DOI:
10.1039/C5QO00003C
(Research Article)
Org. Chem. Front., 2015,
2, 502-505
Pd-catalyzed cross-coupling of aromatic compounds with carboxylic acids via C–H bond activation†
Received
2nd January 2015
, Accepted 21st February 2015
First published on 2nd March 2015
Abstract
An efficient synthesis for an oxidative coupling of aromatic compounds with carboxylic acids via Pd-catalyzed C–H bond activation and C–O bond formation has been developed. This catalytic system works in a simple and efficient manner, by simply using aromatic compounds and carboxylic acids as coupling partners for C–O bond formation to synthesize esters with good to excellent yields.
In the past few decades, transition-metal-catalyzed functionalization of the C–H bond is emerging as a versatile strategy for chemical synthesis.1 A great number of novel organic reactions via C–H cleavage have been developed, and a variety of chemical bonds can be formed through this efficient manner, including carbon–carbon2 and carbon–heteroatom3–8 bond formations. Among them, C–O bond formation is quite challenging and is less reported in the literature. This is probably due to high electronegativity of the element and may also be due to the metal–oxygen bond strength. To achieve the purpose for coupling an acid with arenes, Crabtree and co-workers9 developed a strategy that employed iodosobenzenediacetate as the carboxylate source. Since then, a variety of C–H acetoxylation reactions have been developed. Concurrently, peroxide has also proven to be an effective promoter in Pd(II)-catalyzed C–H acetoxylation.10 It is noted that direct acyloxylation of C–H bonds was realized with the assistance of directing groups recently,11 while these reactions are limited to acetoxylation.
Recently, Yu12 and Shi13 first reported a Pd-catalyzed cyclization approach to form the benzofuranone through the C–H activation via an intramolecular C–O formation sequence. However, report on catalyzation of intermolecular formation of ester by the coupling of aromatic compounds with a variety of acids via C–H bond activation has not been well developed.14 Herein, we report a successful example of a straightforward and versatile method to obtain a variety of ester structures through palladium catalysed intermolecular oxidative coupling of simple aromatic compounds with readily available carboxylic acids.
Conditions were set up to facilitate high-yielding and direct coupling of aromatic compounds with carboxylic acids using Pd(OAc)2 as the catalyst. Key development of this reaction involved using (1) commercially available iodosobenzene and carboxylic acids and (2) iodosobenzene as an oxidant while the reaction was conducted under mild conditions in order to form the active coupling partner in situ. In these reactions, two general sets of conditions, A and B, were identified to the substrates of the arenes. Condition A was meant for simple and cheap benzene substrates where benzene was used as a solvent. Optimization was obtained when benzene 1a reacted with benzoic acid 2a (Table 1, entries 1–10). The reaction gave phenyl benzoate 3a in 82% yield as the main product by employing Pd(OAc)2 as the catalyst and iodosobenzene as the oxidant (Table 1, entry 6). However, when iodosobenzene diacetate was used instead of iodosobenzene, the product yield decreased to 42% (Table 1, entry 5). Utilization of other oxidants, such as Na2S2O8, oxone, Ag2O, and Cu(OAc)2 did not favor the reaction (Table 1, entries 1–4), neither did other solvents yield a better result (Table 1, entries 7–10). In contrast, condition B was established for heavily functionalized or valuable arenes in which dichloroethane (DCE) was used as the solvent. Optimization was also obtained when 2-bromoanisole 1b reacted with benzoic acid 2a (Table 1, entries 11–18). 3-Bromo-4-methoxyphenyl benzoate 3m was obtained in 88% of the yield in DCE at 120 °C when iodosobenzene and camphorsulfonic acid (CSA) were used as an oxidant and additive, respectively (Table 1, entry 14). Other additives such as p-toluenesulfonic acid (TsOH), trifluoroacetic acid (TFA), and trifluoromethanesulfonic acid (TfOH) did not improve the reaction (Table 1, entries 16–18). The screening of solvents showed that DCE was the most effective solvent for this reaction as compared to chloroform, dimethylformamide, dimethylsulfoxide and acetonitrile (Table 1, entries 11–13 and 15).
Table 1 Optimization of reaction conditions
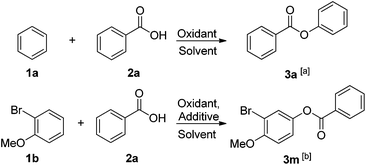
|
Entry |
Solvent |
Oxidant |
Product |
Yieldc |
Condition A: benzoic acid 2a (0.5 mmol), Pd(OAc)2 (5 mol%), oxidant (2.0 mmol), solvent (2 mL), 120 °C, 40 h.
Condition B: 2-bromoanisole 1b (1.0 mmol), benzoic acid 2a (0.5 mmol), Pd(OAc)2 (5 mol%), iodosobenzene (1.5 mmol), additive (1.5 mmol), solvent (2 mL), 120 °C, 40 h.
Isolated yields.
|
1a |
Benzene |
Na2S2O8 |
3a
|
Trace |
2a |
Benzene |
Oxone |
3a
|
NR |
3a |
Benzene |
Ag2O |
3a
|
NR |
4a |
Benzene |
Cu(OAc)2 |
3a
|
NR |
5a |
Benzene |
PhI(OAc)2 |
3a
|
42 |
6a |
Benzene |
PhIO |
3a
|
82 |
7a |
ACN |
PhIO |
3a
|
NR |
8a |
DCE |
PhIO |
3a
|
Trace |
9a |
DMF |
PhIO |
3a
|
NR |
10a |
1,4-Dioxane |
PhIO |
3a
|
NR |
11b |
ACN |
PhIO, CSA |
3m
|
Trace |
12b |
DMF |
PhIO, CSA |
3m
|
NR |
13b |
CHCl3 |
PhIO, CSA |
3m
|
78 |
14b |
DCE |
PhIO, CSA |
3m
|
88 |
15b |
DMSO |
PhIO, CSA |
3m
|
NR |
16b |
DCE |
PhIO, TsOH·H2O |
3m
|
30 |
17b |
DCE |
PhIO, TFA |
3m
|
Trace |
18b |
DCE |
PhIO, TfOH |
3m
|
Trace |
Under the optimized conditions, the substrate scope was examined as shown in Table 2. A variety of carboxylic acid reagents were used and they reacted with various aromatic compounds to afford the corresponding esters in moderate to good yields (3a–3t). Electron-donating, electron-withdrawing, and potentially sensitive functional groups including fluoro (3c), chloro (3b, 3n), bromo (3d, 3m), iodo (3o), nitro (3h), methyl (3i) and methoxy (3j, 3m–q) groups as well as heterocycles, such as pyridyl (3e–g) and indolyl groups (3l, 3s) were tolerated. The results in Table 2 revealed that this procedure exhibited electronic dependence. Electron-deficient carboxylic acids exhibited higher reactivity than those of electron-rich substrates. Carboxylic acids substituted with electron-withdrawing groups, such as chloro (3b), fluoro (3c), bromo (3d) and nitro (3h) were converted into the desired products smoothly in good to excellent yields, whereas only moderate yields were obtained for electron rich carboxylic acids, e.g. o-toluic acid (3i), anisic acid (3j) and N-methyl-3-indolecarboxylic acid (3l). Furthermore, no desired product was observed when employing 2,4,6-trimethoxybenzoic acid (3k) as the substrate. The substituents on the aromatic substrates also influenced the efficiency of the coupling reaction significantly. It was noted that only electron rich aromatic compounds can be employed for our procedure. In addition, aliphatic carboxylic acid can also be subjected to the reaction, with product (3t) obtained in good yield when phenyl-acetic acid was employed as the substrate. The present catalytic systems are also applicable to the chelation assisted C–H bond functionalization reaction. Under the optimized condition B, product (3u) was obtained in 81% yield when 2-phenylpyridine and benzoic acid were used as the coupling partners in the absence of additive CSA (Scheme 1).
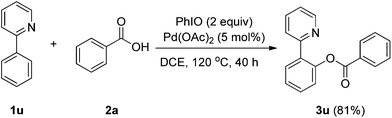 |
| Scheme 1 Chelation assisted C–H bond functionalization. | |
Table 2 Palladium catalyzed direct coupling of aromatic compounds 1 with carboxylic acids 2
Condition A: carboxylic acid 2 (0.5 mmol), Pd(OAc)2 (5 mol%), iodosobenzene (1.0 mmol), benzene 1a (2 mL), 120 °C, 40 h.
Condition B: aromatic compound 1 (1.0 mmol), benzoic acid 2a (0.5 mmol), Pd(OAc)2 (5 mol%), iodosobenzene (1.0 mmol), camphorsulfonic acid (0.75 mmol), DCE (2 mL), 120 °C, 40 h.
Isolated yields.
|
|
To investigate the mechanism, competitive reaction was carried out using a 1
:
1 ratio of benzene and benzene-d6. The KIE was determined to be kH/kD = 8.5/1, indicating that the C–H bond cleavage process should be the rate-determining step (Scheme 2).
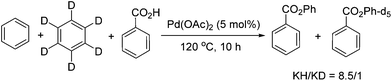 |
| Scheme 2 KIE competitive reaction. | |
A mechanism for the C–O bond formation reaction could involve Pd(II)-catalyzed C–H cleavage of the C–H bond of arene to form an Ar–Pd(II) intermediate I (Scheme 3). Intermediate II was formed by a ligand exchange process of intermediate I with carboxylic acid, followed by C–O reductive elimination to give out the ester product and Pd(0) species. Oxidation of Pd(0) to Pd(II) by iodosobenzene in the presence of acid to complete the catalytic cycle.
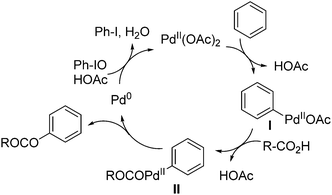 |
| Scheme 3 Plausible mechanism. | |
Conclusions
In summary, we have developed a versatile strategy for C–O bond formation by coupling aromatic compounds with carboxylic acids via Pd-catalyzed C–H bond activation. The present method not only serves as a practical, versatile, and atom-economical alternative to existing synthetic methods, but it also allows facile construction of ester skeletons that have not been easily accessible. Further synthetic exploration of the Pd-catalyzed C–H bond activation and C–O bond formation reaction is currently underway.
Experimental section
General procedure for condition A
Carboxylic acid (0.5 mmol), iodosobenzene (1 mmol), Pd(OAc)2 (5 mol%) and 2 mL benzene were added into the Schlenk tube. The mixture was stirred at 120 °C for 40 h and cooled down to room temperature, quenched with 50 mL saturated sodium bicarbonate solution and extracted thrice with ethyl acetate (30 mL) and the combined organic phase was dried over Na2SO4. After evaporation of the solvents the residue was purified by silica gel chromatography or thin layer chromatography (TLC).
General procedure for condition B
Benzoic acid (0.5 mmol), aromatic compound (1 mmol), iodosobenzene (1 mmol) and CSA (0.75 mmol) in 2 mL DCE were added into the Schlenk tube. The mixture was heated at 120 °C for 40 h and cooled down to room temperature, quenched with 50 mL saturated sodium bicarbonate solution and extracted thrice with ethyl acetate (30 mL) and the combined organic phase was dried over Na2SO4. After evaporation of the solvents the residue was purified by silica gel chromatography or thin layer chromatography (TLC).
Acknowledgements
We gratefully acknowledge the support by Nanyang Technological University (RG6/13), Singapore for financial support.
Notes and references
-
(a) T. W. Lyons and M. S. Sanford, Chem. Rev., 2010, 110, 1147 CrossRef CAS PubMed;
(b) R. Giri, B.-F. Shi, K. M. Engle, N. Maugel and J.-Q. Yu, Chem. Soc. Rev., 2009, 38, 3242 RSC;
(c) D. A. Colby, R. G. Bergman and J. A. Ellman, Chem. Rev., 2010, 110, 624 CrossRef CAS PubMed;
(d) D. Alberico, M. E. Scott and M. Lautens, Chem. Rev., 2007, 107, 174 CrossRef CAS PubMed;
(e) C. S. Yeung and V. M. Dong, Chem. Rev., 2011, 111, 1215 CrossRef CAS PubMed;
(f) C.-L. Sun, B.-J. Li and Z.-J. Shi, Chem. Rev., 2011, 111, 1293 CrossRef CAS PubMed;
(g) L. Ackermann, Chem. Rev., 2011, 111, 1315 CrossRef CAS PubMed;
(h) I. A. I. Mkhalid, J. H. Barnard, T. B. Marder, J. M. Murphy and J. F. Hartwig, Chem. Rev., 2010, 110, 890 CrossRef CAS PubMed;
(i) J. Yamaguchi, A. Yamaguchi and K. Itami, Angew. Chem., Int. Ed., 2012, 51, 8960 CrossRef CAS PubMed;
(j) J. Wencel-Delord and F. Glorius, Nat. Chem., 2013, 5, 369 CrossRef CAS PubMed.
- C–C bond formation via C–H bond activation:
(a) N. Grimster, C. Gauntlett, C. Godfrey and M. Gaunt, Angew. Chem., Int. Ed., 2005, 44, 3125 CrossRef CAS PubMed;
(b) Z. Shi, B. Li, X. Wan, J. Cheng, Z. Fang, B. Cao, C. Qin and Y. Wang, Angew. Chem., Int. Ed., 2007, 46, 5554 CrossRef CAS PubMed;
(c) H. Zhou, Y. Xu, W. Chung and T. Loh, Angew. Chem., Int. Ed., 2009, 48, 5355 CrossRef CAS PubMed;
(d) D. Wang, M. Wasa, R. Giri and J. Yu, J. Am. Chem. Soc., 2008, 130, 7190 CrossRef CAS PubMed;
(e) D. Pintori and M. Greaney, J. Am. Chem. Soc., 2011, 133, 1209 CrossRef CAS PubMed;
(f) L. Campeau, S. Rousseaux and K. Fagnou, J. Am. Chem. Soc., 2005, 127, 18020 CrossRef CAS PubMed;
(g) Y. Zhang, J. Feng and C. Li, J. Am. Chem. Soc., 2008, 130, 2900 CrossRef CAS PubMed;
(h) K. Gao, P. Lee, T. Fujita and N. Yoshikai, J. Am. Chem. Soc., 2010, 132, 12249 CrossRef CAS PubMed.
- C–N bond formation via C–H bond activation:
(a) J. Neumann, S. Rakshit, T. Dröge and F. Glorius, Angew. Chem., Int. Ed., 2009, 48, 6892 CrossRef CAS PubMed;
(b) A. Armstrong and J. Collins, Angew. Chem., Int. Ed., 2010, 49, 2282 CrossRef CAS PubMed;
(c) H. Wang, Y. Wang, C. Peng, J. Zhang and Q. Zhu, J. Am. Chem. Soc., 2010, 132, 13217 CrossRef CAS PubMed;
(d) H. Thu, W. Yu and C. Che, J. Am. Chem. Soc., 2006, 128, 9048 CrossRef CAS PubMed;
(e) C. Liang, F. Collet, F. Robert-Peillard, P. Müller, R. Dodd and P. Dauban, J. Am. Chem. Soc., 2008, 130, 343 CrossRef CAS PubMed;
(f) T. Kawano, K. Hirano, T. Satoh and M. Miura, J. Am. Chem. Soc., 2010, 132, 6900 CrossRef CAS PubMed.
- C–O bond formation via C–H bond activation:
(a) X. Wang, Y. Lu, H. Dai and J. Yu, J. Am. Chem. Soc., 2010, 132, 12203 CrossRef CAS PubMed;
(b) B. Xiao, T. Gong, Z. Liu, J. Liu, D. Luo, J. Xu and L. Liu, J. Am. Chem. Soc., 2011, 133, 9250 CrossRef CAS PubMed;
(c) Z. Yin, X. Jiang and P. Sun, J. Org. Chem., 2013, 78, 10002 CrossRef CAS PubMed;
(d) A. Dick, J. Kampf and M. Sanford, J. Am. Chem. Soc., 2005, 127, 12790 CrossRef CAS PubMed;
(e) Z. Ye, W. Wang, F. Luo, S. Zhang and J. Cheng, Org. Lett., 2009, 11, 3974 CrossRef CAS PubMed;
(f) A. Cook, M. Emmert and M. Sanford, Org. Lett., 2013, 15, 5428 CrossRef CAS PubMed;
(g) L. Desai, H. Malik and M. Sanford, Org. Lett., 2006, 8, 1141 CrossRef CAS PubMed;
(h) N. Jalalian, T. Petersen and B. Olofsson, Chem. – Eur. J., 2012, 18, 14140 CrossRef CAS PubMed;
(i) T. Petersen, R. Khan and B. Olofsson, Org. Lett., 2011, 13, 3462 CrossRef CAS PubMed.
- C–halides bond formation via C–H bond activation:
(a) R. Giri, X. Chen and J. Yu, Angew. Chem., Int. Ed., 2005, 44, 2112 CrossRef CAS PubMed;
(b) X. Wang, T. Mei and J. Yu, J. Am. Chem. Soc., 2009, 131, 7520 CrossRef CAS PubMed;
(c) X. Wan, Z. Ma, B. Li, K. Zhang, S. Cao, S. Zhang and Z. Shi, J. Am. Chem. Soc., 2006, 128, 7416 CrossRef CAS PubMed.
- C–P bond formation via C–H bond activation: C. Feng, M. Ye, K. Xiao, S. Li and J. Yu, J. Am. Chem. Soc., 2013, 135, 9322 CrossRef CAS PubMed.
- C–B bond formation via C–H bond activation:
(a) I. Mkhalid, J. Barnard, T. Marder, J. Murphy and J. Hartwig, Chem. Rev., 2010, 110, 890 CrossRef CAS PubMed;
(b) C. Liskey and J. Hartwig, J. Am. Chem. Soc., 2013, 135, 3375 CrossRef CAS PubMed.
- C–Si bond formation via C–H bond activation: E. Simmons and J. Hartwig, J. Am. Chem. Soc., 2010, 132, 17092 CrossRef CAS PubMed.
- T. Yoneyama and R. Crabtree, J. Mol. Catal. A: Chem., 1996, 108, 35 CrossRef CAS.
-
(a) L. Desai, H. Malik and M. Sanford, Org. Lett., 2006, 8, 1141 CrossRef CAS PubMed;
(b) B. Reddy, L. Reddy and E. J. Corey, Org. Lett., 2006, 8, 3391 CrossRef CAS PubMed;
(c) G. Wang, T. Yuan and X. Wu, J. Org. Chem., 2008, 73, 4717 CrossRef CAS PubMed.
-
(a) Z. Ye, W. Wang, F. Luo, S. Zhang and J. Cheng, Org. Lett., 2009, 11, 3974 CrossRef CAS PubMed;
(b) C. Hu, X. Zhang, Q. Ding, T. Lv, S. Ge and P. Zhong, Tetrahedron Lett., 2012, 53, 2465 CrossRef CAS PubMed;
(c) A. Dick, J. Kampf and M. Sanford, J. Am. Chem. Soc., 2005, 127, 12790 CrossRef CAS PubMed.
- X. Cheng, Y. Li, Y. Su, F. Yin, J. Wang, J. Sheng, H. Vora, X. Wang and J. Yu, J. Am. Chem. Soc., 2013, 135, 1236 CrossRef CAS PubMed.
- M. Yang, X. Jiang, W. Shi, Q. Zhu and Z. Shi, Org. Lett., 2013, 15, 690 CrossRef CAS PubMed.
-
(a) H. Liu, G. Shi, S. Pan, Y. Jiang and Y. Zhang, Org. Lett., 2013, 15, 4098 CrossRef CAS PubMed;
(b) K. Padala and M. Jeganmohan, Chem. Commun., 2013, 49, 9651 RSC.
Footnote |
† Electronic supplementary information (ESI) available: Experimental procedures and spectral data for all new compounds. See DOI: 10.1039/c5qo00003c |
|
This journal is © the Partner Organisations 2015 |
Click here to see how this site uses Cookies. View our privacy policy here.