, B.-J. Zhao and Z.-M. Zhou, Tetrahedron: Asymmetry, 2017, 28, 876 CrossRef CAS;
(d) H. A. Döndas, M. G. Retamosa and J. M. Sansano, Synthesis, 2017, 12434 Search PubMed;
(e) T. Hashimoto and K. Maruoka, Chem. Rev., 2015, 115, 5366 CrossRef CAS PubMed;
(f) J. Adrio and J. C. Carretero, Chem. Commun., 2014, 50, 12434 RSC;
(g) C. Nájera and J. M. Sansano, J. Organomet. Chem., 2014, 771 Search PubMed;
(h) Y. Xing and N.-X. Wang, Coord. Chem. Rev., 2012, 256, 938 CrossRef CAS;
(i) J. Adrio and J. C. Carretero, Chem. Commun., 2011, 47, 67834 RSC;
(j) C. Nájera, J. M. Sansano and M. Yus, J. Braz. Chem. Soc., 2010, 21, 377 CrossRef;
(k) C. Nájera and J. M. Sansano, Top. Heterocycl. Chem., 2008, 12, 117 Search PubMed;
(l) L. M. Stanley and M. P. Sibi, Chem. Rev., 2008, 108, 2887 CrossRef CAS PubMed;
(m) H. Pellisier, Tetrahedron, 2007, 63, 3235 CrossRef;
(n) G. Pandey, P. Banerjee and S. R. Gadre, Chem. Rev., 2006, 106, 4484 CrossRef CAS PubMed;
(o) T. M. V. D. Pinho e Melo, Eur. J. Org. Chem., 2006, 2873 CrossRef CAS;
(p) M. Bonin, A. Chauveau and L. Micouin, Synlett, 2006, 2349 CrossRef CAS;
(q) S. Husinec and V. Savic, Tetrahedron:
Asymmetry, 2005, 16, 2047 CrossRef CAS;
(r) I. Coldham and R. Hufton, Chem. Rev., 2005, 105, 2765 CrossRef CAS PubMed;
(s) C. Nájera and J. M. Sansano, Angew. Chem., Int. Ed., 2005, 44, 6272 CrossRef PubMed.
-
(a) R. Narayan, M. Potowski, Z.-J. Jia, A. P. Antonchick and H. Waldmann, Acc. Chem. Res., 2014, 47, 1292 CrossRef PubMed;
(b) H. Liu, Y. Wu, Y. Zhao, Z. Li, L. Zhang, W. Yang, H. Jiang, C. Jing, H. Yu, B. Wang, Y. Xiao and H. Guo, J. Am. Chem. Soc., 2014, 136, 2625 CrossRef CAS PubMed;
(c) H.-L. Teng, L. Yao and C.-J. Wang, J. Am. Chem. Soc., 2014, 136, 4075 CrossRef CAS PubMed;
(d) H. Guo, H. Liu, F.-L. Zhu, R. Na, H. Jiang, Y. Wu, L. Zhang, Z. Li, H. Yu, B. Wang, Y. Xiao, X.-P. Hu and M. Wang, Angew. Chem., Int. Ed., 2013, 52, 12641 CrossRef CAS PubMed;
(e) M.-C. Tong, X. Chen, H.-Y. Tao and C.-J. Wang, Angew. Chem., Int. Ed., 2013, 52, 12377 CrossRef CAS PubMed.
- For recent reviews, see:
(a) I. P. Beletskaya, C. Nájera and M. Yus, Chem. Rev., 2018, 118, 5080 CrossRef CAS PubMed;
(b) S. Krautwald and E. M. Carreira, J. Am. Chem. Soc., 2017, 129, 5627 CrossRef PubMed;
(c) M. Bihani and J. C.-G. Zhao, Adv. Synth. Catal., 2017, 359, 534 CrossRef CAS.
- J. M. Longmire, B. Wang and X. Zhang, J. Am. Chem. Soc., 2002, 124, 13400 CrossRef CAS PubMed.
- A. S. Gothelf, K. V. Gothelf, R. G. Hazell and K. A. Jørgensen, Angew. Chem., Int. Ed., 2002, 41, 4236 CrossRef CAS PubMed.
-
(a) C. Chen, X. Li and S. L. Schreiber, J. Am. Chem. Soc., 2003, 125, 10174 CrossRef CAS PubMed;
(b) W. Zeng and Y.-G. Zhou, Org. Lett., 2005, 7, 5055 CrossRef CAS PubMed;
(c) W. Zeng and Y.-G. Zhou, Tetrahedron Lett., 2007, 48, 4619 CrossRef CAS;
(d) C. Nájera, M. de Gracia Retamosa and J. M. Sansano, Org. Lett., 2007, 9, 4025 CrossRef PubMed;
(e) C. Nájera, M. de Gracia Retamosa and J. M. Sansano, Angew. Chem., Int. Ed., 2008, 47, 6055 CrossRef PubMed;
(f) S.-B. Yu, X.-P. Hu, J. Deng, D.-Y. Wang, Z.-C. Duan and Z. Zheng, Tetrahedron: Asymmetry, 2009, 20, 621 CrossRef CAS;
(g) C.-J. Wang, Z.-Y. Xue, G. Liang and Z. Lu, Chem. Commun., 2009, 2905 RSC;
(h) X. Zheng, Q. Deng, Q. Hou, K. Zhang, P. Wen, S. Hu and H. Wang, Synthesis, 2018, 2347 CAS;
(i) K. Shimizu, K. Ogata and S.-I. Fukuzawa, Tetrahedron Lett., 2010, 51, 5068 CrossRef CAS;
(j) X.-F. Bai, J. Zhang, C.-G. Xia, J.-X. Xu and L.-W. Xu, Tetrahedron, 2016, 72, 2690 CrossRef CAS;
(k) S. Yan, C. Zhan, Y.-H. Wang, Z. Cao, Z. Zheng and X.-P. Hu, Tetrahedron Lett., 2013, 54, 3669 CrossRef CAS.
- C. Nájera, M. de Gracia Retamosa, M. Martín-Rodríguez, J. M. Sansano, A. de Cózar and F. P. Cossío, Eur. J. Org. Chem., 2009, 5622 CrossRef.
- I. Oura, K. Shimizu, K. Ogata and S.-I. Fukuzawa, Org. Lett., 2010, 12, 1752 CrossRef CAS PubMed.
- C. Zhang, S.-B. Yu, X.-P. Hu, D.-Y. Wang and Z. Zheng, Org. Lett., 2010, 12, 5542 CrossRef CAS PubMed.
- H.-C. Liu, K. Liu, Z.-Y. Xue, Z.-L. He and C.-J. Wang, Org. Lett., 2015, 17, 5440 CrossRef CAS PubMed.
- K. Shimizu, K. Ogata and S.-I. Fukuzawa, Tetrahedron Lett., 2010, 51, 5068 CrossRef CAS.
- A. Cayuelas, R. Ortiz, C. Nájera, J. M. Sansano, O. Larrañaga, A. de Cózar and F. P. Cossío, Org. Lett., 2016, 18, 2926 CrossRef CAS PubMed.
- G. Liang, M.-C. Tong and C.-J. Wang, Adv. Synth. Catal., 2009, 351, 3101 CrossRef CAS.
- M.-C. Tong, J. Li, H.-Y. Tao, Y.-X. Li and C.-J. Wang, Chem. – Eur. J., 2011, 17, 12922 CrossRef CAS PubMed.
- M. Martín-Rodríguez, C. Nájera, J. M. Sansano, P. Costa, E. de Lima and A. Dias, Synlett, 2010, 962 Search PubMed.
- Y. Yamashita, T. Imaizumi and S. Kobayashi, Angew. Chem., Int. Ed., 2011, 50, 4893 CrossRef CAS PubMed.
-
(a) L. M. Castelló, C. Nájera, J. M. Sansano, O. Larrañaga, A. de Cózar and F. P. Cossío, Adv. Synth. Catal., 2014, 356, 3861 CrossRef;
(b) L. M. Castelló, C. Nájera, J. M. Sansano, O. Larrañaga, A. de Cózar and F. P. Cossío, Synthesis, 2015, 934 Search PubMed.
- X.-F. Bai, T. Song, Z. Xu, C.-G. Xia, W.-S. Huang and L.-W. Xu, Angew. Chem., Int. Ed., 2015, 54, 5255 CrossRef CAS PubMed.
- X.-F. Bai, Z. Xu, C.-G. Xia, Z.-J. Zheng and L.-W. Xu, ACS Catal., 2015, 5, 6016 CrossRef CAS.
- Y. Oderaotoshi, W. Cheng, S. Fujitomi, Y. Kasano, S. Minakata and M. Komatsu, Org. Lett., 2003, 5, 5043 CrossRef CAS PubMed.
- W. Gao, X. Zhang and M. Raghunath, Org. Lett., 2005, 7, 4241 CrossRef CAS PubMed.
- S. Cabrera, R. Gómez Arrayás and J. C. Carretero, J. Am. Chem. Soc., 2005, 127, 16394 CrossRef CAS PubMed.
- H. Oki and S.-I. Fukuzawa, Org. Lett., 2008, 10, 1747 CrossRef PubMed.
- C.-J. Whang, G. Liang, Z. Y. Zue and F. Gao, J. Am. Chem. Soc., 2008, 130, 17250 CrossRef PubMed.
- M. Shi and J.-W. Shi, Tetrahedron: Asymmetry, 2007, 18, 645 CrossRef CAS.
- B. Bdiri, L. Dai and Z.-M. Zhou, Tetrahedron Lett., 2017, 58, 2475 CrossRef CAS.
- J. Hernández-Toribio, R. Gómez-Arrayás, B. Martín-Matute and J. C. Carretero, Org. Lett., 2009, 11, 393 CrossRef PubMed.
- J. Corpas, A. Ponce, J. Adrio and J. C. Carretero, Org. Lett., 2018, 20, 3179 CrossRef CAS PubMed.
- T. Arai, A. Mishiro, N. Yokoyama, K. Suzuki and H. Sato, J. Am. Chem. Soc., 2010, 132, 5338 CrossRef CAS PubMed.
- L. M. Castelló, C. Nájera, J. M. Sansano, O. Larrañaga, A. de Cózar and F. P. Cossío, Org. Lett., 2013, 15, 2902 CrossRef PubMed.
-
(a) T. Llamas, R. Gómez Arrayás and J. C. Carretero, Org. Lett., 2006, 8, 1795 CrossRef CAS PubMed;
(b) T. Llamas, R. Gómez Arrayás and J. C. Carretero, Synthesis, 2007, 950 CAS.
- A. López-Pérez, J. Adrio and J. C. Carretero, J. Am. Chem. Soc., 2008, 130, 10084 CrossRef PubMed.
- A. López-Pérez, J. Adrio and J. C. Carretero, Angew. Chem., Int. Ed., 2009, 48, 340 CrossRef PubMed.
- O. Dogan, H. Koyuncu, P. Garner, A. Bulut, W. J. Youngs and M. Panzner, Org. Lett., 2006, 8, 4687 CrossRef CAS PubMed.
- J.-W. Shi, M.-X. Zhao, Z.-Y. Lei and M. Shi, J. Org. Chem., 2008, 73, 305 CrossRef CAS PubMed.
-
(a) S. Susumu, T. Tsubogo and S. Kobayashi, J. Am. Chem. Soc., 2007, 129, 5364 CrossRef PubMed;
(b) T. Tsubogo, S. Saito, K. Seki, Y. Yamashita and S. Kobayashi, J. Am. Chem. Soc., 2008, 130, 13321 CrossRef CAS PubMed;
(c) S. Kobayashi and Y. Yamashita, Acc. Chem. Res., 2011, 44, 58 CrossRef CAS PubMed.
- M. Martín-Rodríguez, C. Nájera, J. M. Sansano, A. de Cózar and F. P. Cossío, Chem. – Eur. J., 2011, 17, 14224 CrossRef PubMed.
- H. Wu, B. Wang, H. Liu and L. Wang, Tetrahedron, 2011, 67, 1210 CrossRef CAS.
- T. Arai, N. Yokoyama, A. Mishiro and H. Sato, Angew. Chem., Int. Ed., 2010, 49, 7895 CrossRef CAS PubMed.
-
(a) S. Vivanco, B. Lecea, A. Arrieta, P. Prieto, I. Morao, A. Linden and F. P. Cossıío, J. Am. Chem. Soc., 2000, 122, 6078 CrossRef CAS;
(b) H. Y. Kim, J.-Y. Li, S. Kim and K. Oh, J. Am. Chem. Soc., 2011, 133, 20750 CrossRef CAS PubMed.
- A. Awata and T. Arai, Angew. Chem., Int. Ed., 2014, 53, 10462 CrossRef CAS PubMed.
- X.-X. Yan, Q. Peng, Y. Zhang, K. Zhang, W. Hong, X.-L. Hou and Y.-D. Wu, Angew. Chem., Int. Ed., 2006, 45, 1979 CrossRef CAS PubMed.
- R. Robles-Machín, M. González-Esguevillas, J. Adrio and J. C. Carretero, J. Org. Chem., 2010, 75, 233 CrossRef PubMed.
- Q.-H. Li, M.-C. Tong, J. Li, H.-Y. Tao and C.-J. Wang, Chem. Commun., 2011, 47, 11110 RSC.
- Q.-H. Li, Z.-Y. Xue, H.-Y. Tao and C.-J. Wang, Tetrahedron Lett., 2012, 53, 3650 CrossRef CAS.
- E. Conde, D. Bello, A. de Cózar, M. Sánchez, M. A. Vázquez and F. P. Cossío, Chem. Sci., 2012, 3, 1486 RSC.
- M. Gozález-Esguevillas, A. Pascual-Escudero, J. Adrio and J. C. Carretero, Chem. – Eur. J., 2015, 21, 4561 CrossRef PubMed.
-
(a) K. Liu, Y. Xiong, Z.-F. Wang, H.-Y. Tao. and C.-J. Wang, Chem. Commun., 2016, 52, 9458 RSC;
(b) For a recent endo diastereoselective example, see: S. Kato, Y. Suzuki, K. Suzuki, R. Haraguchi and S.-I. Fukuzawa, J. Org. Chem., 2018, 83, 13965 CrossRef CAS PubMed.
- Z.-M. Zhang, B. Xu, S. Xu, H.-H. Wu and J. Zhang, Angew. Chem., Int. Ed., 2016, 55, 6324 CrossRef CAS PubMed.
- B. Xu, Z.-M. Zhang, B. Liu, S. Xu, L.-J. Zhou and J. Zhang, Chem. Commun., 2017, 53, 8152 RSC.
- M. Potowski, J. O. Bauer, C. Strohmann, A. P. Antonchick and H. Waldmann, Angew. Chem., Int. Ed., 2012, 51, 9512 CrossRef CAS PubMed.
- M. Potowski, A. P. Antonchick and H. Waldmann, Chem. Commun., 2013, 49, 7800 RSC.
- Z.-L. He, H.-L. Teng and C.-J. Wang, Angew. Chem., Int. Ed., 2013, 52, 2934 CrossRef CAS PubMed.
- H. Deng, R. Jia, W.-L. Yang, X. Yu and W.-P. Deng, Chem. Commun., 2019, 55, 7346 RSC.
- S. Filippone, E. E. Maroto, A. Martín-Domenech, M. Suarez and N. Martín, Nat. Chem., 2009, 1, 578 CrossRef CAS PubMed.
- E. E. Maroto, S. Filippone, A. Martín-Domenech, M. Suarez and N. Martín, J. Am. Chem. Soc., 2012, 134, 12936 CrossRef CAS PubMed.
- E. E. Maroto, A. de Cózar, S. Filippone, A. Martín-Domenech, M. Suarez, F. P. Cossío and N. Martín, Angew. Chem., Int. Ed., 2011, 50, 6060 CrossRef CAS PubMed.
- E. E. Maroto, M. Izquierdo, M. Murata, S. Filippone, K. Komatsu, Y. Murata and N. Martín, Chem. Commun., 2014, 50, 740 RSC.
- M. Suárez, A. Ruiz, L. Almagro, J. Coro, E. E. Maroto, S. Filippone, D. Molero, R. Martínez-Ávarez and N. Martín, J. Org. Chem., 2017, 82, 4654 CrossRef PubMed.
- A. P. Antonchick, C. Gerding-Reimers, M. Catarinella, M. Schürmann, H. Preut, S. Ziegler, D. Rauh and H. Waldmann, Nat. Chem., 2010, 2, 735 CrossRef CAS PubMed.
- T.-L. Liu, Z.-Y. Xue, H.-Y. Tao and C.-J. Wang, Org. Biomol. Chem., 2011, 9, 1980 RSC.
- A. Awata and T. Arai, Chem. – Eur. J., 2012, 12, 8278 CrossRef PubMed.
- A. Pascual-Escudero, A. de Cózar, F. P. Cossío, J. Adrio and J. C. Carretero, Angew. Chem., Int. Ed., 2016, 55, 15334 CrossRef CAS PubMed.
- W. Zeng, G. Chen, Y.-G. Zhou and Y. X. Li, J. Am. Chem. Soc., 2007, 129, 750 CrossRef CAS PubMed.
-
(a) H. Y. Kim and K. Oh, Synlett, 2015, 2067 CAS;
(b) H. Y. Kim, H.-J. Shih, W. E. Knabe and K. Oh, Angew. Chem., Int. Ed., 2009, 48, 7420 CrossRef CAS PubMed.
- J.-Y. Li, H. Y. Kim and K. Oh, Adv. Synth. Catal., 2016, 358, 984 CrossRef CAS.
- X.-H. Chen, Q. Wei, S.-W. Luo, H. Xiao and L.-Z. Gong, J. Am. Chem. Soc., 2009, 131, 13819 CrossRef CAS PubMed.
- S. Xu, Z. M. Zhang, B. Xu, B. Liu, Y. Liu and J. Zhang, J. Am. Chem. Soc., 2018, 140, 2272 CrossRef CAS PubMed.
- C. Shen, Y. Yang, L. Wei, W.-W. Dong, L. W. Chung and C.-J. Wang, Science, 2019, 11, 146 CAS.
|
This journal is © The Royal Society of Chemistry 2019 |
Click here to see how this site uses Cookies. View our privacy policy here.