DOI:
10.1039/D0RA08228G
(Review Article)
RSC Adv., 2020,
10, 39808-39813
ACE2 as a potential therapeutic target for pandemic COVID-19
Received
25th September 2020
, Accepted 26th October 2020
First published on 1st November 2020
Abstract
SARS-CoV-2 virus invades the host through angiotensin-converting enzyme 2 (ACE2) receptors by decreasing the ACE2 expression of the host. This disturbs the dynamic equilibrium between the ACE/Ang II/AT1R axis and ACE2/Ang (1–7)/Mas receptor axis. Therefore, the clinically approved drugs belonging to (i) angiotensin converting enzyme (ACE) inhibitors such as captopril, and enalaprilat, (ii) angiotensin-receptor blockers (ARBs) such as losartan, candesartan, olmesartan, azilsartan, irbesartan, and telmisartan and (iii) the combination of ACE inhibitors and ARBs such as losartan with lisinopril and captopril with losartan, and (iv) recombinant ACE2, were studied for their ability to activate ACE2 in different medical conditions including hypertension, inflammation, cardiovascular, renal and lung diseases. These clinically approved drugs were found to activate ACE2 that had been downregulated in different medical conditions including hypertension, inflammation, cardiovascular, renal and lung diseases. Therefore, these drugs may be repurposed to re-activate the downregulated ACE2 of COVID-19 patients. These drugs either alone or in combination may be repurposed as prophylactics and therapeutics against SARS-CoV-2 virus.
Introduction
Coronavirus disease (COVID-19) is a life-threatening infectious viral disease that emerged in Wuhan, China. It is caused by SARS-CoV-2 infection1 which has infected more than 41
104
946 humans worldwide and has caused more than 1
128
325 deaths as reported by the World Health Organization (WHO). SARS-CoV-2 infects the respiratory tract and causes symptoms associated with cardiovascular, digestive and neurological diseases.2,3 Elderly people with cardiovascular conditions including hypertension are more prone to develop COVID-19.4 COVID-19 patients have higher levels of angiotensin II as compared to healthy individuals.5 It has been found that this disease infects humans and is transmitted from human to human and also from humans to animals.6,7 This disease is pandemic causing a more serious global health threat than severe acute respiratory syndrome (SARS) and the Middle East Respiratory Syndrome (MERS) epidemics, therefore there is an urgent requirement for therapeutic and prophylactic interventions for COVID-19.
The pathogen, SARS-CoV-2 is a single stranded RNA virus belonging to β-coronavirus genus with lineage B.8 Further, S1 subunits of spike (S) proteins of SARS-CoV-2 and SARS-CoV have affinity towards human angiotensin-converting enzyme 2 (hACE2) receptors.8 ACE2 is involved in conversion of angiotensin II (Ang II) to angiotensin-(1–7) [Ang (1–7)] and angiotensin I (Ang I) to angiotensin-(1–9) [Ang (1–9)].9 Angiotensin-converting enzyme 2 (ACE2) is homolog of the angiotensin converting enzyme (ACE) that is expressed in cell membranes of heart, kidney, lungs, arteries and intestines.10 Interestingly, SARS-CoV-2 binds to ACE2 receptor, 10–20 folds higher compared to SARS-CoV resulting in higher infection and transmissibility.11
Interestingly, ACE2 protects lungs from severe acute lung injury (Imai et al., 2005)17 and kidneys from damage.12,13 The deficiency of ACE2 is related with advanced glomerulosclerosis, oxidative stress in kidneys and diabetic nephropathy.14–16 ACE2 is a member of renin–angiotensin–aldosterone system (RAAS) that help in regulating hypertension, electrolyte balance and contributes to pathophysiology of cardiovascular/renal/lung diseases. ACE2 expression is decreased during SARS-CoV infections resulting in disturbance of dynamic equilibrium state between ACE/Ang II/AT1R axis and ACE2/Ang (1–7)/Mas receptor axis17 and similarly this may happen during SARS-CoV-2 infection.
Interestingly, upregulation of ACE2 has also been found to be species specific upon interferon stimulation. Notably, interferon upregulate the human ACE2 in nasal epithelia and lung tissue while it is not able to upregulate the mouse ACE2 thereby helping in generating more targets for SARS-COV-2 and exploring more areas for invasion of virus.18
There is mixed findings on ACE2 levels by ACE inhibitors and angiotensin-receptor blockers (ARBs)19–34 Notably, the effect of angiotensin converting enzyme (ACE) inhibitors and angiotensin-receptor blockers (ARBs) on ACE2 is expected to be different as they have dissimilar effects on angiotensin II.35
As SARS-CoV-2 sneaks into humans through hACE2 receptors and then downregulates its expression such that ACE2 forgoes its protective effect in different organs, therefore activating ACE2 may lead to the treatment for COVID-19 (Fig. 1). Till date, there is no effective therapeutic drug to cure COVID-19. Considering the complexity of the pathogenesis of COVID-19, we are highlighting the studies on clinically approved drugs which are activating ACE2 and may act as potential COVID-19 therapeutics (Fig. 1). The drugs activating ACE2 belongs to (i) angiotensin converting enzyme (ACE) inhibitors (Fig. 2), (ii) angiotensin-receptor blockers (ARBs) (Fig. 2), (iii) the combinations of ACE inhibitor and ARB, (iv) recombinant ACE2.
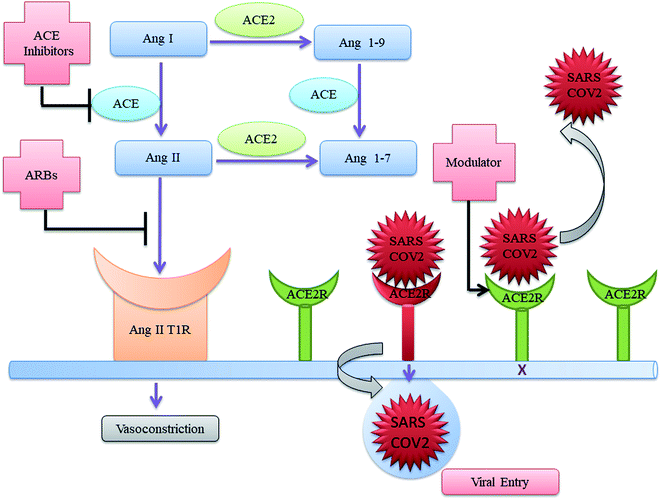 |
| Fig. 1 Schematic mechanism for ACE inhibitor, ARBs and recombinant ACE2 as repurposed drugs for COVID-19. | |
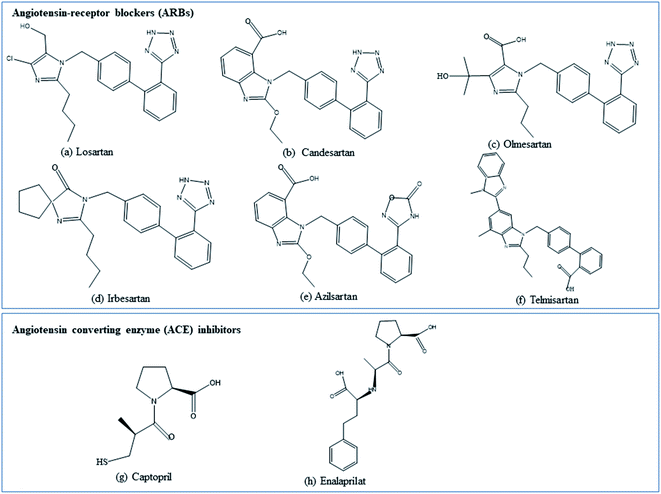 |
| Fig. 2 Chemical structures of the angiotensin converting enzyme (ACE) inhibitors and angiotensin-receptor blockers (ARBs) as repurposed drugs for COVID-19. | |
(i) Angiotensin converting enzyme (ACE) inhibitors
Since the active sites of ACE and ACE2 are different, therefore ACE inhibitors does not directly affect ACE2 activity36 but can cause activation of ACE2. Captopril, an ACE inhibitor activates ACE2/angiotensin-(1–7) level/Mas receptor axis,37 lowering mean pulmonary arterial pressure (PAP) and right ventricular index.38 Furthermore, pre-treatment with captopril prevents lipopolysaccharide-induced injury in pulmonary microvascular endothelial cells of rat by bringing back the ACE/ACE2 ratio to the normal levels and lowers the ratio of angiotensin II to angiotensin-(1–7).37 Captopril prevents lung apoptosis by maintaining the normal level of ACE2/ACE axis during acute pulmonary embolism demonstrated in porcine model.39
Treatment with enalapril at in neurogenic pulmonary edema expressed in rabbit models reduces the injury of lungs by reducing angiotensin II concentration and ratio of ACE mRNA to ACE2 mRNA in lungs.40 Further, enalapril has the ability to inhibit the inflammation in airway caused by acrolein.41
As there is a well-established association between obesity, hypertension and diabetes, enalapril treatment gives protection to pancreatic islets and controls body mass, glucose intolerance and insulin resistance with the increase of ACE2/Ang (1–7)/Mas receptor axis and level of adiponectin.42
(ii) Angiotensin-receptor blockers (ARBs)
The treatment with ARBs causes an upregulation of ACE2 as seen from animal studies20,25,43 The angiotensin II type 1 receptor (AT1R) is the main receptor mediating angiotensin II effects. Losartan is angiotensin II type 1 receptor antagonists that demonstrate lung protectiveness. Its treatment in pulmonary arterial hypertension decreases the level of angiotensin II, ACE expression and increases ACE2 expression in lung tissue.44 Interestingly, losartan prevents lipopolysaccharide induced lung injury by lowering cytokines and high mobility group box 1 (HMGB1) protein levels and controls ACE2 activity in rat model system.45 In smoke-induced pulmonary arterial remodeling and pulmonary arterial hypertension model system, losartan plays by lowering the angiotensin II level in lungs and increasing ACE2 levels.46
In spontaneously hypertensive rats, losartan administration resulted in high level of renal ACE2 and angiotensin-(1–7) generation suggesting the renal protection.47 Candesartan is renoprotective in intermediate doses in diabetic mice by upregulating ACE2/AT2R/Mas axis protective components.48 In addition, olmesartan treatment in hypertensive patients resulted in increase of ACE2 expression in urine thus providing renoprotective effects.34 Iwanami49 et al. studied the impact of treatment with azilsartan and olmesartan which are angiotensin II type 1 (AT1) receptor blockers in transgenic mice with the human renin and angiotensinogen genes (hRN/hANG-Tg) and found that azilsartan decreases blood pressure and increases sodium concentration in urine more than that of olmesartan with activation of ACE2/Ang-(1–7)/Mas axis.
Losartan or telmisartan is also used for treatment of non-alcoholic fatty liver disease (NAFLD) in obese and/or hypertensive model system by lowering hepatic triacylglycerol levels, PLIN 2 and improve glycemic control by using ACE2/rMAS axis.50 Additionally, ARBs have anti-inflammatory properties as seen from irbesartan which decreases the intestinal inflammation induced by stress via activation of ACE2 pathway.51
Increase in ACE2 expression in heart is observed in rat after administration of losartan and olmesartan.29 In the patients having essential hypertension, olmesartan usage for long period decreases left ventricular mass index.52 Interestingly, cardiac hypertrophy not dependent on blood pressure is inhibited by olmesartan through the original AT1R blockade and also with the increase of ACE2/Ang (1–7)/Mas axis with decrease of Nox4 expression.53 Losartan or captopril combined with angiotensin-(1–7) resulted in cardioprotection for diabetes-induced cardiac dysfunction by ACE2/Ang-(1–7)/Mas receptor axis.54 The treatment with candesartan controlled the progression of dilated cardiomyopathy with the upregulation of Ang (1–7), ACE2 and mas1 along with the modulation of endoplasmic reticulum stress and cardiac apoptosis.55 Furthermore, telmisartan treatment provided cardioprotective effects in dilated cardiomyopathy of mice model through stimulation of ACE2/ANG 1–7/Mas receptor.56 Similarly, olmesartan treatment also ameliorates dilated cardiomyopathy through modulating ACE2/Ang (1–7)/Mas receptor axis.57
(iii) Combinations of angiotensin converting enzyme (ACE) inhibitors and angiotensin-receptor blockers (ARBs)
Interesting, lungs are protected from pneumonitis and fibrosis induced by radiation with the help of ACE inhibitors and ARBs.58 Further, increase of cardio protective biomarkers is associated with combined use of ACE inhibitor, ARBs and beta blocker.59 Notably, increase of cardiac ACE2 activity is associated with combined effect of losartan and lisinopril.20 The inhibition of ACE may result from the total effect of lower angiotensin II formation and metabolism of angiotensin-(1–7) whereas angiotensin II type 1 receptor antagonists may work by raising angiotensin II metabolism by ACE2 in cardiac system.20
Furthermore, under hypoxia conditions, when mouse Lewis lung carcinoma cells are treated with captopril and losartan, an increase in ACE2 and angiotensin II type 2 receptors expression and a decrease in ACE and angiotensin II type 1 receptor expression is observed.60
(iv) Recombinant ACE2
Recombinant human ACE2 is known as therapeutic RAS modulator. Supplementation with recombinant human ACE2 results in lowering of Ang II and increase of Ang-(1–7)/Ang II ratio.61 It has been found to reverse lung injury and lower angiotensin II levels in viral infections.62–64 Additionally, it has been found to play cardioprotective role in heart failure65 and ameliorate kidney fibrosis.66 Further, the diabetic kidney injury is reduced when treated with recombinant human ACE2.67
GSK2586881, a recombinant human ACE2 was found to decrease the level of Ang II with increase of Ang (1–7) in acute respiratory distress syndrome patients.64 Notably, in early stages of SARS-Cov-2 infection, human recombinant soluble ACE2 was found to be active against SARS-CoV-2 virus and blocks this viral infection as found from in vitro studies.68,69
Conclusions and outlook
On the basis of the available evidences, these drugs have the ability to activate the ACE2 expression that had been downregulated in different diseases including hypertension, cardiovascular, renal and lung diseases. As SARS-CoV-2 binds to host ACE2 receptor and downregulates its expression, therefore these drugs either alone or in combination may be repurposed to re-activate the host ACE2 thereby serving as probable SARS-CoV-2 therapeutics. Notably, these drugs may enhance the immune responses; reduce inflammations and organ damage due to COVID-19 complications. Further, these drugs may also reduce the death rate associated with COVID-19. Therefore, repurposing these clinically approved drugs might be a good option. The advantage of repurposing clinically approved drugs are their side effects is known and they might serve as the good candidates for SARS-CoV-2 therapeutics and prophylactics.
Author contributions
BC and SST have conceived the idea and wrote the manuscript.
Funding
There is no funding for this work.
Conflicts of interest
The authors declare no conflict of interest.
Acknowledgements
BC has received the fellowship from Department of Science and Technology (DST) in area of Women Scientist Scheme A (WOS-A).
References
- A. E. Gorbalenya, S. C. Baker, R. S. Baric, R. J. de Groot, C. Drosten, A. A. Gulyaeva, B. L. Haagmans, C. Lauber, A. M. Leontovich, B. W. Neuman, D. Penzar, S. Perlman, L. L. M. Poon, D. V. Samborskiy, I. A. Sidorov, I. Sola and J. Ziebuhr, Nat. Microbiol., 2020, 5, 536–544 CrossRef
. - C. Huang, Y. Wang, X. Li, L. Ren, J. Zhao, Y. Hu, L. Zhang, G. Fan, J. Xu, X. Gu, Z. Cheng, T. Yu, J. Xia, Y. Wei, W. Wu, X. Xie, W. Yin, H. Li, M. Liu, Y. Xiao, H. Gao, L. Guo, J. Xie, G. Wang, R. Jiang, Z. Gao, Q. Jin, J. Wang and B. Cao, Lancet, 2020, 395, 497–506 CrossRef CAS
. - N. Chen, M. Zhou, X. Dong, J. Qu, F. Gong, Y. Han, Y. Qiu, J. Wang, Y. Liu, Y. Wei, J. Xia, T. Yu, X. Zhang and L. Zhang, Lancet, 2020, 395, 507–513 CrossRef CAS
. - Z. Wu and J. M. McGoogan, JAMA, J. Am. Med. Assoc., 2020, 323, 1239–1242 CrossRef
. - Y. Liu, Y. Yang, C. Zhang, F. Huang, F. Wang, J. Yuan, Z. Wang, J. Li, J. Li, C. Feng, Z. Zhang, L. Wang, L. Peng, L. Chen, Y. Qin, D. Zhao, S. Tan, L. Yin, J. Xu, C. Zhou, C. Jiang and L. Liu, Sci. China: Life Sci., 2020, 63, 364–374 CrossRef
. - P. J. Halfmann, M. Hatta, S. Chiba, T. Maemura, S. Fan, M. Takeda, N. Kinoshita, S.-I. Hattori, Y. S. Tagawa, K. I. Horimoto, M. Imai and Y. Kawaoka, N. Engl. J. Med., 2020, 383, 592–594 CrossRef
. - T. H. C. Sit, C. J. Brackman, S. Ming Ip, K. W. S. Tam, P. Y. T. Law, E. M. W. To, V. Y. T. Yu, L. D. Sims, D. N. C. Tsang, D. K. W. Chu, R. A. P. M. Perera, L. L. M. Poon and M. Peiris, Nature, 2020 DOI:10.1038/s41586-020-2334-5
. - P. Zhou, X.-L. Yang, X.-G. Wang, B. Hu, L. Zhang, W. Zhang, H.-R. Si, Y. Zhu, B. Li, C.-L. Huang, H.-D. Chen, J. Chen, Y. Luo, H. Guo, R.-D. Jiang, M.-Q. Liu, Y. Chen, X.-R. Shen, X. Wang, X.-S. Zheng, K. Zhao, Q.-J. Chen, F. Deng, L.-L. Liu, B. Yan, F.-X. Zhan, Y.-Y. Wang, G.-F. Xiao and Z.-L. Shi, Nature, 2020, 579, 270–273 CrossRef
. - C. Vickers, P. Hales, V. Kaushik, L. Dick, J. Gavin, J. Tang, K. Godbout, T. Parsons, E. Baronas, F. Hsieh, S. Acton, M. Patane, A. Nichols and P. Tummino, J. Biol. Chem., 2002, 277, 14838–14843 CrossRef
. - I. Hamming, W. Timens, M. L. C. Bulthuis, A. T. Lely, G. J. Navis and H. van Goor, J. Pathol., 2004, 203, 631–637 CrossRef
. - D. Wrapp, N. Wang, K. S. Corbett, J. A. Goldsmith, C.-L. Hsieh, O. Abiona, B. S. Graham and J. S. McLellan, Science, 2020, 367, 1260–1263 CrossRef
. - M. Ye, J. Wysocki, P. Naaz, M. R. Salabat, M. S. LaPointe and D. Batlle, Hypertension, 2004, 43, 1120–1125 CrossRef
. - G. Wang, F. M.-M. Lai, K.-B. Lai, K.-M. Chow, C.-H. B. Kwan, K.-T. P. Li and C.-C. Szeto, Diabetologia, 2008, 51, 1062–1067 CrossRef
. - G. Y. Oudit, A. M. Herzenberg, Z. Kassiri, D. Wong, H. Reich, R. Khokha, M. A. Crackower, P. H. Backx, J. M. Penninger and J. W. Scholey, Am. J. Pathol., 2006, 168, 1808–1820 CrossRef
. - D. W. Wong, G. Y. Oudit, H. Reich, Z. Kassiri, J. Zhou, Q. C. Liu, P. H. Backx, J. M. Penninger, A. M. Herzenberg and J. W. Scholey, Am. J. Pathol., 2007, 171, 438–451 CrossRef
. - J. Wysocki, D. I. Ortiz-Melo, N. K. Mattocks, K. Xu, J. Prescott, K. Evora, M. Ye, M. A. Sparks, S. K. Haque, D. Batlle and S. B. Gurley, Physiol. Rep., 2014, 2, e00264 CrossRef
. - K. Kuba, Y. Imai, S. Rao, H. Gao, F. Guo, B. Guan, Y. Huan, P. Yang, Y. Zhang, W. Deng, L. Bao, B. Zhang, G. Liu, Z. Wang, M. Chappell, Y. Liu, D. Zheng, A. Leibbrandt, T. Wada, A. S. Slutsky, D. Liu, C. Qin, C. Jiang and J. M. Penninger, Nat. Med., 2005, 11, 875–879 CrossRef
. - C. G. K. Ziegler, S. J. Allon, S. K. Nyquist, I. M. Mbano, V. N. Miao, C. N. Tzouanas, Y. Cao, A. S. Yousif, J. Bals, B. M. Hauser, J. Feldman, C. Muus, M. H. Wadsworth 2nd, S. W. Kazer, T. K. Hughes, B. Doran, G. J. Gatter, M. Vukovic, F. Taliaferro, B. E. Mead, Z. Guo, J. P. Wang, D. Gras, M. Plaisant, M. Ansari, I. Angelidis, H. Adler, J. M. S. Sucre, C. J. Taylor, B. Lin, A. Waghray, V. Mitsialis, D. F. Dwyer, K. M. Buchheit, J. A. Boyce, N. A. Barrett, T. M. Laidlaw, S. L. Carroll, L. Colonna, V. Tkachev, C. W. Peterson, A. Yu, H. B. Zheng, H. P. Gideon, C. G. Winchell, P. L. Lin, C. D. Bingle, S. B. Snapper, J. A. Kropski, F. J. Theis, H. B. Schiller, L.-E. Zaragosi, P. Barbry, A. Leslie, H.-P. Kiem, J. L. Flynn, S. M. Fortune, B. Berger, R. W. Finberg, L. S. Kean, M. Garber, A. G. Schmidt, D. Lingwood, A. K. Shalek and J. Ordovas-Montanes, HCA Lung Biological Network. Electronic address: lung-network@humancellatlas.org; HCA Lung Biological Network, Cell, 2020, 181, 1016–1035.e19 CrossRef
. - I. Hamming, H. van Goor, A. J. Turner, C. A. Rushworth, A. A. Michaud, P. Corvol and G. Navis, Exp. Physiol., 2008, 93, 631–638 CrossRef
. - C. M. Ferrario, J. Jessup, M. C. Chappell, D. B. Averill, K. B. Brosnihan, E. A. Tallant, D. I. Diz and P. E. Gallagher, Circulation, 2005, 111, 2605–2610 CrossRef
. - M. P. Ocaranza, I. Godoy, J. E. Jalil, M. Varas, P. Collantes, M. Pinto, M. Roman, C. Ramirez, M. Copaja, G. Diaz-Araya, P. Castro and S. Lavandero, Hypertension, 2006, 48, 572–578 CrossRef
. - L. J. Burchill, E. Velkoska, R. G. Dean, K. Griggs, S. K. Patel and L. M. Burrell, Clin. Sci., 2012, 123, 649–658 CrossRef
. - L. M. Burrell, J. Risvanis, E. Kubota, R. G. Dean, P. S. MacDonald, S. Lu, C. Tikellis, S. L. Grant, R. A. Lew, A. I. Smith, M. E. Cooper and C. I. Johnston, Eur. Heart J., 2005, 26, 369–375 CrossRef
. - R. N. Vuille-dit-Bille, S. M. Camargo, L. Emmenegger, T. Sasse, E. Kummer, J. Jando, Q. M. Hamie, C. F. Meier, S. Hunziker, Z. Forras-Kaufmann, S. Kuyumcu, M. Fox, W. Schwizer, M. Fried, M. Lindenmeyer, O. Götze and F. Verrey, Amino Acids, 2015, 47, 693–705 CrossRef
. - M. J. Soler, M. Ye, J. Wysocki, J. William, J. Lloveras and D. Batlle, Am. J. Physiol. Renal Physiol., 2009, 296, F398–F405 CrossRef
. - V. Sukumaran, P. T. Veeraveedu, N. Gurusamy, A. P. Lakshmanan, K. Yamaguchi, M. Ma, K. Suzuki, M. Nagata, R. Takagi, M. Kodama and K. Watanabe, Mol. Cell. Endocrinol., 2012, 351, 208–219 CrossRef
. - A. P. Lakshmanan, R. A. Thandavarayan, K. Watanabe, F. R. Sari, H. Meilei, V. V. Giridharan, V. Sukumaran, V. Soetikno, S. Arumugam, K. Suzuki and M. Kodama, Mol. Cell. Endocrinol., 2012, 348, 104–111 CrossRef
. - C. M. Ferrario, S. Ahmad and L. Groban, Nat. Rev. Cardiol., 2020, 17, 378 CrossRef
. - Y. Ishiyama, P. E. Gallagher, D. B. Averill, E. A. Tallant, K. B. Brosnihan and C. M. Ferrario, Hypertension, 2004, 43, 970–976 CrossRef
. - M. Igase, W. B. Strawn, P. E. Gallagher, R. L. Geary and C. M. Ferrario, Am. J. Physiol.: Heart Circ. Physiol., 2005, 289, H1013–H1019 CrossRef
. - J.-C. Zhong, J.-Y. Ye, H.-Y. Jin, X. Yu, H.-M. Yu, D.-L. Zhu, P.-J. Gao, D.-Y. Huang, M. Shuster, H. Loibner, J.-M. Guo, X.-Y. Yu, B.-X. Xiao, Z.-H. Gong, J. M. Penninger and G. Y. Oudit, Regul. Pept., 2011, 166, 90–97 CrossRef
. - V. Sukumaran, P. T. Veeraveedu, N. Gurusamy, K. Yamaguchi, A. P. Lakshmanan, M. Ma, K. Suzuki, M. Kodama and K. Watanabe, Int. J. Biol. Sci., 2011, 7, 1077–1092 CrossRef
. - V. Sukumaran, H. Tsuchimochi, E. Tatsumi, M. Shirai and J. T. Pearson, Biochem. Pharmacol., 2017, 144, 90–99 CrossRef
. - M. Furuhashi, N. Moniwa, T. Mita, T. Fuseya, S. Ishimura, K. Ohno, S. Shibata, M. Tanaka, Y. Watanabe, H. Akasaka, H. Ohnishi, H. Yoshida, H. Takizawa, S. Saitoh, N. Ura, K. Shimamoto and T. Miura, Am. J. Hypertens., 2015, 28, 15–21 CrossRef
. - M. Vaduganathan, O. Vardeny, T. Michel, J. J. V. McMurray, M. A. Pfeffer and S. D. Solomon, N. Engl. J. Med., 2020, 382, 1653–1659 CrossRef
. - G. I. Rice, D. A. Thomas, P. J. Grant, A. J. Turner and N. M. Hooper, Biochem. J., 2004, 383, 45–51 CrossRef
. - Y. Li, Z. Zeng, Y. Li, W. Huang, M. Zhou, X. Zhang and W. Jiang, Shock, 2015, 43, 395–404 CrossRef
. - X. M. Wang, K. Shi, J. J. Li, T. T. Chen, Y. H. Guo, Y. L. Liu, Y. F. Yang and S. Yang, Genet. Mol. Res., 2015, 14, 1707–1717 CrossRef
. - H.-L. Xiao, L.-X. Zhao, J. Yang, N. Tong, L. An, Q.-T. Liu, M.-R. Xie and C.-S. Li, Mol. Med. Rep., 2018, 17, 4221–4228 Search PubMed
. - Y. Chen, F. Song, G. Lu and Z. Lu, Zhonghua Erke Zazhi, 2014, 52, 602–606 Search PubMed
. - Z.-b. Xu, Y.-l. Feng, J. Xiao, Y.-j. Tang and T. Qiu, Sichuan Daxue Xuebao, Yixueban, 2008, 39, 583–587 Search PubMed
. - E. D. C. Frantz, C. Crespo-Mascarenhas, A. R. C. Barreto-Vianna, M. B. Aguila and C. A. Mandarim-de-Lacerda, PLoS One, 2013, 8, e67192 CrossRef
. - X. Wang, Y. Ye, H. Gong, J. Wu, J. Yuan, S. Wang, P. Yin, Z. Ding, L. Kang, Q. Jiang, W. Zhang, Y. Li, J. Ge and Y. Zou, J. Mol. Cell. Cardiol., 2016, 97, 180–190 CrossRef
. - Y.-M. Yuan, L. Luo, Z. Guo, M. Yang, R.-S. Ye and C. Luo, J. Renin-Angiotensin-Aldosterone Syst., 2015, 16, 249–253 CrossRef CAS
. - S. Hagiwara, H. Iwasaka, S. Hidaka, A. Hasegawa, H. Koga and T. Noguchi, Intensive Care Med., 2009, 35, 1471–1478 CrossRef CAS
. - S.-X. Han, G.-M. He, T. Wang, L. Chen, Y.-Y. Ning, F. Luo, J. An, T. Yang, J.-J. Dong, Z.-L. Liao, D. Xu and F.-Q. Wen, Toxicol. Appl. Pharmacol., 2010, 245, 100–107 CrossRef CAS
. - J. Klimas, M. Olvedy, K. Ochodnicka-Mackovicova, P. Kruzliak, S. Cacanyiova, F. Kristek, P. Krenek and P. Ochodnicky, J. Cell. Mol. Med., 2015, 19, 1965–1974 CrossRef CAS
. - G. E. Callera, T. T. Antunes, J. W. Correa, D. Moorman, A. Gutsol, Y. He, A. N. D. Cat, A. M. Briones, A. C. Montezano, K. D. Burns and R. M. Touyz, Biosci. Rep., 2016, 36, e00398 CrossRef
. - J. Iwanami, M. Mogi, K. Tsukuda, X.-L. Wang, H. Nakaoka, K. Ohshima, T. Chisaka, H.-Y. Bai, H. Kanno, L.-J. Min and M. Horiuchi, Hypertens. Res., 2014, 37, 616–620 CrossRef CAS
. - F. Graus-Nunes, F. de Oliveira Santos, T. de Souza Marinho, C. S. Miranda, S. Barbosa-da-Silva and V. Souza-Mello, World J. Hepatol., 2019, 11, 359–369 CrossRef
. - M. Yisireyili, Y. Uchida, K. Yamamoto, T. Nakayama, X. Wu Cheng, T. Matsushita, S. Nakamura, T. Murohara and K. Takeshita, Brain Behav. Immun., 2018, 69, 167–179 CrossRef CAS
. - T. Tsutamoto, K. Nishiyama, M. Yamaji, C. Kawahara, M. Fujii, T. Yamamoto and M. Horie, Hypertens. Res., 2010, 33, 118–122 CrossRef CAS
. - T. Tanno, H. Tomita, I. Narita, T. Kinjo, K. Nishizaki, H. Ichikawa, Y. Kimura, M. Tanaka, T. Osanai and K. Okumura, J. Cardiovasc. Pharmacol., 2016, 67, 503–509 CrossRef CAS
. - M. H. M. Yousif, G. S. Dhaunsi, B. M. Makki, B. A. Qabazard, S. Akhtar and I. F. Benter, Pharmacol. Res., 2012, 66, 269–275 CrossRef CAS
. - S. Arumugam, R. A. Thandavarayan, S. S. Palaniyandi, V. V. Giridharan, W. Arozal, F. R. Sari, V. Soetikno, M. Harima, K. Suzuki, M. Kodama and K. Watanabe, Toxicology, 2012, 291, 139–145 CrossRef CAS
. - V. Sukumaran, P. T. Veeraveedu, N. Gurusamy, A. P. Lakshmanan, K. Yamaguchi, M. Ma, K. Suzuki, M. Kodama and K. Watanabe, Life Sci., 2012, 90, 289–300 CrossRef CAS
. - V. Sukumaran, P. T. Veeraveedu, A. P. Lakshmanan, N. Gurusamy, K. Yamaguchi, M. Ma, K. Suzuki, M. Kodama and K. Watanabe, Free Radic. Res., 2012b, 46, 850–860 Search PubMed
. - A. Molteni, L. F. Wolfe, W. F. Ward, C. H. Ts'ao, L. B. Molteni, P. Veno, B. L. Fish, J. M. Taylor, N. Quintanilla, B. Herndon and J. E. Moulder, Curr. Pharm. Des., 2007, 13, 1307–1316 CrossRef CAS
. - D. Lezama-Martinez, J. Flores-Monroy, S. Fonseca-Coronado, M. E. Hernandez-Campos, I. Valencia-Hernandez and L. Martinez-Aguilar, J. Cardiovasc. Pharmacol., 2018, 72, 291–295 CrossRef CAS
. - L. Fan, Y. Feng, H. Y. Wan, L. Ni, Y. R. Qian, Y. Guo, Y. Xiang and Q. Y. Li, Genet. Mol. Res., 2014, 13, 10562–10573 CrossRef CAS
. - R. Basu, M. Poglitsch, H. Yogasundaram, J. Thomas, B. H. Rowe and G. Y. Oudit, J. Am. Coll. Cardiol., 2017, 69, 805–819 CrossRef CAS
. - Z. Zou, Y. Yan, Y. Shu, R. Gao, Y. Sun, X. Li, X. Ju, Z. Liang, Q. Liu, Y. Zhao, F. Guo, T. Bai, Z. Han, J. Zhu, H. Zhou, F. Huang, C. Li, H. Lu, N. Li, D. Li, N. Jin, J. M. Penninger and C. Jiang, Nat. Commun., 2014, 5, 3594 CrossRef CAS
. - H. Gu, Z. Xie, T. Li, S. Zhang, C. Lai, P. Zhu, K. Wang, L. Han, Y. Duan, Z. Zhao, X. Yang, L. Xing, P. Zhang, Z. Wang, R. Li, J. J. Yu, X. Wang and P. Yang, Sci. Rep., 2016, 6, 19840 CrossRef CAS
. - A. Khan, C. Benthin, B. Zeno, T. E. Albertson, J. Boyd, J. D. Christie, R. Hall, G. Poirier, J. J. Ronco, M. Tidswell, K. Hardes, W. M. Powley, T. J. Wright, S. K. Siederer, D. A. Fairman, D. A. Lipson, A. I. Bayliffe and A. L. Lazaar, Crit. Care, 2017, 21, 234 CrossRef
. - V. B. Patel, J. N. Lezutekong, X. Chen and G. Y. Oudit, Can. J. Cardiol., 2017, 33, 943–946 CrossRef
. - E. H. Bae, F. Fang, V. R. Williams, A. Konvalinka, X. Zhou, V. B. Patel, X. Song, R. John, G. Y. Oudit, Y. Pei and J. W. Scholey, Kidney Int., 2017, 91, 1347–1361 CrossRef CAS
. - G. Y. Oudit, G. C. Liu, J. Zhong, R. Basu, F. L. Chow, J. Zhou, H. Loibner, E. Janzek, M. Schuster, J. M. Penninger, A. M. Herzenberg, Z. Kassiri and J. W. Scholey, Diabetes, 2010, 59, 529–538 CrossRef CAS
. - V. Monteil, H. Kwon, P. Prado, A. Hagelkrüys, R. A. Wimmer, M. Stahl, A. Leopoldi, E. Garreta, C. H. D. Pozo, F. Prosper, J. P. Romero, G. Wirnsberger, H. Zhang, A. S. Slutsky, R. Conder, N. Montserrat, A. Mirazimi and J. M. Penninger, Cell, 2020, 181, 905–913.e7 CrossRef CAS
. - F. Alhenc-Gelas and T. B. Drueke, Kidney Int., 2020, 97, 1091–1093 CrossRef CAS
.
|
This journal is © The Royal Society of Chemistry 2020 |
Click here to see how this site uses Cookies. View our privacy policy here.