Received
11th August 2011
, Accepted 13th October 2011
First published on 2nd November 2011
Abstract
Laser ablation-ICP MS was optimized for the sensitive detection of selenoproteins in polyacrylamide gel and PVDF membrane after blotting. For this purpose, two interlaboratory reference samples were prepared: glutathione peroxidase band in the gel and on the membrane, respectively. The optimisation was carried out using two systems: 213 nm laser (Newwave)—Agilent 7500ce ICP MS, and a 1030 nm high repetition rate femtosecond laser with galvanometric optics (Novalase)—PE/SCIEX DRCII. Sensitivity and signal-to-noise ratio were benchmarked to those obtained for the same sample by a recently published method in a reference lab. The optimization allowed a 12-fold gain of the S/N ratio during ablation of gels and a 3.5-fold gain in the ablation of blots in comparison with the method using an essentially similar system published by the reference lab. The gain of S/N by increasing ablation surface using the high repetition rate laser was not as spectacular as expected (2.5-fold for the gels and 1.5-fold for the blots) as the background noise increased considerably when a larger surface is ablated due to selenoproteins peak tailing. The study allowed for the first time LA-ICP MS detection of selenoproteins (separated by gel electrophoresis) in human cell extracts with the selenium concentration at the 10 ng ml−1 level.
Introduction
Selenium is an essential trace element known for its antioxidant properties. The physiological role of selenium is principally awarded to its co-translational incorporation into selenoproteins as selenocysteine (SeCys), referred to as the 21st amino acid.1 Twenty-five selenoproteins have been predicted in man by bioinformatics and they constitute the human selenoproteome.2 Out of them, the most widely studied have been the families of glutathione peroxidases (GPx), essential for fundamental defence mechanisms against oxidative stress, and thioredoxin reductases (TR) where selenium is crucial for catalytic activity. One third of the putative (predicted by bioinformatics using genomic sequence analysis) human selenoproteins has never been identified in vivo and their functions are unknown.
The understanding, on the molecular level, of the function and regulation of selenoproteins is critically dependent on the availability of adequate analytical methodology.3 It should allow the specific in vivo identification and quantification of individual selenoproteins, and, ideally, the simultaneous characterization of the entire selenoproteome. To date, the only universal detection has been based on the use of gamma-radioactive 75Se labeling and 1D gel electrophoresis autoradiography which is inappropriate for human studies and difficult to handle.4 Alternatively, Western immunoblotting has been successfully used but only for the few selenoproteins for which antibodies are available.5 The canonical proteomics protocols fail because of the very low selenoprotein/total protein ratio (<10−5) and the lack of specificity allowing to screen the selenoproteome fraction of the entire proteome.
Laser ablation ICP MS was first applied to the scanning of the electrophoretic gels for selenium-containing proteins in a waterfowl embryo and fish-ovary collected from Se-contaminated sites by Fan et al.6 The method was later applied to the detection of purified glutathione peroxidase7,8 but was limited to standards. Typically a 266 nm laser was used.6,7
The only, to our knowledge, application of GE-LA ICP MS to human samples was reported by Ballihaut et al.9 who detected two selenoproteins, in a human plasma fraction obtained after depletion of the seven most abundant proteins by immunoaffinity chromatography. This was possible owing to the 80-fold gain in sensitivity after the protein blotting onto a PVDF membrane. Electroblotting was also recommended to decrease the detection limits in a study of selenoprotein detection in fish.10 Another way reported to improve the sensitivity was the use of a femtosecond laser fitted with galvanometric optics allowing the ablation of a larger surface of the gel on the time scale of dwell time of the mass spectrometer offering an increase in sensitivity by a factor of 40.11
The literature reports are difficult to compare in terms of figures of merit as different systems (lasers and ICP mass spectrometers) are used and the detection limits and sensitivities are expressed in different ways. Therefore, in this study, interlaboratory reference samples (selenoprotein in the gel and on the membrane, respectively) were analysed in the only laboratory having so far reported the selenoprotein detection in human samples (NIST Charleston).9 The samples were then used to optimize in our laboratory the performance of the two LA-ICP MS systems: a commercial 213 nm laser coupled to a ICP collision cell MS (Agilent 7500) and an experimental high repetition rate femtosecond laser with galvanometric optic-ICP MS system described in detail elsewhere.11 The ultimate goal of our research was to verify the capacity of LA-ICP MS to speciate selenoproteins at ultratrace levels (10 ng g−1, i.e., 10-time lower than human serum) which is the prerequisite for probing the selenoproteome in cell lines. An extract of LNCaP cell line established as a model of human prostatic adenocarcinoma was investigated.
Experimental
New wave research (Freemont, CA) UP-213 laser coupled with Agilent 7500cs ICP MS (Agilent, Tokyo, Japan).
The laser was operated in a focused spot mode at the repetition rate of 20 Hz, spot size of 250 μm and 50 or 100 μm s−1 scan speed for gels or membranes respectively. Ablated matter was carried with He gas flow (500 ml min−1) and mixed in a T-connector with aerosol obtained using a Micromist nebuliser and a double pass Scott spray chamber from 1 ppb 89Y solution in 2% nitric acid and 1% methanol for gels and membranes, respectively. ICP MS was used in the collision cell mode using 3.6 ml min−1 of H2 as the collision/reaction gas. A 1.5 mm i.d. injector torch and Pt cones were used. The spectrometer was tuned off-line for the maximum Se signal-to-noise ratio.
Alfamet/novalase (Canejan, France) prototype laser coupled with Elan DRC II ICP MS (PE/Sciex, Toronto, ON).
The femtosecond laser ablation device (1030 nm) using galvanometric optics was described in detail elsewhere.12 The spot size of the focused laser beam was 20 μm. Scanning was performed in raster mode with scan speed of 50 μm s−1 for gels and 100 μm s−1 for membranes and with transversal beam speed of 100 mm s−1 resulting in an effective scan width of 1 to 2 mm.11 Repetition rate was 10 kHz, and fluence was ∼10 J cm−2. The aerosol was carried by helium at 150 ml min−1 and mixed with argon 820 ml min−1 before the entrance to the injector. A 2 mm i.d. injector torch and nickel cones were used. No aerosol was introduced in addition to that of the ablated sample as it was found to increase the probability of clogging the injector. The collision/reaction gas was hydrogen at 2.8 ml min−1. The spectrometer was tuned off-line for the maximum Se S/N ratio.
Reference data were obtained in NIST (Charleston, SC) using a New Wave Research UP-213 laser coupled with Thermo X II Series ICP MS (Thermo, Bremen, Germany) described in detail elsewhere.9 The operating conditions of all the systems used are summarised in Table 1.
Table 1 Summary of instrumental and data acquisition conditions
Set-up |
UP213/Thermo X II |
UP213/Agilent7500ce |
Novalase/Elan 6100 DRC II |
ICP MS
|
Injector ID, mm |
1.5 |
1.5 |
2 |
Plasma conditions |
Wet (1% butanol) |
Wet (blots: 1% MeOH, gels: 2% HNO3) |
Dry |
Carrier gas flow (He), ml min−1 |
401 |
500 |
150 |
Blend gas (Ar), ml min−1 |
680 |
760 |
820 |
Cones set |
Nickel |
Platinum |
Nickel |
RF power, W |
1349 |
1600 |
1000 |
Collision gas |
8% H2/92% He |
H2 |
H2 |
Collision gas flow, ml min−1 |
5.84 |
3.6 |
2.8 |
78Se dwell time, ms |
100 |
250 |
100 |
Laser
|
Laser source |
Nd-YAG |
Nd-YAG |
Diode-pumped KGW-Yb |
Repetition rate, Hz |
20 |
20 |
104 |
Fluence, J cm−2 |
22 |
6 |
10 |
Spot size/scan width, μm |
110 |
250 |
20 (width of scan 2 mm) |
Scan mode |
Line |
Line |
Raster |
Scan speed, μm s−1 |
75 |
50 (gels), 100 (blots) |
50 (gels); 100 (blots) (transversal speed 100 mm s−1) |
Electrophoresis was done in Criterion (Bio-Rad) cell. Tris-glycine was used as running buffer. 4% and 15% acrylamide gels were used as stacking and separating gels, respectively. Protein solution (cell extract or GPx solution) were mixed with Laemmli loading dye and loaded on gel. Migration was performed under 120 V. After electrophoresis gels were either stained with Coommassie blue or were blotted on PVDF membranes in iBlot (Invitrogen) dry system. After staining, gels were washed with water and put on Whatman 3MM Chromatography paper, covered with Saran film. Gels were dried using a vacuum dryer (Amersham). Membranes were stained with Coommasie blue for 10 min and then destained with 10% acetic acid solution in 30% methanol.
Preparation and characterization of the reference sample
The glutathione peroxidase 1 (GPx) preparation from bovine erythrocytes (Sigma-Aldrich) contained 2120 ± 14 ppm Se (determined by flow-injection ICP MS, theoretical value 3607 ppm). The purity of the sample was verified by size-exclusion chromatography-ICP MS using a Superdex 200 10/300 GL column and 0.1 M ammonium acetate (pH 7.6) at 0.7 ml min−1. The chromatogram is shown in Fig. 1a. A GPx solution containing 50.7 μg of protein was loaded on 15% SDS-PAGE into a 6 cm long well and migrated for 1.5 h until the migration front reached a distance of ca. 3 mm from the bottom of the gel. The gel was stained with Coomassie Blue, and vacuum dried at 80 °C for 1 h on 3MM Whatman chromatography paper. A gel prepared in parallel was transferred by electroblotting onto a PVDF membrane using a dry blotting system (iBlot Invitrogen).
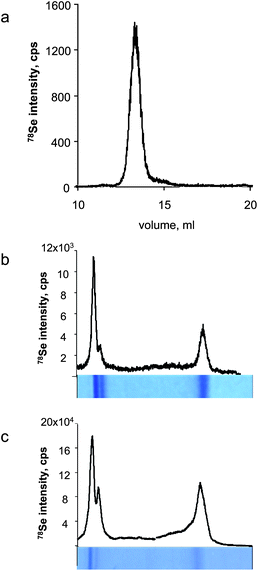 |
| Fig. 1 Characterisation of the glutathione peroxidase reference sample. a) SEC-ICP MS, b) LA-ICP MS of Coomassie Blue stained gel, c) LA-ICP MS of Coomassie Blue stained blot membrane. | |
Glutathione peroxidase gave two bands in gel electrophoresis (and the corresponding blots) corresponding to the tetramer and the monomer (Fig. 1bc). The resolution of the gel allows other GPx isoforms to be partly separated. The smaller peak of the monomer was chosen as the reference sample. Selenium was determined by FI-ICP MS in a 1 mm large and 4 mm long section of the gel. The gel was separated from the paper support using a scalpel, placed in a 5 ml tube and incubated with 500 μl of 30% H2O2 containing 0.25% NH3OH for 24 h at 85 °C. Then, 13.5 μl of conc. HNO3 was added, the sample was incubated for another ∼12 h at 85 °C, transferred to a 0.5 ml Eppendorf tube and centrifuged at 3900 g for 3 min. The quantification was done by external calibration using matrix-matched solution prepared by digestion of a 4 mm2 area of gel using the same procedure. The gel was found to contain 4.8 ng Se per mm2.
The LNCaP cells were grown in media supplemented with 30 nM sodium selenite for 3 days before harvesting. The extract contained about 10 ng ml−1 of selenium (10 times less than human serum) and was submitted to gel electrophoresis according to the protocol outlined above.
Results and discussion
Optimization of the new wave UP213/Agilent 7500ce system
Ablation of gels.
Fig. 2 (left panel) shows that the highest signal intensities were obtained for large spot sizes (>200 μm) and low scan velocities. This can be explained by the fact that the ablation of a large surface of gel requires time. Only at small spot sizes (<100 μm) higher scan velocities were acceptable but the sensitivity was 4 times lower than for a 250 μm spot scanning at 25 μm s−1. The latter conditions were selected as optimal, especially where the signal-to-noise ratio was by far the highest. A lower scanning velocity using a 250 μm spot allows an increase in the ablation depth which increases in the more material ablated without significant increase of the noise. The quasi linear increase of the S/N ratio from 150 μm indicates that for smaller spots an increase in noise was proportional to the amount of the ablated material. For the spot sizes below 150 μm, the signal and S/N ratios are quasi constant regardless of the scan velocity. Spots bigger than 250 μm were not investigated.
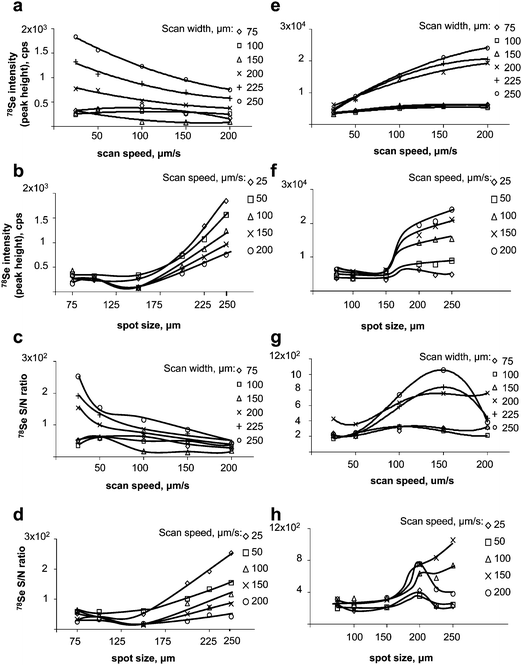 |
| Fig. 2 Optimisation of LA-ICP MS (UP213/Agilent7500ce). Left panel: gel. a) effect of the scan speed on the signal intensity; b) effect of the scan width on the intensity; c) effect of the scan speed on the S/N ratio; d) effect of the scan width on the S/N ratio. Right panel: membrane after blotting. e) effect of the scan speed on the signal intensity; f) effect of the scan width on the intensity; g) effect of the scan speed on the S/N ratio; h) effect of the scan width on the S/N ratio. | |
Ablation of membranes.
Selenoproteins blotted on the membrane are located on the surface and are easier accessible to the laser beam. Fig. 2 (right panel) shows that the sensitivity increases with the scan velocity as more material is introduced into the plasma per time unit. At high scan speeds the noise increases as well. Therefore, in terms of S/N ratio an optimum scan speed of 150 μm s−1 is recommended. The ease of ablating the membrane makes the work with large spot sizes privileged. Indeed, the intensity increases linearly with an increase in spot size from 200 to 250 μm. The optimum conditions were the spot size of 250 μm moving at 150 μm s−1. Note that, similarly as for the gels the sensitivities for small sizes are lower than it would result from a purely mathematical surface calculation and are not affected by the scan speed.
Optimization of the Novalase/DRCII system
Ablation of gels.
The ablation modus operandi using the femtosecond laser with galvanometric optics is different. A small laser spot size (20 μm) controlled by the optic scans at high repetition rate on a larger surface of the gel. In contrast to the UP213 system the scan width is not equal to the laser beam diameter but can be much larger (up to 2 mm). Consequently, the effects of the scan velocity (vertical to the band) and the scan width (horizontal to the band) on the sensitivity are different (Fig. 3 left panel). The maximum and reproducible sensitivity and the highest S/N ratio were obtained with the scan width of 1500 μm and the scan velocity of 150 μm s−1.
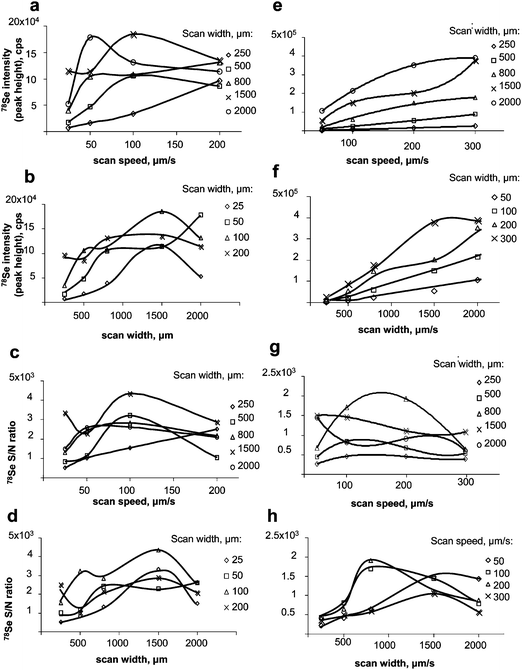 |
| Fig. 3 Optimisation of LA-ICP MS (Novalase/Elan DRC II). Left panel: gel. a) effect of the scan speed on the signal intensity; b) effect of the scan width on the intensity; c) effect of the scan speed on the S/N ratio; d) effect of the scan width on the S/N ratio. Right panel: membrane after blotting. e) effect of the scan speed on the signal intensity; f) effect of the scan width on the intensity; g) effect of the scan speed on the S/N ratio; h) effect of the scan width on the S/N ratio. | |
Ablation of membranes.
The easy accessibility of the blotted protein on the membrane makes the sensitivity increase quasi-linearly with the vertical scan velocity and the scan width (Fig. 3 right panel). The scan velocity reaches it optimum at 200 μm s−1 and the scan width of 1500 μm. There is no gain observed for higher scan widths as the large amount of organic particles introduced into the plasma affects negatively its ionization capacity. The work at high scan velocities is attractive as a gel can be scanned quicker but negatively affects the resolution if the bands of proteins of interest are close to each other.
Figures of merit
The figures of merit of the two systems and the reference data (NewWave UP213-Thermo ICP MS) are summarized in Table 2.
Table 2 Figures of merit for the ablation of a 2 mm wide gel (9.6 ng Se)
Set-up |
UP 213/Thermo X II |
UP 213/Agilent7500ce |
Novalase/Elan DRC II |
Material |
PAGE |
PVDF |
PAGE |
PVDF |
PAGE |
PVDF |
Height |
1472 |
28199 |
1842 |
14788 |
28199 |
171940 |
Noise |
103 |
224 |
10.2 |
36.5 |
68.9 |
272 |
S/N |
14.3 |
126 |
181 |
405 |
409 |
632 |
LOD, ng Se per 2mm |
1.5 |
0.17 |
0.12 |
0.053 |
0.053 |
0.034 |
Absolute LOD, pg Se |
83 |
9.4 |
15 |
6.7 |
40 |
26 |
Sensitivity and detection limits.
Fig. 4 shows the peaks obtained at the optimum conditions using the different systems. It can be seen that an increase in the laser beam spot size and ablation of membrane instead of gel results in a considerable gain in sensitivity. The instrumental detection limits (the minimum detectable amount of ablated selenium) are on the tens pg level being the smallest for the Agilent system (15 pg) and the highest for the Thermo ICP MS (83 pg). The fairly high values are due to the fact that only part of the gel containing selenium is ablated and the relatively rich matrix enters the plasma in the same time. Indeed, the simplification of the matrix results in the drop of absolute detection limits below 10 pg. This decrease is the most remarkable for the NewWave/Thermo system (8-times) and the least for the Novalase/Amplitude–Elan 6100 DRC II system because of the large surface of the gel scanned.
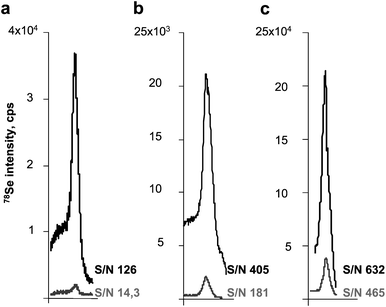 |
| Fig. 4 Comparison of the peak shape and height obtained for the analysis of gels (lower trace) and membranes (upper trace). a) UP 213/Thermo X II; b) UP 213/Agilent7500ce; c) Novalase/Elan DRC II. | |
Data in Table 2 show that the high effectiveness of ablation gives similar detection limits for the powerful Novalase/Amplitude system for gels and blots. For Agilent the ablation of blots offers two-times lower detection limits. In order to be effective the New Wave/Thermo system requires blotting.
Assuming the minimum width of gel as 2 mm and expressing the detection limits as a minimum detectable amount in such a band we arrive at the best performance of the Novalase/Amplitude system but the values offered by the UP213 Agilent are only 50%–100% higher.
Note that the background values are relatively high as there is a considerable tailing of selenoprotein throughout the gel, increasing the noise. It is expected that by working at much lower concentrations, the advantage of the Novalase/Amplitude system would be more pronounced because of the distinctly higher sensitivity.
Repeatability and linearity.
Fig. 5 shows the repeatability of the scans carried out with the different systems on the gels and blots. For gels a typical repeatability of 5% is achieved except for the UP213-Thermo system because the amount of selenium in the band was much closer to the detection limits obtained with this system in comparison with the other ones. Regarding the membranes, a considerable improvement of repeatability can be seen, after rejection of the 1st and possibly the 2nd scan with the nanosecond laser. It is unclear why the first scan always gave a much higher peak than the following ones. This phenomenon was not observed using the Novalase/Amplitude – Elan 6100 DRC II system.
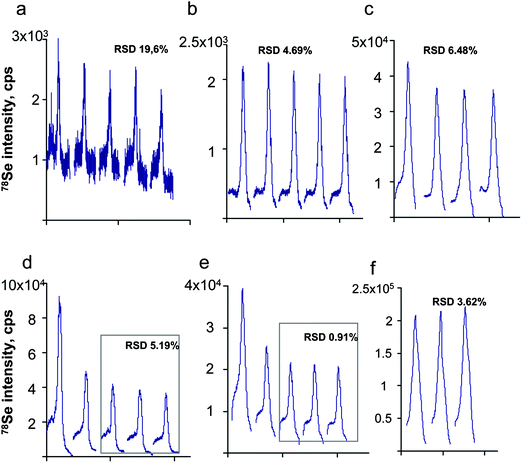 |
| Fig. 5 Repeatability of the peak shape during the analysis of: a,b,c) gels and d,e,f) blots. a,d) UP2 13/Thermo X II; b,e) UP 213/Agilent7500ce; c,f) Novalase/Elan DRCII. | |
Mass dependent enrichment of proteins during blotting.
The sensitivity of the detection of selenoproteins on the membrane depends not only on the laser ablation efficiency but also on the transfer efficiency from gel onto membrane. In order to investigate this, a gel containing Se-containing protein bands was prepared from a Se-rich yeast sample, analysed by LA-ICP MS (Fig. 6a) and transferred on the membrane which was also analysed by LA-ICP MS (Fig. 6b). The result showed a selective enrichment of proteins in the middle range of the molecular weight (30–45 kDa) (Fig. 6c); the larger proteins were not transferred whereas the smaller were not retained on the membrane. Indeed, the gel after blotting, analysed by LA-ICP MS (Fig. 6d), showed enrichment into high molecular weight proteins and impoverishment into the others (Fig. 6e).
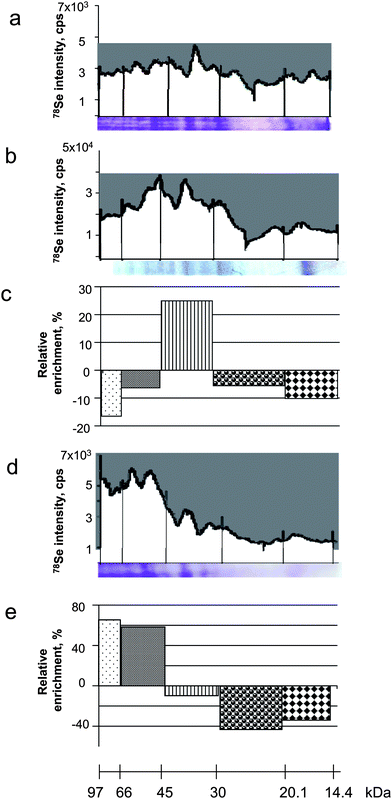 |
| Fig. 6 Efficiency of the selenoprotein transfer onto the membrane during blotting. a) LA-ICP MS electropherogram of the gel (Coomassie blue stained gel below); b) LA-ICP MS electropherogram of the membrane (Coomassie blue stained blot below); c) relative impoverishment/enrichment factor on the membrane as a function of the molecular weight of the protein; d) LA-ICP MS electropherogram of the remaining gel after blotting (Coomassie blue stained gel below); e) relative impoverishment/enrichment factor in the gel as a function of the molecular weight of the protein. | |
Detection of selenoproteins in extracts of cell lines
The method was applied to the detection of selenoproteins expressed in a LNCaP cell line referred to as a model of human prostatic adenocarcinoma. The cell line analysed contained ca. 10 ng ml−1 Se, which is an order of magnitude less than human serum. Coomassie Blue stained gels did not allow the observation of any clear bands. LA-ICP MS analysis, however, allowed the observation of a few well distinguished peaks in the electropherogram of the membrane (Fig. 7a) ablated using the NewWave/Agilent 7500ce system. The intensity of these peaks was increased by a factor of 2 when the membrane was analysed by the Novalase/Amplitude – ELAN 6100 DRC II system (Fig. 7b). The identity of three of the peaks was attributed by Western blotting to thioredoxine reductase and two glutathione peroxidase isoforms.
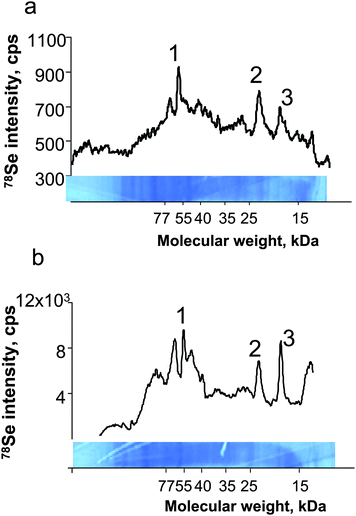 |
| Fig. 7 Laser ablation-ICP MS analysis of cell culture extracts. a) UP213/Agilent7500ce; b) Novalase/Elan DRC II. 1) TRxR, 2) GPx1, 3) GPx4. | |
Conclusions
The potential of LA-ICP MS detection in the literature is underestimated as we could reach a 12-fold gain of the S/N ratio during ablation of gels and 3.5-fold in the ablation of blots in comparison to a state-of-the-art report using a similar system (213 nm ns laser coupled to a collision cell ICP MS).9 The work allowed, for the first time, LA-ICP MS detection of selenoproteins in human cell extracts with the total selenium concentration at the 10 ng ml−1 level. The gain of S/N by increasing ablation surface using high repetition rate femtosecond laser with galvanometric optics was not as spectacular as expected (2.5-fold for the gels and 1.5-fold for the blots). This was due to a simultaneous increase in the background noise as a larger surface of the gel was ablated due to selenoproteins peak tailing.
Acknowledgements
The authors acknowledge the support of the ANR Project No ANR-09-BLAN-0048-01 SELENOPROTEOME as well as of Aquitaine Region and FEDER (Convention 20071303002PFP). JB acknowledges the fellowship of the French Ministry of Education.
References
- S. Gromer, J. K. Eubel, B. L. Lee and J. Jacob, Cell. Mol. Life Sci., 2005, 62, 2414 CrossRef CAS.
- G. V. Kryukov, S. Castellano, S. V. Novoselov, A. V. Lobanov, O. Zehtab, R. Guigo and V. N. Gladyshev, Science, 2003, 300, 1439 CrossRef CAS.
- I. L. Heras, M. Palomo and Y. Madrid, Anal. Bioanal. Chem., 2011, 400, 1717 CrossRef CAS.
- D. Behne, D. Alber and A. Kyriakopoulos, J. Radioanal. Nucl. Chem., 2004, 259, 435 CrossRef CAS.
- C. Méplan, F. Nicol, B. T. Burtle, L. K. Crosley, J. R. Arthur, J. C. Mathers and J. E. Hesketh, Antioxid. Redox Signaling, 2009, 11, 2631 CrossRef.
- T. W.-M. Fan, E. Pruszkowski and S. Shuttleworth, J. Anal. At. Spectrom., 2002, 17, 1621 RSC.
- C. C. Chéry, D. Günther, R. Cornelis, F. Vanhaecke and L. Moens, Electrophoresis, 2003, 24, 3305 CrossRef.
- G. Ballihaut, S. Mounicou and R. Lobinski, Anal. Bioanal. Chem., 2007, 388, 585 CrossRef CAS.
- G. Ballihaut, L. E. Kilpatrick, E. L. Kilpatrick and W. C. Davis, J. Anal. At. Spectrom., 2011, 26, 383 RSC.
- Z. Pedrero, Y. Madrid, C. Camara, E. Schram, J. B. Luten, I. Feldmann, L. Waentig, H. Hayen and N. Jakubowski, J. Anal. At. Spectrom., 2009, 24, 775 RSC.
- G. Ballihaut, F. Claverie, C. Pécheyran, S. Mounicou, R. Grimaud and R. Lobinski, Anal. Chem., 2007, 79, 6874 CrossRef CAS.
- C. Pécheyran, S. Cany, P. Chabassier, E. Mottay and O. F. X. Donard, J. Phys. Conf. Ser., 2007, 59, 112 CrossRef.
|
This journal is © The Royal Society of Chemistry 2012 |
Click here to see how this site uses Cookies. View our privacy policy here.