DOI:
10.1039/C7RA00868F
(Paper)
RSC Adv., 2017,
7, 25753-25764
2,3-Disubstituted-1,4-naphthoquinones containing an arylamine with trifluoromethyl group: synthesis, biological evaluation, and computational study†
Received
20th January 2017
, Accepted 18th April 2017
First published on 12th May 2017
Abstract
Antibacterial and antifungal organic compounds are becoming increasingly important for biomedical applications. This study deals with the synthesis, characterization of structures, in silico PASS prediction, and the discovery of antibacterial and antifungal properties based on new sulfanyl-1,4-naphthoquinone derivatives containing an arylamine with a trifluoromethyl group at different positions, which can be further applied in drug discovery and development. The in vitro antimicrobial potential of the newly synthesized compounds was evaluated in a panel of seven bacterial strains (three Gram-positive and four Gram-negative bacteria) and one yeast, with an additional study on antibiofilm activities. The compounds (5b and 5e) were identified as having strong antibacterial efficiency against the human-originated pathogen S. epidermidis, with minimal inhibitory concentration values (4.88 and 2.44 μg mL−1, respectively). The toxicity of both compounds (5b and 5e) was studied in detail to compare these compounds with Cefuroxime (a clinically proven drug). The antibacterial activity of the compound 5f was equal to that of Cefuroxime. Moreover, three compounds (5b, 5e, and 5f) exhibited excellent antibacterial activity, and 5b and 5e were two and four times more active than the reference antimicrobial compound (Cefuroxime), respectively. For this reason, these three compounds (5b, 5e, and 5f) are being considered as promising antibacterial agents. In addition, docking studies were used to better rationalize the action and prediction of the binding modes of these compounds.
1. Introduction
Although there has been a decline in the incidence of infectious diseases, there is no place in the world where infectious diseases are an insignificant cause of illness and death.1,2 The number of deaths caused by pathogens and parasites have shown a minor decline of only 1% per year. Over 10 million people have died from infections since 1990.1a,2 The World Health Organization (WHO) estimates 13 million deaths from infectious diseases in 2050.2 People in low- and middle-income countries suffer from diseases such as HIV/AIDS, tuberculosis, malaria, other pathogens, and infections that have been causing the majority of deaths since 2010.1a,2
Infectious diseases are among the most serious threats of the “top global health risk factors”, just like the high risk factors of chemical spills and nuclear accidents.1b,c,2,3 WHO has, therefore, provided effective global strategies for the control of major infectious diseases that are being dubbed as the “infectious diseases control programs”, which are necessary in order to help build these health systems.4
The fact that the resistance of bacterial and fungal pathogens leads to an ever-increasing global public health threat reflects the widespread and growing demand for new antibacterial and antifungal compounds, which are among the most prevalently used drugs.5 Unfortunately, very few new classes of antibacterial drugs have been registered for clinical practice in the past 50 years.6 Not surprisingly, this has recently caused a rapidly growing interest in the synthesis of new and efficient antibacterial and antifungal compounds that may be drug candidates for reducing infections caused by pathogens.7
The antimicrobial activities of an increasing number of quinone compounds are being investigated for the discovery of new antibacterial and antifungal compounds.7,8 A series of sulfanyl-1,4-naphthoquinone derivatives was synthesized and potent antifungal activity was exhibited in vitro by one compound, compared to the clinically proven antifungal drug fluconazole, against S. schenckii, and the clinically proven drug amphotericin-B against T. mentagraphytes.9 1,4-Naphthoquinones containing an amino group have been used as antibacterials, antimalarials, antituberculars, larvicides and molluscicides, herbicides, and fungicides in many medical and biological applications.10–14 A series of phenylamino-1,4-naphthoquinones was synthesized to investigate their antitumor effects against cancer cell lines and healthy fibroblasts, and it was reported that the presence of a chlorine atom in the acceptor quinone nucleus and/or the presence of a methyl group at the nitrogen atom of the donor phenylamino group induced changes in cytotoxic activity.15
Since the trifluoromethyl group (–CF3) is one of the most important substituents in organic chemistry because of its interesting stereoelectronic profile,16 our attention was turned to the naphthoquinone compounds containing the –CF3 on the arylamines at the o-, m-, and p-positions, in light of previous studies.17 The −CF3 has an effect based on the different electron density distributions on the reactivity of the molecule. The efficiency of –CF3 on the physiological activity is a very significant topic in pharmaceutical studies. The physiological profile is dependent on the position of –CF3 within the bioactive molecules.16
A series of naphthoquinone derivatives has been synthesized and tested for their biological activity against human African trypanosomiasis, normally known as sleeping sickness, a dangerous and often ignored parasitic disease.17 The activities of some compounds have been compared to evaluate the importance of the position of the –CF3 group on the phenyl amine ring and its substitution that caused by the inhibition of T. brucei cell proliferation. Compounds shown in Fig. 1 with –CF3 (electron withdrawing group) at different positions on the phenyl amine ring showed T. brucei inhibitory activity with average cytotoxicity. It was found that the presence of a chlorine atom instead of a second arylamine led to an increase in the activity. Additionally, changing the –CF3 from the para position to the ortho position led to an increase in the activity. It was reported that the reason for this enhancement in T. brucei inhibitory activity might have been the strong electron withdrawing groups (–CF3, –NO2, and Cl) within the structure; replacing the –CF3 with the methyl group at different positions led to activity loss. In this study, it can be clearly seen that the substitution patterns (2nd and 4th positions) on aniline and monoamino- or diamino-substituted compounds affect the T. brucei inhibitory activity.17
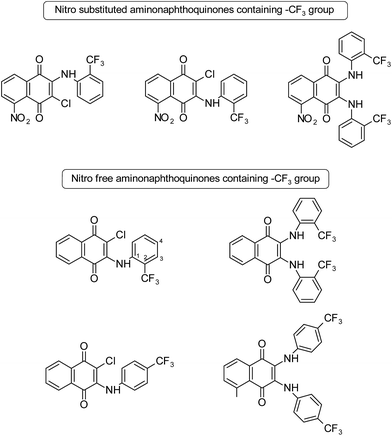 |
| Fig. 1 Structures of some nitro free and nitro substituted aminonaphthoquinones.17 | |
Sulfanyl aminonaphthoquinone and aminonaphthoquinone derivatives have important applications in medicinal chemistry as biologically active substances. Among them, some most striking examples are shown in Fig. 2. Tandon et al. reported the synthesis of some sulfanyl aminonaphthoquinone compounds (I–IV) and their biological evaluation. Some of these compounds showed significant antibacterial activity.7c,d Very recently, another research study on the synthesis of the sulfanyl arylamino quinone compounds was reported by Ryu et al. Some of the tested compounds (V and VI) completely inhibited fungal growth when compared to the Candida species.8a Tandon et al. synthesized other series of sulfanyl aminonaphthoquinone and aminonaphthoquinone derivatives, and the synthesized compounds were tested for antibacterial and antifungal activities. Particularly, sulfanyl aminonaphthoquinone derivatives containing the arylsulfanyl group did not exhibit any significant activity (VII). In this study, the antifungal activity was observed to decrease when the 2,3-disubstituted moiety was altered by the phenyl group.8b
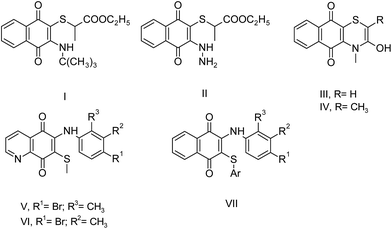 |
| Fig. 2 Structures of some important sulfanyl aminonaphthoquinones with biological activity in literature. | |
There are several factors that affect the biological evaluation of the quinone structures, which are listed as follows: (a) a naphthoquinoidal moiety; (b) an aromatic ring such as aniline; (c) the presence and position of substituents on the phenyl amine ring, and insertion of additional electronegative atoms (Cl, S, N etc.).15,17,18 Encouraged by all these facts, and in order to continue our previous studies, which were mainly about the synthesis of biologically active 1,4-naphthoquinone structures, we chose our compounds by (i) using sulfanyl derivatives of arylamino-1,4-naphthoquinone as the core structure; (ii) using arylamine ring containing the CF3 group at different positions (o-, m-, and p-); (iii) using different types of thiols, as shown in Fig. 3. From this point of view, profound pharmacological effects of related compounds in previous studies gave us clues regarding the synthesis, characterization, and evaluation of some new sulfanyl-1,4-naphthoquinone derivatives containing an arylamine with the –CF3 at different positions, as antimicrobial agents.
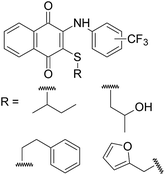 |
| Fig. 3 Structures of the sulfanyl derivatives of 1,4-naphthoquinone based on aryl amines containing −CF3 group. | |
2. Results and discussion
2.1. The synthesis of substituted naphthoquinones
Herein, the synthesis of 3-arylamino-1,4-naphthoquinone derivatives (3a–c) was carried out via the reactions of 2,3-dichloro-1,4-naphthoquinone (1) and primary arylamines (2a–c) containing an electron withdrawing substituent (–CF3) on the rings at the o-, m-, and p-positions as the starting materials in different mediums.17,19,27 Unlike the synthesis of 3a and 3b reported previously in the scientific literature, compound 3c was obtained in low yields in DMF in the presence of K2CO3 (10%). The synthesis of new sulfanyl 1,4-naphthoquinone derivatives containing an arylamine with the –CF3 at different positions (5a–k) was achieved at room temperature via the reaction of 3-arylamino-1,4-naphthoquinone derivatives (3a–c) with a variety of thiols (4a–d) in CH2Cl2 in the presence of Et3N (1.1 eq.) as a base (shown in Scheme 1) in high yields.8 Additionally, compound 6 was obtained as a side-product from the reaction of 2-chloro-3-((4-(trifluoromethyl)phenyl)amino)naphthalene-1,4-dione (3a) with butane-2-thiol (4a). Among the synthesized compounds, the sulfanyl 1,4-naphthoquinone derivatives (5a–k) and the side product (6) are new, while the arylamino-1,4-naphthoquinone derivatives (3a–c) have been reported in earlier studies.17,19,27 New sulfanyl 1,4-naphthoquinone derivatives containing an arylamine with the –CF3 at different positions (5a–k) were obtained in the range of 30–85% yield as orange and red powders or oils. Spectroscopic methods like one dimensional NMR techniques (1H, 13C), FTIR spectra and mass spectrometry were applied to confirm the structures of obtained compounds. The FTIR spectra of 5a–k and 6 showed some characteristic absorptions around 3275–3346 cm−1 (NH), 1657–1676 cm−1 (C
O), 3419–3433 (OH) cm−1, 2851–2978 cm−1 (CHaliphatic) and 3024–3076 cm−1 (CHaromatic). The 1H NMR spectra of 5a–k and 6 (CDCl3-d1) exhibited the NH signal as a singlet between 7.64–7.93 ppm in the aromatic region and at 5.86–8.09 ppm corresponding to the aromatic protons. The signals associated with the other functional groups all appeared in the expected regions. Outstandingly, the presence of two peaks for the carbonyl groups (–C
O) in 13C NMR spectra was the proof of the two substituents (amino and sulfanyl groups) attached to the naphthoquinone core. There was only one peak for the carbonyl group in the 13C NMR spectrum of compound 6 because of the symmetry.
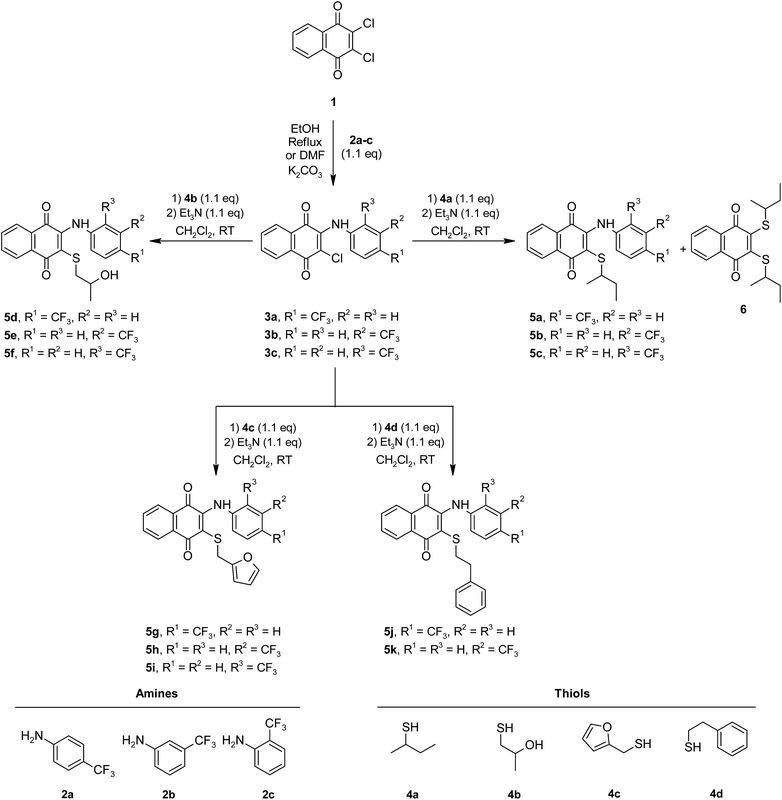 |
| Scheme 1 Synthetic pathway for the preparation of new sulfanyl 1,4-naphthoquinone derivatives substituted with arylamines containing the −CF3 at o-, m-, and p-positions. | |
2.2. The prediction of biological activity based on spectra
The prediction of biological activity based on the spectral analysis of the synthesized compounds in the present communication was easily carried out by using the freely available website with the internet version of PASS and PharmaExpert: http://www.pharmaexpert.ru/PASSOnline/.20,21 The activity spectrum for a molecule is a list of biological activity for which the probability of the compound being active (Pa) and the probability of the compound being inactive (Pi) are calculated. Pa and Pi values are independent and their values vary from 0 to 1. For example, the PASS prediction results for one of the compound (5e) are summarized in Table 1.
Table 1 PASS prediction results for compound (5e)
Pa |
Pi |
Activity |
0.625 |
0.042 |
TP53 expression enhancer |
0.589 |
0.094 |
Membrane permeability inhibitor |
0.426 |
0.027 |
Pyruvate decarboxylase inhibitor |
0.336 |
0.001 |
CDC25 phosphatase inhibitor |
0.285 |
0.201 |
Aspartyltransferase inhibitor |
0.233 |
0.173 |
Anti-infective |
0.173 |
0.070 |
Protein synthesis inhibitor |
0.179 |
0.144 |
Phosphatidylcholine-sterol O-acyltransferase inhibitor |
0.158 |
0.045 |
Antibacterial, ophthalmic |
0.138 |
0.025 |
Antibiotic anthracycline-like |
It was found that for antibacterial and anti-infective activity, the compounds had Pa nearly equal to 0.2. These findings also showed some good experimental results and can be considered to be valuable while planning the experiment, but some important factors should be taken into account such as the particular interest in some kind of activity, desirable novelty, the available facilities for experimental testing, etc.
2.3. The antimicrobial activity
All the newly synthesized sulfanyl 1,4-naphthoquinone derivatives containing an arylamine with the –CF3 at different positions (5a–k) and 2,3-disulfanyl 1,4-naphthoquinone (6) were evaluated for their in vitro antibacterial and antifungal activities. The antimicrobial assay results for all the newly synthesized 1,4-naphthoquinone structures (5a–k and 6) are given in Table 2.
Table 2 In vitro antibacterial and antifungal activity results for the synthesized compounds
|
|
Microorganisms |
Gram-negative bacteria |
Gram-positive bacteria |
Fungi |
P. aeruginosa |
E. coli |
K. pneumoniae |
P. mirabilis |
S. aureus |
S. epidermidis |
E. faecalis |
C. albicans |
MIC values (μg mL−1) |
5a |
— |
— |
— |
— |
— |
— |
— |
— |
5b |
— |
— |
— |
— |
— |
4.88 |
— |
— |
5c |
— |
625 |
312.5 |
— |
— |
1250 |
— |
— |
5d |
— |
— |
— |
312.5 |
— |
156.2 |
312.5 |
— |
5e |
— |
— |
— |
— |
— |
2.44 |
625 |
— |
5f |
— |
— |
— |
— |
— |
9.76 |
— |
— |
5g |
— |
— |
625 |
625 |
625 |
156.2 |
625 |
— |
5h |
— |
— |
— |
— |
— |
— |
— |
— |
5i |
— |
— |
— |
— |
— |
1250 |
— |
— |
5j |
— |
— |
— |
— |
— |
— |
625 |
312.5 |
5k |
— |
— |
— |
— |
— |
— |
— |
312.5 |
6 |
— |
— |
— |
— |
— |
78.12 |
— |
— |
Reference antimicrobials |
2.4 Ceftazidime |
4.9 Cefuroxime-Na |
4.9 Cefuroxime-Na |
2.4 Cefuroxime-Na |
1.2 Cefuroxime-Na |
9.8 Cefuroxime |
128 Amikacin |
4.9 Clotrimazole |
In vitro activities of the studied molecules against planktonic cells are summarized in Table 2. Concerning the antibacterial activity, the results revealed that some compounds displayed varying effects on the growth of the tested Gram-positive and Gram-negative bacterial strains. As indicated in Table 2, among the synthesized compounds of 5b–g and 6, they generally showed potent antibacterial activity against some pathogenic strains that were tested. Actually, the activities of compounds 5b and 5e were superior as compared to that of Cefuroxime: 5b and 5e completely inhibited the growth of S. epidermidis, and were tested at the MIC level of 4.88 and 2.44 μg mL−1, respectively. In addition to these, the activity of compound 5f was equal to that of Cefuroxime. In general, most of the tested compounds showed better antibacterial activity against the Gram-positive bacteria compared to the Gram-negative ones. The results revealed that compounds 5d and 5g exhibited moderate activity against Gram-positive bacteria. The test-cultures P. aeruginosa, E. coli, and S. aureus appeared non-effective to most of the synthesized compounds. Some of the synthesized compounds (5d–e, 5g and 5j) possessed activity against E. faecalis, which had MIC values between 312.5–625 μg mL−1. In addition to E. faecalis, the compounds (5d and 5g) possessed activity against S. epidermidis, which had the MIC value of 156.2 μg mL−1. 2,3-Disulfanyl 1,4-naphthoquinone (6) was only able to induce appreciable growth inhibitory activity against S. epidermidis, which had the MIC value of 78.12 μg mL−1. The compounds (5a–b, 5e–f, and 5h–k) exhibited no antibacterial activity against the Gram-negative bacteria. Regarding the antifungal activity of the tested compounds, only 2 compounds (5j and 5k) were able to induce appreciable growth inhibitory activity against C. albicans.
To evaluate the importance of the position of the –CF3 on the phenyl amine ring with respect to the biological efficiency, the activities of all the synthesized compounds were compared (Scheme 1). The decrease in the antibacterial activities of the compounds with the –CF3 at the p-positions on the phenylamine ring, (5a, 5d, 5g, and 5j) was the proof of an important factor for the antibacterial activity. In terms of the structure–activity relationship, the sulfanyl 1,4-naphthoquinone derivatives containing an arylamine with the –CF3 at the m-positions (5b, 5e, 5h, and 5k) showed, in general, more potent antibacterial activities than the other sulfanyl 1,4-naphthoquinone derivatives with an arylamine containing the –CF3 at the p-positions (5a, 5d, 5g, and 5j). The sulfanyl 1,4-naphthoquinone derivatives containing an arylamine with the –CF3 at the o-positions (5c, 5f, and 5i) showed, in general, more potent antibacterial activity than other sulfanyl 1,4-naphthoquinone derivatives with an arylamine containing the –CF3 at the p-position, but unfortunately, not better than other sulfanyl 1,4-naphthoquinone derivatives with an arylamine containing the –CF3 at the m-positions. The replacement of a halogen with the different types of sulfanyl derivatives led to variable types of activities. However, aliphatic substitutions like sec-butylthio and 2-hydroxypropylthio moieties of the compounds (5b–f) in comparison to aralkyl or heteroaryl substitutions (5g, 5h, and 5k) may contribute partially toward biological potency with the additional effect of the position of –CF3. Moreover, this particular modification does not significantly affect the antifungal activity. Indeed, the aralkyl derivatives (5j and 5k) showed moderate inhibition effect against C. albicans; this leads to the conclusion that the moiety may be a factor affecting the antimicrobial activity of the synthesized sulfanyl 1,4-naphthoquinone derivatives. In this study, when the in vitro antimicrobial activities of all the newly synthesized sulfanyl 1,4-naphthoquinone derivatives were investigated, three molecules (5b, 5e, and 5f) had strong antibacterial efficiencies against S. epidermidis, which is usually considered as a common human originated pathogen.
The cytotoxic effects of the two compounds (5b and 5e) were obtained as the IC50 values, which represent the molar drug concentrations required to cause 50% inhibition of cell viability. Consequently, all tested compounds significantly induced dose-dependent loss of viability in HepG2 cells after 24 h. The IC50 values (5b: 19.54 μg mL−1, 5e: 10.18 μg mL−1) for the compounds were four times higher than the MIC results shown in Fig. 4 and 5.
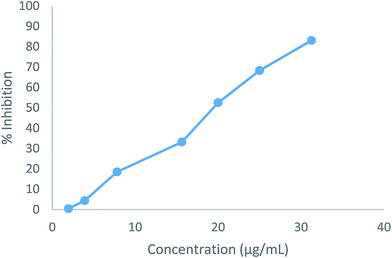 |
| Fig. 4 The effect of the compound 5b on the % inhibition at different concentrations. | |
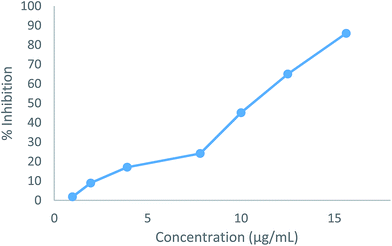 |
| Fig. 5 The effect of the compound 5e on the % inhibition at different concentrations. | |
2.4. Antibiofilm activity
A biofilm is a community of microbes embedded in a self-produced polymeric matrix, which adheres to a surface. Microbial cells that grow in biofilms behave quite differently from their planktonic counterparts. The bacteria on the biofilm structures are protected from the environmental conditions, antimicrobial agents, and host immune responses, and the biofilm organisms are far more resistant to antimicrobial agents than the organisms in the suspension (up to 1000-fold increase).22
When we considered the antibiofilm activities of the antibacterial molecules 5b and 5e against the S. epidermidis biofilms, the MBEC values were 5000 and 1250 μg mL−1, respectively. The MBEC/MIC ratio, which is one of the important parameters for choosing antibiotics in the treatment of biofilm associated infections, was found to be 1024 and 512 fold, respectively, in contrast to many antibiotics (up to 10
000 fold).22a
Since the only biofilm susceptible to the studied active molecules was S. epidermidis, both the biofilm attachment and inhibition of the biofilm formation assays were performed. When these tests were carried out, 5b inhibited the biofilm attachment according to the time, and it showed a significant inhibition activity against the biofilm formation at the 24th hour according to its varying concentrations (Fig. 6). In contrast to these findings, there was an inhibition in the biofilm attachment of 5e at the end of the 2nd hour; following that, as the number of cells increased within the environment, this activity was decreased at the 4th hour.
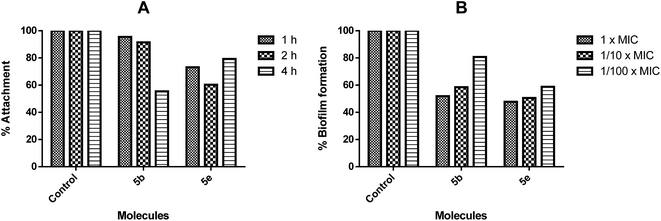 |
| Fig. 6 The inhibition of S. epidermidis. (A) The surface attachment to the wells containing 1/10× MIC of molecules and an inoculum of 1 × 107 cfu per 200 μL, incubated for 1, 2, or 4 h at 37 °C. (B) The biofilm formation in each well containing 1×, 1/10×, or 1/100× MIC of molecules and an inoculum of 5 × 105 cfu per 200 μL, incubated for 24 h at 37 °C. The control bars indicating bacteria without the molecule of interest are accepted as 100%. Six wells were used for each molecule. Each experiment is representative of the two independent tests, and the error bars indicate the standard deviations. All the differences between the control and molecule-treated biofilms are statistically significant (p < 0.001). | |
The biofilms are not affected by “therapeutically achievable concentrations” of antimicrobial agents. The antibiofilm therapies are generally focused on the inhibition of the biofilm formation.22b For this purpose, the inhibitions of bacterial attachment to the surfaces were investigated; the inhibition of the biofilm production at the MIC or subMIC values of molecules were investigated as well. 5b and 5e were able to inhibit the attachment of bacteria at the MIC or subMIC values, and the biofilm formation after 24 hours was approximately 50% (at 1/10× MIC p < 0.001). Since inhibiting a mature biofilm can be very difficult, the inhibition of the biofilm formation in the early stages may seem to be more applicable and advantageous.
2.5. Docking studies
In silico approaches like docking studies are currently gaining much attention to pre-access the probable mechanism for the action and design of new templates towards more potential antibacterial agents.21f The significant experimental antibacterial activities of the compounds 5b and 5e gave us a hint to perform molecular docking studies to understand the protein–ligand interactions. The docking was performed via the Molegro Virtual Docker 2010.4.1.0 program23 for the active site of Staphylococcus epidermidis mevalonate diphosphate decarboxylase (PDB ID: 4DPT) in order to predict the binding mode and support the biological results.24
Mevalonate diphosphate decarboxylase (MDD) catalyzed the formation of isopentenyl 5-diphosphate in an ATP-dependent irreversible reaction and was therefore an attractive target for the inhibitor development that could lead to the new antimicrobial agents.24b In the case of compound 5b, it revealed the MolDock score of −72.77 and formed three interactions, shown as green dotted lines (Fig. 7), which were expressed as hydrogen bonds formed with the oxygen atom of the C
O (naphthoquinone) moiety at the position 4 with Arg 144 and Ala 14, with distances of 2.59 Å and 3.19 Å, respectively and one hydrogen bond formed between the –NH– moiety at the position of 3 and Asn 12 of distance 3.35 Å.
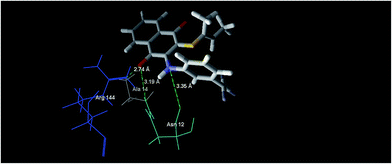 |
| Fig. 7 The binding mode of compound 5b in S. epidermidis mevalonate diphosphate decarboxylase [PDB ID: 4DPT]. | |
In the case of the compound 5e, it revealed the MolDock score of −78.46 and formed the main interactions as depicted in Fig. 8, expressed as one hydrogen bond formed with the oxygen atom of the C
O (naphthoquinone) moiety at the position 4, and bonds with the oxygen atom of the 2-hydroxypropylthio group with Ala 284 and Ser 141, with distances of 3.14 Å and 2.93 Å, respectively.
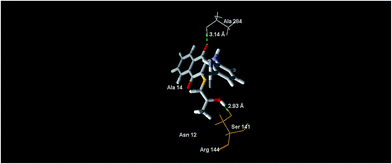 |
| Fig. 8 The binding mode of compound 5e in S. epidermidis mevalonate diphosphate decarboxylase [PDB ID: 4DPT]. | |
Compound 6 revealed the MolDock score of −56.46. The supposed Moldock score was found to be positively correlated with the experimental result values, where the antibacterial activities against S. epidermidis of the compounds of 5e, 5b and 6 decrease from left to right. Interactions with the Arg 144 (catalytic site residue, ligand to mevalonate C1 carboxyl) and Ser 141 (ATP binding motif residue) polar active side chains indicate the binding affinity of the compounds with the protein. This could be further investigated under the topic of MDD inhibitors.25
3. Conclusion
The sulfanyl 1,4-naphthoquinone derivatives, containing an arylamine with the –CF3 at the different positions (5a–k), and 2,3-disulfanyl 1,4-naphthoquinone (6) were synthesized via the nucleophilic substitution of aminonaphthoquinones (3a–c) with 1.1 eq. of aryl- and alkylthiols (4a–d) in CH2Cl2 in the presence of Et3N (1.1 eq.) as a base at the room temperature.8 All of the newly synthesized compounds (5a–k and 6) were tested for their antimicrobial activities. Based on the testing data for the presented compounds, the in vitro antimicrobial activities were evaluated against the different Gram-positive and Gram-negative bacterial strains in addition to the antifungal activities. The antibacterial profile of the synthesized compounds (5a–k) indicated that compounds (5b–k) had potent antibacterial activities. Among the most promising antibacterial compounds, 5b and 5e exhibited better antibacterial activity than clinically prevalent antibacterial drug Cefuroxime against S. epidermidis, while 5f had similar activity. The compounds (5b, 5e, and 5f) are therefore lead compounds as potent antibacterial agents for further studies. The tested compounds (5b and 5e) significantly induced dose-dependent loss of viability in HepG2 cells after 24 h. The IC50 values (5b: 19.54 μg mL−1, 5e: 10.18 μg mL−1) for the compounds were four times higher, compared to the MIC results. These results suggest that the sec-butylthio and 2-hydroxypropylthio moieties with the additional effect of the position of –CF3 are promising leads for the development of antibacterial agents. 5b and 5e were able to inhibit the attachment of bacteria at the MIC or subMIC values and the biofilm formation after 24 hours was approximately 50%, at 1/10× MIC (p < 0.001). The molecular docking studies mentioned above further helped in supporting the experimental results. These experimental findings and structures with the additional efforts will pave the way for more potent biologically active derivatives containing the quinone chromophore.
4. Experimental
4.1. Chemistry
All of the reagents were obtained from the commercial suppliers and were used without further purification unless otherwise noted. Petroleum ether had a boiling range of 40–60 °C. Analytical thin layer chromatography (TLC) plates were purchased from Merck KGaA (silica gel 60 F254) based on Merck DC-plates (aluminum based). Visualization of the chromatogram was performed by UV light (254 nm). Column chromatographic separations were carried out using silica gel 60 (Merck, 63–200 μm particle size, 60–230 mesh). 1H NMR and 13C NMR spectra were recorded with the VarianUNITY INOVA spectrometers with 500 MHz frequency for 1H and 125 MHz frequency for 13C NMR in ppm (δ). 1H NMR spectra and 13C NMR spectra in CDCl3 were referenced to the solvent signals centered at δ 7.19 and δ 76.0 ppm, respectively. Standard abbreviations indicating multiplicity were used as follows: s (singlet), br s (broad singlet), d (doublet), t (triplet), and m (multiplet). Coupling constants J were given in Hz. FTIR spectra were recorded as ATR on either a Thermo Scientific Nicolet 6700 spectrometer or Alpha T FTIR spectrometer. Mass spectra were obtained on either a Thermo Finnigan LCQ Advantage MAX MS/MS spectrometer equipped with ESI (electrospray ionization) sources. The purity of the sulfanyl 1,4-naphthoquinone derivatives was obtained by HPLC analysis. HPLC was performed on a Shimadzu/DGU-20A5 HPLC apparatus fitted with a 25 cm Chiralpac AD-H chiral column. The melting points (mp) were determined with a Buchi B-540 melting point apparatus, but were uncorrected. The 3-arylamino-1,4-naphthoquinone derivatives (3a–c) were prepared via the reaction between the 2,3-dichloro-1,4-naphthoquinone (1) and trifluoromethyl substituted aryl amines (2a–c) according to the literature reported previously and the references cited therein.17,19,26,27 All of the synthesized sulfanyl 1,4-naphthoquinone derivatives (5a–k) and side product (6) are new.
4.1.1. General procedure for synthesis of the sulfanyl 1,4-naphthoquinone derivatives (5a–k). The 3-arylamino-1,4-naphthoquinone derivatives (3a–c) and thiols in CH2Cl2 were stirred at room temperature using Et3N. The resulting solution was extracted with 100 mL chloroform, and then washed with water (4 × 100 mL) and dried over calcium chloride. The solvent was removed in vacuo. The residue was subjected to column chromatography on silica gel using suitable solvents to give the products.
4.1.1.1. 2-(Sec-butylthio)-3-(4-(trifluoromethyl)phenylamino)naphthalene-1,4-dione (5a). This compound was synthesized from 2-chloro-3-((4-(trifluoromethyl)phenyl)amino)naphthalene-1,4-dione (3a) and butane-2-thiol (4a) as a red oil by using the general procedure. Yield: 0.030 g, 52%. FTIR (ATR) ν (cm−1): 3323 (–NH), 3030 (CHarom), 2967, 2929, 2875 (CHaliphatic), 1659 (C
O). 1H NMR (500 MHz, CDCl3) δ (ppm): 8.10–8.08 dd, J: 7.81, 0.98 Hz, 1H (–CHarom); 8.03–8.01 dd, J: 7.81, 0.98 Hz, 1H (–CHarom); 7.75 bs, 1H (–NH); 7.70–7.66 td, J: 7.33, 1.47 Hz, 1H (–CHarom); 7.63–7.60 td, J: 7.81, 1.47 Hz, 1H (–CHarom); 7.51–7.49 d, J: 8.30 Hz, 2H (–CHarom); 6.99–6.97 d, J: 8.30 Hz, 2H (–CHarom); 3.02–2.98 q, J: 6.83 Hz, 1H (–CH–); 1.38–1.28 m, 2H (–CH2–); 0.99–0.97 d, J: 6.83 Hz, 3H (–CH3); 0.71–0.68 t, J: 7.32 Hz, 3H (–CH3). 13C NMR (125 MHz, CDCl3) δ (ppm): 180.2, 179.1 (C
O), 143.4, 140.5 (Cq), 133.6, 132.1, 126.0, 125.7, 120.5 (CHarom), 132.2, 129.7, 124.7 (Cq), 43.3 (–CH–); 28.8 (–CH2–); 19.4, 10.1 (–CH3). MS (ESI−) m/z (%): 402 (100, [M − 3H]+), 403 (22, [M − 2H]+). Anal. calcd for C21H18F3NO2S (405.43).Additionally, 6 was also obtained from 2-chloro-3-((4-(trifluoromethyl)phenyl)amino)naphthalene-1,4-dione (3a) and butane-2-thiol (4a) by using the general procedure.
4.1.1.1.1 2,3-Bis(sec-butylthio)naphthalene-1,4-dione (6).
Red oil, yield: 0.035 g, 37%. FTIR (ATR) ν (cm−1): 2967, 2925, 2875 (–CHaliphatic), 1661 (C
O). 1H NMR (500 MHz, CDCl3) δ (ppm): 7.99–7.98 dd, J: 5.37, 2.93 Hz, 2H (–CHarom); 7.62–7.61 dd, J: 5.86, 2.93 Hz, 2H (–CHarom); 4.04–4.00 q, J: 6.83 Hz, 2H (–CH–); 1.61–1.48 m, 4H (–CH2–); 1.24–1.22 dd, J: 6.84, 2.93 Hz, 6H (–CH3); 0.96–0.93 td, J: 7.32, 2.44 Hz, 6H, (–CH3). 13C NMR (125 MHz, CDCl3) δ (ppm): 178.3 (–C
O); 148.1 (Cq); 132.4, 125.9 (–CHarom); 132.1 (Cq); 44.5 (–CH–); 29.8 (–CH2–); 20.2, 20.1, 10.4, 10.3 (–CH3). MS (ESI+) m/z (%): 334 (100, [M]+), 335 (71, [M + H]+). Anal. calcd for C18H22O2S2 (334.50).
4.1.1.2. 2-(Sec-butylthio)-3-(3-(trifluoromethyl)phenylamino)naphthalene-1,4-dione (5b). This compound was synthesized from 2-chloro-3-((3-(trifluoromethyl)phenyl)amino)naphthalene-1,4-dione (3b) and butane-2-thiol (4a) as a red powder by using the general procedure. Yield: 0.045 g, mp: 109–110 °C, 39%. FTIR (ATR) ν (cm−1): 3275 (–NH), 3076 (–CHarom), 2967, 2929 (–CHaliphatic), 1662 (–C
O). 1H NMR (500 MHz, CDCl3) δ (ppm): 8.09–8.08 d, J: 7.81 Hz, 1H (–CHarom); 8.01–8.00 d, J: 7.81 Hz, 1H (–CHarom); 7.78 s, 1H (–NH); 7.69–7.65 td, J: 7.32, 0.97 Hz, 1H (–CHarom); 7.62–7.59 t, J: 7.32 Hz, 2H, (–CHarom); 7.38–7.35 t, J: 7.81 Hz, 1H (–CHarom); 7.32–7.30 d, J: 7.81 Hz, 1H (–CHarom); 7.09–7.08 d, J: 7.81 Hz, 1H, (–CHarom); 2.98–2.93 m, 1H (–CH–); 1.36–1.23 m, 2H (–CH2–); 0.97–0.95 d, J: 6.83 Hz, 3H (–CH3); 0.69–0.66 t, J: 7.32 Hz, 3H (–CH3). 13C NMR (125 MHz, CDCl3) δ (ppm): 180.2, 179.1 (–C
O); 143.7, 137.8, 132.3, 129.7, 127.8, 124.4, 119.9, 118.9, 118.1 (Cq); 133.6, 132.0, 126.0, 125.7 (–CHarom); 43.2 (–CH–); 28.7 (–CH2–); 19.3, 10.1 (–CH3). MS (ESI+) m/z (%): 406 (100, [M + H]+). Anal. calcd for C21H18F3NO2S (405.43).
4.1.1.3. 2-(Sec-butylthio)-3-((2-(trifluoromethyl)phenyl)amino)naphthalene-1,4-dione (5c). This compound was synthesized from 2-chloro-3-((2-(trifluoromethyl)phenyl)amino)naphthalene-1,4-dione (3c) and butane-2-thiol (4a) as a red oil by using the general procedure. Yield: 0.039 g, 70%. FTIR (ATR) ν (cm−1): 3328 (–NH), 3072 (–CHarom), 2963, 2922, 2872 (–CHaliphatic), 1664 (–C
O). 1H NMR (500 MHz, CDCl3) δ (ppm): 8.08–8.07 dd, J: 7.32, 1.46 Hz, 1H (–CHarom); 8.00–7.98 dd, J: 7.32, 1.46 Hz, 1H (–CHarom); 7.91 s, 1H (–NH); 7.68–7.65 td, J: 7.81, 0.98 Hz, 1H (–CHarom); 7.61–7.58 m, 2H, (–CHarom); 7.43–7.40 t, J: 7.81 Hz, 1H (–CHarom); 7.19–7.16 t, J: 7.81 Hz, 1H (–CHarom); 6.90–6.88 d, J: 8.30 Hz, 1H, (–CHarom); 3.07–3.02 m, 1H (–CH–); 1.42–1.26 m, 2H (–CH2–); 0.99–0.98 d, J: 6.34 Hz, 3H (–CH3); 0.73–0.70 t, J: 7.32 Hz, 3H (–CH3). 13C NMR (125 MHz, CDCl3) δ (ppm): 180.1, 178.8 (–C
O); 144.9, 132.2, 130.8, 129.8, 125.3, 118.9 (Cq); 133.5, 125.9, 125.7, 124.0, 123.4 (–CHarom); 43.3 (–CH–); 28.9 (–CH2–); 19.3, 10.0 (–CH3). MS (ESI+) m/z (%): 406 (100, [M + H]+). Anal. calcd for C21H18F3NO2S (405.43).
4.1.1.4. 2-(2-Hydroxypropylthio)-3-(4-(trifluoromethyl)phenylamino)naphthalene-1,4-dione (5d). This compound was synthesized from 2-chloro-3-((4-(trifluoromethyl)phenyl)amino)naphthalene-1,4-dione (3a) and 3-mercaptobutan-2-ol (4b) as an orange powder by using the general procedure. Yield: 0.085 g, mp: 139–140 °C, 73%. FTIR (ATR) ν (cm−1): 3419 (–OH), 3336 (–NH), 3030 (–CHarom), 2978, 2961, 2929 (–CHaliphatic), 1669 (–C
O). 1H NMR (500 MHz, CDCl3) δ (ppm): 8.10–8.08 dd, J: 7.81, 1.46 Hz, 1H (–CHarom); 8.03–8.01 dd, J: 7.32, 0.98 Hz, 1H (–CHarom); 7.86 s, 1H (–NH); 7.71–7.68 td, J: 7.32, 1.47 Hz, 1H (–CHarom); 7.64–7.61 td, J: 7.32, 1.46 Hz, 1H (–CHarom); 7.53–7.51 d, J: 8.78 Hz, 2H (–CHarom); 7.05–7.03 d, J: 8.79 Hz, 2H (–CHarom); 3.64–3.60 m, 1H (–CH–); 2.77–2.73 dd, J: 13.18, 2.93 Hz, 1H (–CH2–); 2.48–2.43 dd, J: 13.66, 8.78 Hz, 1H (–CH2–); 1.06–1.05 d, J: 6.34 Hz, 3H (–CH3). 13C NMR (125 MHz, CDCl3) δ (ppm): 181.4, 180.3 (–C
O); 135.1, 133.5, 127.4, 127.1 (–CHarom); 146.2, 142.1, 133.5, 130.8, 126.2, 122.5 (Cq); 66.5 (–CH–); 43.6 (–CH2–); 22.1 (–CH3). MS (ESI+) m/z (%): 429 (100, [M + Na]+), 407 (52, [M]+). Anal. calcd for C20H16F3NO3S (407.41).
4.1.1.5. 2-(2-Hydroxypropylthio)-3-(3-(trifluoromethyl)phenylamino)naphthalene-1,4-dione (5e). This compound was synthesized from 2-chloro-3-((3-(trifluoromethyl)phenyl)amino)naphthalene-1,4-dione (3b) and 3-mercaptobutan-2-ol (4b) as a red oil by using the general procedure. Yield: 0.076 g, 65%. FTIR (ATR) ν (cm−1): 3433 (–OH), 3346 (–NH), 3050 (–CHarom), 2980, 2930 (–CHaliphatic), 1664 (–C
O). 1H NMR (500 MHz, CDCl3) δ (ppm): 8.09–8.07 d, J: 7.81 Hz, 1H (–CHarom); 8.01–7.99 d, J: 7.32 Hz, 1H (–CHarom); 7.91 s, 1H (–NH); 7.70–7.66 td, J: 7.81, 1.46 Hz, 1H (–CHarom); 7.62–7.59 td, J: 7.32, 1.47 Hz, 1H (–CHarom); 7.41–7.35 t, J: 7.81 Hz, 1H (–CHarom); 7.37–7.35 t, J: 7.81 Hz, 1H (–CHarom); 7.25 s, 1H (–CHarom); 7.16–7.15 d, J: 7.32 Hz, 1H (–CHarom); 3.62–3.58 m, 1H (–CH–); 2.71–2.68 dd, J: 13.67, 3.42 Hz, 1H (–CH2–); 2.43–2.39 dd, J: 13.67, 8.79 Hz, 1H (–CH2–); 1.05–1.03 d, J: 6.35 Hz, 3H (–CH3). 13C NMR (125 MHz, CDCl3) δ (ppm): 181.5, 180.4 (–C
O); 146.7, 139.6, 133.5, 130.7, 129.4, 126.4, 122.0, 120.1, 117.4 (Cq); 135.1, 133.3, 127.4, 127.1 (–CHarom); 66.4 (–CH–); 43.8 (–CH2–); 22.1 (–CH3). MS (ESI−) m/z (%): 407 (16, [M]+), 406 (35, [M − H]+), 405 (100, [M − 2H]+). Anal. calcd for C20H16F3NO3S (407.41).
4.1.1.6. 2-((2-Hydroxypropyl)thio)-3-((2-(trifluoromethyl)phenyl)amino)naphthalene-1,4-dione (5f). This compound was synthesized from 2-chloro-3-((2-(trifluoromethyl)phenyl)amino)naphthalene-1,4-dione (3c) and 3-mercaptobutan-2-ol (4b) as a red powder by using the general procedure. Yield: 0.059 g, mp: 85–87 °C, 66%. FTIR (ATR) ν (cm−1): 3423 (–OH), 3328 (–NH), 3075 (–CHarom), 2966, 2919 (–CHaliphatic), 1667 (–C
O). 1H NMR (500 MHz, CDCl3) δ (ppm): 8.09–8.07 dd, J: 7.32, 0.98 Hz, 1H (–CHarom); 8.01–7.99 dd, J: 7.81, 0.97 Hz, 1H (–CHarom); 7.93 s, 1H (–NH); 7.70–7.66 td, J: 7.81, 1.46 Hz, 1H (–CHarom); 7.62–7.60 m, 2H (–CHarom); 7.47–7.44 t, J: 7.80 Hz, 1H (–CHarom); 7.26–7.23 t, J: 7.80 Hz, 1H (–CHarom); 7.00–6.99 d, J: 7.80 Hz, 1H (–CHarom); 3.62–3.54 m, 1H (–CH–); 2.96 s, 1H (–OH); 2.78–2.75 dd, J: 13.67, 2.96 Hz, 1H (–CH2–); 2.42–2.38 dd, J: 13.67, 8.79 Hz, 1H (–CH2–); 1.04–1.03 d, J: 6.35 Hz, 3H (–CH3). 13C NMR (125 MHz, CDCl3) δ (ppm): 180.3, 178.8 (–C
O); 146.2, 132.2, 131.1, 129.5, 125.6 (Cq); 133.8, 132.1, 126.2, 125.9, 124.9, 124.4 (–CHarom); 65.0 (–CH–); 42.7 (–CH2–); 20.7 (–CH3). MS (ESI+) m/z (%): 408 (100, [M + H]+), 430 (54, [M + Na]+). Anal. calcd for C20H16F3NO3S (407.41).
4.1.1.7. 2-(Furan-2-ylmethylthio)-3-(4-(trifluoromethyl)phenylamino)naphthalene-1,4-dione (5g). This compound was synthesized from 2-chloro-3-((4-(trifluoromethyl)phenyl)amino)naphthalene-1,4-dione (3a) and furan-2-ylmethanethiol (4c) as a red powder by using the general procedure. Yield: 0.037 g, mp: 91–92 °C, 30%. FTIR (ATR) ν (cm−1): 3334 (–NH), 3104 (–C–O–ether), 2964, 2918, 2851 (–CHaliphatic), 1659 (–C
O). 1H NMR (500 MHz, CDCl3) δ (ppm): 8.10–8.08 dd, J: 7.81, 1.46 Hz, 1H (–CHarom); 7.99–7.97 dd, J: 7.81, 1.46 Hz, 1H (–CHarom); 7.70–7.67 td, J: 7.32, 1.46 Hz, 1H (–CHarom); 7.66 bs, 1H (–NH); 7.62–7.59 td, J: 7.81, 1.47 Hz, 1H (–CHarom); 7.48–7.47 d, J: 8.30 Hz, 2H (–CHarom); 7.12–7.11 t, J: 0.98 Hz, 1H, (–CHarom); 6.93–6.91 d, J: 8.29 Hz, 2H (–CHarom); 6.09–6.08 dd, J: 2.93, 1.95 Hz, 1H (–CHarom); 5.88–5.87 d, J: 3.42 Hz, 1H (–CHarom); 3.91 s, 2H (–CH2–). 13C NMR (125 MHz, CDCl3) δ (ppm): 179.7, 178.9 (–C
O); 149.7, 144.8, 141.2, 140.9 (Cq); 133.7, 132.3, 132.0, 129.6, 125.9, 125.8, 121.0 (–CHarom); 124.8, 117.4, 109.5, 107.2 (Cq); 29.4 (–CH2–). MS (ESI+) m/z (%): 452 (27, [M + Na]+), 451 (100, [M + Na − 1]+). Anal. calcd for C22H14F3NO3S (429.41).
4.1.1.8. 2-(Furan-2-ylmethylthio)-3-(3-(trifluoromethyl)phenylamino)naphthalene-1,4-dione (5h). This compound was synthesized from 2-chloro-3-((3-(trifluoromethyl)phenyl)amino)naphthalene-1,4-dione (3b) and furan-2-ylmethanethiol (4c) as a red oil by using the general procedure. Yield: 0.08 g, 66%. FTIR (ATR) ν (cm−1): 3308 (–NH), 3072 (–C–Oether), 2928 (–CHaliphatic), 1668 (–C
O). 1H NMR (500 MHz, CDCl3) δ (ppm): 8.11–8.10 d, J: 7.81 Hz, 1H (–CHarom); 7.99–7.97 d, J: 7.80 Hz, 1H (–CHarom); 7.71–7.67 td, J: 7.81, 1.46 Hz, 1H (–CHarom); 7.66 s, 1H (–NH); 7.62–7.59 td, J: 7.81, 1.46 Hz, 1H (–CHarom); 7.35–7.34 m, 2H (–CHarom); 7.13–7.12 m, 2H (–CHarom); 7.03–7.01 m, 1H (–CHarom); 6.10–6.09 dd, J: 3.41, 1.47 Hz, 1H (–CHarom); 5.87–5.86 d, J: 3.41 Hz, 1H (–CHarom); 3.88 s, 2H (–CH2–). 13C NMR (125 MHz, CDCl3) δ (ppm): 179.6, 179.0 (–C
O); 149.8, 145.7, 141.2, 138.5, 132.4, 129.6, 128.1, 125.0, 120.5, 118.7, 115.9 (Cq); 133.7, 131.9, 126.0, 125.8, 109.5, 107.2 (–CHarom); 29.6 (–CH2–). MS (ESI−) m/z (%): 428 (100, [M − H]+). Anal. calcd for C22H14F3NO3S (429.41).
4.1.1.9. 2-((Furan-2-ylmethyl)thio)-3-((2-(trifluoromethyl)phenyl)amino)naphthalene-1,4-dione (5i). This compound was synthesized from 2-chloro-3-((2-(trifluoromethyl)phenyl)amino)naphthalene-1,4-dione (3c) and furan-2-ylmethanethiol (4c) as a red oil by using the general procedure. Yield: 0.07 g, 85%. FTIR (ATR) ν (cm−1): 3294 (–NH), 2922 (–CHaliphatic), 1676 (–C
O). 1H NMR (500 MHz, CDCl3) δ (ppm): 8.09–8.07 dd, J: 7.81, 0.98 Hz, 1H (–CHarom); 7.97–7.95 dd, J: 7.81, 0.98 Hz, 1H (–CHarom); 7.75 s, 1H (–NH); 7.68–7.65 td, J: 7.32, 1.46 Hz, 1H (–CHarom); 7.60–7.56 m, 2H (–CHarom); 7.39–7.36 t, J: 7.80 Hz, 1H (–CHarom); 7.21–7.17 q, J: 7.32 Hz, 1H (–CHarom); 7.12–7.10 d, J: 2.44 Hz, 1H (–CHarom); 6.82–6.80 d, J: 7.81 Hz, 1H (–CHarom); 6.07–6.06 dd, J: 3.44, 1.96 Hz, 1H (–CHarom); 5.86–5.85 d, J: 3.90 Hz, 1H (–CHarom); 3.91 s, 2H (–CH2–). 13C NMR (125 MHz, CDCl3) δ (ppm): 179.6, 178.7 (–C
O); 149.7, 145.9, 141.2, 132.4, 130.9, 129.5, 125.5, 116.6, 116.1 (Cq); 133.7, 131.9, 131.8, 125.9, 125.7, 124.5, 124.0 (–CHarom); 29.4 (–CH2–). MS (ESI+) m/z (%): 452 (100, [M + Na]+), 430 (22, [M + H]+). Anal. calcd for C22H14F3NO3S (429.41).
4.1.1.10. 2-(Phenethylthio)-3-(4-(trifluoromethyl)phenylamino)naphthalene-1,4-dione (5j). This compound was synthesized from 2-chloro-3-((4-(trifluoromethyl)phenyl)amino)naphthalene-1,4-dione (3a) and 2-phenylethanethiol (4d) as a red powder by using the general procedure. Yield: 0.074 g, mp: 85–87 °C, 57%. FTIR (ATR) ν (cm−1): 3321 (–NH), 3272, 3060, 3025 (–CHarom); 2930 (–CHaliphatic), 1662 (–C
O). 1H NMR (500 MHz, CDCl3) δ (ppm): 8.07–8.05 dd, J: 7.33, 1.46 Hz, 1H (–CHarom); 8.00–7.98 dd, J: 7.32, 1.47 Hz, 1H (–CHarom); 7.69–7.66 td, J: 7.32, 1.47 Hz, 1H (–CHarom); 7.64 bs, 1H (–NH); 7.63–7.60 td, J: 7.33, 1.46 Hz, 1H (–CHarom); 7.50–7.48 d, J: 8.78 Hz, 2H (–CHarom); 7.06–7.03 t, J: 7.33 Hz, 2H (–CHarom); 6.98–6.96 d, J: 7.32 Hz, 1H (–CHarom); 6.94–6.92 d, J: 8.30 Hz, 4H (–CHarom); 2.88–2.85 t, J: 7.32 Hz, 2H (–CH2–); 2.66–2.63 t, J: 7.81 Hz, 2H (–CH2–). 13C NMR (125 MHz, CDCl3) δ (ppm): 180.0, 178.9 (–C
O); 142.5, 140.5, 138.5, 134.2 (Cq); 133.5, 132.3, 132.0, 127.5, 127.3, 125.9, 125.7 (–CHarom); 129.6, 125.4, 124.7, 120.2 (Cq); 35.3, 33.5 (–CH2–). MS (ESI−) m/z (%): 453 (20, [M]+), 452 (48, [M − H]+), 451 (100, [M − 2H]+). Anal. calcd for C25H18F3NO2S (453.48).
4.1.1.11. 2-(Phenethylthio)-3-(3-(trifluoromethyl)phenylamino)naphthalene-1,4-dione (5k). This compound was synthesized from 2-chloro-3-((3-(trifluoromethyl)phenyl)amino)naphthalene-1,4-dione (3b) and 2-phenylethanethiol (4d) as a red powder by using the general procedure. Yield: 0.05 g, mp: 93–95 °C, 39%. FTIR (ATR) ν (cm−1): 3308 (–NH), 3063, 3025 (–CHarom), 2931 (–CHaliphatic), 1657 (–C
O). 1H NMR (500 MHz, CDCl3) δ (ppm): 8.06–8.05 dd, J: 7.32, 1.46 Hz, 1H (–CHarom); 7.99–7.97 dd, J: 7.32, 0.97 Hz, 1H (–CHarom); 7.68–7.65 td, J: 7.32, 1.47 Hz, 1H (–CHarom); 7.66 s, 1H (–NH); 7.61–7.58 td, J: 7.80, 1.46 Hz, 1H (–CHarom); 7.36–7.33 t, J: 7.81 Hz, 1H (–CHarom); 7.31–7.29 d, J: 7.81 Hz, 1H (–CHarom); 7.15 s, 1H, (–CHarom); 7.06–7.03 m, 3H (–CHarom); 6.98–6.93 m, 3H (–CHarom); 2.86–2.83 t, J: 7.33 Hz, 2H (–CH2–); 2.63–2.60 t, J: 7.32 Hz, 2H (–CH2–). 13C NMR (125 MHz, CDCl3) δ (ppm): 179.9, 179.0 (–C
O); 143.1, 138.6, 138.0, 132.4, 129.6, 127.9, 125.4, 124.0, 119.9, 118.6, 117.8 (Cq); 133.6, 132.0, 127.5, 127.3, 125.9, 125.7 (–CHarom); 35.2, 33.6 (–CH2–). MS (ESI−) m/z (%): 453 (25, [M]+), 452 (100, [M − H]+). Anal. calcd for C25H18F3NO2S (453.48).
4.2. In vitro antimicrobial activity
4.2.1. Determination of minimum inhibitory concentrations (MIC). Antibacterial activities against the three Gram-positive bacteria (Staphylococcus aureus ATCC 29213, Staphylococcus epidermidis ATCC 12228, and Enterococcus faecalis ATCC 29212) and four Gram-negative bacteria (Pseudomonas aeruginosa ATCC 27853, Escherichia coli ATCC 25922, Klebsiella pneumoniae ATCC 4352, and Proteus mirabilis ATCC 14153) and the antifungal activity against a yeast Candida albicans ATCC 10231 were determined by the microbroth dilutions technique using the Clinical Laboratory Standards Institute (CLSI) recommendations.28,29 Mueller–Hinton broth for bacteria and RPMI-1640 medium for the yeast strain were used as the test media. Serial two-fold dilutions ranging from 2500 μg mL−1 to 0.61 μg mL−1 were prepared in the medium. Inoculum was prepared using a 4–6 h broth culture for each bacteria type and 24 hours of culture of yeast strains were adjusted to a turbidity equivalent to the 0.5 McFarland standard, diluted in broth media to give a final concentration of 5 × 105 cfu mL−1 for the bacteria and 5 × 103 cfu mL−1 for the yeast in the test tray. The trays were covered and placed into plastic bags to prevent evaporation. The trays containing Mueller–Hinton broth were incubated at 35 °C for 18–20 h, while the trays containing RPMI-1640 medium were incubated at 35 °C for 46–50 h. The MIC was defined as the lowest concentration of compound, where the complete inhibition of visible growth can be seen. As the control, the antimicrobial effects of the solvents were investigated against the test microorganisms. According to these values of the control groups, the results were evaluated. The MIC values of the compounds are given in Table 2.
4.2.2. Determination of antibiofilm activity. Measurements of the antimicrobial susceptibilities of the bacterial and C. albicans biofilms were assessed using a minimum biofilm eradication concentration (MBEC) assay, which was performed with the stated mentioned techniques as in the following modifications.30 The 24 h biofilms were grown in a 96 well tissue culture, the microtiter plates were washed three times with 250 μL PBS solutions and air-dried. Serial two-fold dilutions ranging from 10
000 to 625 μg mL−1 for the molecules were prepared in cation adjusted Mueller–Hinton broth (CAMHB). Following that, 200 μL of each sample concentration were added to each corresponding well and the plates were incubated for 24 h at 37 °C. After the incubation, the antibiotics were gently aspirated, the plates were washed, thoroughly scraped, and the contents of each well were incubated in a sonicating water bath for 5 minutes to disrupt the biofilms. 100 μL samples were plated on TSA and the colonies were counted after 24 h of incubation at 37 °C. MBEC was defined as the lowest concentration of molecules where the microorganism failed to regrow after the exposure.Biofilm attachment and inhibition of the biofilm formation assays were performed as in the previously described method with some further modifications.30 1/10× MIC of molecules were added to the 24 h biofilm and the plates were incubated for 1, 2 and 4 h at 37 °C; molecules at 1×, 1/10× and 1/100× MIC concentrations were added to the 24 h biofilm, and plates were incubated for 24 h at 37 °C, respectively. Six wells were used for each molecule. The positive controls were microorganisms in TSB-glucose without the molecules of interest. After the incubation, wells were washed with PBS solutions and measured at OD595 nm.
4.2.3. Statistical analysis. All of the experiments were performed in two independent assays. In the determination of MIC and MBEC values, when the results were different in the both experiments, we made another test for the final result. In the assays of biofilm attachment and the inhibition of biofilm formation, results were presented as mean ± standard deviation of two independent experiments. One way ANOVA-Bonferroni's multiple comparison test was used to compare the differences between the control and the antimicrobial treated biofilms. P value < 0.001 was considered as a statistically significant parameter.
4.3. Cell culture and cytotoxicity assays
Compounds 5b and 5e were assayed for in vitro cytotoxicity against the hepatocellular carcinoma (HepG2) cell line, which was kindly provided by the Department of Pharmaceutical Toxicology in the Faculty of Pharmacy at Istanbul University. HepG2 cells were grown in Eagle's Minimum Essential Medium (EMEM) (Gibco), and were supplemented with 10% FBS (Gibco), and 100 units per mL penicillin G and 100 units per mL streptomycin under the humidified, 5% CO2 atmosphere at 37 °C. For the assays, the cells were seeded as 1 × 104 cells per well in 96 well plates and were allowed 24 h to ensure the attachment. Following the 24 h, the cells were incubated either in the absence or presence of serial dilutions of test compounds for a further 24 h. After the 24 h, cell viability was determined by XTT (Xenometrix) (2,3-bis-(2-methoxy-4-nitro-5-sulfophenyl)-2H-tetrazolium-5-carboxanilide) assay in accordance with the manufacturer's protocol.31 In each assay, three replicates for each concentration were used and this process, which used three replicates was repeated four times. The cytotoxic effects of each compound were obtained as IC50 values, which represent the molar drug concentrations required to cause 50% inhibition of cell viability.
4.4. Docking studies
The molecules were built using ChemSketch 12.0.1 and converted to 3D structure from the 2D structures, and further 3D structures were energetically minimized and saved as MDL Mol File (*.mol2). The docking was performed by Molegro Virtual Docker 2010.4.1.0 program.23 Staphylococcus epidermidis mevalonate diphosphate decarboxylase [PDB ID: 4DPT] was taken as the target enzyme for the rationalization of the antibacterial activity.24 The polar hydrogen atoms, partial atomic charges and solvation parameters were added. The MolDock optimization search algorithm with a maximum of 100 runs was used for the calculations, while all the other parameters were kept as default. All the spatial structures were examined manually, and the best one was attained for further use.
Acknowledgements
This work was financially supported by the Scientific Research Projects Coordination Unit of Istanbul University (Project number: 53374) in the supply of the equipment and materials. We also gratefully thank to Prof. Dr Buket Alpertunga and Res. Assist. Ayşe Tarbın Jannuzzi for providing us the hepatocellular carcinoma (HepG2) cell lines and valuable suggestions from the Department of Pharmaceutical Toxicology in the Faculty of Pharmacy at Istanbul University.
References
-
(a) C. Dye, Philos. Trans. R. Soc., B, 2014, 369 Search PubMed
;
(b) M. L. Cohen, Nature, 2000, 406, 762–767 CrossRef CAS PubMed
;
(c) A. S. Fauci, Clin. Infect. Dis., 2001, 32, 675–685 CrossRef CAS PubMed
. - World Health Organization, Mortality and Global Health Estimates, Geneva, Switzerland, 2013 Search PubMed
. - K. F. Smith, M. Goldberg, S. Rosenthal, L. Carlson, J. Chen, C. Chen and S. Ramachandran, J. R. Soc., Interface, 2014, 11, 20140950 CrossRef
. - World Health Organization, Global Tuberculosis Control, Geneva, Switzerland, 2010 Search PubMed
. -
(a) M. N. Gwynn, A. Portnoy, S. F. Rittenhouse and D. J. Payne, Ann. N. Y. Acad. Sci., 2010, 1213, 5–19 CrossRef PubMed
;
(b) D. Sanglard, Curr. Opin. Microbiol., 2002, 5, 379–385 CrossRef CAS PubMed
;
(c) M. L. Cohen, Science, 1992, 257, 1050–1055 CAS
;
(d) H. S. Gold and R. C. Moellering Jr, N. Engl. J. Med., 1996, 335, 1445–1453 CrossRef CAS PubMed
. -
(a) A. R. M. Coates and Y. Hu, Br. J. Pharmacol., 2007, 152, 1147–1154 CrossRef CAS PubMed
;
(b) K. Lewis, Nature, 2012, 485, 439–440 CrossRef CAS PubMed
. -
(a) A. Riffel, L. F. Medina, V. Stefani, R. C. Santos, D. Bizani and A. Brandelli, Braz. J. Med. Biol. Res., 2002, 35, 811–818 CrossRef CAS PubMed
;
(b) C. K. Ryu, R. E. Park, M. Y. Ma and J. H. Nho, Bioorg. Med. Chem. Lett., 2007, 17, 2577–2580 CrossRef CAS PubMed
;
(c) V. K. Tandon, H. K. Maurya, D. B. Yadav, A. Tripathi, M. Kumar and P. K. Shukla, Bioorg. Med. Chem. Lett., 2006, 16, 5883–5887 CrossRef CAS PubMed
;
(d) V. K. Tandon, H. K. Maurya, M. K. Verma, R. Kumar and P. K. Shukla, Eur. J. Med. Chem., 2010, 45, 2418–2426 CrossRef CAS PubMed
. -
(a) C. K. Ryu, J. A. Choi and S. H. Kim, Arch. Pharmacal Res., 1998, 21, 440–444 CrossRef CAS
;
(b) V. K. Tandon, H. K. Maurya, N. N. Mishra and P. K. Shukla, Eur. J. Med. Chem., 2009, 44, 3130–3137 CrossRef CAS PubMed
;
(c) V. K. Tandon, D. B. Yadav, H. K. Maurya, A. K. Chaturvedi and P. K. Shukla, Bioorg. Med. Chem., 2006, 14, 6120–6126 CrossRef CAS PubMed
. - V. K. Tandon, H. K. Maurya, A. Tripathi, G. B. ShivaKeshava, P. K. Shukla, P. Srivastava and D. Panda, Eur. J. Med. Chem., 2009, 44, 1086–1092 CrossRef CAS PubMed
. - R. F. Silver and H. L. Holmes, Can. J. Chem., 1968, 46, 1859–1864 CrossRef CAS
. - I. Oeriu, Biokhimiia, 1963, 28, 380–383 CAS
. - B. Prescott, J. Med. Chem., 1969, 12, 181–182 CrossRef CAS PubMed
. - E. M. Hodnett, C. Wongwiechintana, W. J. Dunn 3rd and P. Marrs, J. Med. Chem., 1983, 26, 570–574 CrossRef CAS PubMed
. - N. G. Clark, Pestic. Sci., 1984, 15, 235–240 CrossRef CAS
. - J. Benites, J. A. Valderrama, K. Bettega, R. C. Pedrosa, P. B. Calderon and J. Verrax, Eur. J. Med. Chem., 2010, 45, 6052–6057 CrossRef CAS PubMed
. - S. V. Druzhinin, E. S. Balenkova and V. G. Nenajdenko, Tetrahedron, 2007, 63, 7753–7808 CrossRef CAS
. - B. S. Samant and C. Chakaingesu, Bioorg. Med. Chem. Lett., 2013, 23, 1420–1423 CrossRef CAS PubMed
. - V. K. Tandon, H. K. Maurya, D. B. Yadav, A. Tripathi, M. Kumar and P. K. Shukla, Bioorg. Med. Chem. Lett., 2006, 16, 5883–5887 CrossRef CAS PubMed
. - A. F. Tuyun, N. Bayrak, H. Yildirim, N. Onul, E. M. Kara and B. O. Celik, J. Chem, 2015, 645902 Search PubMed
. - Website: http://www.ibmc.msk.ru/PASS.
-
(a) V. V. Poroikov, D. A. Filimonov, Y. V. Borodina, A. A. Lagunin and A. Kos, J. Chem. Inf. Comput. Sci., 2000, 40, 1349–1355 CrossRef CAS PubMed
;
(b) V. V. Poroikov and D. A. Filimonov, J. Comput.-Aided Mol. Des., 2002, 16, 819–824 CrossRef CAS PubMed
;
(c) A. Geronikaki, E. Babaev, J. Dearden, W. Dehaen, D. Filimonov, I. Galaeva, V. Krajneva, A. Lagunin, F. Macaev, G. Molodavkin, V. Poroikov, S. Pogrebnoi, V. Saloutin, A. Stepanchikova, E. Stingaci, N. Tkach, L. Vlad and T. Voronina, Bioorg. Med. Chem., 2004, 12, 6559–6568 CrossRef CAS PubMed
;
(d) A. Geronikaki, A. Lagunin, V. Poroikov, D. Filimonov, D. Hadjipavlou-Litina and P. Vicini, SAR QSAR Environ. Res., 2002, 13, 457–471 CrossRef CAS PubMed
;
(e) R. K. Goel, V. Kumar and M. P. Mahajan, Bioorg. Med. Chem. Lett., 2005, 15, 2145–2148 CrossRef CAS PubMed
;
(f) G. K. Gupta, V. Saini, R. Khare and V. Kumar, Med. Chem. Res., 2014, 23, 4209–4220 CrossRef CAS
;
(g) A. Kumar, P. Lohan, D. K. Aneja, G. K. Gupta, D. Kaushik and O. Prakash, Eur. J. Med. Chem., 2012, 50, 81–89 CrossRef CAS PubMed
. -
(a) R. M. Donlan and J. W. Costerton, Clin. Microbiol. Rev., 2002, 15, 167 CrossRef CAS PubMed
;
(b) P. Jorge, A. Lourenco and M. O. Pereira, Biofouling, 2012, 28, 1033–1061 CrossRef CAS PubMed
. - R. Thomsen and M. H. Christensen, J. Med. Chem., 2006, 49, 3315–3321 CrossRef CAS PubMed
. -
(a) M. L. Barta, D. A. Skaff, W. J. McWhorter, T. J. Herdendorf, H. M. Miziorko and B. V. Geisbrecht, J. Biol. Chem., 2011, 286, 23900–23910 CrossRef CAS PubMed
;
(b) D. A. Skaff, W. J. McWhorter, B. V. Geisbrecht, G. J. Wyckoff and H. M. Miziorko, Arch. Biochem. Biophys., 2015, 566, 1–6 CrossRef CAS PubMed
. -
(a) D. V. Krepkiy and H. M. Miziorko, Biochemistry, 2005, 44, 2671–2677 CrossRef CAS PubMed
;
(b) D. Krepkiy and H. M. Miziorko, Protein Sci., 2004, 13, 1875–1881 CrossRef CAS PubMed
;
(c) N. E. Voynova, Z. J. Fu, K. P. Battaile, T. J. Herdendorf, J. J. P. Kim and H. M. Miziorko, Arch. Biochem. Biophys., 2008, 480, 58–67 CrossRef CAS PubMed
. - N. P. Buu-Hoi, R. Royer and M. Hubert-Habart, Recl. Trav. Chim. Pays-Bas, 1954, 73, 188–192 CrossRef CAS
. - A. Mital, M. Sonawane, S. Bindal, S. Mahlavat and V. Negi, Der Pharma Chem., 2010, 2(3), 63–73 CAS
. - Clinical and Laboratory Standards Institute, Reference Method for Broth Dilution Antifungal Susceptibility Testing of Yeasts, CLSI, Wayne, PA, 2th edn, 1997, Approved Standard M27-A2 Search PubMed
. - Clinical and Laboratory Standards Institute, Methods for dilution antimicrobial susceptibility tests for bacteria that grow aerobically, CLSI, Wayne, PA, 7th edn, 2006, Approved standard M7-A7 Search PubMed
. - E. Mataraci and S. Dosler, Antimicrob. Agents Chemother., 2012, 56, 6366–6371 CrossRef CAS PubMed
. - http://www.aniara.com/Resources/Xenometrix-Literature.aspx.
.
Footnote |
† Electronic supplementary information (ESI) available. See DOI: 10.1039/c7ra00868f |
|
This journal is © The Royal Society of Chemistry 2017 |
Click here to see how this site uses Cookies. View our privacy policy here.