DOI:
10.1039/C4QO00330F
(Research Article)
Org. Chem. Front., 2015,
2, 497-501
New building blocks for iminosugars: a concise synthesis of polyhydroxylated N-alkoxypiperidines through an intramolecular azepine ring contraction†
Received
16th December 2014
, Accepted 12th March 2015
First published on 13th March 2015
Abstract
Polyhydroxylated piperidines are a functionally rich class of biologically active molecules that have broad therapeutic potential. Recently developed aza-[4 + 3] cycloadditions of putative aza-oxyallylic cations provide heterocyclic scaffolds that enabled a concise synthesis of polyhydoxylated piperidines. Chemoselective amide reduction and reductive hemiaminal ring opening was achieved in one pot by the action of aluminium hydride generated in situ via aluminium chloride and lithium aluminium hydride. Aziridinium ion mediated ring contraction and chloride displacement was triggered by silver acetate, followed by simple acetate hydrolysis using potassium carbonate to give four tetrahydropyridine diols. Olefin oxidation by osmium tetroxide installed the final hydroxyl groups, which yielded four novel polyhydroxylated N-alkoxypiperidines in good overall yield and high diastereoselectivity.
Introduction
Iminosugars are a class of small organic compounds that mimic carbohydrates in their general structure as well as their hydrolysis transition states.1 As small polar molecules they resemble carbohydrates enough to allow efficient uptake by the body, while avoiding metabolism by carbohydrate processing enzymes.2 This “dual personality” gives iminosugars the potential to serve as a special class of molecules in the on-going search for new drug candidates.3 The concise stereoselective synthesis of these molecules has received considerable attention from researchers due to their glycosidase inhibitory properties, which give them therapeutic applications for the treatment of diseases such as HIV, cancer, and diabetes.4,5 Miglitol 1 (Fig. 1) is an FDA approved anti-diabetic drug that functions by inhibiting the body's ability to hydrolyze carbohydrates into glucose, and has more recently been shown to reduce plasma lipids as well as inhibit free radical generation.6 Deoxynojirimycin (DNJ) 2 is an archetypal aza sugar and analogue of D-glucose. DNJ itself is an alpha-glucosidase inhibitor with antiviral activity, while derivatives of DNJ show promising anti-HIV activity.4 Miglustat (marketed under the trade name Zavesca) 3 is an inhibitor of glucosylceramide synthase, and is used to treat adults with mild to moderate Type I Gaucher disease.7 Recently, iminosugar derivatives functionalized through a hydroxylamine N–O bond have been an attractive synthetic target due to the fact that the barrier to inversion at the nitrogen atom of trialkylhydroxylamines is higher than simple amines.8 However, at approximately 15 kcal mol−1 this barrier is not sufficient to prevent rapid inversion at room temperature.8 Ideally with this low barrier to inversion, it is anticipated that any iminosugar derivative possessing a hydroxylamine motif could sample the full extent of conformational space available at room temperature and adapt in order to bind to enzymes specific for either axial or equatorially linked substrates.9 Therefore, we viewed this as a motive for developing a general and concise synthesis of N-alkoxy iminosugar analogs that had the potential for incorporating a wide variety of alkyl side chains on the nitrogen terminus.
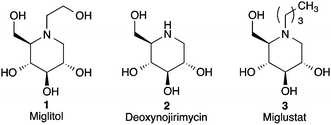 |
| Fig. 1 Representative examples of biologically and pharmaceutically relevant iminosugars. | |
Recently, we have reported a new aza-[4 + 3] cycloaddition reaction that proceeds through an aza-oxyallylic cation and allows for the rapid construction of 7-membered nitrogen heterocycles from commercially available starting materials (Scheme 1).10,11 We recently found that α-chloroazepane 4 (Scheme 2) underwent facile ring contraction when treated with a nucleophile to give tetrahydropyridine 6. Given the exceptional electron rich character of the nitrogen atom, a plausible mechanism would involve the ring contraction going through an aziridinium ion intermediate such as 5 and ultimately collapsing to the six-membered ring.12 It was this discovery which led us to believe that simple functional group manipulations could provide access to the iminosugar class of compounds in a way that was not only stereoselective and concise, but that also was scalable. Moreover, we envisioned that the stereochemical flexibility of our approach would enable the diversity-oriented approach for the synthesis of a library of compounds for biological screening as well as structure activity relationship (SAR) studies. We envisioned an α-chlorocycloadduct formed from the aza-[4 + 3] cycloaddition of an aza-oxyallylic cation as a general and concise method to stereoselectively construct the cyclic core of polyhydroxylated N-alkoxypiperidines (Scheme 3).
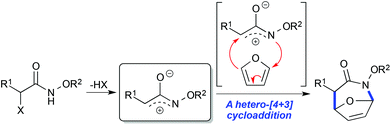 |
| Scheme 1 An aza-[4 + 3] cycloaddition of a stabilized aza-oxyallylic cation with furan to form a 7-membered azacycle. | |
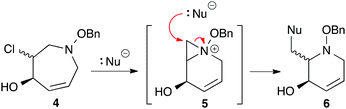 |
| Scheme 2 Aziridinium ion mediated ring contraction of reduced α-chlorocycloadduct to give an N-benzyloxy tetrahydropyridine. | |
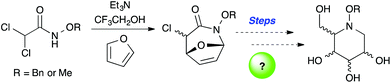 |
| Scheme 3 Proposal to synthesize polyhydroxylated N-alkoxypiperidines from general aza-[4 + 3] cycloadduct skeletons. | |
Additionally, we thought that the rich functionality our method afforded could provide the necessary handles needed for functional group manipulations in order to elaborate these cores to the desired targets.13 Herein, we wish to report a general strategy for the preparation of polyhydroxylated N-alkoxypiperidines that is concise, scalable, diastereoselective, and versatile to allow for the construction of a library of derivatives for both biological activity and SAR studies.
Results and discussion
Initial studies began by reacting dichloroamides 7 and 10 with furan to give α-chlorocycloadducts 8 and 9 in 79% yield; and 11 and 12 in 80% yield respectively and with a diastereoisomeric ratio of 2
:
1 endo
:
exo (Scheme 4). We observed the diastereoisomeric ratio of this reaction to be high at early conversion (≥19
:
1 at ca. 40% conversion), however epimerization of the endo cycloadduct resulted in the origin of the final 2
:
1 ratio at complete conversion.10 It is worth mentioning that even though the diastereoselectivity of these reactions was rather poor, both diastereoisomers in each case were considered useful and taken on and elaborated to the desired targets. Our original goal was to perform a one-pot reduction on the bicyclic caprolactams that would reduce the amide as well as trigger hemiaminal ether ring opening, all while leaving the halogen unaltered to give the pre-functionalized azepine. After extensive screening of reduction conditions, aluminium hydride (generated in situ from the addition of lithium aluminium hydride to a solution of aluminium chloride) proved to be the optimal reagent for affecting this desired transformation. Slow addition of cycloadducts 8, 9, 11, and 12 to solutions of aluminium hydride at 0 °C followed by refluxing for 90 minutes cleanly gave the desired azepine products 13–16 in good yield as single diastereoisomers (Scheme 5). The relative configuration of 14 was unambiguously confirmed by single crystal X-ray analysis (Fig. 2). With the desired azepines in hand, the stage was set for the pivotal ring contraction and installation of an oxygen functionality from nucleophilic displacement of chloride. Attempts to trigger ring contraction and chloride displacement with various oxygen centred nucleophiles including alkoxides and hydroxide under a variety of conditions proved to be ineffective and failed to give the desired product. However, heating of 13–16 in a DMF–silver acetate suspension was found to successfully affect the ring contraction and install the necessary oxygen functionality, giving 17–20 (Table 1, entries 1–4) respectively as single diastereoisomers in moderate yields. To the best of our knowledge, this reaction represents the first report of an intramolecular azepine ring contraction whereby the substrate is pre-functionalized at the 2-position with a leaving group. A similar azepine ring contraction example was reported in the literature by Davies and co-workers, albeit their substrate was not pre-functionalized with an appended leaving group.12 In this respect, we have developed a novel methodology for the rapid and stereoselective construction of tetrahydropyridine cores in only a few synthetic steps from commercially available starting materials. Additionally, the resulting skeletons are richly functionalized and could be envisioned as versatile building blocks for the construction of other iminosugar derivatives or piperidine natural products of interest (Table 1).
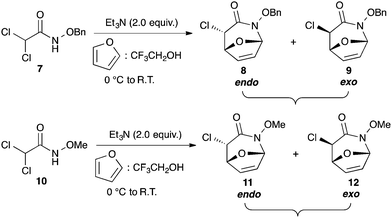 |
| Scheme 4 Reaction of dichloroamide starting materials with furan to give endo and exo α-chloro cycloadducts. | |
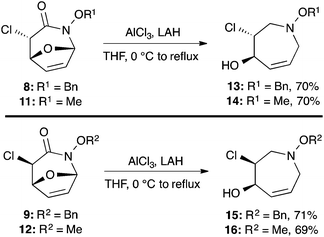 |
| Scheme 5 Aluminum hydride mediated one pot reductions to give pre-functionalized azepines 13–16. | |
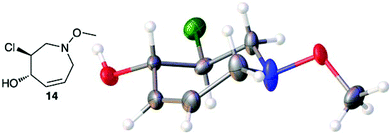 |
| Fig. 2 Thermal ellipsoid plot of 14 at 50% probability. Hydrogen atoms are represented as spheres of arbitrary radius. Grey = carbon, red = oxygen, blue = nitrogen, green = chlorine. | |
Table 1 Silver acetate mediated ring contraction products 17–20 and acetate hydrolysis products 21–24
Acetate hydrolysis using potassium carbonate in methanol14 produced diols 21–24 (Table 1) in high yields, with 21 being of particular interest due to its high crystallinity and potential for X-ray analysis. Indeed, slow evaporation of benzene from 21 afforded crystals of suitable quality for X-ray diffraction studies (Fig. 3). We found the observed stereochemistry of diol 21 intriguing, and suggests a double inversion-type mechanism, whereby a Lewis acid catalyzed abstraction of chloride by silver would lead to backside nucleophilic attack by ring nitrogen to form the aziridinium ion intermediate 25 (Scheme 6). Subsequent nucleophilic attack by acetate onto the aziridinium ion 25 would provide tetrahydropyridine 17. With the desired diols in hand, the last step to complete the syntheses would involve utilization of the alkene to install the remaining alcohol groups. Dihydroxylation of the alkene using catalytic osmium tetroxide was found to be a simple and high-yielding method for installing the remaining hydroxyl groups. Exposure of the substrates 21–24 to a solution of osmium tetroxide in water and NMO as the co-oxidant provided the final polyhydroxylated products 25–28 in good yields and as single diastereoisomers (Scheme 7). Upon analysis of 25 by single crystal X-ray diffraction, the oxidation was determined to occur selectively from the opposite face of the allylic carbinol group resulting in the trans-configuration relative to the C-4 alcohol group in all cases and is consistent with coupling constant analysis (Fig. 4) (see ESI†).15 These scaffolds represent 4 novel iminosugar derivatives with unique stereochemical configurations that are scarcely found in the literature (Scheme 7).16
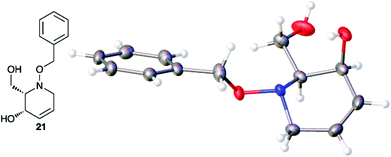 |
| Fig. 3 Thermal ellipsoid plot of tetrahydropyridine 21 at 50% probability showing relative cis stereochemistry configuration. The crystal structure is centrosymmetric but only one enantiomer is shown. Hydrogen atoms are represented as spheres of arbitrary radius. Grey = carbon, red = oxygen, blue = nitrogen. | |
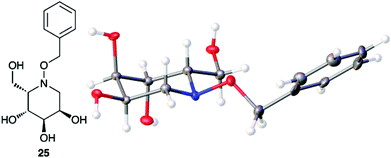 |
| Fig. 4 Thermal ellipsoid plot of polyhydroxylated piperidine 25 at 50% probability. The crystal structure is centrosymmetric but only one enantiomer is shown. Hydrogen atoms are represented as spheres of arbitrary radius. Grey = carbon, red = oxygen, blue = nitrogen. | |
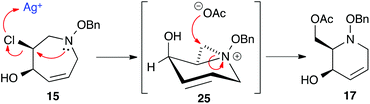 |
| Scheme 6 Mechanistic proposal of aziridinium ion mediated ring contraction of 15 to 17. | |
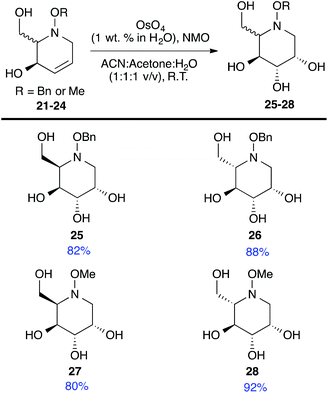 |
| Scheme 7 Osmium tetroxide-mediated olefin oxidation to install final hydroxyl groups and give iminosugar derivatives 25–28. | |
Conclusions
In conclusion, we have developed a concise approach to the stereoselective synthesis of polyhydroxylated N-alkoxypiperidines from common 7-membered azacyclic cores. This strategy hinged on the rich functionality that is provided from the aza-[4 + 3] cycloaddition reactions of putative aza-oxyallylic cation intermediates with furan. A chemoselective double reduction using alane provided the prefunctionalized azepines. Silver acetate promoted ring contraction and subsequent acetate hydrolysis with potassium carbonate provided a novel method for the construction of tetrahydropyridine cores in good yield and high diastereoselectivity. Finally, stereoselective catalytic dihydroxylation by osmium tetroxide 21–24 gave the final polyhydroxylated products 25–28 in high yields. This method represents a versatile approach to tetrahydropyridine cores and iminosugar derivatives that is only 5 steps from furan and 1,1-dichloroacetyl chloride. Current efforts to better understand the ring contraction mechanism and elaborate the tetrahydropyridine scaffolds to other piperidine natural products of interest are underway.
Crystal structure determination of complexes 14, 21, and 25
Crystal data for 14 (CCDC no. 1028235)
C7H7ClNO2, M = 172.59, monoclinic, a = 24.849(3), b = 4.7231(5), c = 14.8643(17) Å, U = 1696.6(3) Å3, T = 100.15 K, space group C2/c (no. 15), Z = 8, 12
847 reflections measured, 1736 unique (Rint = 0.0616) which were used in all calculations. The final wR(F2) was 0.1969 (all data).
Crystal data for 21 (CCDC no. 1028236)
C26H34N2O6, M = 470.55, triclinic, a = 9.9549(4), b = 11.3328(5), c = 11.8818(5) Å, U = 1219.60(9) Å3, T = 99.65 K, space group P
(no. 2), Z = 2, 23
662 reflections measured, 5015 unique (Rint = 0.0515) which were used in all calculations. The final wR(F2) was 0.0727 (all data).
Crystal data for 25 (CCDC no. 1028237)
C26H38N2O10, M = 538.58, triclinic, a = 5.3906(3), b = 15.9299(9), c = 17.3564(10) Å, U = 1326.85(13) Å3, T = 99.65 K, space group P
(no. 2), Z = 2, 33
786 reflections measured, 8134 unique (Rint = 0.0579) which were used in all calculations. The final wR(F2) was 0.1352 (all data).
Acknowledgements
This work was supported through an ACS-PRF grant (51442-DNI1) and through start-up funding from the University of Nevada, Reno.
Notes and references
-
Iminosugars: From Synthesis to Therapeutic Applications, ed. P. Compain and O. R. Martin, John Wiley and Sons ltd, 2007 Search PubMed.
- H. R. Mellor, D. C. A. Neville, D. J. Harvey, F. M. Platt, R. A. Dwek and T. D. Butters, Biochem. J., 2004, 381, 861 CrossRef CAS PubMed.
- D. D'Alonzo, A. Guaragna and G. Palumbo, Curr. Med. Chem., 2009, 16, 473 CrossRef.
- B. G. Davis, Tetrahedron: Asymmetry, 2009, 20, 652 CrossRef CAS PubMed.
- B. G. Winchester, Tetrahedron: Asymmetry, 2009, 20, 645 CrossRef CAS PubMed.
- A. Shrivastava, U. Chaturvedi, S. V. Singh, J. K. Saxena and G. Bhatia, Lipids, 2013, 48, 597 CrossRef CAS PubMed.
-
(a) P. L. van Giersbergen and J. Dingemanse, J. Clin. Pharmacol., 2007, 47, 1277 CrossRef CAS PubMed;
(b) T. Cox, J. Inherited Metab. Dis., 2003, 26, 513 CrossRef CAS;
(c) E. H. Kolodny, O. Neudorfer, J. Gianutsos, C. Zaroff, N. Barnett, B. J. Zeng, S. Raghavan, P. Torres and G. M. Pastores, J. Neurochem., 2004, 90, 54 CrossRef PubMed.
-
(a) F. G. Riddell, E. S. Turner and A. Boyd, Tetrahedron, 1979, 35, 259 CrossRef CAS;
(b) A. Hassan, M. I. M. Wazeer, H. P. Perzanowski and S. A. Ali, J. Chem. Soc., Perkin Trans. 2, 1997, 411 RSC;
(c) M. Raban and D. Kost, Tetrahedron, 1984, 40, 3345 CrossRef CAS.
- G. Malik, A. Ferry, X. Guinchard, T. Cresteil and D. Crich, Chem. – Eur. J., 2013, 19, 2168 CrossRef CAS PubMed.
- C. S. Jeffrey, K. L. Barnes, J. A. Eickhoff and C. R. Carson, J. Am. Chem. Soc., 2011, 133, 7688 CrossRef CAS PubMed.
- K. L. Barnes, A. K. Koster and C. S. Jeffrey, Tetrahedron Lett., 2014, 55, 4690 CrossRef CAS PubMed.
- S. K. Bagal, S. G. Davies, J. A. Lee, P. M. Roberts, A. J. Russell, P. M. Scott and J. E. Thomson, Org. Lett., 2010, 12, 136 CrossRef CAS PubMed.
- For other examples of using furans in the synthesis of iminosugars see:
(a) M. A. Ciufolini, C. Y. W. Hermann, Q. Dong, T. Shimizu, S. Swaminathan and N. Xi, Synlett, 1998, 105 CrossRef CAS PubMed;
(b) N. Xi and M. A. Ciufolini, Tetrahedron Lett., 1995, 36, 6595 CrossRef CAS;
(c) C. F. Yang, Y. M. Xu, L. Liao and W. S. Zhou, Tetrahedron Lett., 1998, 39, 9227 CrossRef CAS;
(d) M. H. Haukaas and G. A. O'Doherty, Org. Lett., 2001, 3, 401 CrossRef CAS;
(e) M. L. Bushey, M. H. Haukaas and G. A. O'Doherty, J. Org. Chem., 1999, 64, 2984 CrossRef CAS;
(f) H. H. Guo and G. A. O'Doherty, Org. Lett., 2006, 8, 1609 CrossRef CAS PubMed;
(g) J. N. Abrams, R. S. Babu, H. Guo, D. Le, J. Le, J. M. Osbourn and G. A. O'Doherty, J. Org. Chem., 2008, 73, 1935 CrossRef CAS PubMed;
(h) H. Guo and G. A. O'Doherty, Tetrahedron, 2008, 64, 304 CrossRef CAS PubMed.
- S. K. Baga, S. G. Davies, J. A. Lee, P. M. Roberts, P. M. Scott and J. E. Thomson, J. Org. Chem., 2010, 75, 8133 CrossRef PubMed.
- For a similar osmium tetroxide-mediated diastereoselective dihydroxylation of a tetrahydropyridine ring see: M. H. Haukaas and G. A. O'Doherty, Org. Lett., 2001, 3, 401 CrossRef CAS.
- For examples see:
(a) E. W. Baxter and A. B. Reitz, Bioorg. Med. Chem. Lett., 1992, 2, 1419 CrossRef CAS;
(b) E. W. Baxter and A. B. Reitz, J. Org. Chem., 1994, 59, 3175 CrossRef CAS;
(c) C. R. Johnson, A. Golebiowski, E. Schoffers, H. Sundram and M. P. Braun, Synlett, 1994, 313 CAS.
Footnote |
† Electronic supplementary information (ESI) available. CCDC 1028235–1028237. For ESI and crystallographic data in CIF or other electronic format see DOI: 10.1039/c4qo00330f |
|
This journal is © the Partner Organisations 2015 |
Click here to see how this site uses Cookies. View our privacy policy here.