DOI:
10.1039/C5QO00416K
(Research Article)
Org. Chem. Front., 2016,
3, 368-374
A concise total synthesis of sespenine, a structurally unusual indole terpenoid from Streptomyces†
Received
9th December 2015
, Accepted 13th January 2016
First published on 14th January 2016
Abstract
Sespenine is a structurally unusual indole sesquiterpenoid isolated from endophytic Streptomyces sp. HKI0595. Herein, we report a ten-step (the longest linear sequence) synthesis of this molecule from commercially available materials, on the basis of our first generation synthesis. Sharpless asymmetric epoxidation and Stille–Miyata coupling were used to construct a functionalized epoxy ester, which underwent Ti(III) mediated reductive radical cyclization to give a trans-decalin intermediate with a 2-methoxycarbonylindole side chain. Oxidation of this compound afforded a pair of epimeric 3-hydroxyindolenines, and the major isomer entered a bioinspired cascade of Prins cyclization/Friedel–Crafts/retro Friedel–Crafts under acidic conditions, to furnish the polycyclic core of sespenine. Sespenine analogues bearing different C2 substituents were prepared with similar chemistry. Xiamycin A, a carbazole congener of sespenine, was synthesized from the minor hydroxyindolenine epimer as well.
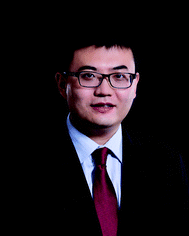 Ang Li | Ang Li was born in Jilin, China, in 1982. In 2004, he received his B.Sc. degree at Peking University, where he worked with Prof. Zhen Yang. He earned his PhD under the supervision of Prof. K. C. Nicolaou at The Scripps Research Institute in 2009. After a brief stay as a research fellow at the Institute of Chemical and Engineering Sciences in Singapore, he joined Shanghai Institute of Organic Chemistry, Chinese Academy of Sciences, as a professor in 2010. His current research is focused on the total synthesis of structurally and biologically interesting natural products. |
Introduction
Indole terpenoids have been of increasing interest in the area of chemical synthesis1–3 and biosynthesis4 because of their fascinating structures and promising biological activities. Recently, we have accomplished total syntheses of a series of indole terpenoids such as those from the anominine, drimentine, mycoleptodiscin, and hapalindole families,5 during which some useful methods and strategies have been developed.6 In 2011, Ding et al. discovered sespenine (1, Fig. 1), a structurally unusual polycyclic molecule from Streptomyces sp. HKI0595,7 a bacterial endophyte of the mangrove Kandelia candel, together with a number of biogenetically relevant indole sesquiterpenoids such as xiamycin A (2) and indosespene (3). Soon after, Zhang and coworkers independently isolated several xiamycin A related natural products.8 This indole terpenoid family quickly drew attention from the perspectives of biosynthesis and chemical synthesis.9 In 2014, we accomplished the first total synthesis of sespenine (1),10 which featured a Lewis acid promoted conjugate addition and a bioinspired cationic cascade reaction. The Baran group disclosed elegant syntheses of xiamycin A (2) and dixiamycin B; the latter possessing an N–N linkage was assembled through an electrochemical oxidative dimerization of the former.11 We then reported syntheses of xiamycin A, indosespene, oridamycins A and B (2–5, Fig. 1), and dixiamycin C12 using two parallel strategies of 6π electrocyclization/aromatization13–15 and indole C2 C–H bond activation/Heck annulation.16 Herein, we report the second generation syntheses of sespenine (1) and xiamycin A (2) as well as the preparation of sespenine analogues with various substituents at C2.
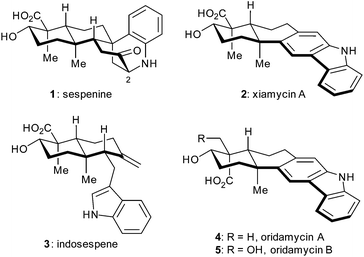 |
| Fig. 1 The structures of sespenine and related indole sesquiterpenoids. | |
Results and discussion
The retrosynthetic analysis of sespenine (1) is illustrated in Scheme 1. The molecule is traced back to an advanced intermediate 6, which may undergo a cascade sequence of Prins cyclization/Friedel–Crafts/retro Friedel–Crafts to reach 1, via the intermediacy of the dearomatized compound 7. It is well precedented that an electron rich arene serves as a suitable nucleophile to trap the carbocation generated through a Prins reaction.17,18 The retro Friedel–Crafts reaction from 7 to 1, which can also be viewed as a retro aldol reaction, would take advantage of the driving force of re-aromatization. Notably, Ding et al. hypothesized a similar reaction cascade in the biosynthetic pathway toward 1,7 and another relevant process was proposed for the biogenesis of aspernomine, a structurally more complex indole diterpenoid, by McWhorter and Tantillo, respectively.19 A subtle change in our plan of chemical synthesis of 1, compared with the above mentioned biosynthetic speculations, is the existence of a C2 substituent (a methoxycarbonyl group in the case), which helps stabilizing the 3-hydroxyindolenine intermediate.20,21 Compound 6 is expected to arise from the precursor 8via chemoselective oxidation of its indole moiety in the presence of an exocyclic C
C bond. trans-Decalin 8 is simplified to α,β-epoxy ester 9. Ti(III) induced epoxide opening radical reaction, which was pioneered by RajanBabu and Gansäuer,22 has been elegantly exploited by the groups of Barrero, Oltra, Fernández-Mateos, Cuerva, Roy, Reisman, etc. in complex molecule synthesis.23 Borrowing from a precedent12 in our synthesis of indosespene (3), we may rely on a reductive opening of the epoxide followed by radical cascade cyclization catalyzed by Ti(III) species in situ generated by Cp2TiCl2 and Mn, to prepare 8 from 9. The indole motif should be tolerated under such conditions. Compound 9 would be disassembled into readily available building blocks allyl acetate 10 and stannane 11, the recombination of which could be effected through allyl Stille–Miyata coupling.24
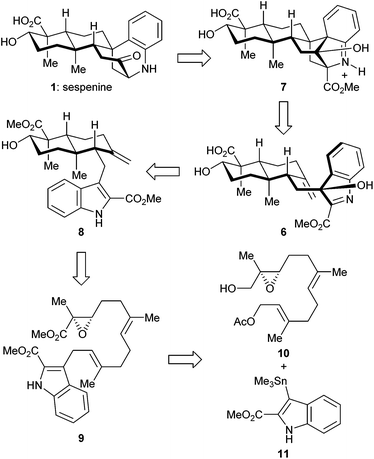 |
| Scheme 1 Retrosynthetic analysis of sespenine. | |
The synthesis of sespenine (1) commenced with the construction of a functionalized epoxy ester 9, as shown in Scheme 2. We first explored the feasibility of Friedel–Crafts type allylation at the C3 position of commercially available methyl indole-2-carboxylate (12). However, these reactions proved to be inefficient under a variety of acidic or basic conditions,25 presumably due to the electron-deficiency of 12. Stannane 11 was then prepared from 12via a two step sequence reported by Routier et al.26 Iodination under basic conditions afforded the corresponding C3 iodide, which was converted to 11 through a Pd-catalyzed stannylation [Pd(PPh3)4, Me3SnSnMe3]. We then synthesized allyl acetate 14, which later served as a coupling partner of 11. Farnesyl acetate (13) was subjected to a two step process of allylic oxidation (SeO2, t-BuOOH) and Sharpless asymmetric epoxidation [Ti(i-PrO)4, (+)-diethyl L-tartrate, t-BuOOH] to reach the epoxy alcohol 10, according to the known literature.27 Although this compound could be oxidized stepwise to give the corresponding carboxylic acid with good overall efficiency, treatment with a catalytic amount of 2-azaadamantane N-oxyl (AZADO, Scheme 2) developed by Iwabuchi28 and stoichiometric PhI(OAc)2 effected the transformation in one pot.29 The resultant carboxylic acid was methylated by using MeI and K2CO3 to afford the ester 14 in 77% yield for the two steps. A variety of conditions for the allyl Stille–Miyata coupling between 14 and 11 were examined. The conditions [Pd2(dba)3, i-Pr2NEt, LiCl] modified from those described by Hegedus and coworkers proved to be optimal for delivering the coupling product 9 (78% yield).24 Notably, the quality of stannane 11 was crucial to this reaction; the freshly prepared stannane was required to ensure a good coupling efficiency.
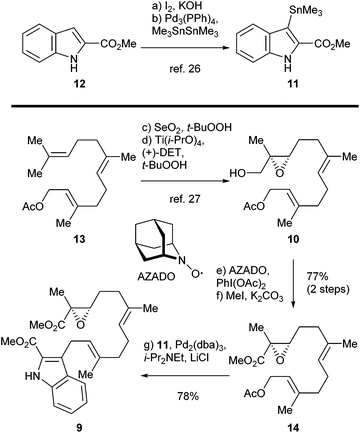 |
| Scheme 2 Synthesis of functionalized α,β-epoxy ester 9. Reagents and conditions: (e) AZADO (4 mol%), PhI(OAc)2 (3.0 equiv.), CH2Cl2, 22 °C, 12 h; (f) MeI (2.0 equiv.), K2CO3 (1.5 equiv.), DMF, 22 °C, 30 min, 77% (2 steps); (g) 11 (1.2 equiv.), Pd2(dba)3 (5 mol%), LiCl (3.0 equiv.), i-Pr2NEt (3.0 equiv.), DMF, 50 °C, 1 h, 78%. | |
We then directed our attention to the Ti(III) mediated radical cascade cyclization for synthesizing 8 from 9. The conditions [Cp2TiCl2, Mn, TMSCl, collidine] developed by Cuerva and coworkers were employed for the initial investigation.23c The anticipated product 8 with an exocyclic C
C bond was obtained with an excellent level of stereochemical control, albeit in less than 20% yield. Over-reduction and deoxygenation, which are illustrated as reaction paths a and b in Scheme 3, respectively, turned out to be severe side reactions accompanied by the desired one. From a mechanistic perspective, the activated C16–O bond of α,β-epoxy ester 9 was cleaved by Ti(III) species generated in situ by Cp2TiCl2 and Mn, to initiate an electron deficient radical 15, which then underwent cascade cyclization. However, this radical could also be trapped by an excess of Ti(III) species to form a Ti(IV) enolate 16;30 similar transformations are well-precedented in the context of SmI2 chemistry.31 Further β elimination of OTi(IV) gave an α,β-unsaturated ester 17 as a byproduct. High concentration of Ti(III) in the reaction system increased the formation of 17. On the other hand, a tertiary radical 18 generated from the tandem cyclization may enter a Ti(III) mediated mix disproportionation pathway to reach the desired product 19,30a or unfortunately terminate via a hydrogen atom transfer to form the byproduct 21.32 Inspired by Cuerva's studies of the amine effect in Ti(III) chemistry,33 we found that i-Pr2NEt was superior to collidine as an additive for suppressing the over-reduction, and strictly anhydrous conditions were critical to inhibit the hydrogen atom transfer. Under our optimized conditions [Cp2TiCl2 (20 mol%), Mn, TMSCl, i-Pr2NEt], compound 8 was isolated in 34% yield (Scheme 4). A suitable initial concentration of Cp2TiCl2 (0.08 M) was important as well. Considering the stereochemistry and functionality outcome of this reaction, such efficiency was acceptable and thus allowed us to carry on the synthesis.
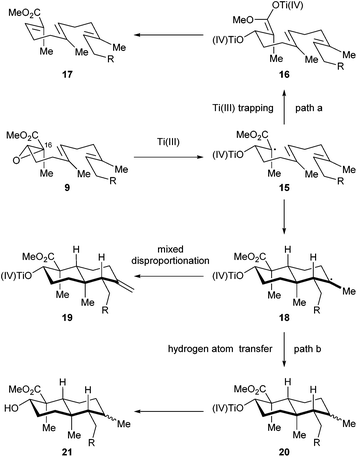 |
| Scheme 3 Postulated pathways of the Ti(III) mediated radical reaction. | |
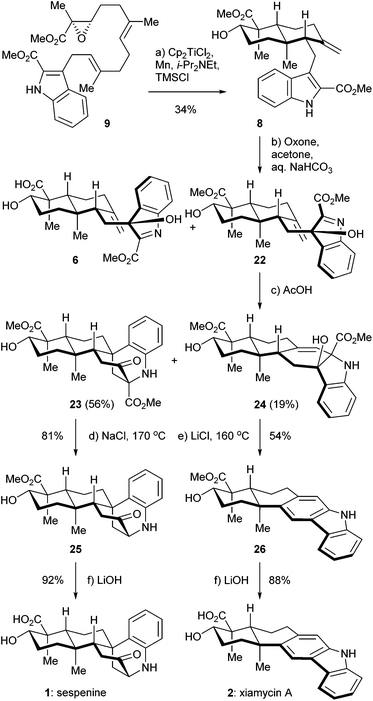 |
| Scheme 4 Total syntheses of sespenine and xiamycin A. Reagents and conditions: (a) Cp2TiCl2 (20 mol%), Mn (8.0 equiv.), i-Pr2NEt (6.0 equiv.), TMSCl (5.0 equiv.), THF, 22 °C, 12 h, 34%; (b) Oxone (2.0 equiv.), acetone/saturated aq. NaHCO3 (1 : 1), 22 °C, 15 min; (c) AcOH (5.0 equiv.), CH2Cl2, 22 °C, 5 h, 56% for 23 (2 steps), 19% for 24 (2 steps); (d) NaCl (10 equiv.), DMSO/water, 170 °C, 2 h, 81%; (e) LiCl (10 equiv.), DMSO/water, 160 °C, 1 h, 51%; (f) aq. LiOH (2.0 M)/THF/MeOH, 50 °C, 10 h, 92% for 1; 88% for 2. | |
With compound 8 in hand, we completed the syntheses of sespenine (1) and xiamycin A (2) through a divergent approach, as depicted in Scheme 4. Treatment of 8 with Oxone in acetone in the presence of aq. NaHCO3 buffer afforded a C3 epimeric mixture of hydroxyindolenine 6 and 22 in a ca. 2.8
:
1 ratio. The structure of 22 was indirectly verified by X-ray crystallographic analysis of its bis-acetylated derivative.34 Exposure of this chromatographically inseparable mixture to AcOH for 5 h at ambient temperature provided compounds 23 and 24, in 56% and 19% yields over the two steps, respectively. The former presumably arose from the devised cascade reaction, while the latter was possibly generated via a straightforward aza-Prins cyclization.5b The Krapcho decarboxylation (NaCl, 170 °C) of 23 delivered sespenine methyl ester 25 in 81% yield,35 the structure of which was confirmed by X-ray crystallographic analysis.34 Under these conditions, the other methoxycarbonyl of the molecule remained untouched. Hydrolysis of 25 with aq. LiOH rendered sespenine (1) smoothly. Interestingly, under similar Krapcho conditions (LiCl, 160 °C), compound 24 was directly converted into xiamycin A methyl ester 26 in 54% yield. This one pot process may involve sequential ester hydrolysis, β-lactone formation, decarboxylation,36 and dehydrogenative aromatization. The cis orientations of the hydroxyl and carboxylic acid functionalities set the geometric basis of β-lactone formation. Basic hydrolysis furnished xiamycin A (2) with good efficiency. The spectra and physical properties of the synthetic sespenine and xiamycin A were consistent with those reported for the naturally occurring samples.
We then investigated the cationic cascade with indole substrates bearing various C2 substituents, as shown in Table 1. Sterically bulky (reverse prenyl) and electron deficient (trifluoromethyl) groups did not interfere with the tandem reaction, which indicates the wide range of stabilizing groups for 3-hydroxyindolenine intermediates. The overall yield of the two step sequence was mainly determined by the efficiency as well as the facial selectivity of the hydroxylation step, and the following cascade reaction was relatively efficient in general. Thus, a number of sespenine analogues (27–30) were readily obtained, setting the stage for further biological studies. Notably, the precursors of these compounds were synthesized through a modified version of our first generation approach (construction of an α,β-unsaturated enone, conjugate addition of indole derivatives, and methylenation; see the ESI† for details), instead of the second generation approach (Stille–Miyata coupling and Ti(III)-mediated cyclization) described in this paper. To assemble one specific indosespene (3) type structure, the latter route was more efficient because of the shorter linear sequence. However, the former route was superior when a collection of such compounds needed to be synthesized for preliminary biological studies. A considerably large quantity of the enone was prepared first, and a variety of readily available indole derivatives were then added to it, to generate different analogues rapidly. In contrast, protocols for preparing 2-substituted-3-stannylindoles varied according to the electronic effect and functional group compatibility, which hampered the collective synthesis via the second generation strategy.
Table 1 Preparation of sespenine analogues through the cationic cascade reaction
Oxone (2.0 equiv.), acetone/saturated aq. NaHCO3 (1 : 1), 22 °C, 15 min.
AcOH (5.0 equiv.), CH2Cl2, 22 °C, 1 h.
|
|
Conclusions
We developed a ten-step (the longest linear sequence) total synthesis of sespenine from commercially available materials based on our first generation synthesis. Stille–Miyata coupling was exploited to construct a functionalized α,β-epoxy ester, and Ti(III) mediated epoxide opening radical cyclization gave a trans-decalin intermediate bearing a 2-methoxycarbonylindole side chain. Indole C3 oxidation afforded a pair of epimeric 3-hydroxyindolenines, the major isomer of which then underwent a cascade of Prins cyclization/Friedel–Crafts/retro Friedel–Crafts to furnish the sespenine core. Xiamycin A was synthesized from the minor hydroxyindolenine epimer. Four analogues of sespenine with various C2 substituents were prepared. This work may facilitate the biosynthetic and biological studies of sespenine and related natural products.
Acknowledgements
This paper is dedicated to Prof. Tohru Fukuyama for his contribution to indole chemistry. We thank Prof. Changsheng Zhang and Dr Qingbo Zhang for helpful discussions. Financial support was provided by the Ministry of Science & Technology (2013CB836900), the National Natural Science Foundation of China (21525209, 21290180, 21172235, and 21222202), Shanghai Science and Technology Commission (15JC1400400), and Chinese Academy of Sciences.
Notes and references
- Selective examples of chemical synthesis of indole monoterpenoids:
(a) H. Muratake and M. Natsume, Tetrahedron, 1990, 46, 6331 CrossRef CAS;
(b) H. Muratake, H. Kumagami and M. Natsume, Tetrahedron, 1990, 46, 6351 CrossRef CAS;
(c) V. Vaillancourt and K. F. Albizati, J. Am. Chem. Soc., 1993, 115, 3499 CrossRef CAS;
(d) T. Fukuyama and X. Chen, J. Am. Chem. Soc., 1994, 116, 3125 CrossRef CAS;
(e) M. Sakagami, H. Muratake and M. Natsume, Chem. Pharm. Bull., 1994, 42, 1393 CrossRef CAS;
(f) A. C. Kinsman and M. A. Kerr, Org. Lett., 2001, 3, 3189 CrossRef CAS PubMed;
(g) A. C. Kinsman and M. A. Kerr, J. Am. Chem. Soc., 2003, 125, 14120 CrossRef CAS PubMed;
(h) P. S. Baran and J. M. Richter, J. Am. Chem. Soc., 2004, 126, 7450 CrossRef CAS PubMed;
(i) P. S. Baran and J. M. Richter, J. Am. Chem. Soc., 2005, 127, 15394 CrossRef CAS PubMed;
(j) P. S. Baran, T. J. Maimone and J. M. Richter, Nature, 2007, 446, 404 CrossRef CAS PubMed;
(k) J. M. Richter, Y. Ishihara, T. Masuda, B. W. Whitefield, T. Llamas, A. Pohjakallio and P. S. Baran, J. Am. Chem. Soc., 2008, 130, 17938 CrossRef CAS PubMed;
(l) T. J. Maimone, Y. Ishihara and P. S. Baran, Tetrahedron, 2015, 71, 3652 CrossRef CAS;
(m) S. E. Reisman, J. M. Ready, A. Hasuoka, C. J. Smith and J. L. Wood, J. Am. Chem. Soc., 2006, 128, 1448 CrossRef CAS PubMed;
(n) A. Chandra and J. N. Johnston, Angew. Chem., Int. Ed., 2011, 50, 7641 CrossRef CAS PubMed;
(o) R. J. Rafferty and R. M. Williams, J. Org. Chem., 2012, 77, 519 CrossRef CAS PubMed;
(p) V. Bhat, K. M. Allan and V. H. Rawal, J. Am. Chem. Soc., 2011, 133, 5798 CrossRef CAS PubMed;
(q) K. M. Allan, K. Kobayashi and V. H. Rawal, J. Am. Chem. Soc., 2012, 134, 1392 CrossRef CAS PubMed;
(r) A. D. Huters, K. W. Quasdorf, E. D. Styduhar and N. K. Garg, J. Am. Chem. Soc., 2011, 133, 15797 CrossRef CAS PubMed;
(s) K. W. Quasdorf, A. D. Huters, M. W. Lodewyk, D. J. Tantillo and N. K. Garg, J. Am. Chem. Soc., 2012, 134, 1396 CrossRef CAS;
(t) A. D. Huters, E. D. Styduhar and N. K. Garg, Angew. Chem., Int. Ed., 2012, 51, 3758 CrossRef CAS PubMed;
(u) E. D. Styduhar, A. D. Huters, N. A. Weires and N. K. Garg, Angew. Chem., Int. Ed., 2013, 52, 12422 CrossRef CAS PubMed;
(v) N. A. Weires, E. D. Styduhar, E. L. Baker and N. K. Garg, J. Am. Chem. Soc., 2014, 136, 14710 CrossRef CAS PubMed.
- Selective examples of chemical synthesis of indole sesquiterpenoids:
(a) E. J. Velthuisen and S. J. Danishefsky, J. Am. Chem. Soc., 2007, 129, 10640 CrossRef CAS PubMed;
(b) I. S. Marcos, R. F. Moro, I. P. Costales, P. Basabe, D. Díez, F. Mollinedo and J. G. Urones, Tetrahedron, 2012, 68, 7932 CrossRef CAS;
(c) I. S. Marcos, R. F. Moro, I. Costales, P. Basabe and D. Díez, Nat. Prod. Rep., 2013, 30, 1509 RSC;
(d) I. S. Marcos, R. F. Moro, I. Costales, P. Basabe, D. Díez, F. Mollinedo and J. G. Urones, Tetrahedron, 2013, 69, 7285 CrossRef CAS;
(e) A. Asanuma, M. Enomoto, T. Nagasawa and S. Kuwahara, Tetrahedron Lett., 2013, 54, 4561 CrossRef CAS.
- Selective examples of chemical synthesis of indole diterpenoids:
(a) A. B. Smith III and R. Mewshaw, J. Am. Chem. Soc., 1985, 107, 1769 CrossRef;
(b) A. B. Smith III, T. Sunazuka, T. L. Leenay and J. Kingery-Wood, J. Am. Chem. Soc., 1990, 112, 8197 CrossRef;
(c) A. B. Smith III, N. Kanoh, H. Ishiyama and R. A. Hartz, J. Am. Chem. Soc., 2000, 122, 11254 CrossRef;
(d) Y. Zou, J. E. Melvin, S. S. Gonzales, M. J. Spafford and A. B. Smith III, J. Am. Chem. Soc., 2015, 137, 7095 CrossRef CAS PubMed;
(e) B. Bradshaw, G. Etxebarria-Jardí and J. Bonjoch, J. Am. Chem. Soc., 2010, 132, 5966 CrossRef CAS PubMed;
(f) A. E. Goetz, A. L. Silberstein, M. A. Corsello and N. K. Garg, J. Am. Chem. Soc., 2014, 136, 3036 CrossRef CAS PubMed;
(g) M. Enomoto, A. Morita and S. Kuwahara, Angew. Chem., Int. Ed., 2012, 51, 12833 CrossRef CAS PubMed;
(h) R. J. Sharpe and J. S. Johnson, J. Am. Chem. Soc., 2015, 137, 4968 CrossRef CAS PubMed;
(i) R. J. Sharpe and J. S. Johnson, J. Org. Chem., 2015, 80, 9740 CrossRef CAS PubMed.
- Selected studies of biosynthesis of indole terpenoids:
(a)
S. E. O'Connor and E. McCoy, Terpene Indole Alkaloid Biosynthesis, in Recent Advances in Phytochemistry, ed. J. T. Romeo, Elsevier, Amsterdam, 2006, vol. 40, pp. 1–22 Search PubMed;
(b) M. Baunach, J. Franke and C. Hertweck, Angew. Chem., Int. Ed., 2015, 54, 2604 CrossRef CAS PubMed;
(c) K. Tagami, C. Liu, A. Minami, M. Noike, T. Isaka, S. Fueki, Y. Shichijo, H. Toshima, K. Gomi, T. Dairi and H. Oikawa, J. Am. Chem. Soc., 2013, 135, 1260 CrossRef CAS;
(d) M. Tang, H. Lin, D. Li, Y. Zou, J. Li, W. Xu, R. Cacho, M. E. Hillenmeyer, N. K. Garg and Y. Tang, J. Am. Chem. Soc., 2015, 137, 13724 CrossRef CAS PubMed.
- Total syntheses of indole terpenoids from our group:
(a) M. Bian, Z. Wang, X. Xiong, Y. Sun, C. Matera, K. C. Nicolaou and A. Li, J. Am. Chem. Soc., 2012, 134, 8078 CrossRef CAS PubMed;
(b) Y. Sun, R. Li, W. Zhang and A. Li, Angew. Chem., Int. Ed., 2013, 52, 9201 CrossRef CAS PubMed;
(c) Z. Lu, M. Yang, P. Chen, X. Xiong and A. Li, Angew. Chem., Int. Ed., 2014, 53, 13840 CrossRef CAS PubMed;
(d) S. Zhou, H. Chen, Y. Luo, W. Zhang and A. Li, Angew. Chem., Int. Ed., 2015, 54, 6878 CrossRef CAS;
(e) Z. Lu, H. Li, M. Bian and A. Li, J. Am. Chem. Soc., 2015, 137, 13764 CrossRef CAS PubMed.
-
(a) S. Zhou, D. Zhang, Y. Sun, R. Li, W. Zhang and A. Li, Adv. Synth. Catal., 2014, 356, 2867 CrossRef CAS;
(b) X. Xiong, D. Zhang, J. Li, Y. Sun, S. Zhou, M. Yang, H. Shao and A. Li, Chem. – Asian J., 2015, 10, 869 CrossRef CAS PubMed;
(c) H. Yu, C. Wan, J. Han and A. Li, Acta Chim. Sin., 2013, 71, 1488 CrossRef CAS.
-
(a) L. Ding, A. Maier, H.-H. Fiebig, W.-H. Lin and C. Hertweck, Org. Biomol. Chem., 2011, 9, 4029 RSC; discovery of relevant natural products:
(b) L. Ding, J. Münch, H. Goerls, A. Maier, H.-H. Fiebig, W.-H. Lin and C. Hertweck, Bioorg. Med. Chem. Lett., 2010, 20, 6685 CrossRef CAS PubMed.
- Q. Zhang, A. Mándi, S. Li, Y. Chen, W. Zhang, X. Tian, H. Zhang, H. Li, W. Zhang, S. Zhang, J. Ju, T. Kurtán and C. Zhang, Eur. J. Org. Chem., 2012, 5256 CrossRef CAS.
-
(a) Z. Xu, M. Baunach, L. Ding and C. Hertweck, Angew. Chem., Int. Ed., 2012, 51, 10293 CrossRef CAS PubMed;
(b) M. Baunach, L. Ding, T. Bruhn, G. Bringmann and C. Hertweck, Angew. Chem., Int. Ed., 2013, 52, 9040 CrossRef CAS PubMed;
(c) M. Baunach, L. Ding, K. Willing and C. Hertweck, Angew. Chem., Int. Ed., 2015, 54, 13279 CrossRef CAS PubMed;
(d) H. Li, Q. Zhang, S. Li, Y. Zhu, G. Zhang, H. Zhang, X. Tian, S. Zhang, J. Ju and C. Zhang, J. Am. Chem. Soc., 2012, 134, 8996 CrossRef CAS PubMed;
(e) Q. Zhang, H. Li, S. Li, Y. Zhu, G. Zhang, H. Zhang, W. Zhang, R. Shi and C. Zhang, Org. Lett., 2012, 14, 6142 CrossRef CAS PubMed;
(f) H. Li, Y. Sun, Q. Zhang, Y. Zhu, S.-M. Li, A. Li and C. Zhang, Org. Lett., 2015, 17, 306 CrossRef CAS PubMed;
(g) A. H. Trotta, Org. Lett., 2015, 17, 3358 CrossRef CAS PubMed.
- Y. Sun, P. Chen, D. Zhang, M. Baunach, C. Hertweck and A. Li, Angew. Chem., Int. Ed., 2014, 53, 9012 CrossRef CAS PubMed.
- B. R. Rosen, E. W. Werner, A. G. O'Brien and P. S. Baran, J. Am. Chem. Soc., 2014, 136, 5571 CrossRef CAS PubMed.
- Z. Meng, H. Yu, L. Li, W. Tao, H. Chen, M. Wan, P. Yang, D. J. Edmonds, J. Zhong and A. Li, Nat. Commun., 2015, 6, 6096 CrossRef CAS PubMed.
- Selected examples of 6π-electrocyclization/aromatization in natural product synthesis:
(a) E. A. Anderson, E. J. Alexanian and E. J. Sorensen, Angew. Chem., Int. Ed., 2004, 43, 1998 CrossRef CAS PubMed;
(b) K. Ohmori, K. Mori, Y. Ishikawa, H. Tsuruta, S. Kuwahara, N. Harada and K. Suzuki, Angew. Chem., Int. Ed., 2004, 43, 3167 CrossRef CAS PubMed;
(c) T. Suzuki, T. Hamura and K. Suzuki, Angew. Chem., Int. Ed., 2008, 47, 2248 CrossRef CAS PubMed;
(d) D. L. Sloman, B. Mitasev, S. S. Scully, J. A. Beutler and J. A. Porco, Angew. Chem., Int. Ed., 2011, 50, 2511 CrossRef CAS PubMed;
(e) D. L. Sloman, J. W. Bacon and J.
A. Porco, J. Am. Chem. Soc., 2011, 133, 9952 CrossRef CAS PubMed;
(f) D. K. Winter, M. A. Endoma-Arias, T. Hudlicky, J. A. Beutler and J. A. Porco, J. Org. Chem., 2013, 78, 7617 CrossRef CAS PubMed;
(g) A. Fürstner, M. M. Domostoj and B. Scheiper, J. Am. Chem. Soc., 2005, 127, 11620 CrossRef PubMed;
(h) T. J. Greshock and R. L. Funk, J. Am. Chem. Soc., 2006, 128, 4946 CrossRef CAS PubMed;
(i) T. J. Greshock and R. L. Funk, Org. Lett., 2006, 8, 2643 CrossRef CAS PubMed;
(j) R. J. Huntley and R. L. Funk, Org. Lett., 2006, 8, 3403 CrossRef CAS PubMed;
(k) R. J. Huntley and R. L. Funk, Org. Lett., 2006, 8, 4775 CrossRef CAS PubMed;
(l) S. T. Staben, J. J. Kennedy-Smith, D. Huang, B. K. Corkey, R. L. LaLonde and F. D. Toste, Angew. Chem., Int. Ed., 2006, 45, 5991 CrossRef CAS.
- Other examples of 6π-electrocyclization/aromatization in natural product synthesis from our group:
(a) Z. Lu, Y. Li, J. Deng and A. Li, Nat. Chem., 2013, 5, 679 CrossRef CAS PubMed;
(b) J. Li, P. Yang, M. Yao, J. Deng and A. Li, J. Am. Chem. Soc., 2014, 136, 16477 CrossRef CAS PubMed;
(c) M. Yang, J. Li and A. Li, Nat. Commun., 2015, 6, 6445 CrossRef CAS PubMed;
(d) M. Wan, M. Yao, J.-Y. Gong, P. Yang, H. Liu and A. Li, Chin. Chem. Lett., 2015, 26, 272 CrossRef CAS;
(e) M. Yang, X. Yang, H. Sun and A. Li, Angew. Chem., Int. Ed. DOI:10.1002/anie.201510568.
- An excellent review of biosynthetic and biomimetic electrocyclizations: C. M. Beaudry, J. P. Malerich and D. Trauner, Chem. Rev., 2005, 105, 4757 CrossRef CAS PubMed.
-
(a) P. S. Baran and E. J. Corey, J. Am. Chem. Soc., 2002, 124, 7904 CrossRef CAS PubMed;
(b) E. M. Ferreira and B. M. Stoltz, J. Am. Chem. Soc., 2003, 125, 9578 CrossRef CAS PubMed;
(c) N. P. Grimster, C. Gauntlett, C. R. A. Godfrey and M. J. Gaunt, Angew. Chem., Int. Ed., 2005, 44, 3125 CrossRef CAS PubMed;
(d) A. Kong, X. Han and X. Lu, Org. Lett., 2006, 8, 1339 CrossRef CAS PubMed;
(e) X. Han and X. Lu, Org. Lett., 2009, 11, 2381 CrossRef CAS.
-
(a) C. Olier, M. Kaafarani, S. Gastaldi and M. P. Bertrand, Tetrahedron, 2010, 66, 413 CrossRef CAS;
(b) X. Han, G. R. Peh and P. E. Floreancig, Eur. J. Org. Chem., 2013, 1193 CrossRef CAS.
- Application of Prins cyclization in natural product synthesis from our group: J. Deng, S. Zhou, W. Zhang, J. Li, R. Li and A. Li, J. Am. Chem. Soc., 2014, 136, 8185 CrossRef CAS PubMed . Also see ref. 5b, c and e.
-
(a) Y. Liu, W. W. McWhorter Jr. and C. E. Hadden, Org. Lett., 2003, 5, 333 CrossRef CAS PubMed;
(b) G. A. Ho, D. H. Nouri and D. J. Tantillo, Tetrahedron Lett., 2009, 50, 1578 CrossRef CAS.
- 2-substituted 3-hydroxyindolenines as natural product motifs:
(a) M. Bittner, M. Silva, E. M. Gopalakrishna, W. H. Watson, V. Zabel, S. A. Matlin and P. G. Sammes, J. Chem. Soc., Chem. Commun., 1978, 79 RSC;
(b) I. R. C. Bick, M. A. Hai and N. W. Preston, Heterocycles, 1983, 20, 667 CrossRef CAS;
(c) D. Ponglux, S. Wongseripipatana, H. Takayama, M. Kikuchi, M. Kurihara, M. Kitajima, N. Aimi and S. Sakai, Planta Med., 1994, 60, 580 CrossRef CAS PubMed.
- Selected examples of syntheses of 2-substituted 3-hydroxyindolenines:
(a) B. Witkop and J. B. Patrick, J. Am. Chem. Soc., 1951, 73, 2188 CrossRef CAS;
(b) T. Hino, H. Yamaguchi, K. Matsuki, K. Nakano, M. Sodeoka and M. Nakagawa, J. Chem. Soc., Perkin Trans. 1, 1983, 141 RSC;
(c) R. Güller and H.-J. Borschberg, Helv. Chim. Acta, 1993, 76, 1847 CrossRef;
(d) J. Éles, G. Kalaus, A. Lévai, I. Greiner, M. Kajtár-Peredy, P. Szabó, L. Szabó and C. Szántay, J. Heterocycl. Chem., 2002, 39, 767 CrossRef;
(e) M. Movassaghi, M. A. Schmidt and J. A. Ashenhurst, Org. Lett., 2008, 10, 4009 CrossRef CAS PubMed;
(f) T. J. Greshock and R. M. Williams, Org. Lett., 2007, 9, 4255 CrossRef CAS PubMed;
(g) T. J. Greshock and R. M. Williams, Org. Lett., 2012, 14, 6377 CrossRef CAS;
(h) S. Liu, J. S. Scotti and S. A. Kozmin, J. Org. Chem., 2013, 78, 8645 CrossRef CAS PubMed;
(i) S. Han, K. C. Morrison, P. J. Hergenrother and M. Movassaghi, J. Org. Chem., 2014, 79, 473 CrossRef CAS PubMed;
(j) X. Qi, H. Bao and U. K. Tambar, J. Am. Chem. Soc., 2011, 133, 10050 CrossRef CAS PubMed;
(k) S. Han and M. Movassaghi, J. Am. Chem. Soc., 2011, 133, 10768 CrossRef CAS PubMed;
(l) S. Liu and X.-J. Hao, Tetrahedron Lett., 2011, 52, 5640 CrossRef CAS;
(m) F. Kolundzic, M. N. Noshi, M. Tjandra, M. Movassaghi and S. J. Miller, J. Am. Chem. Soc., 2011, 133, 9104 CrossRef CAS PubMed.
-
(a) W. A. Nugent and T. V. RajanBabu, J. Am. Chem. Soc., 1988, 110, 8561 CrossRef CAS;
(b) T. V. RajanBabu and W. A. Nugent, J. Am. Chem. Soc., 1994, 116, 986 CrossRef CAS;
(c) A. Gansäuer, M. Pierobon and H. Bluhm, Angew. Chem., Int. Ed., 1998, 37, 101 CrossRef;
(d) A. Gansäuer, H. Bluhm and M. Pierobon, J. Am. Chem. Soc., 1998, 120, 12849 CrossRef;
(e) A. Gansäuer and S. Narayan, Adv. Synth. Catal., 2002, 344, 465 CrossRef;
(f) A. Gansäuer, J. Justicia, C.-A. Fan, D. Worgull and F. Piestert, Top. Curr. Chem., 2007, 279, 25 CrossRef.
-
(a) A. Fernández-Mateos, E. Martin de la Nava, G. Pascual Coca, A. Ramos Silva and R. Rubio González, Org. Lett., 1999, 1, 607 CrossRef;
(b) A. F. Barrero, J. M. Cuerva, M. M. Herrador and M. V. Valdivia, J. Org. Chem., 2001, 66, 4074 CrossRef CAS PubMed;
(c) J. Justicia, A. Rosales, E. Buñuel, J. L. Oller-López, M. Valdivia, A. Haïdour, J. E. Oltra, A. F. Barrero, D. J. Cárdenas and J. M. Cuerva, Chem. – Eur. J., 2004, 10, 1778 CrossRef CAS PubMed;
(d) J. Justicia, J. E. Oltra and J. M. Cuerva, J. Org. Chem., 2004, 69, 5803 CrossRef CAS PubMed;
(e) J. Justicia, J. L. Oller-López, A. G. Campaña, J. E. Oltra, J. M. Cuerva, E. Buñuel and D. J. Cárdenas, J. Am. Chem. Soc., 2005, 127, 14911 CrossRef CAS PubMed;
(f) J. Justicia, J. E. Oltra and J. M. Cuerva, J. Org. Chem., 2005, 70, 8265 CrossRef CAS PubMed;
(g) A. F. Barrero, J. F. Quílez del Moral, E. M. Sánchez and J. F. Arteaga, Eur. J. Org. Chem., 2006, 7, 1627 CrossRef;
(h) J. M. Cuerva, J. Justicia, J. L. Oller-López and J. E. Oltra, Top. Curr. Chem., 2006, 264, 63 CrossRef CAS;
(i) J. Justicia, L. Álvarez de Cienfuegos, A. G. Campaña, D. Miguel, V. Jakoby, A. Gansäuer and J. M. Cuerva, Chem. Soc. Rev., 2011, 40, 3525 RSC;
(j) S. P. Morcillo, D. Miguel, A. G. Campaña, L. Álvarez de Cienfuegos, J. Justicia and J. M. Cuerva, Org. Chem. Front., 2014, 1, 15 RSC;
(k) S. P. Morcillo, D. Miguel, S. Resa, A. Martín-Lasanta, A. Millán, D. Choquesillo-Lazarte, J. M. García-Ruiz, A. J. Mota, J. Justicia and J. M. Cuerva, J. Am. Chem. Soc., 2014, 136, 6943 CrossRef CAS PubMed;
(l) J. Y. Cha, J. T. S. Yeoman and S. E. Reisman, J. Am. Chem. Soc., 2011, 133, 14964 CrossRef CAS PubMed;
(m) J. T. S. Yeoman, V. W. Mak and S. E. Reisman, J. Am. Chem. Soc., 2013, 135, 11764 CrossRef CAS PubMed;
(n) P. K. Mandal, G. Maiti and S. C. Roy, J. Org. Chem., 1998, 63, 2829 CrossRef CAS;
(o) S. C. Roy, K. K. Rana and C. Guin, J. Org. Chem., 2002, 67, 3242 CrossRef CAS PubMed.
- L. Del Valle, J. K. Stille and L. S. Hegedus, J. Org. Chem., 1990, 55, 3019 CrossRef CAS.
-
(a) Z. Liu, L. Liu, Z. Shafiq, D. Wang and Y.-J. Chen, Lett. Org. Chem., 2007, 4, 256 CrossRef CAS;
(b) X. Zhu and A. Ganesan, J. Org. Chem., 2002, 67, 2705 CrossRef CAS PubMed;
(c) M. Westermaier and H. Mayr, Org. Lett., 2006, 8, 4791 CrossRef CAS PubMed.
- A. Bourderioux, A. Ouach, V. Bénéteau, J. Mérour and S. Routier, Synthesis, 2010, 783 CAS.
- J. Lan, Z. Liu, H. Yuan, L. Peng, W. Li, Y. Li, Y. Li and A. C. Chan, Tetrahedron Lett., 2000, 41, 2181 CrossRef CAS.
-
(a) M. Shibuya, M. Tomizawa, I. Suzuki and Y. Iwabuchi, J. Am. Chem. Soc., 2006, 128, 8412 CrossRef CAS PubMed;
(b) Y. Iwabuchi, Chem. Pharm. Bull., 2013, 61, 1197 CrossRef CAS PubMed.
- T. Kuranaga, Y. Sesoko, K. Sakata, N. Maeda, A. Hayata and M. Inoue, J. Am. Chem. Soc., 2013, 135, 5467 CrossRef CAS PubMed.
-
(a) J. Justicia, T. Jiménez, S. P. Morcillo, J. M. Cuerva and J. E. Oltra, Tetrahedron, 2009, 65, 10837 CrossRef CAS;
(b) C. Hardouin, F. Chevallier, B. Rousseau and E. Doris, J. Org. Chem., 2001, 66, 1046 CrossRef CAS PubMed.
- K. C. Nicolaou, S. P. Ellery and J. S. Chen, Angew. Chem., Int. Ed., 2009, 48, 7140 CrossRef CAS PubMed.
-
(a) J. M. Cuerva, A. G. Campaña, J. Justicia, A. Rosales, J. L. Oller-López, R. Robles, D. J. Cárdenas, E. Buñuel and J. E. Oltra, Angew. Chem., Int. Ed., 2006, 45, 5522 CrossRef CAS PubMed;
(b) T. V. Chciuk and R. A. Flowers II, J. Am. Chem. Soc., 2015, 137, 11526 CrossRef CAS PubMed.
- M. Paradas, A. G. Campaña, T. Jiménez, R. Robles, J. E. Oltra, E. Buñuel, J. Justicia, D. J. Cárdenas and J. M. Cuerva, J. Am. Chem. Soc., 2010, 132, 12748 CrossRef CAS PubMed.
- CCDC 987752 (bis-acetylated 22) and 949307 (25) contain the supplementary crystallographic data for this paper.
- A. P. Krapcho, ARKIVOC, 2007, (ii), 54 Search PubMed.
- A. Pommier and J.-M. Pons, Synthesis, 1993, 441 CrossRef CAS.
Footnote |
† Electronic supplementary information (ESI) available: 1H and 13C NMR spectra of all new compounds. See DOI: 10.1039/c5qo00416k |
|
This journal is © the Partner Organisations 2016 |
Click here to see how this site uses Cookies. View our privacy policy here.