DOI:
10.1039/D3RA02104A
(Review Article)
RSC Adv., 2023,
13, 26455-26474
Phytoremediative adsorption methodologies to decontaminate water from dyes and organic pollutants
Received
31st March 2023
, Accepted 9th August 2023
First published on 5th September 2023
Abstract
Persistent organic pollutants and dyes cause major problems during ecofriendly wastewater treatment. To overcome this huge problem, several techniques have been considered and in practice for the safe disposal of organic pollutants in recent years; some of them are discussed and compared herein. This review focuses on new trends for wastewater treatment and compares them with certain other techniques alongside their pros and cons; adsorption is considered the safest among them. Adsorbents derived from agri-wastes have good capacity for the removal of these contaminants owing to their great sorption capacity, high reusability, easy operation, etc. Sometimes they need some modifications for the removal of dyes, which are also discussed in this review. This capacity of adsorbents to chelate dye molecules can be affected by factors, such as pH, the concentration of dyes and adsorbents, and temperature of the system. pH has direct influence on the ionization potential and charge on the outer surface of adsorbents. The findings on isotherms, kinetics, and desorption of plant waste-based biomaterials that are safe for the ecosystem and user friendly and are used for hazardous contaminant removal from water are summarized in this review. Finally, conclusions and future perspectives are presented, and some other materials, such as CNTs and MOFs, are also discussed as efficient adsorbents for eliminating dyes from wastewater. Finally, it is predicted that the adsorption of dyes is a more feasible solution for this dye pollution problem.
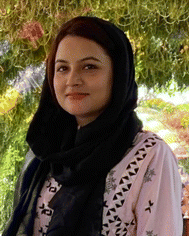 Ayesha Kanwal | She is a PhD Scholar in the Centre for Inorganic Chemistry, PUSC. She has also worked as a research assistant in analytical laboratories of PUSC and as a Chemistry Lecturer in a prestigious institute. Her research interest primarily focuses on the development of cost-effective and eco-friendly porous materials for the elimination of pollutants from effluents. She has also published a number of research articles in the field of inorganic synthesis and waste water treatment. |
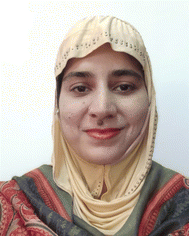 Rabia Rehman | She is currently working as Assistant Professor in the Centre for Inorganic Chemistry, PUSC. She has been working in the field of inorganic chemistry for more than 15 years. Her research mainly focuses on the development of analytical procedures and synthesis of new cost-effective materials from indigenous resources to remove toxic materials from water by green methodologies. She is the author of more than 135 research articles and 7 books. She is an editor of many reputed journals and an active researcher in her field. |
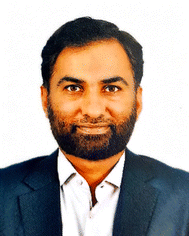 Muhammad Imran | He is currently working as Associate Professor and Director in the Centre for Inorganic Chemistry, PUSC. He has more than 120 research publications and vast experience in the synthesis of organometallic compounds and wastewater treatment by biosorption. |
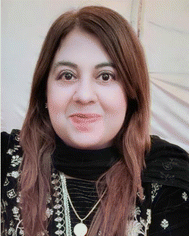 Ghufrana Samin | She is an Associate Professor of Chemistry at UET, Faisalabad campus. She has published more than 25 research papers. Her field of research is the removal of dyes from wastewater, drug development, and analytical techniques for organic compound analysis. |
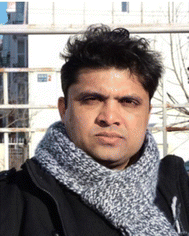 Muhammad Muzammil Jahangir | He is working as Associate Professor at the Institute of Horticultural Sciences, UAF, Pakistan. His field of interest is horticulture, specifically focusing on the production, processing, and storage of vegetable crops for their applications in sustainable industrial products and safe disposal of pollutants from industrial effluents. |
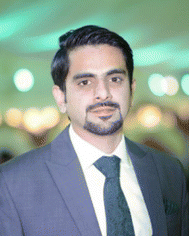 Saadat Ali | He holds a BS from UET Taxila and is currently employed at Brunei Fertilizer Industries as an Analytical Instrumentation Specialist. He has extensive experience in the field of analytical instruments, such as process gas chromatographs, spectrophotometers, and mass spectrometers. |
1. Introduction
At present, owing to the rapid growth of industries, the provision of clean water and food is a problem across the world. As many countries, especially in South Asia and Africa, including Pakistan, India, Bangladesh, Serbia, and Ethiopia, are shifting towards industrial revolutions, their problem of water pollution is getting worse. Being one of the major contributors in the textile sector, textile-related pollution factors are very common in developing countries, which are the ultimate source of water pollution here. Some of the chief polluting agents discharged from such industries are coloring agents or dyes. These dyes are exclusively used in many other industries, such as leather tanning, paper, and sugar industries.1,2 The discharge of colored substances from all such industrial units into water bodies causes severe contamination.3–5 The increasing water contamination poses a serious threat to human health because of the poor waste water management systems present in these countries.
The origin of the textile industry dates back 4000 years. The process of alcohol production by sugar fermentation and dyeing of cloths are two prominent fields that are known to be antique. The root of fabric dyeing stretches back to the ancient Egyptian civilization in which people were aware of dyeing using insect extracts and some naturally derived pigments, such as indigo. This happened to be in practice until the middle of the last century. The first synthetic dye was invented in 1856 by William E. Perkin, from the oxidation of aniline.6,7 Today, tens of thousands of synthetic dyes are prepared and more than 1000 dyes are available on a commercial scale. Textile industries use a major portion of the synthetic dyes produced.
The use of synthetic dyes has drastically increased in many domains of our daily life and it is continuously increasing with the growth of industries, such as paper, leather, cosmetic, and paint.8 About 10
000 diverse types of dying pigments are used in industries. There is a massive annual production of 0.7 million tons of dyeing agents worldwide.9 Out of which 3 × 105 tons of dyeing agents are utilized in the textile sector, which makes them the main user of these dyeing stuffs. Therefore, these industries directly contribute to the pollution of water with a production of 7 × 105 tons of colored untreated waste water discharged directly into water bodies annually. A study revealed that almost 2% of dyes are discharged as effluents from the manufacturing sector, 10% of which are discharged from the textile sector.10,11 This untreated water coming out of industries and directly dumped into water bodies causes huge distress to the aquatic flora and fauna of the area. This results in reduced sunlight penetration due to the thin layer of dye formed over the water surface. This affects the photosynthetic process of plants, resulting into an imbalanced food chain in the aquatic life cycle and causing a decline in dissolved oxygen of water bodies that makes the water unsuitable for consumption.12–14 These effluents discharged also affect the process of seed germination if they penetrate the nearby agricultural land, and production declines consequently.15,16
Industries, especially the textile industry being a big culprit, are responsible for this contamination of water because of their bulk contribution to dye pollution, as studies showed that more than 50% of dyes used in these industries get washed off into the drain. This is due to the weak bonding or inadequate dye-fiber attraction. The consumption of this water containing effluents affects the consumer irrespective of whether it is used for a bathing, washing or drinking purpose.17 It is very important to monitor the concentration of coloring agents or dyes present in water to be consumed, as even 1.0 mg L−1 of dye can be dangerous for human consumption.18 Moreover, it causes toxicity to the aquatic ecosystem and mutagenic and carcinogenic effects in humans, such as damage to the kidney, liver, brain, central nervous system, and reproductive system has been reported to be caused by contaminated water.19–21
Textile dyes widely used in the industry reportedly have various detrimental effects on humans and other living creatures along with the environment, and their discharge into water bodies directly and indirectly affect a wide range of flora and fauna (Table 1).22,23 Various natural and synthetic dyes effect negatively at some stage of their degradation cycle, which may be as parent molecule or derived metabolites, being carcinogenic and skin irritant, these dyes lead in destroying human health and also affect other living creature.24–27 The life of an average person gets affected by even 1 mg L−1 of dye in water, which is associated with the direct discharge of effluent-containing wastewater into water bodies.26,28–30 This affects the level of DO (dissolved oxygen) and light penetration to the life present underneath. Poor light penetration and less dissolved oxygen in water bodies result in the disruption of the aquatic life cycle.31,32 Various heavy metals, such as Al, Cu, Pb, Hg, Fe, Co, As, and Zn, are also related to textile dye molecules and responsible for a number of ill effects on human health, including different cancers, dementia, neural toxicity, and organ failure.33
Table 1 Detrimental effects of various dyes on life and environment
Textile dye |
Dye class |
Detrimental effects |
Disperse blue 56 |
Anthraquinone |
Aquatic biota and human fitness34 |
Disperse blue 291 |
Azo dye |
Apoptosis, DNA destruction35 |
Reactive brilliant red |
— |
Disrupts human protein function36 |
Disperse red 1 |
Azo dye |
Destruction of DNA37 |
Acid violet 7 |
— |
Lipid degradation, abnormal chromosomal activity, acetylcholine degeneration in mice38 |
Disperse orange 1 |
Azo dye |
Micronuclei formation in human lymphocytes39 |
Astrazon red |
Azo dye |
Glutathione levels decline and carboxylesterase rises in Phanerochaete chrysosporium40 |
Ramazol turquoise blue G-A |
Phthalocyanine |
Rise of glutathione s-transferase in Xenopus laevis tadpoles40 |
Cibacron red FN-3G |
Double azo |
Glutathione s-transferase inhibition in Xenopus laevis40 |
Benzopurpurin 4B |
Azo class |
Destroy aquatic biota41 |
Yellow Favina CXL |
— |
Weak estrogen agonist response41 |
Reactive dyes 3BS |
|
Disruption in functionality of liver and gill tissues of Oreochromis niloticus42 |
Direct blue 15 |
— |
Mutagenic in nature43 |
Disperse blue 291 |
|
Mutagenic, genetic and cellular toxicity, DNA disruption44 |
Reactive black 5 |
|
Declines urease function, reduced ammonification rate in soil45 |
Tartrazine |
Azo dye |
Dermatitis, anaphylaxis in nature46,47 |
Acid red 13 |
Azo dye |
Damages DNA chain48 |
In recent years, a great shift has been observed towards environmental protection and huge concern was seen regarding the importance of conservation of environment. It is a need of time, or the price will be borne by the upcoming generations, as small quantity of effluents (dyes) can drastically affect the environment, such as water bodies.49 Dye contamination is a potential threat; hence, current governments are trying to pay attention to wastewater treatment, and there is still a lot to be done. As we need a constant improvement in the development of effective procedures with promising results, various techniques (Fig. 1) including microbial degradation (aerobic and anaerobic), coagulation, membrane separation, and chemical oxidation are in practice for the removal of dyes, and each of the techniques has its own limitation (summarized in Table 2).50 All the above-mentioned techniques are considered effective but are difficult to use and expensive. Moreover, the reasons to get diverted towards biological approaches for environmental protection include inexpensive nature, more effectivity, lesser amount of sludge production and better environment-friendliness than the chemical treatments.7 Among these practices, adsorption has the advantages of low cost and better effectivity for effluent removal, which make it desirable.51
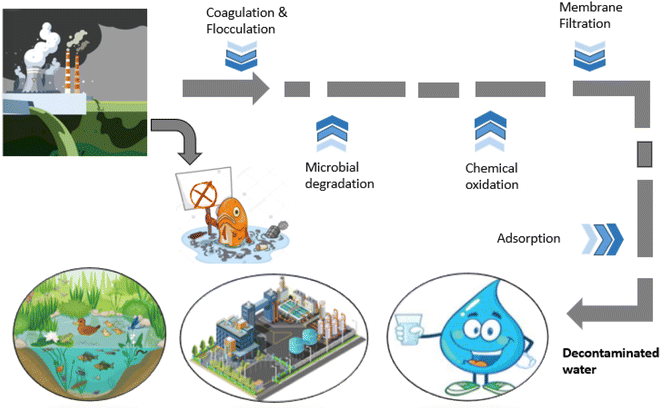 |
| Fig. 1 Multi-steps involved in the removal of contaminants from industrial effluents. | |
Table 2 Comparison of various techniques in practice62
Technique |
Benefits |
Drawbacks |
Fenton's reagent (oxidation) |
Simple implementation |
Production of slush |
Ozonation (introducing gaseous ozone) |
Water volume does not elevate |
Brief half-life (nearly 20 min) |
Photochemical degradation |
Unpleasant smell reduced |
Number of by-products generated |
No slush accumulation |
Biological treatments (using living organisms i.e. fungi) |
Discoloration of dyes by enzyme production |
Release of enzymes uncertain |
Membrane separation |
Complete dye eradication |
Large amount of sludge production |
Ion exchange |
Complete regeneration of adsorbent |
Ineffective for all dye types |
Coagulation |
Low in cost |
Large sludge production |
Adsorption (activated carbon) |
High efficacy |
Economically not feasible |
Photodegradation |
Non-hazardous, high kinetics of photochemical reaction |
Low efficacy, less solar sensitivity |
Photo-Fenton/Advance oxidation |
Less slush production, effective for high volume |
Large Ferrous sludge production |
Water splitting |
Clear process, economical, use solar energy |
Backward reaction, side reaction |
The sorptive removal of contaminants using agricultural wastes is considered more natural and cheaper than adsorbents prepared chemically, as these by-products appear to be quite effective against certain pollutants, such as heavy metals,52,53 phenols,54,55 gases,56 and dyeing agents.57–59 Extensive research has been done to improve the adsorption capacity of natural adsorbents, and various techniques, including chemical and physical activation, i.e., carbonization, are applied. Generally, the Langmuir isotherm is applied to determine the progress of activation. Water coming out of industries is a combination of various effluents, such as heavy metals, salts, dissolved solids, and dyes, and hence, various adsorbents have been applied so far both as untreated and treated with certain activation agents.60,61
1.1. Classification of dyes
Dye molecules are composed of two essential constituents, namely, chromophore and auxochrome. Chromophore acts as a color producing agent, while auxochrome is responsible for the attraction between dye molecules and fibers.63 Following are some of the major classes of dyes used in textiles: basic dyes, acidic dyes, azo dyes, sulfur dyes, mordant dyes, and vat dyes, among which azo dyes cover a huge ratio of dyes applied (Fig. 2).64,65 Dyes can be classified as cationic (basic) dyes and anionic dyes that include acid, reactive, and direct dyes.66
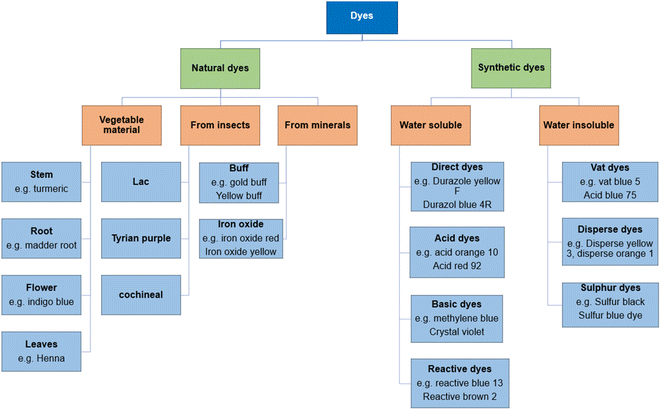 |
| Fig. 2 Classification of dyes according to their origin7. | |
1.1.1. Cationic dyes. Cationic dyes are also known as basic dyes and usually dependent on their positive counter parts, i.e., hydrochloride and zinc chloride complexes.67 These dyes possess positive functionality and release more positive ions (colored cations) in water. This type of behavior is observed in many types of dyes, including azo dyes, solvent dyes, numerous polycarbocyclic dyes, and methane dyes. They also include anthraquinone dyes considered as weak and expensive in comparison to azo dyes, which reduce the cost of dyeing.68 Basic dyes are preferred, on the basis of their brilliancy and vibrant nature, and these dyes, such as brilliant green and methylene blue, are also utilized as a template for dye removal studies. These dyes are extensively used in silk, nylon, and wool dyeing despite considered harmful.66 They are responsible for various medical conditions, such as skin allergies (dermatitis), genetic mutations, and various cancers.69 Methylene blue is one of the commonly used basic dyes in textiles, which can be responsible for heart diseases, quadriplegia, tissue necrosis, and digestive tract disorders in humans.70 Many different research studies are available, in which agriculture wastes are utilized for the removal of methylene blue dye from water, for example, coconut shell (Qmax = 277.9 mg g−1),71 neem leaf (Qmax = 351 mg g−1),72 guava leaf (Qmax = 295 mg g−1),73 and peanut husk (Qmax = 123.5 mg g−1).74
1.1.2. Anionic dyes. Anionic dyes are classified based on the production of negative ions, and this group includes a large variety of diverse dyes sharing a common factor of producing negative ions and water solubility, for example, azo, nitro and triphenyl methane dyes. This group also contains numerous reactive dyes.67,68 Reactive dyes are generally used in wool and cotton dyeing due to their covalent bonding between the reacting entities and fabrics. These reactive dyes when ejected into the aquatic environment become hazardous due to their efficient hydrolyzing ability and are difficult to be fixed.75 Anionic dyes are excessively used in coloring of silk, wool, and synthetic fabrics (polypropylene, polyamide, and modified acrylic fabric). Being effectively soluble in water, these dyes are very efficient for dyeing but have deadly effects on humans owing to their sulfonic nature.76 A number of different dye adsorbents have been applied by the researchers for the removal of acid dyes, such as Reactive Yellow 4 (RY4) by apatitic tricalcium phosphate and apatitic octacalcium phosphate,77 Brilliant yellow using sepiolite,78 Acid black 26, Acid green 25, and Acid blue 7 by pine cone,79 and Acid blue 80 by bagasse ash.80 Recent developments have been seen inclined towards natural adsorbents, such as agro wastes, e.g. mango seed, soy meal, and bamboo, owing to their inexpensiveness and low maintenance.81–83
1.2. Adsorption: an alternative of classical dye elimination procedures
A large diversity of techniques, such as membrane filtration, flotation linked flocculation, ozonation, oxidation, and coagulation, have been employed for the removal of dyes (presented in Table 2),50,84 all of which have drawbacks individually, including slush accumulation, brief life span, huge amounts of side products, and collection of large lumps of sludge.85 Adsorption is feasible at both laboratory and industrial levels, being a surface manifestation related to a visible elevation of one component at the boundary of two phases.86,87 The adsorption procedure is directly linked to the characteristics of dyeing agents and the surface interaction of the adsorbent depending on its type.88 A number of different adsorbents are practically applied in the removal of dyes from waste water and few of them are discussed below and in Fig. 3.
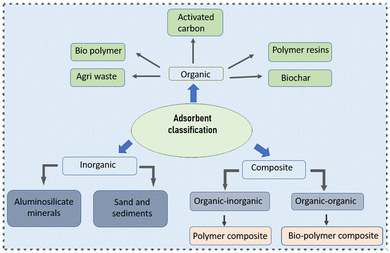 |
| Fig. 3 Classification of adsorbents used for the removal of dyes. | |
1.2.1. Activated carbon. Activated carbon is extensively used for the removal of dyes from the discharged water. Sources of activated carbon include coal, sawdust, and charcoal, and these are pretreated by fractional oxidation to get a porous surface that can act as an adsorbent. These activated carbons are of two types: hydrophilic (water loving) and hydrophobic (water repelling).89,90 Activated carbon is available in different forms: (a) granular activated carbon (GAC), (b) powdered activated carbon (PAC), and (c) activated carbon tablets, and all these are very effective against effluent removal. GAC is efficient owing to its compliance for the contacting substance and not necessary to remove from a large volume of water. Besides, it showed little intra-particulate interaction. PAC composed of smaller size particles, which are less costly and offers large surface interaction; but the problem with them is their slow diffusion ability and safe removal from wastwater after use.91,92 Others like activated carbon tablets are usually obtained from coal and polymeric substances.63 Although this technique is efficient, it is costly and unaffordable for low-resource countries facing water pollution.93
1.2.2. Metal organic frameworks (MOFs). Metal organic frameworks (MOFs), a class of porous materials that are crystalline in nature, are extensively used in the separation of substances, with effective pore size and high surface area (above 6000 m2 g−1). Multidimensional design, the uniform size of pores, and high surface area make MOFs potential adsorbents for catalysis and chemical separation.94,95 MOFs have good capacity of self-assembly, which supports the uptake of relatively large molecules, such as dye molecules. There are several studies in literature of MOFs being used in the removal of dyes, including malachite green, methylene blue, Rhodamine B, xylenol orange, and methyl orange.94,96–100
1.2.3. Nanomaterials and composites. Nanomaterials and composites of various kinds, including metal nanoparticles, carbon nanomaterials (composites and nanotubes), biologically derived nanomaterials, and biochitosan, are widely used in dye removal owing to their better pore volume and surface capacities.101 Nanomaterials (CNTs and carbon nanotubes) show good efficiency in dye removal, but handling them is challenging, which encourages their use in composite forms, such as magnetic nanomaterials, graphene oxides (GOs), and cyclodextrin.102 Various studies in literature have demonstrated successful dye removal using magnetic nanocomposites, including methylene blue removal with β-cyclodextrin carrying CNTs and Fe3O4 nanoparticles carrying CNTs.103,104
1.2.4. Agri solid wastes. Agricultural debris obtained in large quantities every year was found to be very inexpensive, organic in nature, and efficient adsorbents of pollution.105 This agri-waste is composed of lignocellulosic matter, which is made up of lignin, cellulose, and hemi cellulose. Various adsorbents derived from agricultural waste have been reported previously, which effectively remove dyes from waste water. With increasing effluent or waste water from industries, various methods were applied for their removal to protect the ecosystem.106 Agricultural wastes are renewable and inexpensive and require no or very little pretreatment and show excellent adsorption capacity for certain dyes.107Palm oil has quite a big industrial demand that is directly linked to its production and solid debris produced every year, which can be used in dye removal from waste water. The by-products include palm shell,108 palm kernel fiber, and shell.109–111 Similarly, coconut is produced almost in the entire of southeast Asia and has been extensively used in adsorption studies in the form of coconut husk,112 coconut sawdust,113 coconut coir dust,108 etc.
Apart from that, there are plenty of other agricultural wastes having potential adsorption ability but are rarely used, for example, Citrullus lanatus peel (water melon),114 Psidium guajava leaves (guava),115 castor seed shell,116 activated lemon peel/sodium alginate composite,117 and activated carbon from kiwi, cucumber, and potato peel.118
1.3. Modification/synthesis of adsorbents
The performance and efficacy of an adsorbent are characterized by factors, such as (a) large surface area, (b) high penetrability with small-sized pores, (c) thermal and chemical stability, and (d) good mechanical strength.119 All these factors play positively towards the efficiency of adsorbents. Certain surface modification techniques are also in practice related to the enhancement of the adsorption capacity of adsorbent materials that revise the physical, biological, and chemical behaviors of substances (Fig. 4).120 Adsorbent characteristics get enhanced by modifying the surface charge, roughness, surface area, and porosity, and these modifications are carried out by thermal, chemical, or mechanical procedures. Physical modification treatments favor enhancing the porosity, density, and solubility, while chemical treatments (involving alkali, acid, salt etc.) help in increasing the surface area.121,122 Among the physical and chemical modification techniques, chemical treatment gives better results, as it directly influences the surface of adsorbents and not time-consuming along with some additional surface characteristics. These modifications can convert a low-cost raw material into an efficient adsorbent.123,124
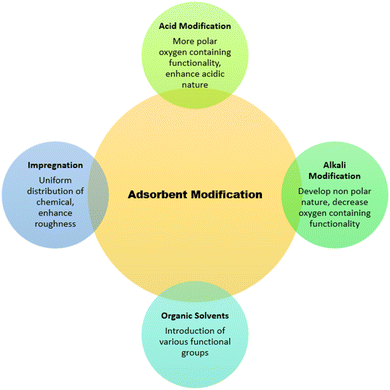 |
| Fig. 4 Types of modification methods of adsorbents and their effects on properties. | |
1.3.1. Acid treatment. The use of mineral acids and oxidizing agents, such as HCl, H3PO4, H2O2, and HNO3, for improving the adsorbent behavior of materials is termed as acid treatment or wet oxidation modification.125 This treatment enhances the hydrophilic quality and acidic surface of adsorbents due to the reduction of mineral matter present.126 It increases the porosity of surface by increasing polar O containing functional moieties, such as carbonyl, carboxyl, and lactone. Moreover, the accumulation of H+ on the adsorbent surface promotes the uptake of negatively charged effluents.127,128 The modification of brown algae by H2SO4 leads to the formation of cylindrical porous structures on the adsorbent surface due to release of trapped gases and the porosity of the adsorbent enhanced, resulting in better adsorption ability.129
1.3.2. Alkali treatment. The introduction of reducing agents, such as LiOH, KOH, and NaOH oxides, increases the non-polar nature of the adsorbent surface. The distribution of negatively charged particles on the surface of adsorbent results in better uptake of Toluidine Blue dye on alkali-treated fuller's earth surface.130 The alkali (NaOH) treatment of activated carbon results in better pore volume and enhanced surface area, which is caused by the reduction of O-containing functional moieties.131
1.3.3. Impregnation. The process of uniform dispersion of chemical substances, such as metals, carbonates, and chlorides, onto the porous adsorbent material is termed impregnation.121 Impregnation elevates the practicality in terms of stability, adsorption ability, and regeneration of adsorbents. Rehman et al., presented that Zr-impregnated activated carbon showed better porosity, thermal stability, and O functionality.126
1.3.4. Organic solvents. Organic solvents, such as alcohols, can be utilized to enhance the adsorption ability and introduction of some more functional moieties. However, owing to the unstable nature and high cost, organic solvents have limited applications in the adsorption process.132A number of other chemical substances are used in the modification of adsorbents other than those mentioned above, which include neutral solutions and oxidants. Wood biochar modified by KMnO4 resulted in a more oxygen-containing porous surface coated with MnOx and showed better uptake of Pb(II), Cd(II), and Cu(II).133
2. Characterization of adsorbents
The characterization of adsorbents shows a number of key features to be considered, including pore size, particle size, pore volume, and morphology. These key factors are directly related to the basic physical properties and behavior of adsorbent materials and are usually characterized using BET, XRD, TEM, and SEM analyses, etc.
2.1. Surface area
As the surface area plays a fundamental role in the adsorption efficiency of adsorbents, it is characterized by BET analysis.134–138 The properties of adsorbents significantly depend on the materials used and modification methods applied for production. Activated carbon analyzed with BET may exhibit a surface area of 2630 m−2 g−1, and similarly, the pore volume may vary up to 1.91 m3 g−1.139,140 However, zeolites may show a surface area around 2300 m2 g−1 with a high proportion of micropores.141,142
Carbon nanotubes and graphene adsorbents show relatively high consideration based on their modification abilities and more surface area as 1000 m2 g−1 based on the composite type and preparation method.143,144 Unlike all these adsorbents, the above-mentioned polymeric adsorbents present a smaller pore size based on the polymerization conditions applied. The pore size is inversely proportional to surface area, still it favors the passage of adsorbates onto adsorbents.145
2.2. Morphology
The morphology, crystal structure, and topography of the adsorbent material are key features in adsorbate uptake onto the adsorbent and are characterized using scanning electron microscopy (SEM), a technique exclusively applied to determine the microstructure of materials.146 Adsorbents with both micro and mesoporous structures can be visualized by SEM images along with the change in the morphology of adsorbents with any type of chemical or physical treatment and adsorbates loaded in the adsorption/desorption cycle validating the stability of adsorbents. CNTs, MOFs, minerals, biomass, and other synthetic adsorbents can be studied morphologically using SEM and this morphology contributes to the designing of the mode and mechanism of adsorbate uptake.146–149
2.3. Particle size
The size, shape, and cluster formation of particles help in understanding the behavior of adsorbent materials in adsorbate solutions and approaches to get them separated, especially materials with layered arrangement, such as kaolinite.150 This characteristic is effectively studied using TEM analysis, describing the shape and size of the particles and the cluster formation of adsorbents and their distribution on solid beds.151–154 Smaller sized adsorbents, such as nanocomposites and graphene-derived adsorbents can be monitored before and after any treatment to determine the defects and other changes associated with layering, particle size, shapes of molecules, and cluster formation.143
3. Parameters affecting dye removal
3.1. Effect of solution pH
The acidity or basicity of a solution is a termed the pH of the solution. The pH of a solution has great influence on the adsorption process by controlling the degree of electrostatic charge produced by the ionization of dye particles. This, in turn, controls the adsorption rate of dyes.155 The pH influence on the adsorption process is studied by introducing a known amount of adsorbent and dye within the fixed range of pH attained by adding NaOH (1 M) or HCl (1 M) solutions. Normally, an increasing adsorption trend is observed for the adsorption of cationic dyes with an elevation in pH. Similarly, a decline in the percentage dye removal for anionic dyes is witnessed and vice versa. This phenomenon is strengthened as the positive potential of the solution decreases with the increase in pH and the surface of adsorbents tends to be negative and more supportive towards cationic dye molecules to be carried.156 While at a low pH, the negative potential of the adsorbent declines and the positive interface improves anionic dye adsorption. Extensive research has been carried out on dye behavior towards the pH change of the solution, i.e. reactive black 5 at sunflower seed shell (adsorbed minimum at 4 pH),157–160 Gemazol turquoise blue-G at sugar beet pulp (adsorption noticed zero at 6 pH),161 methylene blue (MB) dye at banana stalk (adsorption max. at 4 min at 2 pH),162 etc.
Adsorption capacity and active points on the adsorbent surface are determined by the linear span of pH sensitivity, which is termed pHpzc (point of zero charge) or pHIEP (iso electronic point).163 To study the behavior of adsorbents coming from agricultural sources, iso-electronic point has been investigated by many researchers. Cationic dyes have more favorable adsorption condition, where the pHpzc ranges more towards acidic side, as the surface becomes negatively charged, while the basic side of pHPzc range favors anionic dye adsorption.164,165
The pHpzc is found by taking a range of fixed initial pH values having a known amount of adsorbent added, kept on shaking until equilibrium and the final pH is noted. A graph between pHinitial and pHfinal gives a point where pHinitial = pHfinal considered as an iso electronic point.166
3.2. Effect of dye concentration
The percentage removal of dyes is directly associated with the initial amount of dye introduced. To determine the impact of initial dye concentration, solutions containing a known concentration of adsorbent were introduced with different doses of dyes and were shaken until equilibrium is attained. The initial concentration of dye introduced is directly related to the availability of binding sites on the surface of adsorbents. Normally, a descending pattern is observed as the concentration of dye introduced initially increased that is linked to the number of binding sites occupied with dye molecules.167 It is said that the lower the concentration of dye molecules the higher will be the active sites of adsorbents available; however, it favors the loading ability of the adsorbent, which increases induced by the mass transport of dye molecules.72,168 Garg et al.169 suggested that the unit adsorption of methylene blue (MB) on sulfuric acid-modified sawdust increased when the MB concentration was increased (50 mg L−1 to 250 mg L−1) under optimized conditions, while a decline in percentage adsorption was also observed. Previous literature affirms the statement that both anionic and cationic dyes show decreased adsorption whenever the dye concentration increases in the solution. As the actual industrial waste water possesses more dye concentrations than that is mentioned in literature, researchers work on empirical schemes founded on equilibrium adsorption conditions (given in Fig. 5) to calculate the size and the functioning of adsorbent materials. These empirical schemes are of two types: one-step design and two-step design; it was observed that few dyes took more adsorption time for similar adsorption capacity in the one-stage design, while they are more efficient in the two-stage design. This also reduces the process cost.169–171
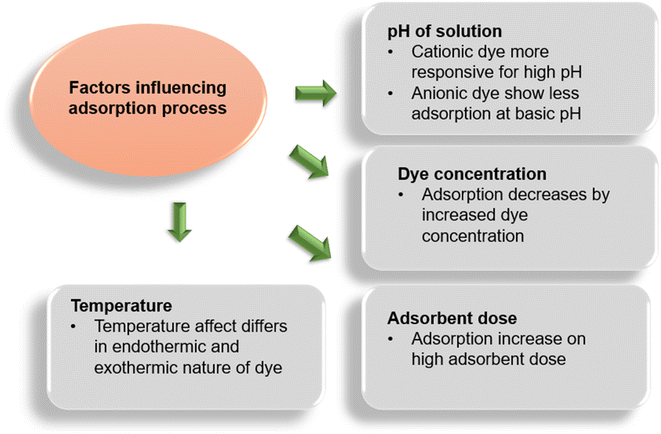 |
| Fig. 5 Influencing parameters of the adsorption process. | |
3.3. Effect of the adsorbent dose
The impact of variable dose concentration is determined by taking adsorbate solutions under optimal conditions with different concentrations of adsorbents and shaken till equilibrium. Generally, an ascending trend in dye removal is seen with the increase in adsorbent dose, which affects dye uptake positively.172 Studying the impact of dose change helps in determining the ability and efficacy of the adsorbent used, which eventually helps in economic perspective. Literature also supports the fact that the dose increase has positive effects on dye removal and vice versa for both cationic and anionic types of dyes.173–175
3.4. Effect of temperature
The influence of temperature on the adsorption of dye is determined by shaking the adsorbent–adsorbate solutions while keeping the other factors constant at different temperatures till the equilibrium is reached. The temperature indicates the nature of dye material, if the adsorption ability of adsorbents elevates with the elevating temperature, then the dye is considered as endothermic because this increase on dye uptake is referred to as increased kinetic energy of the molecules and increased availability of active sites of adsorbents and vice versa.176,177 Sometimes adsorption capacity may decrease with the increase in temperature that depends on the class of dye. As interacting forces between dye particles and the binding sites may decrease with the increase in temperature.109,178,179 A study carried out by Senthilkumaar et al.176 showed that the adsorption ability of activated carbons (by H2SO4 and H3PO4) from male coconut flowers increases by elevating the temperature. Similar results were found by studies of methylene blue, malachite green, and crystal violet with apricot180 and methylene blue with garlic peel.181 The class of dye also plays an important role along with temperature, as studies showed that cationic dyes show endothermic nature while anionic dyes depict the exothermic behavior, e.g. 4-bromoanilineazo-1,8-di-hydronaphthalene-3,6-di-sodium sulphate (BDH) showed exothermic behavior on adsorption with palm kernels. This behavior is explained as increasing temperature may subside the attraction between the binding sites of adsorbents and the dye particles existing in the adsorbed state.109
4. Adsorption isotherms
To study the adsorption ability and the behavior of adsorbates towards adsorbents, few isothermal studies are summarized in Table 3. These models help in determining the type of surface phase, i.e., mono layer or multilayer. One of the famous isotherm models is the Langmuir isothermal model (eqn (1)), which is based on the homogenous type of adsorption mechanism. It demonstrates that the single layer of adsorbate attached at a time to the adsorbent surface.86,182 Following eqn (1) is the mathematical expression for this model: |
 | (1) |
qe (mg g−1) is the adsorbate concentration at equilibrium, qmax (mg g−1) is the maximum adsorption capacity, and Ce (mg L−1) is the equilibrium concentration of adsorbate and KL (L mg−1) is the Langmuir constant.
Table 3 Illustration of linear forms of various isotherm models along with their application in water-soluble dye removal
Isotherm |
Equation (linear) |
Dye removed |
Ref. |
Henry |
qe = KHECe |
Acid yellow 194 |
186–188 |
Acid red 357 |
Acid black no. |
Malachite green |
Methylene blue |
Langmuir isotherm |
 |
Brilliant green |
114 and 189 |
Freundlich isotherm |
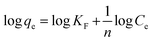 |
Fluorescein |
114 and 189 |
Eosin |
Dubinin–Radushkevich |
ln qe = qs − Kε2 |
Methyl orange |
190 and 191 |
Malachite green |
Temkin |
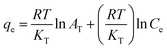 |
Methyl orange |
188, 190 and 192 |
Remazol brilliant blue R |
Methylene blue |
Flory–Huggins |
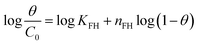 |
Remazol brilliant blue R |
188, 192 and 193 |
Malachite green |
Methylene blue |
Hills |
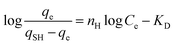 |
Methylene blue |
188 and 194 |
Crystal violet |
Halsey |
Log qe = (1/nH)ln KH − (1/nH)ln Ce |
Methylene blue |
195 and 196 |
Crystal violet |
Malachite green |
Jovanovich |
ln qe = ln qmax − KJCe |
Methylene blue |
188 and 197 |
Erichromr black T |
BET |
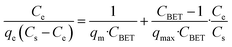 |
Basic blue 9 |
198 and 199 |
Acid blue 29 |
Acid red 91 |
Disperse red 1 |
Basic blue |
Usually, the cationic class of dyes demonstrates more adsorption ability than that of anionic class, and this refers to the presence of carboxylate moieties in most agri-wastes used as adsorbents. This functionality supports cationic dyes to be adsorbed, while it suppresses the anionic dye molecules to be adsorbed.159 Between the acid blue (anionic dye) and basic yellow (cationic dye) dyes adsorbed on coffee waste, the latter showed better adsorption behavior than that of the former.183 Some other isotherm models include Freundlich and Temkin isotherms, each having its own contribution towards the understanding of adsorption behaviors. The Freundlich model explains that the multilayer adsorption of adsorbates on the rough surface of adsorbents depicts non-ideal adsorption nature.184 The Temkin model explains the adsorption behavior and its link with sorption energy, which explains that the heat of adsorption decreases in a linear fashion with the increasing rate of adsorption. In other words, thermal diffusivity declines as more of the active binding sites got covered by adsorbate.185
Altenor et al.200 conducted a study on the removal of methylene blue by vetiver roots (modified physically with carbonization and chemical modification was done by H3PO4). Four isotherm studies, namely, Freundlich, Langmuir, Redlich–Peterson, and Brouers–Sotolongo, were carried. Out of all these, the results were found best aligned with the Brouers–Sotolongo and Redlich–Peterson models, which also found that chemically modified samples showed better adsorption ability towards MB than that of physically modified ones. It shows more alignment with the Langmuir and Brouers–Sotolongo models with adsorption capacities of 423 mg g−1 and 444 mg g−1, respectively. Table 4 presents the list of other research studies made accordingly.
Table 4 Summary of adsorption of dyes by various agricultural wastes
Adsorbent |
Dye/adsorbate |
pH |
Time (min) |
Temp (°C) |
Best fitting isotherm |
Kinetic modelling |
Maximum adsorption capacity (qmax) |
Ref. |
Bamboo dust carbon |
Methylene blue |
— |
35 |
30 |
Langmuir isotherm |
Pseudo first order |
143.20 |
72 |
Wheat shells |
Methylene blue |
5–9 |
60 |
30 |
Langmuir isotherm |
Pseudo second order |
21.50 |
168 |
Waste apricot-based activated carbon |
Malachite green |
— |
— |
50 |
Langmuir isotherm |
— |
163.9 |
195 |
Pineapple stem |
Methylene blue |
10 |
4 |
30 |
Langmuir isotherm |
Pseudo second order |
119.05 |
168 |
Rice husk |
Methylene blue |
8 |
40 |
30 |
Langmuir isotherm |
Pseudo second order |
40.50 |
71 |
Jujuba seeds |
Congo red |
2 |
— |
60 |
Langmuir isotherm |
Pseudo second order |
55.56 |
201 |
Cucumber peel |
Orange G |
2 |
250 |
— |
Langmuir isotherm |
Elovich model, pseudo second order model |
40.5 |
202 |
Wheat straw |
Orange II |
2 |
|
30 |
Langmuir isotherm |
Pseudo second order |
506 |
203 |
Palm kernel |
BTH4-(bromoaniline-azo-1,8-dihydronaphthalene-3,6-disodiumsulphate) |
— |
— |
23 |
Langmuir isotherm |
|
38.6 |
109 |
Polypyrrole/chitason composites (PPy/Ch) |
Acaticd orange-RL |
4 |
60 |
30 |
Langmuir, Freundlich isotherm |
Pseudo first order, pseudo second order |
76.9 |
204 |
Chitosan-aniline |
Crystal violet |
>7 |
60 |
50 |
Freundlich isotherm |
Pseudo second order |
100.6 |
205 |
CaO-biochar composite |
Phosphates from aqueous solution |
11 |
— |
44 (318 K) |
Langmuir isotherm |
Pseudo second order |
240 |
206 |
5. Thermodynamic studies
The heat changes and their effects on the overall adsorption process are classified in this section and summarized in Table 5. It presents the effect of temperature, resulting in evident changes in the kinetic energy of the molecules involved in the process. The elevated temperature usually increases the diffusion of dye molecules into the ligno-cellulosic arrangement of adsorbents. Parameters, such as ΔH0 (enthalpy), ΔS0 (entropy of the system), and ΔG0 (Gibb's free energy) (eqn (2)) present the heat changes responsible for the allocation of the exothermic (−ve ΔG0) or endothermic (+ve ΔG0) nature of reaction, which also describes the spontaneity of the process.
Table 5 Comparative data on the thermodynamics of dye (effluents) removal by agri-wastes
Adsorbent |
Adsorbate |
Temp. (K) |
ΔG0 (kJ mol−1) |
ΔH0 (kJ mol−1) |
ΔS0 (kJ mol−1 K−1) |
Reference |
Orange peel |
Methylene blue |
298 |
−7.65 |
14.8 |
0.07 |
207 |
308 |
−8.24 |
318 |
−9.16 |
Potato husk |
Crystal violet |
293 |
−20.36 |
−3.31 |
−0.009 |
208 |
303 |
−20.67 |
313 |
−21.75 |
Garlic peel |
DR12B |
298 |
−95.39 |
54.45 |
0.214 |
209 |
308 |
−111.2 |
318 |
−130.1 |
328 |
−160.9 |
Cucumber peel |
Methylene blue |
293 |
−4.51 |
−21.50 |
−0.059 |
210 |
303 |
−3.50 |
313 |
−3.29 |
323 |
−2.30 |
333 |
−2.21 |
Banana peel |
Methylene blue |
303 |
0.12 |
2.95 |
0.04 |
211 |
313 |
−0.36 |
323 |
−0.83 |
|
−1.31 |
Cactus fruit peel |
Brilliant green |
293 |
−3.26 |
−25.43 |
−0.07 |
212 |
303 |
−2.54 |
313 |
−1.75 |
Phosphoric acid activated lemon peel |
Methylene blue |
283 |
−23.85 |
−41.84 |
−63.55 |
117 |
293 |
−23.22 |
303 |
−22.58 |
313 |
−21.98 |
Activated carbon from kiwi peel |
Methylene blue |
298 |
−20.34 |
43.62 |
0.214 |
118 |
308 |
−22.49 |
318 |
−24.64 |
6. Kinetic studies
The refinement of adsorption process is reviewed by the kinetic studies of adsorption regarding rate constants.213 On the basis of adsorption capacity, the rate of adsorption plays a role in better selection of materials used as adsorbents. Generally, the pseudo-first-order and pseudo-second-order models are used for studying rate kinetics. The pseudo-first-order model generally does not go well with real-time data due to adsorption at constant time factor and diverges from the theoretical concept. Hence, the pseudo-second-order kinetic model is used, established on the adsorption ability of adsorbents (solid phase) and suggests the rate-determining step as the chemisorption process.214,215 Linear equations for pseudo-first-order (eqn (3)) and pseudo-second-order (eqn (4)) are presented as follows: |
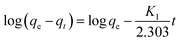 | (3) |
|
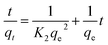 | (4) |
qt (mg g−1) is the adsorbate concentration adsorbed at time t, qe (mg g−1) is the adsorbate concentration adsorbed at equilibrium, K1 (1/min) is the rate constant of pseudo-first-order adsorption, K2 (g mg−1 min−1) is the rate constant of pseudo-second-order adsorption.
Generally, the value of R2 (regression correlation coefficient) assists in determining the best fitting kinetic model. The studies revealed that the pseudo-second-order model is more likely to be followed for the adsorption of anionic and cationic dyes mostly (Table 3). Extensive research has been made on the adsorption of methylene blue using various natural adsorbents where it was confirmed that they all have a value of R2 greater than that of the pseudo-second-order model, proving more likelihood for the pseudo-second-order kinetics.168,175,216
7. Mechanism of dye removal
The sorptive removal of dyes from contaminated water over a porous surface may adopt various adsorption mechanisms. The uptake of effluents (dyes) onto the adsorbent surface is driven by a number of interactions, including electrostatic interaction, van der Waals interaction, H-bonding, hydrophobic interaction, and π–π interactions, as shown in Fig. 6. The sorption of two anionic dyes, namely, congo red and methyl orange on GO–NiFe-LDH has been achieved by both ion exchange (exchange of ions between solid and liquid phases occur) and electrostatic interaction.217 Similarly, two mechanisms have been reported on the removal of methylene blue on base-treated wheat straw infused with Fe3O4 termed as ion exchange and surface composite. This surface composite is formed by the association developed between adsorbates and various functional groups present on the adsorbent surface and an electrostatic interface is developed between adsorbents and adsorbates.218 Rhodamine dye showed adsorption on coffee powder as an adsorbent, and this process was supported by electrostatic intermolecular attraction between adsorbent and adsorbate molecules.219 Cojocaru et al., reported that the mechanism followed by acid orange dye removal on the adsorption surface is H-bonding.220 A similar mechanism for the removal of methylene blue on the prepared adsorbent surface was proposed by Siddiqui et al.221 There are other mechanisms, including π–π interactions or pi-effects, which resembles electrostatic interaction, as positively charged entities interact with negatively charged entities. There are also some findings about the adoption of multiple types of mechanisms by the adsorption process, simultaneously. Thamer et al., reported the adsorption of Coomassie brilliant green R 250 dye by three distinct mechanisms followed, namely, pi-effect, H-bonding, and electrostatic attraction.222
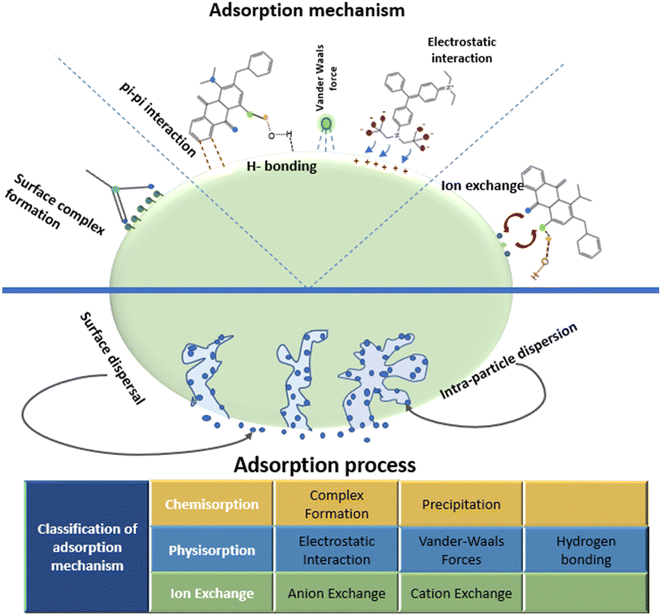 |
| Fig. 6 Brief representation of the classification of adsorption mechanisms adopted by the dye removal process.223 | |
8. Desorption study
To propose the mechanism adopted by the adsorbate adsorbed and also to regenerate it, desorption studies are carried out. The rate of desorption directly related to the primary instigator and the kinetics of desorption help in finding the pattern of effluent transport.224–226
Eluents or solvents used for desorption play a significant role in the separation of adsorbents and adsorbates, and the selection of eluents depends on the adsorbate–adsorbent system.10 Various criteria are checked for the selection of eluents for the desorption process, which include compatibility with the adsorbate and adsorbent, easy breakage of interaction with the adsorbate from the adsorbent, pH, and complex formability.227 Various different solvents or eluents, such as 0.05 N HNO3 (ref. 78), 2% HCl,96 0.1 M HCl23 (acidic eluents), 0.1 M NaOH,87 0.15 M NaOH75 (basic eluents), 4% NaOH + 2% NaCl,7 and 0.005 M thiourea in 1 M HCl56 are commonly used by different scientists.
Desorption is carried out by adding some appropriate solvents (eluents) into dye-rich solutions and shaken till the dye gets extracted into that solvent by filtration and evaporated at elevated temperatures. Then, the spectrophotometric determination of dye is made.228 Similar studies carried by the scientists are presented in Table 6.
Table 6 Previous studies on the desorption and regeneration of adsorbents
Adsorbent |
Dye name |
Dye class |
% age recovery |
References |
Deoiled soy |
Congo red |
Anionic |
90 |
229 |
Bottom ash |
Congo red |
Anionic |
80 |
Corynebacterium glutamicum |
Reactive black 5 |
Anionic |
80 |
230 |
Maize waste |
Basic blue |
Cationic |
0 |
231 |
Acid blue |
Anionic |
12 |
Reactive blue |
Reactive |
40 |
Pine cone |
Acid green 25 |
Anionic |
26.95 |
79 |
Acid black 26 |
Anionic |
93.16 |
Acid blue |
Anionic |
98 |
9. Conclusion
This study presents the recent work done by researchers on the adsorption of dyes using plant wastes, as there is a lot of work going on in this field and there is much scope for future work because of the leading issues we are facing, called water pollution. In this review, we have tried to simplify the evaluation of two classes of dyes, cationic and anionic, and their removal using agricultural by-products. Herein, it is concluded that the plant wastes coming out from agriculture industry can be utilized efficiently for the removal of dyes and cationic dyes, which showed more affinity towards natural forms of adsorbents than anionic dyes. A number of different modification techniques can be used to get their efficiency to be enhanced especially for the anionic type of dyes. The parameters responsible for better dye uptake by adsorbents include pH value (adsorption of cationic dyes favored at high pH values and anionic dyes prefer low pH values). Similarly, the adsorbent dose and dye concentration also affect the adsorption rate along with the temperature of the solution. The Langmuir and other isotherms were also examined to get the adsorption abilities of different adsorbents derived from agricultural wastes, and the results indicate that most of them showed more adsorption capacity towards cationic dyes than towards anionic ones. Moreover, kinetic studies presented here suggest the pseudo-second-order model to be followed more by both types of dyes. The adsorption characterization based on the morphology, surface area, and pore size using advance techniques present the adsorption ability of adsorbents. This review is mainly focused on plant source adsorbents along with their adsorption capacities. Hence, this review presented a comprehensive evaluation of plant wastes used in dye removal and their impacts on waste water treatment, which had shown great potential towards the green solutions to this problem.
Several studies consider adsorption as one of the most effect methods of dye removal from wastewater; however, there is always some room for improvement, so is here. These challenges are associated with the development of cost-effective, highly efficient, and stable adsorbents. Hence, agricultural wastes, non-toxic industrial by-products, and biodegradable nanomaterials can be considered as alternatives.
Moreover, the term reusability is broader in its concept, which assures the maximum restoration and stability of spent adsorbents to get them reutilized effectively. Moreover, the disposal of the used adsorbents in the development of biochar or other energy-producing products is another economic aspect of the project.
Data availability
All data are presented here along with references.
Conflicts of interest
We have no conflict of interest regarding publication of this paper.
Acknowledgements
Authors are thankful to the parent department for facilitating this work.
References
- M. Islam and M. Mostafa, Textile dyeing effluents and environment concerns-a review, Int. J. Environ. Sci Nat. Resour., 2018, 11(1–2), 131–144 Search PubMed.
- M. Ilyas, et al., Environmental and health impacts of industrial wastewater effluents in Pakistan: a review, Rev. Environ. Health, 2019, 34(2), 171–186 CrossRef CAS PubMed.
- R. N. Bharagava, et al., Characterization and identification of recalcitrant organic pollutants (ROPs) in tannery wastewater and its phytotoxicity evaluation for environmental safety, Arch. Environ. Contam. Toxicol., 2018, 75(2), 259–272 CrossRef CAS PubMed.
- R. Chandra, R. N. Bharagava and V. Rai, Melanoidins as major colourant in sugarcane molasses based distillery effluent and its degradation, Bioresour. Technol., 2008, 99(11), 4648–4660 CrossRef CAS PubMed.
- P. Chowdhary, A. Raj and R. N. Bharagava, Environmental pollution and health hazards from distillery wastewater and treatment approaches to combat the environmental threats: a review, Chemosphere, 2018, 194, 229–246 CrossRef CAS PubMed.
- S. Zainith, et al., Microbes an ecofriendly tools for the treatment of industrial waste waters, Microbes and Environmental Management, 2016, vol. 2016, pp. 75–100 Search PubMed.
- S. Mani and R. N. Bharagava, Textile industry wastewater: environmental and health hazards and treatment approaches, in Recent advances in environmental management, CRC Press, 2018, pp. 47–69 Search PubMed.
- G. Saxena, R. Chandra, and R. N. Bharagava, Environmental pollution, toxicity profile and treatment approaches for tannery wastewater and its chemical pollutants, Reviews of Environmental Contamination and Toxicology, vol. 240, 2016, pp. 31–69 Search PubMed.
- H. Zollinger, Azo dyes and pigments, Colour Chemistry-Synthesis, Properties and Applications of Organic Dyes and Pigments, 1987, pp. 92–100 Search PubMed.
- T. K. Sen, S. Afroze and H. Ang, Equilibrium, kinetics and mechanism of removal of methylene blue from aqueous solution by adsorption onto pine cone biomass of Pinus radiata, Water, Air, Soil Pollut., 2011, 218(1), 499–515 CrossRef CAS.
- S. Allen, G. Mckay and J. F. Porter, Adsorption isotherm models for basic dye adsorption by peat in single and binary component systems, J. Colloid Interface Sci., 2004, 280(2), 322–333 CrossRef CAS PubMed.
- R. N. Bharagava and S. Mishra, Hexavalent chromium reduction potential of Cellulosimicrobium sp. isolated from common effluent treatment plant of tannery industries, Ecotoxicol. Environ. Saf., 2018, 147, 102–109 CrossRef CAS PubMed.
- W. Liu, et al., Biodecolorization of azo, anthraquinonic and triphenylmethane dyes by white-rot fungi and a laccase-secreting engineered strain, J. Ind. Microbiol. Biotechnol., 2004, 31(3), 127–132 CrossRef CAS PubMed.
- A. Yadav, et al., Toxic metals in the environment: threats on ecosystem and bioremediation approaches, in Handbook of metal-microbe interactions and bioremediation, CRC Press, 2017, pp. 128–141 Search PubMed.
- D. C. Kalyani, et al., Biodegradation of reactive textile dye Red BLI by an isolated bacterium Pseudomonas sp. SUK1, Bioresour. Technol., 2008, 99(11), 4635–4641 CrossRef CAS PubMed.
- S. Mishra and R. N. Bharagava, Toxic and genotoxic effects of hexavalent chromium in environment and its bioremediation strategies, J. Environ. Sci. Health, Part C: Environ. Carcinog. Ecotoxicol. Rev., 2016, 34(1), 1–32 CrossRef CAS PubMed.
- M. K. Sharma and R. Sobti, Rec effect of certain textile dyes in Bacillus subtilis, Mutat. Res. Genet. Toxicol. Environ. Mutagen., 2000, 465(1–2), 27–38 CrossRef CAS PubMed.
- R. Malik, D. Ramteke and S. Wate, Adsorption of malachite green on groundnut shell waste based powdered activated carbon, Waste Manage., 2007, 27(9), 1129–1138 CrossRef CAS PubMed.
- K. Kadirvelu, et al., Utilization of various agricultural wastes for activated carbon preparation and application for the removal of dyes and metal ions from aqueous solutions, Bioresour. Technol., 2003, 87(1), 129–132 CrossRef CAS PubMed.
- A. R. Dinçer, et al., Comparison of activated carbon and bottom ash for removal of reactive dye from aqueous solution, Bioresour. Technol., 2007, 98(4), 834–839 CrossRef PubMed.
- D. Shen, et al., Adsorption kinetics and isotherm of anionic dyes onto organo-bentonite from single and multisolute systems, J. Hazard. Mater., 2009, 172(1), 99–107 CrossRef CAS PubMed.
- L. Young and J. Yu, Ligninase-catalysed decolorization of synthetic dyes, Water Res., 1997, 31(5), 1187–1193 CrossRef CAS.
- W. A. Al-Amrani, M. A. K. M. Hanafiah and A.-H. A. Mohammed, A comprehensive review of anionic azo dyes adsorption on surface-functionalised silicas, Environ. Sci. Pollut. Res., 2022, 29(51), 76565–76610 CrossRef CAS PubMed.
- M. M. Islam, et al., Assessment of environmental impacts for textile dyeing industries in Bangladesh, in International Conference on Green technology and environmental Conservation (GTEC-2011), IEEE, 2011 Search PubMed.
- D. M. Yacout and M. Hassouna, Identifying potential environmental impacts of waste handling strategies in textile industry, Environ. Monit. Assess., 2016, 188, 1–13 CrossRef CAS PubMed.
- T. A. Khattab, M. S. Abdelrahman and M. Rehan, Textile dyeing industry: environmental impacts and remediation, Environ. Sci. Pollut. Res., 2020, 27, 3803–3818 CrossRef CAS PubMed.
- J. M. Rosa, et al., Application of continuous H2O2/UV advanced oxidative process as an option to reduce the consumption of inputs, costs and environmental impacts of textile effluents, J. Cleaner Prod., 2020, 246, 119012 CrossRef CAS.
- S. Madhav, et al., A review of textile industry: Wet processing, environmental impacts, and effluent treatment methods, Environ. Qual. Manag., 2018, 27(3), 31–41 CrossRef.
- S. Madhav, et al., Water pollutants: sources and impact on the environment and human health, Sensors in Water Pollutants Monitoring, Role of Material, 2020, pp. 43–62 Search PubMed.
- J. M. Rosa, et al., Toxicity and environmental impacts approached in the dyeing of polyamide, polyester and cotton knits, J. Environ. Chem. Eng., 2019, 7(2), 102973 CrossRef CAS.
- R. Kant, Textile dyeing industry an environmental hazard. 2011 Search PubMed.
- A. Roy Choudhury, Environmental impacts of the textile industry and its assessment through life cycle assessment, Roadmap to sustainable textiles and clothing: environmental and social aspects of textiles and clothing supply chain, 2014, pp. 1–39 Search PubMed.
- T. Islam, et al., Impact of textile dyes on health and ecosystem: A review of structure, causes, and potential solutions, Environ. Sci. Pollut. Res., 2023, 30(4), 9207–9242 CrossRef CAS PubMed.
- P.-h. Chou, et al., Isolation and identification of xenobiotic aryl hydrocarbon receptor ligands in dyeing wastewater, Environ. Sci. Technol., 2007, 41(2), 652–657 CrossRef CAS PubMed.
- M. Tsuboy, et al., Genotoxic, mutagenic and cytotoxic effects of the commercial dye CI Disperse Blue 291 in the human hepatic cell line HepG2, Toxicol. In Vitro, 2007, 21(8), 1650–1655 CrossRef CAS PubMed.
- X. Wang, et al., Degradation of reactive brilliant red in aqueous solution by ultrasonic cavitation, Ultrason. Sonochem., 2008, 15(1), 43–48 CrossRef CAS PubMed.
- F. M. D. Chequer, et al., The azo dyes Disperse Red 1 and Disperse Orange 1 increase the micronuclei frequencies in human lymphocytes and in HepG2 cells, Mutat. Res. Genet. Toxicol. Environ. Mutagen., 2009, 676(1–2), 83–86 CrossRef CAS PubMed.
- H. Ben Mansour, et al., Acid violet 7 and its biodegradation products induce chromosome aberrations, lipid peroxidation, and cholinesterase inhibition in mouse bone marrow, Environ. Sci. Pollut. Res., 2010, 17, 1371–1378 CrossRef CAS PubMed.
- E. Ferraz, et al., Differential toxicity of Disperse Red 1 and Disperse Red 13 in the Ames test, HepG2 cytotoxicity assay, and Daphnia acute toxicity test, Environ. Toxicol., 2011, 26(5), 489–497 CrossRef CAS PubMed.
- A. Güngördü, A. Birhanli and M. Ozmen, Biochemical response to exposure to six textile dyes in early developmental stages of Xenopus laevis, Environ. Sci. Pollut. Res., 2013, 20, 452–460 CrossRef PubMed.
- S. Mekhilef, R. Saidur and M. Kamalisarvestani, Effect of dust, humidity and air velocity on efficiency of photovoltaic cells, Renewable Sustainable Energy Rev., 2012, 16(5), 2920–2925 CrossRef CAS.
- I. Ayadi, et al., Biochemical and histological changes in the liver and gills of Nile tilapia Oreochromis niloticus exposed to Red 195 dye, RSC Adv., 2015, 5(106), 87168–87178 RSC.
- M. Hernández-Zamora and F. Martínez-Jerónimo, Exposure to the azo dye Direct blue 15 produces toxic effects on microalgae, cladocerans, and zebrafish embryos, Ecotoxicology, 2019, 28(8), 890–902 CrossRef PubMed.
- F. H. Fernandes, G. de Aragão Umbuzeiro and D. M. F. Salvadori, Genotoxicity of textile dye CI Disperse Blue 291 in mouse bone marrow, Mutat. Res. Genet. Toxicol. Environ. Mutagen., 2019, 837, 48–51 CrossRef CAS PubMed.
- L. P. Wielewski, et al., Degradation of the textile dye reactive black 5 by basidiomycetes, Rev. Ambiente Agua, 2020, 15, e2464 Search PubMed.
- F. Vazquez-Ortega, I. Lagunes and A. Trigos, Cosmetic dyes
as potential photosensitizers of singlet oxygen generation, Dyes Pigm., 2020, 176, 108248 CrossRef CAS.
- E. Karpińska-Gasztoł, et al., Plethoric facies–multidisciplinary problem. Histamine intolerance secondary to reduced diamine oxydase activity, Adv. Med. Sci., 2014, 843–846 Search PubMed.
- K. Amin, H. A. Hameid II and A. Abd Elsttar, Effect of food azo dyes tartrazine and carmoisine on biochemical parameters related to renal, hepatic function and oxidative stress biomarkers in young male rats, Food Chem. Toxicol., 2010, 48(10), 2994–2999 CrossRef CAS PubMed.
- M.-S. Chiou, P.-Y. Ho and H.-Y. Li, Adsorption of anionic dyes in acid solutions using chemically cross-linked chitosan beads, Dyes Pigm., 2004, 60(1), 69–84 CrossRef CAS.
- M. Sulak, E. Demirbas and M. Kobya, Removal of Astrazon Yellow 7GL from aqueous solutions by adsorption onto wheat bran, Bioresour. Technol., 2007, 98(13), 2590–2598 CrossRef CAS PubMed.
- R. Qadeer, Adsorption behavior of ruthenium ions on activated charcoal from nirtic acid medium, Colloids Surf., A, 2007, 293(1–3), 217–223 CrossRef CAS.
- M. E. Argun, et al., Heavy metal adsorption by modified oak sawdust: Thermodynamics and kinetics, J. Hazard. Mater., 2007, 141(1), 77–85 CrossRef CAS PubMed.
- M. K. Aroua, et al., Real-time determination of kinetics of adsorption of lead (II) onto palm shell-based activated carbon using ion selective electrode, Bioresour. Technol., 2008, 99(13), 5786–5792 CrossRef CAS PubMed.
- N. H. Phan, et al., Production of fibrous activated carbons from natural cellulose (jute, coconut) fibers for water treatment applications, Carbon, 2006, 44(12), 2569–2577 CrossRef CAS.
- N. El Hannafi, et al., Elimination of phenol by adsorption on activated carbon prepared from the peach cores: modelling and optimisation, Desalination, 2008, 223(1–3), 264–268 CrossRef CAS.
- M. Ahmad, W. W. Daud and M. Aroua, Adsorption kinetics of various gases in carbon molecular sieves (CMS) produced from palm shell, Colloids Surf., A, 2008, 312(2–3), 131–135 CrossRef CAS.
- C.-H. Weng, Y.-T. Lin and T.-W. Tzeng, Removal of methylene blue from aqueous solution by adsorption onto pineapple leaf powder, J. Hazard. Mater., 2009, 170(1), 417–424 CrossRef CAS PubMed.
- M. El-Halwany, Study of adsorption isotherms and kinetic models for Methylene Blue adsorption on activated carbon developed from Egyptian rice hull (Part II), Desalination, 2010, 250(1), 208–213 CrossRef CAS.
- M. Arulkumar, P. Sathishkumar and T. Palvannan, Optimization of Orange G dye adsorption by activated carbon of Thespesia populnea pods using response surface methodology, J. Hazard. Mater., 2011, 186(1), 827–834 CrossRef CAS PubMed.
- K. Sharma, et al., A comparative study on characterization of textile wastewaters (untreated and treated) toxicity by chemical and biological tests, Chemosphere, 2007, 69(1), 48–54 CrossRef CAS PubMed.
- S. Şen and G. Demirer, Anaerobic treatment of real textile wastewater with a fluidized bed reactor, Water Res., 2003, 37(8), 1868–1878 CrossRef PubMed.
- T. Robinson, et al., Remediation of dyes in textile effluent: a critical review on current treatment technologies with a proposed alternative, Bioresour. Technol., 2001, 77(3), 247–255 CrossRef CAS PubMed.
- V. Gupta, Application of low-cost adsorbents for dye removal–a review, J. Environ. Manage., 2009, 90(8), 2313–2342 CrossRef CAS PubMed.
- A. Demirbas, Agricultural based activated carbons for the removal of dyes from aqueous solutions: a review, J. Hazard. Mater., 2009, 167(1–3), 1–9 CrossRef CAS PubMed.
- E. Forgacs, T. Cserháti and G. Oros, Removal of synthetic dyes from wastewaters: a review, Environ. Int., 2004, 30(7), 953–971 CrossRef CAS PubMed.
- G. Mishra and M. Tripathy, A critical review of the treatments for decolourization of textile effluent, Colourage, 1993, 40, 35 CAS.
- O. Tyagi, M. Yadav, and M. Yadav, A Textbook of Synthetic Dyes, Anmol-PVT Ltd, 2002, 67 Search PubMed.
- K. Hunger, Dyes, general survey. Industrial Dyes: Chemistry, Properties, Applications, Wiley Subscription Services, Inc., A Wiley Company, Frankfurt, 2003, pp. 1–10 Search PubMed.
- E. Eren and B. Afsin, Investigation of a basic dye adsorption from aqueous solution onto raw and pre-treated sepiolite surfaces, Dyes Pigm., 2007, 73(2), 162–167 CrossRef CAS.
- E. Eren, Investigation of a basic dye removal from aqueous solution onto chemically modified Unye bentonite, J. Hazard. Mater., 2009, 166(1), 88–93 CrossRef CAS PubMed.
- V. Vadivelan and K. V. Kumar, Equilibrium, kinetics, mechanism, and process design for the sorption of methylene blue onto rice husk, J. Colloid Interface Sci., 2005, 286(1), 90–100 CrossRef CAS PubMed.
- N. Kannan and M. M. Sundaram, Kinetics and mechanism of removal of methylene blue by adsorption on various carbons—a comparative study, Dyes Pigm., 2001, 51(1), 25–40 CrossRef CAS.
- V. Ponnusami, S. Vikram and S. Srivastava, Guava (Psidium guajava) leaf powder: novel adsorbent for removal of methylene blue from aqueous solutions, J. Hazard. Mater., 2008, 152(1), 276–286 CrossRef CAS PubMed.
- D. Özer, G. Dursun and A. Özer, Methylene blue adsorption from aqueous solution by dehydrated peanut hull, J. Hazard. Mater., 2007, 144(1–2), 171–179 CrossRef PubMed.
- A. Tabak, et al., Determination of adsorptive properties of a Turkish Sepiolite for removal of Reactive Blue 15 anionic dye from aqueous solutions, J. Hazard. Mater., 2009, 161(2–3), 1087–1094 CrossRef CAS PubMed.
- A. A. Attia, W. E. Rashwan and S. A. Khedr, Capacity of activated carbon in the removal of acid dyes subsequent to its thermal treatment, Dyes Pigm., 2006, 69(3), 128–136 CrossRef CAS.
- H. El Boujaady, et al., Removal of a textile dye by adsorption on synthetic calcium phosphates, Desalination, 2011, 275(1–3), 10–16 CrossRef CAS.
- D. Bingol, N. Tekin and M. Alkan, Brilliant Yellow dye adsorption onto sepiolite using a full factorial design, Appl. Clay Sci., 2010, 50(3), 315–321 CrossRef CAS.
- N. M. Mahmoodi, et al., Adsorption of textile dyes on pine cone from colored wastewater: kinetic, equilibrium and thermodynamic studies, Desalination, 2011, 268(1–3), 117–125 CrossRef CAS.
- M. Valix, W. Cheung and G. McKay, Preparation of activated carbon using low temperature carbonisation and physical activation of high ash raw bagasse for acid dye adsorption, Chemosphere, 2004, 56(5), 493–501 CrossRef CAS PubMed.
- M. M. Dávila-Jiménez, M. P. Elizalde-González and V. Hernández-Montoya, Performance of mango seed adsorbents in the adsorption of anthraquinone and azo acid dyes in single and binary aqueous solutions, Bioresour. Technol., 2009, 100(24), 6199–6206 CrossRef PubMed.
- M. Arami, et al., Equilibrium and kinetics studies for the adsorption of direct and acid dyes from aqueous solution by soy meal hull, J. Hazard. Mater., 2006, 135(1–3), 171–179 CrossRef CAS PubMed.
- E. L. Mui, et al., Dye adsorption onto char from bamboo, J. Hazard. Mater., 2010, 177(1–3), 1001–1005 CrossRef CAS PubMed.
- K. Xie, W. Zhao and X. He, Adsorption properties of nano-cellulose hybrid containing polyhedral oligomeric silsesquioxane and removal of reactive dyes from aqueous solution, Carbohydr. Polym., 2011, 83(4), 1516–1520 CrossRef CAS.
- T. G. Chuah, et al., Rice husk as a potentially low-cost biosorbent for heavy metal and dye removal: an overview, Desalination, 2005, 175(3), 305–316 CrossRef CAS.
- A. Dąbrowski, Adsorption—from theory to practice, Adv. Colloid Interface Sci., 2001, 93(1–3), 135–224 CrossRef PubMed.
- K. Noll, V. Gounaris, and W. Hou, Adsorption Technology for Air and Water Pollution ControlII Lewis, Chelsea, MI, 1992, pp. 21–22 Search PubMed.
- B. Noroozi, et al., Equilibrium and kinetic adsorption study of a cationic dye by a natural adsorbent—Silkworm pupa, J. Hazard. Mater., 2007, 139(1), 167–174 CrossRef CAS PubMed.
- J. S. Mattson and H. B. Mark, Activated carbon: surface chemistry and adsorption from solution, M. Dekker, 1971 Search PubMed.
- M. Corapcioglu and C. Huang, The surface acidity and characterization of some commercial activated carbons, Carbon, 1987, 25(4), 569–578 CrossRef CAS.
- I. N. Najm, et al., Using powdered activated carbon: a critical review, J. - Am. Water Works Assoc., 1991, 83(1), 65–76 CrossRef CAS.
- M. O. Abdullah, I. A. W. Tan and L. S. Lim, Automobile adsorption
air-conditioning system using oil palm biomass-based activated carbon: A review, Renewable Sustainable Energy Rev., 2011, 15(4), 2061–2072 CrossRef CAS.
- V. C. Srivastava, I. D. Mall and I. M. Mishra, Adsorption thermodynamics and isosteric heat of adsorption of toxic metal ions onto bagasse fly ash (BFA) and rice husk ash (RHA), Chem. Eng. J., 2007, 132(1–3), 267–278 CrossRef CAS.
- M. A. Nazir, et al., Surface induced growth of ZIF-67 at Co-layered double hydroxide: Removal of methylene blue and methyl orange from water, Appl. Clay Sci., 2020, 190, 105564 CrossRef CAS.
- C. Arora, et al., Iron based metal organic framework for efficient removal of methylene blue dye from industrial waste, J. Mol. Liq., 2019, 284, 343–352 CrossRef CAS.
- Z. Cheng, et al., One-step fabrication of graphene oxide enhanced magnetic composite gel for highly efficient dye adsorption and catalysis, ACS Sustain. Chem. Eng., 2015, 3(7), 1677–1685 CrossRef CAS.
- X. Zhao, et al., Co-Mn layered double hydroxide as an effective heterogeneous catalyst for degradation of organic dyes by activation of peroxymonosulfate, Chemosphere, 2018, 204, 11–21 CrossRef CAS PubMed.
- H. Zeng, et al., Degradation of dyes by peroxymonosulfate activated by ternary CoFeNi-layered double hydroxide: Catalytic performance, mechanism and kinetic modeling, J. Colloid Interface Sci., 2018, 515, 92–100 CrossRef CAS PubMed.
- P. González-García, Activated carbon from lignocellulosics precursors: A review of the synthesis methods, characterization techniques and applications, Renewable Sustainable Energy Rev., 2018, 82, 1393–1414 CrossRef.
- M. A. Nazir, et al., Heterointerface engineering of water stable ZIF-8@ ZIF-67: Adsorption of rhodamine B from water, Surf. Interfaces, 2022, 34, 102324 CrossRef CAS.
- C. Santhosh, et al., Role of nanomaterials in water treatment applications: a review, Chem. Eng. J., 2016, 306, 1116–1137 CrossRef CAS.
- G. Z. Kyzas and K. A. Matis, Nanoadsorbents for pollutants removal: a review, J. Mol. Liq., 2015, 203, 159–168 CrossRef CAS.
- P. Wang, et al., Kinetics and thermodynamics of adsorption of methylene blue by a magnetic graphene-carbon nanotube composite, Appl. Surf. Sci., 2014, 290, 116–124 CrossRef CAS.
- J. Cheng, et al., Characterization of magnetic carbon nanotube–cyclodextrin composite and its adsorption of dye, Ind. Eng. Chem. Res., 2014, 53(4), 1415–1421 CrossRef CAS.
- M. Rafatullah, et al., Adsorption of methylene blue on low-cost adsorbents: a review, J. Hazard. Mater., 2010, 177(1–3), 70–80 CrossRef CAS PubMed.
- M. Mannan and C. Ganapathy, Concrete from an agricultural waste-oil palm shell (OPS), Build. Sci., 2004, 39(4), 441–448 Search PubMed.
- A. S. Franca, L. S. Oliveira and M. E. Ferreira, Kinetics and equilibrium studies of methylene blue adsorption by spent coffee grounds, Desalination, 2009, 249(1), 267–272 CrossRef CAS.
- J. de Souza Macedo, et al., Kinetic and calorimetric study of the adsorption of dyes on mesoporous activated carbon prepared from coconut coir dust, J. Colloid Interface Sci., 2006, 298(2), 515–522 CrossRef PubMed.
- A. E. Ofomaja and Y.-S. Ho, Equilibrium sorption of anionic dye from aqueous solution by palm kernel fibre as sorbent, Dyes Pigm., 2007, 74(1), 60–66 CrossRef CAS.
- A. E. Ofomaja, Sorption dynamics and isotherm studies of methylene blue uptake on to palm kernel fibre, Chem. Eng. J., 2007, 126(1), 35–43 CrossRef CAS.
- T. S. Choong, et al., Film-pore-concentration-dependent surface diffusion model for the adsorption of dye onto palm kernel shell activated carbon, J. Colloid Interface Sci., 2006, 301(2), 436–440 CrossRef CAS PubMed.
- R. Jain and M. Shrivastava, Adsorptive studies of hazardous dye Tropaeoline 000 from an aqueous phase on to coconut-husk, J. Hazard. Mater., 2008, 158(2–3), 549–556 CrossRef CAS PubMed.
- K. Kadirvelu, et al., Activated carbon from an agricultural by-product, for the treatment of dyeing industry wastewater, Bioresour. Technol., 2000, 74(3), 263–265 CrossRef CAS.
- S. Latif, et al., Removal of acidic dyes from aqueous media using Citrullus lanatus peels: an agrowaste-based adsorbent for environmental safety, J. Chem., 2019, 2019, 6704953 Search PubMed.
- R. Rehman, T. Mahmud and M. Irum, Brilliant green dye elimination from water using Psidium guajava leaves and Solanum tuberosum peels as adsorbents in environmentally benign way, J. Chem., 2015, 2015, 126036 Search PubMed.
- N. Oladoja, et al., Studies on castor seed shell as a sorbent in basic dye
contaminated wastewater remediation, Desalination, 2008, 227(1–3), 190–203 CrossRef CAS.
- A. Aichour, et al., Bioadsorbent beads prepared from activated biomass/alginate for enhanced removal of cationic dye from water medium: Kinetics, equilibrium and thermodynamic studies, J. Mol. Liq., 2018, 256, 533–540 CrossRef CAS.
- N. M. Mahmoodi, M. Taghizadeh and A. Taghizadeh, Mesoporous activated carbons of low-cost agricultural bio-wastes with high adsorption capacity: preparation and artificial neural network modeling of dye removal from single and multicomponent (binary and ternary) systems, J. Mol. Liq., 2018, 269, 217–228 CrossRef CAS.
- S. Yakout, Effect of porosity and surface chemistry on the adsorption-desorption of uranium (VI) from aqueous solution and groundwater, J. Radioanal. Nucl. Chem., 2016, 308(2), 555–565 CrossRef CAS.
- S. Bose, S. F. Robertson and A. Bandyopadhyay, Surface modification of biomaterials and biomedical devices using additive manufacturing, Acta Biomater., 2018, 66, 6–22 CrossRef CAS PubMed.
- S. M. Abegunde, et al., A review on the influence of chemical modification on the performance of adsorbents, Resour. Environ. Sustain., 2020, 1, 100001 Search PubMed.
- I. Tole, K. Habermehl-Cwirzen and A. Cwirzen, Mechanochemical activation of natural clay minerals: an alternative to produce sustainable cementitious binders–review, Mineral. Petrol., 2019, 113(4), 449–462 CrossRef CAS.
- R. Manory, Some principles for understanding surface modification of metals by glow discharge processes, Mater. Manuf. Processes, 1990, 5(3), 445–470 CrossRef CAS.
- E. Rostami, R. Norouzbeigi and A. Rahbar, Thermal and chemical modification of bentonite for adsorption of an anionic dye, Adv. Environ. Sci. Technol., 2018, 4(1), 1–12 Search PubMed.
- S. Mishra, Adsorption–desorption of heavy metal ions, Curr. Sci., 2014, 601–612 CAS.
- A. Rehman, M. Park and S.-J. Park, Current progress on the surface chemical modification of carbonaceous materials, Coatings, 2019, 9(2), 103 CrossRef.
- G. Enaime, Biochar for wastewater treatment conversion technologies and applications, Appl. Sci., 2020, 10, 3492 CrossRef CAS.
- B. Huang, et al., Effect of nitric acid modification on characteristics and adsorption properties of lignite, Processes, 2019, 7(3), 167 CrossRef CAS.
- P. M. Godwin, et al., Progress in preparation and application of modified biochar for improving heavy metal ion removal from wastewater, J. Bioresour. Bioprod., 2019, 4(1), 31–42 CrossRef.
- G. Hisarli, The effects of acid and alkali modification on the adsorption performance of fuller's earth for basic dye, J. Colloid Interface Sci., 2005, 281(1), 18–26 CrossRef CAS PubMed.
- C. Zheng, et al., Treatment technologies for organic wastewater, Water Treat., 2013, 11, 250–286 Search PubMed.
- S. Sonal, et al., Synthesis, characterization and sorption studies of a zirconium (iv) impregnated highly functionalized mesoporous activated carbons, RSC Adv., 2020, 10(23), 13783–13798 RSC.
- H. Wang, et al., Removal of Pb (II), Cu (II), and Cd (II) from aqueous solutions by biochar derived from KMnO4 treated hickory wood, Bioresour. Technol., 2015, 197, 356–362 CrossRef CAS PubMed.
- P. M. Stähelin, et al., Benzene and toluene removal from synthetic automotive gasoline by mono and bicomponent adsorption process, Fuel, 2018, 231, 45–52 CrossRef.
- K. Yang, et al., Preparation of high surface area activated carbon from coconut shells using microwave heating, Bioresour. Technol., 2010, 101(15), 6163–6169 CrossRef CAS PubMed.
- Z. Hu and M. Srinivasan, Mesoporous high-surface-area activated carbon, Microporous Mesoporous Mater., 2001, 43(3), 267–275 CrossRef CAS.
- M. L. N. Cerutti, et al., Copper-exchanged Y zeolites for gasoline deep-desulfurization, Adsorption, 2019, 25, 1595–1609 CrossRef CAS.
- E. López-Maya, et al., Textile/Metal–Organic-Framework Composites as Self-Detoxifying Filters for Chemical-Warfare Agents, Angew. Chem., Int. Ed., 2015, 54(23), 6790–6794 CrossRef PubMed.
- Q. Cao, et al., Process effects on activated carbon with large specific surface area from corn cob, Bioresour. Technol., 2006, 97(1), 110–115 CrossRef CAS PubMed.
- R. C. Bansal and M. Goyal, Activated carbon adsorption, CRC press, 2005 Search PubMed.
- F. H. Norris, et al., Community resilience as a metaphor, theory, set of capacities, and strategy for disaster readiness, Am. J. Community Psychol., 2008, 41, 127–150 CrossRef PubMed.
- S. Blasioli, et al., Removal of sulfamethoxazole sulfonamide antibiotic from water by high silica zeolites: A study of the involved host–guest interactions by a combined structural, spectroscopic, and computational approach, J. Colloid Interface Sci., 2014, 419, 148–159 CrossRef CAS PubMed.
- L. Pellenz, et al., A comprehensive guide for characterization of adsorbent materials, Sep. Purif. Technol., 2022, 122435 Search PubMed.
- P. L. Yap, et al., Graphene-based sorbents for multipollutants removal in water: a review of recent progress, Adv. Funct. Mater., 2021, 31(9), 2007356 CrossRef CAS.
- C. P. Bergmann and F. M. Machado, Carbon nanomaterials as adsorbents for environmental and biological applications, Springer, 2015 Search PubMed.
- S. Kotz, N. Balakrishnan, C.B. Read and B. Vidakovic, Encyclopedia of Statistical Sciences, John Wiley & Sons, 2005, vol. 1 Search PubMed.
- A. Mahmad, et al., Experimental and molecular modelling approach for rapid adsorption of Bisphenol A using Zr and Fe based metal–organic frameworks, Inorg. Chem. Commun., 2022, 142, 109604 CrossRef CAS.
- M. Dias, et al., Adsorption of natural annatto dye by kaolin: kinetic and equilibrium, Environ. Technol., 2020, 41(20), 2648–2656 CrossRef CAS PubMed.
- J. Correia, et al., Adsorbents made from textile scraps: preparation, characterization and application for removal of reactive dye, Clean Technol. Environ. Policy, 2018, 20, 839–853 CrossRef CAS.
- C. R. S. de Oliveira, et al., Synthesis of superacid sulfated TiO2 prepared by sol-gel method and its use as a titania precursor in obtaining a kaolinite/TiO2 nano-hybrid composite, Powder Technol., 2021, 381, 366–380 CrossRef CAS.
- M. Y. Rather and S. Sundarapandian, Facile green synthesis of copper oxide nanoparticles and their rhodamine-b dye adsorption property, J. Cluster Sci., 2022, 1–9 Search PubMed.
- Q. Zhang, et al., An efficient SO2-adsorbent from calcination of natural magnesite, Ceram. Int., 2017, 43(15), 12557–12562 CrossRef CAS.
- C. Yang, et al., High removal rate and selectivity of Hg (II) ions using the magnetic composite adsorbent based on starch/polyethyleneimine, J. Mol. Liq., 2021, 337, 116418 CrossRef CAS.
- M. Tsodikov, et al., Formation of adsorbents from Fe-containing processing residues of lignin, Microporous Mesoporous Mater., 2020, 298, 110089 CrossRef CAS.
- Y. Önal, et al., Adsorption kinetics of malachite green onto activated carbon prepared from Tunçbilek lignite, J. Hazard. Mater., 2006, 128(2–3), 150–157 CrossRef PubMed.
- A. Özcan, et al., Modification of bentonite with a cationic surfactant: an adsorption study of textile dye Reactive Blue 19, J. Hazard. Mater., 2007, 140(1–2), 173–179 CrossRef PubMed.
- J. F. Osma, et al., Sunflower seed shells: a novel and effective low-cost adsorbent for the removal of the diazo dye Reactive Black 5 from aqueous solutions, J. Hazard. Mater., 2007, 147(3), 900–905 CrossRef CAS PubMed.
- P. K. Malik, Use of activated carbons prepared from sawdust and rice-husk for adsorption of acid dyes: a case study of Acid Yellow 36, Dyes Pigm., 2003, 56(3), 239–249 CrossRef CAS.
- R. Gong, et al., Utilization of powdered peanut hull as biosorbent for removal of anionic dyes from aqueous solution, Dyes Pigm., 2005, 64(3), 187–192 CrossRef CAS.
- C. Namasivayam, et al., ‘Waste’coir pith—a potential biomass for the treatment of dyeing wastewaters, Biomass Bioenergy, 2001, 21(6), 477–483 CrossRef CAS.
- Z. Aksu and I. A. Isoglu, Use of agricultural waste sugar beet pulp for the removal of Gemazol turquoise blue-G reactive dye from aqueous solution, J. Hazard. Mater., 2006, 137(1), 418–430 CrossRef CAS PubMed.
- B. Hameed, D. Mahmoud and A. Ahmad, Sorption equilibrium and kinetics of basic dye from aqueous solution using banana stalk waste, J. Hazard. Mater., 2008, 158(2–3), 499–506 CrossRef CAS PubMed.
- A. Poghossian, Determination of the pHpzc of insulators surface from capacitance–voltage characteristics of MIS and EIS structures, Sens. Actuators, B, 1997, 44(1–3), 551–553 CrossRef CAS.
- L. Radovic, et al., An experimental and theoretical study of the adsorption of aromatics possessing electron-withdrawing and electron-donating functional groups by chemically modified activated carbons, Carbon, 1997, 35(9), 1339–1348 CrossRef CAS.
- D. Savova, et al., The influence of the texture and surface properties of carbon adsorbents obtained from biomass products
on the adsorption of manganese ions from aqueous solution, Carbon, 2003, 41(10), 1897–1903 CrossRef CAS.
- B. Hameed, I. Tan and A. Ahmad, Adsorption isotherm, kinetic modeling and mechanism of 2, 4, 6-trichlorophenol on coconut husk-based activated carbon, Chem. Eng. J., 2008, 144(2), 235–244 CrossRef CAS.
- Z. Eren and F. N. Acar, Adsorption of Reactive Black 5 from an aqueous solution: equilibrium and kinetic studies, Desalination, 2006, 194(1–3), 1–10 CrossRef CAS.
- Y. Bulut and H. Aydın, A kinetics and thermodynamics study of methylene blue adsorption on wheat shells, Desalination, 2006, 194(1–3), 259–267 CrossRef CAS.
- V. K. Garg, et al., Basic dye (methylene blue) removal from simulated wastewater by adsorption using Indian Rosewood sawdust: a timber industry waste, Dyes Pigm., 2004, 63(3), 243–250 CrossRef CAS.
- Q. Li, et al., Equilibrium, thermodynamics and process design to minimize adsorbent amount for the adsorption of acid dyes onto cationic polymer-loaded bentonite, Chem. Eng. J., 2010, 158(3), 489–497 CrossRef CAS.
- M. Özacar and I. A. Şengýl, Two-stage batch sorber design using second-order kinetic model for the sorption of metal complex dyes onto pine sawdust, Biochem. Eng. J., 2004, 21(1), 39–45 CrossRef.
- A. E. Ofomaja, Sorptive removal of Methylene blue from aqueous solution using palm kernel fibre: Effect of fibre dose, Biochem. Eng. J., 2008, 40(1), 8–18 CrossRef CAS.
- V. S. Mane, I. D. Mall and V. C. Srivastava, Kinetic and equilibrium isotherm studies for the adsorptive removal of Brilliant Green dye from aqueous solution by rice husk ash, J. Environ. Manage., 2007, 84(4), 390–400 CrossRef CAS PubMed.
- K. Porkodi and K. V. Kumar, Equilibrium, kinetics and mechanism modeling and simulation of basic and acid dyes sorption onto jute fiber carbon: Eosin yellow, malachite green and crystal violet single component systems, J. Hazard. Mater., 2007, 143(1–2), 311–327 CrossRef CAS PubMed.
- U. R. Lakshmi, et al., Rice husk ash as an effective adsorbent: Evaluation of adsorptive characteristics for Indigo Carmine dye, J. Environ. Manage., 2009, 90(2), 710–720 CrossRef CAS PubMed.
- S. Senthilkumaar, P. Kalaamani and C. Subburaam, Liquid phase adsorption of crystal violet onto activated carbons derived from male flowers of coconut tree, J. Hazard. Mater., 2006, 136(3), 800–808 CrossRef CAS PubMed.
- B. Nandi, A. Goswami and M. Purkait, Removal of cationic dyes from aqueous solutions by kaolin: kinetic and equilibrium studies, Appl. Clay Sci., 2009, 42(3–4), 583–590 CrossRef CAS.
- G. Mckay, M. El Geundi and M. Nassar, External mass transport processes during the adsorption of dyes onto bagasse pith, Water Res., 1988, 22(12), 1527–1533 CrossRef CAS.
- M. Sureshkumar and C. Namasivayam, Adsorption behavior of Direct Red 12B and Rhodamine B from water onto surfactant-modified coconut coir pith, Colloids Surf., A, 2008, 317(1–3), 277–283 CrossRef CAS.
- Y. Önal, Kinetics of adsorption of dyes from aqueous solution using activated carbon prepared from waste apricot, J. Hazard. Mater., 2006, 137(3), 1719–1728 CrossRef PubMed.
- B. Hameed and A. Ahmad, Batch adsorption of methylene blue from aqueous solution by garlic peel, an agricultural waste biomass, J. Hazard. Mater., 2009, 164(2–3), 870–875 CrossRef CAS PubMed.
- W. Rudzinski and W. Plazinski, Theoretical description of the kinetics of solute adsorption at heterogeneous solid/solution interfaces: On the possibility of distinguishing between the diffusional and the surface reaction kinetics models, Appl. Surf. Sci., 2007, 253(13), 5827–5840 CrossRef CAS.
- A. Namane, et al., Determination of the adsorption capacity of activated carbon made from coffee grounds by chemical activation with ZnCl2 and H3PO4, J. Hazard. Mater., 2005, 119(1–3), 189–194 CrossRef CAS PubMed.
- I. Langmuir, The adsorption of gases on plane surfaces of glass, mica and platinum, J. Am. Chem. Soc., 1918, 40(9), 1361–1403 CrossRef CAS.
- S. Basharat, et al., Tartaric acid-modified Holarrhena antidysenterica and Citrullus colocynthis biowaste for efficient eradication of crystal violet dye from water, J. Chem., 2020, 2020, 8862167 Search PubMed.
- J. Piccin, et al., Kinetics and isotherms of leather dye adsorption by tannery solid waste, Chem. Eng. J., 2012, 183, 30–38 CrossRef CAS.
- A. Zhul-quarnain, et al., Adsorption of malachite green dye using orange peel, Int. J. Biomater., 2018, 2(2), 31–40 Search PubMed.
- F. Nworie, et al., Removal of methylene blue from aqueous solution using activated rice husk biochar: Adsorption isotherms, kinetics and error analysis, J. Chil. Chem. Soc., 2019, 64(1), 4365–4376 CrossRef CAS.
- R. Rehman, B. Salariya and L. Mitu, Batch scale adsorptive removal of brilliant green dye using Trapa natans peels in cost effective manner, Rev. Chim., 2016, 67, 1333–1337 CAS.
- N. Elhadiri, M. Benchanaa and R. Chikri, Activated carbon for dyes removal: modeling and understanding the adsorption process, J. Chem., 2020, 2020, 2096834 Search PubMed.
- A. Altınışık, E. Gür and Y. Seki, A natural sorbent, Luffa cylindrica for the removal of a model basic dye, J. Hazard. Mater., 2010, 179(1–3), 658–664 CrossRef PubMed.
- A. Ergene, et al., Removal of Remazol Brilliant Blue R dye from aqueous solutions by adsorption onto immobilized Scenedesmus quadricauda: Equilibrium and kinetic modeling studies, Desalination, 2009, 249(3), 1308–1314 CrossRef CAS.
- U. Etim, et al., Dye removal from aqueous solution using coconut coir dust extract-modified polyvinyl alcohol: a novel adsorbent, Int. J. Environ. Bioenergy, 2013, 5(2), 62–79 Search PubMed.
- A. Wathukarage, et al., Mechanistic understanding of crystal violet dye sorption by woody biochar: implications for wastewater treatment, Environ. Geochem. Health, 2019, 41(4), 1647–1661 CrossRef CAS PubMed.
- C. A. Başar, Applicability of the various adsorption models of three dyes adsorption onto activated carbon prepared waste apricot, J. Hazard. Mater., 2006, 135(1–3), 232–241 CrossRef PubMed.
- T. Chakraborty, et al., Jute (Corchorus olitorius) stick charcoal as a low-cost adsorbent for the removal of methylene blue dye from aqueous solution, SN Appl. Sci., 2020, 2(4), 1–10 Search PubMed.
- M. A. Aly-Eldeen, et al., The uptake of Eriochrome Black T dye from aqueous solutions utilizing waste activated sludge: Adsorption process optimization using factorial design, Egypt. J. Aquat. Res., 2018, 44(3), 179–186 CrossRef.
- K. R. Ramakrishna and T. Viraraghavan, Dye removal using low cost adsorbents, Water Sci. Technol., 1997, 36(2–3), 189–196 CrossRef CAS.
- Y. Fu and T. Viraraghavan, Removal of a dye from an aqueous solution by the fungus Aspergillus niger, Water Qual. Res. J., 2000, 35(1), 95–112 CrossRef CAS.
- S. Altenor, et al., Adsorption studies of methylene blue and phenol onto vetiver roots activated carbon prepared by chemical activation, J. Hazard. Mater., 2009, 165(1–3), 1029–1039 CrossRef CAS PubMed.
- M. S. Reddy, L. Sivaramakrishna and A. V. Reddy, The use of an agricultural waste material, Jujuba seeds for the removal of anionic dye (Congo red) from aqueous medium, J. Hazard. Mater., 2012, 203, 118–127 CrossRef PubMed.
- A. Stavrinou, C. Aggelopoulos and C. Tsakiroglou, Exploring the adsorption mechanisms of cationic and anionic dyes onto agricultural waste peels of banana, cucumber and potato: Adsorption kinetics and equilibrium isotherms as a tool, J. Environ. Chem. Eng., 2018, 6(6), 6958–6970 CrossRef CAS.
- Q. Lin, et al., Effectively removal of cationic and anionic dyes by pH-sensitive amphoteric adsorbent derived from agricultural waste-wheat straw, J. Taiwan Inst. Chem. Eng., 2017, 76, 65–72 CrossRef CAS.
- S. Noreen, et al., Removal of actacid orange-RL dye using biocomposites: modeling studies, Pol. J. Environ. Stud., 2017, 26(5), 2125–2134 CrossRef CAS PubMed.
- N. Tahir, et al., Biopolymers composites with peanut hull waste biomass and application for Crystal Violet adsorption, Int. J. Biol. Macromol., 2017, 94, 210–220 CrossRef CAS PubMed.
- X. Liu, F. Shen and X. Qi, Adsorption recovery of phosphate from aqueous solution by CaO-biochar composites prepared from eggshell and rice straw, Sci. Total Environ., 2019, 666, 694–702 CrossRef CAS PubMed.
- M. Boumediene, et al., Characterization of two cellulosic waste materials (orange and almond peels) and their use for the removal of methylene blue from aqueous solutions, Maderas: Cienc. Tecnol., 2015, 17(1), 69–84 CAS.
- M. Mafra, et al., Adsorption of remazol brilliant blue on an orange peel adsorbent, Braz. J. Chem. Eng., 2013, 30, 657–665 CrossRef CAS.
- A. Asfaram, et al., Removal of Direct Red 12B by garlic peel as a cheap adsorbent: kinetics, thermodynamic and equilibrium isotherms study of removal, Spectrochim. Acta, Part A, 2014, 127, 415–421 CrossRef CAS PubMed.
- G. Akkaya and F. Güzel, Application of some domestic wastes as new low-cost biosorbents for removal of methylene blue: kinetic and equilibrium studies, Chem. Eng. Commun., 2014, 201(4), 557–578 CrossRef CAS.
- R.-L. Liu, et al., Biomass-derived highly porous functional carbon fabricated by using a free-standing template for efficient removal of methylene blue, Bioresour. Technol., 2014, 154, 138–147 CrossRef CAS PubMed.
- R. Kumar and M. Barakat, Decolourization of hazardous brilliant green from aqueous solution using binary oxidized cactus fruit peel, Chem. Eng. J., 2013, 226, 377–383 CrossRef CAS.
- V. Gomez, M. Larrechi and M. Callao, Kinetic and adsorption study of acid dye removal using activated carbon, Chemosphere, 2007, 69(7), 1151–1158 CrossRef CAS PubMed.
- G. Crini and P.-M. Badot, Application of chitosan, a natural aminopolysaccharide, for dye removal from aqueous solutions by adsorption processes using batch studies: A review of recent literature, Prog. Polym. Sci., 2008, 33(4), 399–447 CrossRef CAS.
- S. K. Lagergren, About the theory of so-called adsorption of soluble substances, Sven. Vetenskapsakad. Handingarl, 1898, 24, 1–39 Search PubMed.
- F. A. Pavan, et al., Methylene blue biosorption from aqueous solutions by yellow passion fruit waste, J. Hazard. Mater., 2008, 150(3), 703–712 CrossRef CAS PubMed.
- Y. Zheng, et al., 3D hierarchical graphene oxide-NiFe LDH composite with enhanced adsorption affinity to Congo red, methyl orange and Cr (VI) ions, J. Hazard. Mater., 2019, 369, 214–225 CrossRef CAS PubMed.
- A. E. Pirbazari, E. Saberikhah and S. H. Kozani, Fe3O4–wheat straw: preparation, characterization and its application for methylene blue adsorption, Water Resour. Ind., 2014, 7, 23–37 CrossRef.
- K. Shen and M. Gondal, Removal of hazardous Rhodamine dye from water by adsorption onto exhausted coffee ground, J. Saudi Chem. Soc., 2017, 21, S120–S127 CrossRef CAS.
- C. Cojocaru, P. Samoila and P. Pascariu, Chitosan-based magnetic adsorbent for removal of water-soluble anionic dye: artificial neural network modeling and molecular docking insights, Int. J. Biol. Macromol., 2019, 123, 587–599 CrossRef CAS PubMed.
- S. I. Siddiqui, et al., Nigella sativa seed based nanocomposite-MnO2/BC: An antibacterial material for photocatalytic degradation, and adsorptive removal of Methylene blue from water, Environ. Res., 2019, 171, 328–340 CrossRef CAS PubMed.
- B. M. Thamer, A. Aldalbahi, M. Moydeen, H. El-Hamshary, A. M. Al-Enizi and M. H. El-Newehy, Effective adsorption of Coomassie brilliant blue dye using poly (phenylene diamine) grafted electrospun carbon nanofibers as a novel adsorbent, Mater. Chem. Phys., 2019, 234, 133–145 CrossRef CAS.
- S. Dutta, et al., Recent advances on the removal of dyes from wastewater using various adsorbents: A critical review, Mater. Adv., 2021, 2(14), 4497–4531 RSC.
- M. Purkait, S. DasGupta and S. De, Adsorption of eosin dye on activated carbon and its surfactant based desorption, J. Environ. Manage., 2005, 76(2), 135–142 CrossRef CAS PubMed.
- R. Cherbański and E. Molga, Intensification of desorption processes by use of microwaves—an overview of possible applications and industrial perspectives, Chem. Eng. Process., 2009, 48(1), 48–58 CrossRef.
- P. C. van Noort, et al., Slow and very slow desorption of organic compounds from sediment: influence of sorbate planarity, Water Res., 2003, 37(10), 2317–2322 CrossRef CAS PubMed.
- H. Patel, Review on solvent desorption study from exhausted adsorbent, J. Saudi Chem. Soc., 2021, 25(8), 101302 CrossRef CAS.
- T. Robinson, B. Chandran and P. Nigam, Studies on desorption of individual textile dyes and a synthetic dye effluent from dye-adsorbed agricultural residues using solvents, Bioresour. Technol., 2002, 84(3), 299–301 CrossRef CAS PubMed.
- A. Mittal, et al., Adsorptive removal of hazardous anionic dye “Congo red” from wastewater using waste materials and recovery by desorption, J. Colloid Interface Sci., 2009, 340(1), 16–26 CrossRef CAS PubMed.
- S. W. Won, et al., Performance, kinetics and equilibrium in biosorption of anionic dye Reactive Black 5 by the waste biomass of Corynebacterium glutamicum as a low-cost biosorbent, Chem. Eng. J., 2006, 121(1), 37–43 CrossRef CAS.
- M. P. Elizalde-Gonzàlez, et al., Characterization of an adsorbent prepared from maize waste and adsorption of three classes of textile dyes, Colloids Surf., A, 2006, 278(1–3), 89–97 CrossRef.
|
This journal is © The Royal Society of Chemistry 2023 |