DOI:
10.1039/D3NR06476J
(Paper)
Nanoscale, 2024,
16, 7965-7975
Biomaterial-based combinatorial approach of aescin-comprised zein-coated gelatin nanoparticles alleviates synovial inflammation in experimental inflammatory arthritis†
Received
19th December 2023
, Accepted 12th March 2024
First published on 19th March 2024
Abstract
Rheumatoid arthritis (RA) is a chronic inflammatory autoimmune disease that mostly affects joints. Although RA therapy has made significant progress, difficulties including extensive medication metabolism and its quick clearance result in its inadequate bioavailability. The anti-inflammatory effect of zein was reported with other medications, but it has certain limitations. There are reports on the anti-oxidant and anti-inflammatory effect of aescin, which exhibits low bioavailability for the treatment of rheumatoid arthritis. Also, the combinatorial effect of zein with other effective drug delivery systems is still under investigation for the treatment of experimental collagen-induced rheumatoid arthritis. The focus of this study was to formulate and define the characteristics of zein-coated gelatin nanoparticles encapsulated with aescin (Ze@Aes-GNPs) and to assess and contrast the therapeutic effectiveness of Ze@Aes-GNPs towards collagen-induced RA in Wistar rats. Nanoprecipitation and the layer-by-layer coating process were used to fabricate Ze@Aes-GNPs and their hydrodynamic diameter was determined to be 182 nm. Scanning electron microscopy (SEM) and transmission electron microscopy (TEM) were used to further validate the size, shape, and surface morphology of Ze@Aes-GNPs. When tested against foreskin fibroblasts (BJ), these nanoparticles demonstrated significantly high cytocompatibility. Both Aes and Ze@Aes-GNPs were effective in treating arthritis, as shown by the decreased edoema, erythema, and swelling of the joints, between which Ze@Aes-GNPs were more effective. Further, it was demonstrated that Aes and Ze@Aes-GNPs reduced the levels of oxidative stress (articular elastase, lipid peroxidation, catalase, superoxide dismutase and nitric oxide) and inflammatory indicators (TNF-α, IL-1β and myeloperoxidase). The histopathology findings further demonstrated that Ze@Aes-GNPs considerably reduced the infiltration of inflammatory cells at the ankle joint cartilage compared to Aes. Additionally, immunohistochemistry examination showed that treatment with Ze@Aes-GNPs suppressed the expression of pro-inflammatory markers (COX-2 and IL-6) while increasing the expression of SOD1. In summary, the experiments indicated that Aes and Ze@Aes-GNPs lowered the severity of arthritis, and critically, Ze@Aes-GNPs showed better effectiveness in comparison to Aes. This suppression of oxidative stress and inflammation was likely driven by Aes and Ze@Aes-GNPs.
1. Introduction
Around 1% of the global population suffers from the common persistent condition rheumatoid arthritis (RA), which has high morbidity.1 Pannus development, angiogenesis, cartilage and bone deterioration, gradual joint degeneration, and impairment are a part of the RA pathophysiology.2–4 The exact origin of RA is still unknown, although there are several potential explanations for its onset, including an aberrant autoimmune action, genetic abnormalities, and various biological and external factors such as hormone changes, infections from microbes, and metal exposure.5,6 Presently, RA treatments focus on methods that reduce discomfort, prevent bone and joint deterioration, and address other symptoms.7 TNF-alpha and interleukins (interleukin 1) are cytokines that promote inflammation, which are essential integrators of inflammatory acute-phase and responses of immune cells, and two major factors in the pathogenesis of RA. Additionally, TNF-α participates in the activation of various additional cytokines that are proinflammatory during acute inflammatory conditions, which are linked to discomfort and damage to tissues.8–11 These cytokines also mobilise and trigger extra inflammatory cell infiltration, resulting in the production of enzymes that damage cartilage and bone.12–14 Presently, non-steroidal anti-inflammatory medicines (NSAIDs), corticosteroids, and disease-modifying antirheumatic drugs (DMARDs) are used in typical RA therapy methods; however, these medications have significant drawbacks and adverse effects.15–20
Zein, the primary storage protein in maize, makes up 30% to 60% of the total protein composition of this plant.21 It lacks acidic and basic amino acids but includes over 50% amino acids, which are non-polar such as proline, leucine, phenylalanine, alanine, isoleucine and valine.22 The insufficient nutrient content in zein is caused by its unbalanced amino acid profile. However, zein is readily available, biodegradable and biocompatible.23 Thus, due to its high bioavailability and biocompatibility, zein has been widely used in the food and pharmacological sectors. Zein has prospective usage in food packaging, electrical appliances, and improving oral drug delivery. Zein has the ability to generate hydrophobic coatings, encapsulation and biological films via the process of self-assembly.24
This ability of zein was used to encapsulate the flax oil, alpha-tocopherol, vitamin D3, citral and lime.23,25–28 Due to their distinct interior-shell architectures, amphiphilic molecules can serve as nano drug vehicles, and the core of these molecules makes a perfect pocket for loading drugs, in particular medicines with a hydrophobic nature.29,30
The triterpene saponin aescin is the main active ingredient of Aesculus hippocastanum L. (Hippocastanaceae).31 According to reports, aescin has anti-edamatous, anti-inflammatory, antioxidant, and anti-exudative activities and protective properties.32–37 Therefore, aescin is often employed in the therapeutic management of subchronic inflammation, haemorrhoids and traumatic edoema.38 Aescin is a powerful anti-inflammatory medicine and has been found to have a lasting effect on inflammation and not inhibit the immune system.39,40
Currently, aescin injection is commonly utilized therapeutically to minimize inflammatory edoema following trauma such a dislocation and surgery.41 Nevertheless, aescin injection may result in several adverse consequences that affect many organs and systems, where phlebitis is one of most frequent side effects with an allergic response.42 Severe outcomes are likely contributed by the pH value, impurity and dilution of the treatment solution.42,43
Considering the above-mentioned drawbacks of aescin, the focus of this study was to evaluate the effectiveness of zein-coated aescin-loaded gelatin nanoparticles (Ze@Aes-GNPs) in overcoming its adverse effects and enhancing its bioavailability and biocompatibility. Despite the advancements in this field of study, aescin nanoformulations have not been evaluated to date for their ability to prevent rheumatoid arthritis. Thus, the aim of this investigation is to elucidate the protective and anti-inflammatory benefits of zein-coated aescin-loaded gelatin nanoparticles (Ze@Aes-GNPs) against CIA-induced rheumatoid arthritis.
2. Methods
2.1 Synthesis and characterization of Ze@Aes-GNPs
The detailed methods, characterization and experimental discussion are included in the ESI.†
2.2 Induction of collagen-induced arthritis (CIA)
Arthritis was induced in Wistar rats following established protocols. In summary, collagen type-II sourced from bovine nasal septum was dissolved in 0.05 M acetic acid at a concentration of 2 mg mL−1. Subsequently, this solution was emulsified with an equal volume of Complete Freund's Adjuvant (CFA) containing 1 mg mL−1Mycobacterium tuberculosis H37 RA and kept on ice until administration. Rats were immunized with this emulsion intradermally, approximately 1.5 cm distal from the base of their tail.
Previous studies and our own standardization efforts for the collagen type-II-induced arthritis animal model showed that rats immunized with nasal bovine collagen type-II emulsified in the CFA-containing Mycobacterium tuberculosis strain H37RA developed swelling in their foot pads and ankles, which is characteristic of arthritis. In contrast, rats immunized with nasal bovine collagen type-II emulsified in incomplete Freund's adjuvant did not exhibit a significant increase in their foot pad and ankle width. As a result, CFA was selected over incomplete adjuvant because it sensitized rats to develop rheumatoid arthritis.
2.3 Ethical statement for use of experimental animals
All animal procedures were performed in accordance with the Guidelines for Care and Use of Laboratory Animals of Era's Lucknow Medical College Hospital, Lucknow, India and approved by the Animal Ethics Committee of Era's Lucknow Medical College Hospital. The standard conditions were maintained and the experiments were carried out according to IAEC (The Institutional Animal Ethics Committee) and CPCSEA guidelines and the project number is IAEC/ELMCH/1/22/-6. Initially, they had six days of acclimatization prior to treatment. The seventh day marked the beginning of the disease induction.
To study the effects of treatment of Ze@Aes-GNPs against collagen-induced arthritis, 30 female Wistar rats were randomly allocated to 5 groups with 6 rats each.
Control: Healthy rats that received a basal diet.
CIA: Rats served as collagen-induced arthritis (CIA) group.
CIA + Free Aes: Rats served as CIA group, Aes 30 mg per kg b.wt. (i.a) from onset of disease once daily, i.e., from 12th day of immunization up to day of sacrifice, i.e., day 21.
CIA + Ze@Aes-GNPs: Rats served as CIA group, treated with Ze@Aes-GNPs in doses equivalent to 30 mg per kg body weight of AES from onset of disease once daily (i.a), i.e. from 12th day of immunization up to day of sacrifice, i.e. day 21.
Ze@GNPs: Served as blank nanoparticle-treated rats.
All rats were anaesthetized with mild anaesthesia and sacrificed by cervical dislocation on the 21st day.
3. Results and discussion
3.1 Preparation of nanocarrier
The nanoprecipitation method was used for the formulation of Ze@Aes-GNPs (zein-coated aescin-loaded gelatin nanoparticles)44 (Fig. 1).
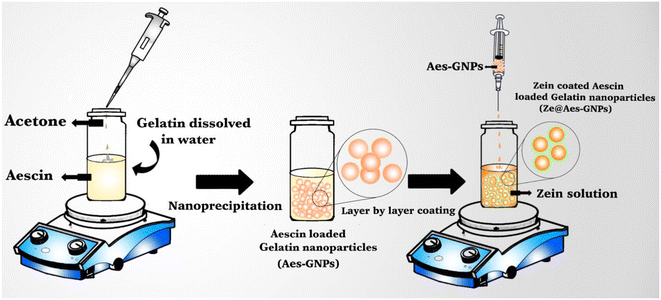 |
| Fig. 1 Schematic representation of Ze@Aes-GNP (zein-coated aescin-loaded gelatin nanoparticles) formulation. | |
To cover the GNPs (gelatin nanoparticles) using zein, 1% (w/v) zein solvent (in 80% ethanol solvent) was introduced dropwise into 1
:
1 (w/v) of GNPs and gently stirred over one hour. Further, the uncoated zein was removed via the centrifugation method (10
000 rpm, 15 min). The precipitate, i.e. zein-coated gelatin nanoparticles (Ze@GNPs), was collected and dried via lyophilization. The same procedure was employed to synthesize the zein-coated aescin (Aes)-loaded gelatin nanoparticles (Ze@Aes-GNPs).
After the synthesis of the optimized nanoformulation, various techniques were used for its characterization.44
3.2 Characterization of nanocarrier
For the hydrodynamic diameter size and zeta potential analysis, dynamic light scattering (DLS) was performed. According to the DLS measurement analysis, GNPs possessed a hydrodynamic size (particle size) of 136 nm (Fig. 2a), and the average hydrodynamic diameter increased to 159 nm and 182 nm after loading Aes in GNPs and coating them with zein, i.e. Ze@Aes-GNPs (Fig. 2b and c). Furthermore, we found that GNPs, Aes-GNPs and Ze@Aes-GNPs exhibited monodispersity (no aggregation formation), as indicated by their polydispersity index (PDI) values of 0.31, 0.23, and 0.29 and zeta potential of −12.5 mV, −14.6 mV and −6.71 mV (Fig. 2a′–c′), respectively. The high zeta potential values of the particles prevent their aggregation and precipitation, making them suitable for long-term storage. Our zeta potential results are in accordance with previous reports, where a high zeta potential confirmed better stability.45 Furthermore, high-level electron microscopy was used for the analysis of the shape, size and surface morphology of Ze@Aes-GNPs. The SEM and TEM findings confirmed that Ze@Aes-GNPs possessed a hydrodynamic size in the range of 170–190 nm, which are close to spherical in shape and monodisperse, indicating the presence of particles without aggregation (Fig. 2f and g).
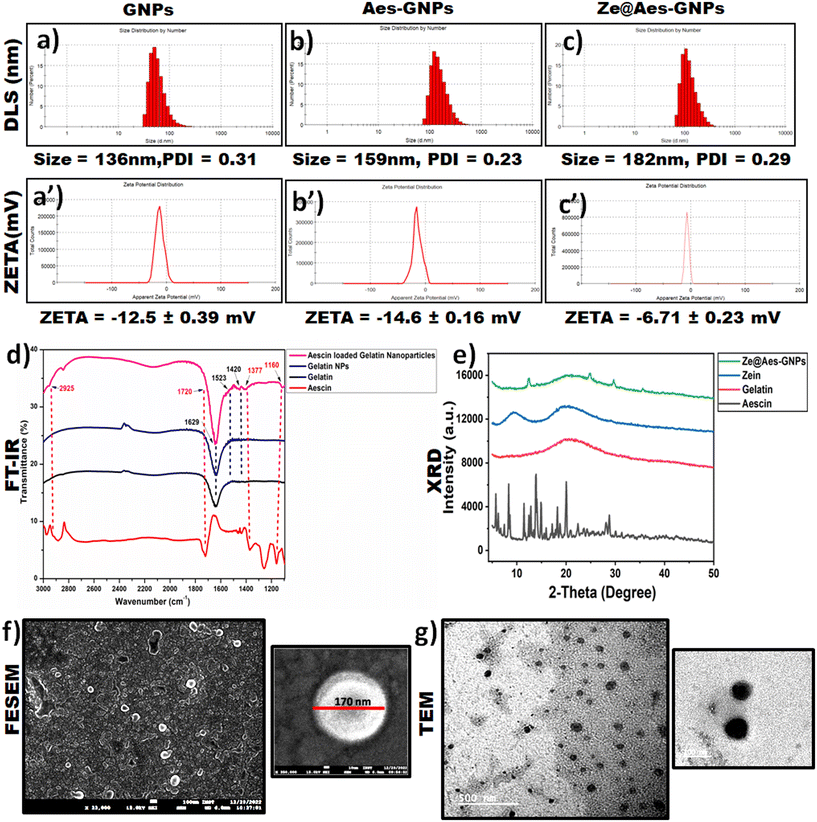 |
| Fig. 2 Characterization of nanoparticles: mean hydrodynamic particle size, polydispersity index (PDI) measurements and zeta potential of nanoparticles. Hydrodynamic size of (a) GNPs (gelatin nanoparticles), (b) Aes-GNPs (aescin loaded-gelatin nanoparticles), (c) Ze@Aes-GNPs (zein-coated aescin-loaded gelatin nanoparticles) and zeta potential of (a′) GNPs (gelatin nanoparticles), (b′) Aes-GNPs (aescin loaded-gelatin nanoparticles), and (c′) Ze@Aes-GNPs (zein-coated aescin-loaded gelatin nanoparticles). (d) FTIR spectra of free aescin, free gelatin, Ze@GNPs (zein-coated gelatin nanoparticles), Ze@Aes-GNPs (zein-coated aescin-loaded gelatin nanoparticles). (e) XRD spectroscopy of aescin, gelatin, zein, and Ze@Aes-GNPs. Morphological characterization by electron microscopy of Ze@Aes-GNPs (zein-coated aescin-loaded gelatin NPs). (f) FESEM and (g) TEM images. | |
3.3 Drug loading and release kinetics
UV-VIS spectroscopy was used for the confirmation of the aescin (Aes) loading in Ze@Aes-GNPs (Fig. 2e). Ze@Aes-GNPs had the optimised encapsulation and Aes loading of 76.61% and 22.27%, respectively. The study of the Aes release kinetics from Ze@Aes-GNPs was performed as previously stated,46 but with a minor change (Fig. 3a). We observed the sustained release of Aes from Ze@Aes-GNPs. Initially in 24 h, 48–50% of Aes started to be release. As a result, the release of Aes from Ze@Aes-GNPs continued for up to 84 h, during which about 80% of Aes was released from Ze@Aes-GNPs.
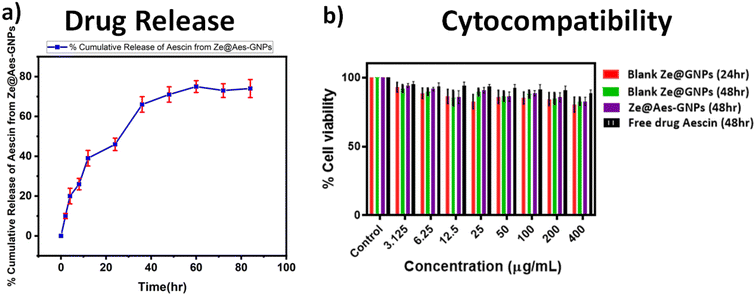 |
| Fig. 3 Representative graph of drug release kinetics and cytocompatibility: (a) kinetics of cumulative drug release of aescin from Ze@Aes-GNPs (zein-coated aescin-loaded gelatin nanoparticles) at pH 7.4 and 37 °C in PBS (phosphate buffered saline). (b) Cytocompatibility, MTT assay exhibiting of free aescin, Ze@GNPs (zein-coated gelatin nanoparticles) and Ze@Aes-GNPs (zein-coated aescin-loaded gelatin nanoparticles) at normal hTERT-BJ (human foreskin fibroblasts cells). | |
3.4 Cytocompatibility
The cytocompatibility of Ze@GNPs and Ze@Aes-GNPs to ensure that these biomaterials are not harmful to cells was deduced towards normal hTERT-BJ (human skin fibroblast BJ) cells at intervals of 24 and 48 h. We observed that a dose of Ze@GNPs and Ze@Aes-GNPs of up to 200 μg mL−1 was not harmful (cytocompatible) to hTERT-BJ cells and the minimal cytotoxic effect of Ze@GNPs and Ze@Aes-GNPs because of the non-significant change in the cell viability (Fig. 3b). Alternatively, the cell viability started to show a modest decline at a higher dose of 400 μg mL−1 at both intervals; nonetheless, the decrease was not statistically significant. Our finding demonstrated that on normal cells, a dose of up to 200 μg mL−1 nanoparticles can be considered a safe concentration.
4. Results and discussion
4.1 Effect of treatment with Ze@Aes-GNPs on disease clinical severity
Erythema and edoema, which are manifested by the swelling and redness of the joints, are clinical indicators of disease. The inflammation in one or several ankle joints, followed by engagement in the interphalangeal, metatarsal and joints, which first showed in the back paws between days 10 and 12 after receiving the CIA and reached 100% prevalence on day 13 ± 1. The rats with arthritis showed both arthritic and inflammatory alterations in their back paw edoema. In comparison to the CIA-group, it was discovered in the current investigation that administering Aes and Ze@Aes-GNPs to arthritic rats (collagen-immunized) considerably prevented the development of arthritis (Fig. 4a). Following the treatment using Ze@Aes-GNPs, decreased edema and inflammation were also shown by the change in the paw diameter of the rats (Fig. 4b and c).
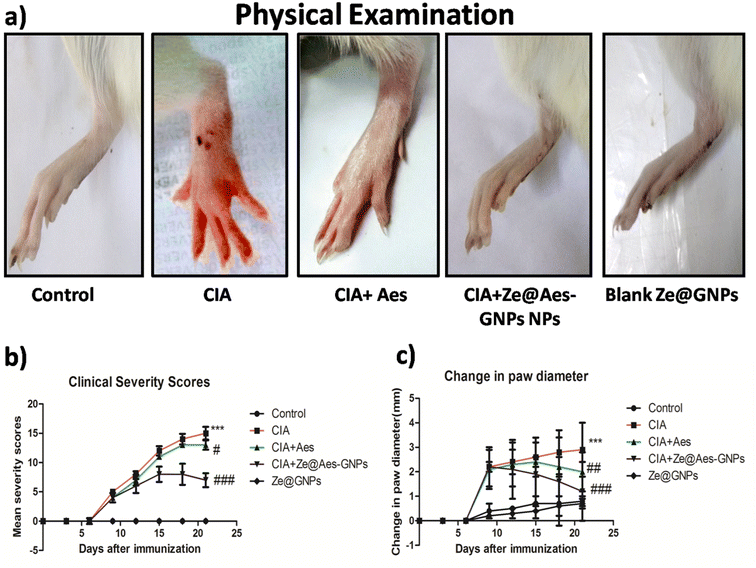 |
| Fig. 4 (a) Representative photographs showing status of inflammation on day of sacrifice in different groups. Representative graph showing effect of free aescin and Ze@Aes-GNPs on mean (b) change in score of clinical severity and (c) paw diameter change in collagen type II-immunized rats (n = 6, mean ± SD). Statistical changes are shown by #p ≤ 0.05, ##p ≤ 0.01, and ###p ≤ 0.001 compared to disease group and ***p ≤ 0.001 compared to the control. | |
4.2 Effect of treatment with Ze@Aes-GNPs on articular elastase (ELA) and other biochemical parameters
The amount of articulate elastase (ELA), which is produced by activated granulocytes at the place of damage, is directly correlated with the buildup, and stimulation of ELA activities significantly increased when compared with the control. Subsequently, it was shown that Aes (30 mg per kg b.wt.) and Ze@Aes-GNPs (equivalent to 30 mg per kg b.wt. based on the carrying potential) ELA therapy greatly reduced the infiltration and stimulation of neutrophils, consequently reducing arthritic synovial tissue inflammation. Ze@Aes-GNPs dramatically reduced the expression of ELA in comparison to Aes, and hence they had a greater impact than Aes. The ELA levels of activity remained considerably higher in the CIA + Ze@GNP group than the control, but there was no discernible difference between the Ze@GNP group and the control (Fig. 5a).
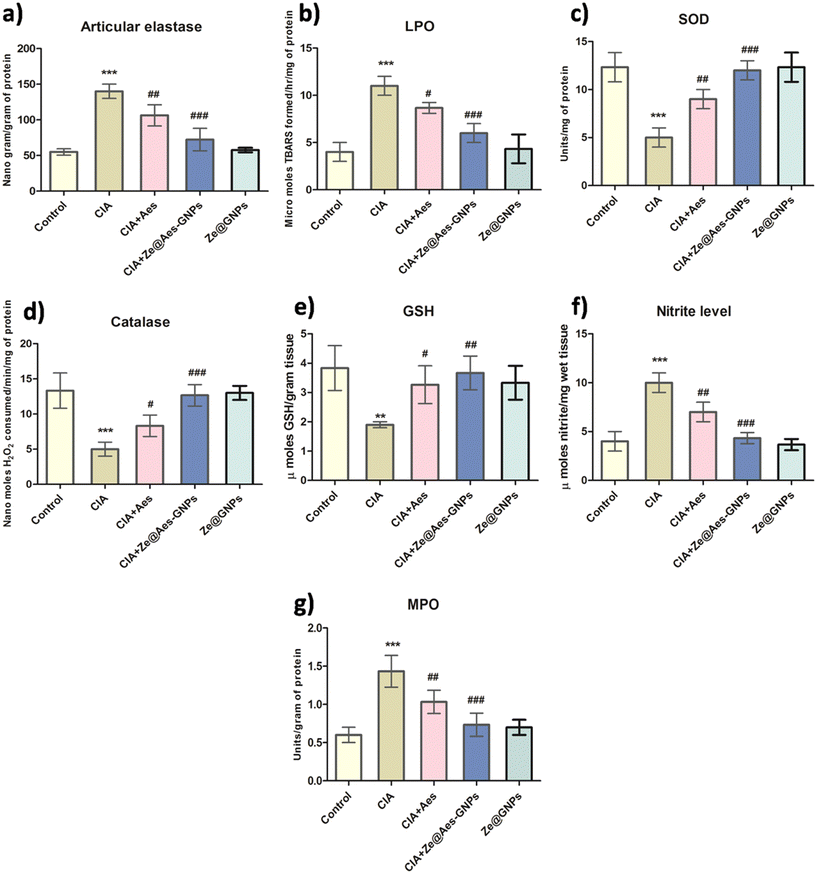 |
| Fig. 5 Representative graph showing effect of free Aesin and Ze@Aes-GNPs on oxidative stress and inflammatory markers (a) ELA level, (b) lipid peroxidation (LPO), (c) SOD activities, (d) catalase activities, (e) GSH content, (f) nitrite level, and (g) MPO levels in joints (n = 6, mean ± SD). Statistical changes are shown by #p ≤ 0.05, ##p ≤ 0.01, and ###p ≤ 0.001 compared to disease group and ***p ≤ 0.001 compared to the control. | |
The barrier of endothelial cells is breached when infiltrating PMNs cause oxidative stress, causing the interendothelial spaces to close. The roles of Aes and Ze@Aes-GNPs in oxidative stress-related indicators such superoxide dismutase (SOD), glutathione (GSH), lipid peroxidation (LPO), and catalase were further studied in the context of the aforementioned findings.
Aes has been observed to have free radical scavenging effects47 and antioxidant activity, whereas TBARS, a hallmark of LPO, are produced partially due to elevated ROS and damage cell membranes. Our findings showed that the TBARS levels in the CIA group dramatically increased compared with the control, whereas Aes improved the integrity of the membrane by lowering the LPO levels, and Ze@Aes-GNPs considerably decreased the LPO levels when correlated with arthritic animals (CIA group). Treatments with Ze@GNPs had no effect on the level of TBARS, which remained markedly elevated in comparison to the control (Fig. 5b).
Catalase, SOD, and GSH are components of the intracellular detoxifying process, which help to keep the internal redox states of cells stable by scavenging excess free radicals.48 Catalase, a key enzyme that scavenges free radicals, acts to avoid the creation of harmful free oxidative radicals. In contrast, SOD serves as the first line of defence by the body against free radical assaults by catalysing the conversion of the anion superoxide (O2−) to hydrogen peroxide (H2O2) and molecular oxygen (O2).48 A crucial cellular antioxidant, GSH is a tripeptide comprised of cysteine, glutamic acid, and glycine.48 According to our findings, the CIA group had significantly lower catalase and SOD activity and elevated GSH levels than the control group. Subsequently, it was discovered that Aes therapy considerably enhanced the GSH level, catalase and SOD activities compared to the arthritic CIA group. Additionally, Ze@Aes-GNPs were shown to be more effective than Aes at drastically reducing catalase and SOD activities in addition to GSH levels when compared to the arthritic group. The catalase, SOD activity, and levels of GSH after administration with Ze@GNPs did not significantly vary between the CIA + Ze@GNP group and CIA group, or between the Ze@GNP group and the control animals. Compared with control animals, all these indicators were quantitatively different in the CIA + Ze@GNP group, indicating that Ze@GNPs had no positive impacts (Fig. 5c–e). Our findings decisively show that the protective properties of Ze@Aes-GNPs were controlled by their antioxidant effects, which reduced lipid peroxidation and strengthened the natural antioxidant defences of the body. Based on the ability of Ze@Aes-GNPs to improve joint edoema and erythema, we also looked at how Aes and Ze@Aes-GNPs affected biomarkers of inflammation including myeloperoxidase (MPO) and nitric oxide (NO). The signalling NO creates nitrite, which signals NO synthesis in numerous tissues and inflammatory reactions. Nitrite is a robust physiological repository for NO.49,50 Therefore, substances that reduce the overactive generation of NO may benefit those who have arthritis by preventing cartilage breakdown.51 The adverse consequences of NO are caused by its capacity to react with superoxide anions (O2−) and produce the peroxynitrite radical (ONOO˙). MPO oxidase is required for the oxidation of nitrite into nitrogen dioxide, which is a significant inflammatory indicator.52 As a result, inflammation is characterised by the correlation between the MPO activity and NO level.
Compared with the control, the MPO activity and nitrite level in the joints of the CIA-induced arthritic animals were considerably higher, and when the CIA-induced arthritic rats were treated with Aes and Ze@Aes-GNPs, these levels and MPO activity were dramatically reduced. The MPO activity and nitrite level in CIA + Ze@GNPs were not altered, and as a result of treatment with Ze@GNPs, and they continued to be much higher than that in the control (Fig. 5f and g).
4.3 Effect of treatment with Ze@Aes-GNPs on pro-inflammatory cytokines (TNF-α and IL-1β)
TNF-α, IL-6, and other cytokines that are pro-inflammatory are also secreted in greater quantities as a result of systemic inflammation.53 Pro-inflammatory TNF-α and IL-6 cytokines were shown to be more expressed during rheumatoid arthritis, autoimmune diseases, and inflammation. Leucocytes and hepatocytes and are the major cells that release CRP during systemic inflammation.53 According to reports, IL-6 controls CRP release, but TNF-α has a linear relationship with both IL-6 and CRP.54 On the 21st day, the plasma CRP levels in every group were measured, and it was discovered that the level in arthritic rats was much greater than the usual control. In comparison to the CIA group, treatment with Aes and Ze@Aes-GNPs considerably decreased the CRP level. Additionally, the serum TNF-α levels of the CIA group were considerably higher than that of the control. The levels of TNF-α considerably decreased upon administration with Aes and Ze@Aes-GNPs compared to the arthritic-induced group. Similarly, the blood serum IL-1β levels of the CIA group were considerably higher than that of the control.
The IL-1β levels decreased considerably following administration with Aes and Ze@Aes-GNPs compared to the CIA-induced arthritic animals. The blood serum IL-1β levels in both the control group and CIA-induced arthritic animals were unaffected by Ze@GNPs. When CIA + Ze@GNPs were compared to the standard control, Ze@GNPs had no favourable impacts on the levels of CRP, TNF-α, and IL-1β (Fig. 6a and b).
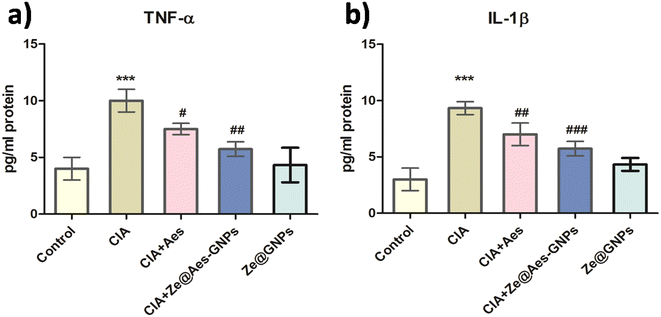 |
| Fig. 6 Representative graph showing effect of free aescin and Ze@Aes-GNPs on pro-inflammatory cytokine levels in rat serum showing (a) TNF-α level and (b) IL-1β level in serum (n = 6, mean ± SD). Statistical changes are shown by #p ≤ 0.05, ##p ≤ 0.01, and ###p ≤ 0.001 compared to disease group and ***p ≤ 0.001 compared to the control. | |
4.4 Immunohistochemistry of Ze@Aes-GNP-treated synovium cells for COX-2 and IL-6 expression
The key inflammation regulators are COX-2 and IL-6, which are mediated by pro-inflammatory cytokines. The COX-2 and IL-6 levels are known to be elevated in rheumatoid arthritis (RA) patients, and elevated levels of cytokines are signs of the development of disease.55,56 Our results showed that compared to the CIA group, administration with Aes and Ze@Aes-GNPs significantly reduced the IL-6 and COX-2 in the immunopositive cells and synovium (Fig. 7). The immunohistochemical staining of the cells was also subjected to a semi-quantitative tests analysis. The CIA-induced arthritic group showed a considerably increased IL-6 and COX-2 level, while treatments with Aes and Ze@Aes-GNPs considerably decreased their expression. The IL-6 and COX-2 secretion in CIA + Ze@GNPs relative to the control and Ze@GNPs compared to CIA and the control; accordingly, Ze@GNPs did not show any improvements. In comparison to the CIA group, the immunostaining of these specific inflammatory indicators was diminished in the groups receiving Ze@Aes-GNPs (Fig. 7).
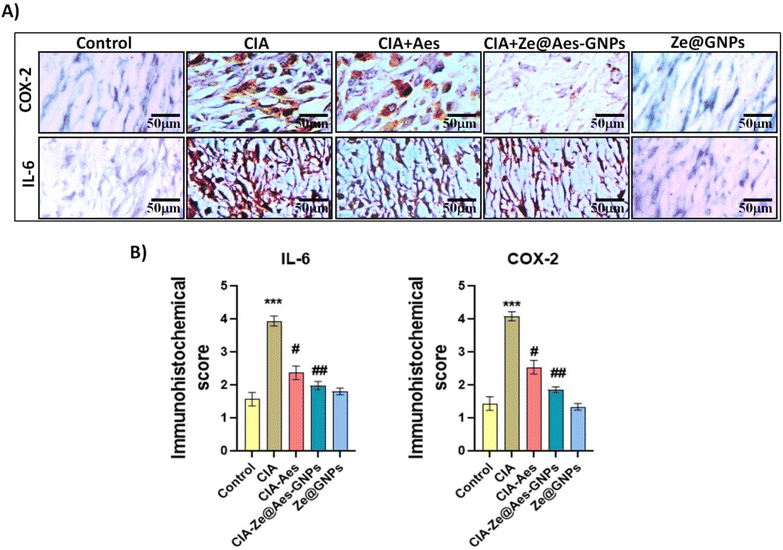 |
| Fig. 7 (A) Representative photomicrographs showing the effect of free aescin and Ze@Aes-GNPs on COX-2 and IL-6 expression in synovium. Brown shade denotes particular COX-2 and IL-6 immunostaining. The control group had either very little or no discoloration, CIA group has much more immunopositive stained cells than the control group compared to CIA and the CIA + Aes-treated group exhibited reduced immunopositive stained cells. Compared to the CIA group, the CIA + Ze@Aes-GNP-treated group displayed significantly reduced immunopositive stained cells. Relative to the control, the blank NPs (Ze@GNPs) exhibited no immunopositive stained cells (40× – original magnification and 50 μm – scale bar). (B) Quantitative evaluation of IL-6 and COX-2. Statistical changes are shown by #p ≤ 0.05, ##p ≤ 0.01, and ###p ≤ 0.001 compared to disease group and ***p ≤ 0.001 compared to the control. | |
4.5 Histological examinations of the synovium cells treated with Ze@Aes-GNPs
Based on the histological data, the synovium of the CIA group showed the extensive infiltration of inflammatory cells, which was followed by articular cartilage degradation. Compared to synovial hyperplasia, the bone underwent erosion and pannus development. Aes treatment reduced the histological changes and made it possible to greater repair the alterations.
However, Ze@Aes-GNP therapy significantly reduced inflammation in comparison to Aes. There were no noticeable impacts of Ze@GNPs on the joint structures. Histopathological examinations of the joints provided additional support for the biochemical and immunohistochemistry findings. The histological changes were greatly repaired by Ze@Aes-GNPs medication (Fig. 8).
 |
| Fig. 8 Representative photomicrograph showing effect H & E staining of free aescin and Ze@Aes-GNPs on histology of joint synovium. Control group showing no cellular infiltration in the synovium. According to the CIA, the synovium had significantly more cellular infiltration than the control and the CIA + Aes-treated group showed less infiltration of cells in the synovium relative to the CIA arthritic animals, whereas the CIA + Ze@Aes-GNP-treated group showing much less infiltration of cells in the synovium relative to the CIA arthritic animals. Comparison with the control and blank NPs (Ze@GNPs) showing no infiltration of cells within the synovium (40× – original magnification and 50 μm – scale bar). | |
In its entirety, rheumatoid arthritis increased the clinically significant extent of inflammation in the joint, elevated the biomarkers of pro-inflammation in the serum, induced the extensive entry of inflammatory cells in the lining of the synovium, and initiated articular cartilage destruction and erosion of bones. Alternatively, administration with Ze@Aes-GNPs increased the bioavailability of aescin in CIA-induced arthritic rats. Additionally, it improved serum inflammatory indicators, reduced the infiltration of inflammatory cells in the synovium, restored intrinsic antioxidant properties, minimised inflamed joint clinical severity, reduced articular cartilage destruction and erosion of the bone.
5. Conclusion
According to this study, zein-coated gelatin nanoparticles loaded with aescin exhibited anti-arthritic properties in experimental rats. These benefits are likely caused by inhibiting the stimulation and development of PMN cells, followed by regulation of the synthesis of biomarkers of inflammation and enzymes that are antioxidants. Therefore, Ze@Aes-GNPs may be an alternative medication for treating rheumatoid arthritis due to their strong antioxidant and anti-inflammatory capabilities. To further understand the underlying mechanism for the antiarthritic activities of Ze@Aes-GNPs, additional research is required.
Author contributions
Chandrashekhar Jori: Data curation, formal analysis, investigation, methodology, software, visualization, writing-original draft, writing-review & editing. Md. Meraj Ansari: Data curation, formal analysis, investigation, methodology, software, writing-original draft, writing-review & editing. Anas Ahmad: Data curation, formal analysis, investigation, methodology. Nemat Ali: Data curation, formal analysis, investigation, methodology, resources. Syed Shadab Raza: Conceptualization, data curation, formal analysis, investigation, methodology, software, writing-original draft, writing-review & editing. Rehan Khan: Conceptualization, supervision, resources, funding acquisition, validation, formal analysis, investigation, software, writing-original draft, writing-review & editing.
Conflicts of interest
There are no conflicts to declare.
Acknowledgements
This work is supported by the Department of Science and Technology (DST), SERB with Grant No. CRG/2019/004018. The authors acknowledge and extend their appreciation to the Researchers Supporting Project Number (RSPD2024R940), King Saud University, Riyadh, Saudi Arabia.
References
- A. Gibofsky, Am. J. Manag. Care, 2012, 18, S295–S302 Search PubMed.
- H. Liu, Y. Zhu, Y. Gao, D. Qi, L. Zhao, L. Zhao, C. Liu, T. Tao, C. Zhou, X. Sun, F. Guo and J. Xiao, Cell Death Dis., 2020, 11, 1–18 CrossRef PubMed.
- U. Harre, D. Georgess, H. Bang, A. Bozec, R. Axmann, E. Ossipova, P.-J. Jakobsson, W. Baum, F. Nimmerjahn, E. Szarka, G. Sarmay, G. Krumbholz, E. Neumann, R. Toes, H.-U. Scherer, A. I. Catrina, L. Klareskog, P. Jurdic and G. Schett, J. Clin. Invest., 2012, 122, 1791–1802 CrossRef CAS PubMed.
- A. Krishnamurthy, V. Joshua, A. H. Hensvold, T. Jin, M. Sun, N. Vivar, A. J. Ytterberg, M. Engström, C. Fernandes-Cerqueira, K. Amara, M. Magnusson, G. Wigerblad, J. Kato, J. M. Jiménez-Andrade, K. Tyson, S. Rapecki, K. Lundberg, S.-B. Catrina, P.-J. Jakobsson, C. Svensson, V. Malmström, L. Klareskog, H. Wähämaa and A. I. Catrina, Ann. Rheum. Dis., 2016, 75, 721–729 CrossRef CAS PubMed.
- M. Zamanpoor, Clin. Genet., 2019, 95, 547–557 CrossRef CAS PubMed.
-
V. Taneja, in The Autoimmune Diseases, ed. N. R. Rose and I. R. Mackay, Academic Press, 6th edn, 2020, pp. 467–489 Search PubMed.
- L. Liu, F. Hu, H. Wang, X. Wu, A. S. Eltahan, S. Stanford, N. Bottini, H. Xiao, M. Bottini, W. Guo and X.-J. Liang, ACS Nano, 2019, 13, 5036–5048 CrossRef CAS PubMed.
- F. U. Rehman, C. Zhao, C. Wu, X. Li, H. Jiang, M. Selke and X. Wang, Nano Res., 2016, 9, 3305–3321 CrossRef CAS.
- I. M. Oliveira, C. Gonçalves, R. L. Reis and J. M. Oliveira, Nano Res., 2018, 11, 4489–4506 CrossRef CAS.
- Q. Wang, Y. Li, X. Chen, H. Jiang, Z. Zhang and X. Sun, Nano Res., 2019, 12, 421–428 CrossRef CAS.
- Y. He, R. Li, J. Liang, Y. Zhu, S. Zhang, Z. Zheng, J. Qin, Z. Pang and J. Wang, Nano Res., 2018, 11, 6086–6101 CrossRef CAS.
- G. Adami and K. G. Saag, Curr. Rheumatol. Rep., 2019, 21, 34 CrossRef PubMed.
- N. Komatsu and H. Takayanagi, Front. Immunol., 2012, 3, 77 Search PubMed.
- A. Scardapane, L. Breda, M. Lucantoni and F. Chiarelli, Int. J. Rheumatol., 2012, 2012, e756291 Search PubMed.
- S. Bua, L. Di Cesare Mannelli, D. Vullo, C. Ghelardini, G. Bartolucci, A. Scozzafava, C. T. Supuran and F. Carta, J. Med. Chem., 2017, 60, 1159–1170 CrossRef CAS PubMed.
- D. M. Schwartz, Y. Kanno, A. Villarino, M. Ward, M. Gadina and J. J. O'Shea, Nat. Rev.
Drug Discovery, 2017, 16, 843–862 CrossRef CAS PubMed.
- E. Nikiphorou, M. H. Buch and K. L. Hyrich, Nat. Rev. Rheumatol., 2017, 13, 503–510 CrossRef PubMed.
- Q. Feng, J. Xu, K. Zhang, H. Yao, N. Zheng, L. Zheng, J. Wang, K. Wei, X. Xiao, L. Qin and L. Bian, ACS Cent. Sci., 2019, 5, 440–450 CrossRef CAS PubMed.
- L. D. Ferguson, S. Siebert, I. B. McInnes and N. Sattar, Nat. Rev. Rheumatol., 2019, 15, 461–474 CrossRef PubMed.
- G. N. Goulielmos, M. I. Zervou, E. Myrthianou, A. Burska, T. B. Niewold and F. Ponchel, Gene, 2016, 583, 90–101 CrossRef CAS PubMed.
- B. R. Hamaker, A. A. Mohamed, J. E. Habben, C. P. Huang and B. A. Larkins, Cereal Chem., 1995, 72, 583–588 CAS.
- A. R. Patel, Adv. Funct. Mater., 2020, 30, 1806809 CrossRef CAS.
- Y. Luo, B. Zhang, M. Whent, L. L. Yu and Q. Wang, Colloids Surf., B, 2011, 85, 145–152 CrossRef CAS PubMed.
- R. Shukla and M. Cheryan, Ind. Crops Prod., 2001, 13, 171–192 CrossRef CAS.
- J. He, L. Wang, Z. Wei, Y. Yang, C. Wang, X. Han and Z. Nie, ACS Appl. Mater. Interfaces, 2013, 5, 9746–9751 CrossRef CAS PubMed.
- N. Parris, P. H. Cooke and K. B. Hicks, J. Agric. Food Chem., 2005, 53, 4788–4792 CrossRef CAS PubMed.
- S. Quispe-Condori, M. D. A. Saldaña and F. Temelli, LWT – Food Sci. Technol., 2011, 44, 1880–1887 CrossRef CAS.
- X.-Y. Luo, M.-H. Yang, F.-X. Wu, L.-J. Wu, L. Chen, Z. Tang, N.-T. Liu, X.-F. Zeng, J.-L. Guan and G.-H. Yuan, Lupus, 2012, 21, 53–59 CrossRef CAS PubMed.
- S. Shao, J. Si, J. Tang, M. Sui and Y. Shen, Macromolecules, 2014, 47, 916–921 CrossRef CAS.
- Y. Shen, E. Jin, B. Zhang, C. J. Murphy, M. Sui, J. Zhao, J. Wang, J. Tang, M. Fan, E. Van Kirk and W. J. Murdoch, J. Am. Chem. Soc., 2010, 132, 4259–4265 CrossRef CAS PubMed.
- A. G. Sagdicoglu Celep, S. Yilmaz and N. Coruh, Drug Food Anal., 20(3), 692–698, DOI:10.6227/jfda.2012200318.
- W. Xin, L. Zhang, H. Fan, N. Jiang, T. Wang and F. Fu, Eur. J. Pharm. Sci., 2011, 42, 73–80 CrossRef CAS PubMed.
- L. Zhang, F. Fu, X. Zhang, M. Zhu, T. Wang and H. Fan, Neurochem. Int., 2010, 57, 119–127 CrossRef CAS PubMed.
- W. Xin, L. Zhang, F. Sun, N. Jiang, H. Fan, T. Wang, Z. Li, J. He and F. Fu, Phytomedicine, 2011, 18, 272–277 CrossRef CAS PubMed.
- F. Fu, Y. Hou, W. Jiang, R. Wang and K. Liu, World J. Surg., 2005, 29, 1614–1620 CrossRef PubMed , discussion 1621–1622.
- G. Xiao and J. Wei, J. Zhejiang Univ., Sci., B, 2005, 6, 28–32 CrossRef PubMed.
- N. Jiang, W. Xin, T. Wang, L. Zhang, H. Fan, Y. Du, C. Li and F. Fu, Phytomedicine, 2011, 18, 1276–1284 CrossRef CAS PubMed.
- C. R. Sirtori, Pharmacol. Res., 2001, 44, 183–193 CrossRef CAS PubMed.
- R. Holmdahl, R. Bockermann, J. Bäcklund and H. Yamada, Ageing Res. Rev., 2002, 1, 135–147 CrossRef CAS PubMed.
- T. Wang, F. Fu, L. Zhang, B. Han, M. Zhu and X. Zhang, Pharmacol. Rep., 2009, 61, 697–704 CrossRef CAS PubMed.
- Y. Wang, W. G. Zhang, L. Jiang and Q. B. Wang, Chin. J. Biochem. Pharm., 2014, 34, 102–104 CAS.
- Q. Li and L. Wang, China Pharm., 2015, 4515–4518 Search PubMed.
- Z.-W. Li, H. Huang, Y. Qin, K.-Q. Xiao, W.-Z. Luo, Y. Yang and D.-D. Gu, Sichuan Daxue Xuebao, Yixueban, 2014, 45, 147–151 CAS.
- M. M. Ansari, A. Ahmad, A. Kumar, P. Alam, T. H. Khan, G. Jayamurugan, S. S. Raza and R. Khan, Carbohydr. Polym., 2021, 258, 117600 CrossRef CAS PubMed.
- K. Songsurang, K. Siraleartmukul and N. Muangsin, J. Microencapsulation, 2015, 32, 450–459 CrossRef CAS PubMed.
- Y. Liu, R. Lin, L. Ma, H. Zhuang, C. Feng, J. Chang and C. Wu, Appl. Mater. Today, 2020, 19, 100578 CrossRef.
- J. Vašková, A. Fejerčáková, G. Mojžišová, L. Vaško and P. Patlevič, Eur. Rev. Med. Pharmacol. Sci., 2015, 19, 879–886 Search PubMed.
- E. Birben, U. M. Sahiner, C. Sackesen, S. Erzurum and O. Kalayci, World Allergy Organ. J., 2012, 5, 9–19 CrossRef CAS PubMed.
- W. G. Seo, H. O. Pae, G. S. Oh, K. Y. Chai, T. O. Kwon, Y. G. Yun, N. Y. Kim and H. T. Chung, J. Ethnopharmacol., 2001, 76, 59–64 CrossRef CAS PubMed.
- K. de J. F. de Oliveira, C. M. Donangelo, A. V. de Oliveira, C. L. P. da Silveira and J. C. Koury, Cell Biochem. Funct., 2009, 27, 162–166 CrossRef PubMed.
- M. Shukla, K. Gupta, Z. Rasheed, K. A. Khan and T. M. Haqqi, J. Inflammation, 2008, 5, 9 CrossRef PubMed.
- K. Bian, Y. Ke, Y. Kamisaki and F. Murad, J. Pharmacol. Sci., 2006, 101, 271–279 CrossRef CAS PubMed.
- S. Tangvarasittichai, S. Pongthaisong and O. Tangvarasittichai, Indian J. Clin. Biochem., 2016, 31, 68–74 CrossRef CAS PubMed.
- S. Giovannini, G. Onder, R. Liperoti, A. Russo, C. Carter, E. Capoluongo, M. Pahor, R. Bernabei and F. Landi, J. Am. Geriatr. Soc., 2011, 59, 1679–1685 CrossRef PubMed.
- C. Sandler, K. A. Lindstedt, S. Joutsiniemi, J. Lappalainen, T. Juutilainen, J. Kolah, P. T. Kovanen and K. K. Eklund, Inflammation Res., 2007, 56, 230–239 CrossRef CAS PubMed.
- E. Molina-Holgado, S. Ortiz, F. Molina-Holgado and C. Guaza, Br. J. Pharmacol., 2000, 131, 152–159 CrossRef CAS PubMed.
|
This journal is © The Royal Society of Chemistry 2024 |