DOI:
10.1039/D2QO00861K
(Research Article)
Org. Chem. Front., 2022,
9, 4854-4860
Photocatalytic fluorosulfonylation of aliphatic carboxylic acid NHPI esters†
Received
27th May 2022
, Accepted 23rd July 2022
First published on 25th July 2022
Abstract
Sulfonyl fluorides have been increasingly favored by medicinal chemists and chemical biologists because of their unique reactivity and stability, but their synthetic methods still have certain limitations. Based on the SO2 radical insertion/fluorination strategy, we have developed a novel method for photocatalytic induced synthesis of alkylsulfonyl fluorides (low to 1 mol% P.C. and up to 92% yield), which covers primary, secondary and tertiary aliphatic carboxylic acid NHPI esters, providing a facile method for the late-stage fluorosulfonylation of natural products and drugs. This method provides an efficient and reliable approach for the synthesis and application of alkylsulfonyl fluorides.
Introduction
The discovery of sulfonyl fluorides can be traced back to 1921, when Steinkopf treated toluene with fluorosulfonic acid.1 However, sulfonyl fluorides did not get enough attention until 2014, when Sharpless and colleagues proposed the concept of sulfur(VI) fluoride exchange (SuFEx),2 which became known as an excellent reaction for click chemistry and came to be widely used in organic synthesis,3 chemical biology,4 drug discovery,5 materials science,6etc.7 Then, a new chapter opened up for the syntheses and applications of sulfonyl fluorides. The growing demand for sulfonyl fluorides has led to an increasing emphasis on the development of novel and efficient synthetic methods.
In recent years, the synthesis methods of sulfonyl fluorides have developed rapidly.8 Typical methods mainly include: (1) accessing the fluorosulfonyl group via the chloride–fluoride exchange9 and alternative methods;10 (2) introducing sulfonyl fluoride fragments into the target molecules via sulfonyl fluoride hubs;11 and (3) direct fluorosulfonylation via FSO2 reagents or FSO2 synthons.12 Due to decades of development, the classic approaches to access the fluorosulfonyl group have proven to be a straightforward and efficient way.
DABSO was developed by Santos et al. in 198813 and was first applied as a novel SO2 source by Willis and co-workers to deliver aryl aminosulfonamides in 2010.14 Since then, DABSO has frequently been used as a solid precursor of SO2 gas in the synthesis of various sulfonyl products,15 especially arylsulfonyl fluorides in recent years (Scheme 1, I).16 Unsurprisingly, it can also be used to synthesize aliphatic sulfonyl fluorides.17 For example, the Willis group reported a radical approach toward primary and secondary alkylsulfonyl derivatives via donor–acceptor activation of pyridinium salts which were trapped by DABSO to generate sulfonyl radicals (Scheme 1, II, A).18 Hence, the SO2 radical insertion/fluorination strategy became a popular method for the construction of the fluorosulfonyl group, with special synthetic advantages in the direct synthesis of sulfonyl fluorides via C–SO2F bond formation.8 Very recently, the Weng group reported a photocatalytic decarboxylative fluorosulfonylation approach for converting aldoxime esters to the corresponding sulfonyl fluorides,17f which was enabled by energy-transfer-mediated photocatalysis and N–O bond homolysis. However, this protocol delivers the corresponding sulfonyl fluorides usually in modest yields and is not applicable to tertiary carboxylic acids (Scheme 1, II, B). Moreover, SO2 sources including SO2 gas and its surrogates,15a–c such as inorganic hypervalent sulfur sources like sodium/potassium metabisulfite, are the key factors for the introduction of sulfone groups.19
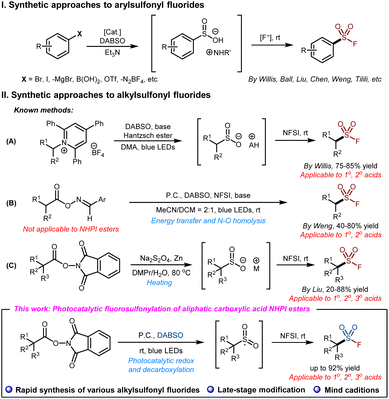 |
| Scheme 1 Approaches for fluorosulfonyl group installation via SO2 radical insertion/fluorination. | |
Aliphatic carboxylic acids, as a type of cheap and easily available organic compound, are widely used in the synthesis of various organic compounds. In particular, redox-active N-hydroxyphthalimide (NHPI) esters, readily prepared from abundant and widely available carboxylic acids, are considered to be reliable precursors of alkyl radicals, which are widely used in the synthesis of various C–X bonds.20 This year, the Liu group developed an efficient method for obtaining alkylsulfonyl fluorides from aliphatic carboxylic acid NHPI esters using sodium dithionite as the SO2 source.19i However, limited by the equivalent amount of zinc powder to be used and high temperature conditions, this method afforded the corresponding alkylsulfonyl fluoride products in moderate yields (Scheme 1, II, C). Our group is dedicated to the synthesis of various types of sulfonyl fluorides by the photocatalytic redox strategy. Based on the SO2 radical insertion/fluorination strategy, we envisaged a facile method to synthesize alkylsulfonyl fluorides through photocatalytic redox active ester decarboxylation with carboxylic acids as the alkyl radical source, DABSO as the SO2 source and NFSI as the fluorine source. Herein, we wish to report our efforts toward this goal and the establishment of a facile method to access aliphatic sulfonyl fluorides based on visible light-mediated decarboxylative radical fluorosulfonylation via SO2 radical insertion/fluorination of alkyl NHPI esters (low to 1 mol% P.C. and up to 92% yield). This method is characterized by the rapid synthesis of a broad range of alkylsulfonyl fluorides from primary, secondary, and tertiary acids, late-stage modification of natural products and drugs, and mild conditions (Scheme 1, II).
Results and discussion
To achieve the photocatalytic decarboxylated fluorosulfonylation of aliphatic carboxylic acid NHPI esters, an initial study was conducted with 4-bromophenylpropionic acid-derived NHPI ester 1a as the model substrate (Table 1). Ir[dF(CF3)ppy]2(dtbbpy)PF6 was first tested as the photocatalyst in combination with DIPEA as the reductant and DABSO as the SO2 source. After 3 h of stirring, NFSI was added as the F source and the reactions proceeded for an additional 1 h. The desired product 2a was obtained in 91% yield (85% isolated yield, Table 1, entry 1). The addition of Hantzsch ester (HE) as the additional reductant slightly reduced the yield (entry 2 vs. entry 1). This is probably due to the formation of a cloudy mixture upon the addition of HE, which negatively affects the light absorption of the photocatalyst. Next, changing the photocatalyst to eosin Y (disodium salt), fac-Ir(ppy)3, or Ru(bpy)3Cl2 would dramatically reduce the yields (entries 3–5). Furthermore, varying the solvent proved that MeCN, DMF, THF or DCM are all not suitable for the reaction (entries 6–9). Replacing the sacrificial agent DIPEA with Et3N also significantly reduced the yield to 13% (entry 10). When reducing the equivalents of DABSO or DIPEA, the yield of 2a also decreased accordingly (entries 11 and 12). In contrast, Selectfluor™ gave a reduced reaction yield of 18% compared to NFSI (entry 13). Also, more equivalents of NFSI did not result in a better yield for this reaction (entry 14). Finally, control experiments showed that photocatalyst, light source and inert gas protection were necessary (entries 15–17).
Table 1 Optimization of the reaction conditionsa

|
Entry |
Variation from the standard conditions |
Yieldb (%) |
Reaction conditions: 1a (0.2 mmol), DABSO (0.4 mmol), DIPEA (1.0 mmol), Ir[dF(CF3)ppy]2(dtbbpy)PF6 (1 mol%), i-PrOH (4.0 mL), 24 W blue LEDs (λmax = 460 nm), rt, argon, 3 h; then NFSI (0.4 mmol), 1 h; N.P. = no product was detected.
Yields were determined by 19F NMR analysis with 4-iodofluorobenzene as the internal standard.
Isolated yield is shown in parentheses.
|
1 |
None |
91 (85)c |
2 |
Addition of Hantzsch esters (2.0 eq.) |
85 |
3 |
Eosin Y-Na2 instead of Ir[dF(CF3)ppy]2(dtbbpy)PF6 |
6 |
4 |
fac-Ir(ppy)3 instead of Ir[dF(CF3)ppy]2(dtbbpy)PF6 |
17 |
5 |
Ru(bpy)3Cl2 instead of Ir[dF(CF3)ppy]2(dtbbpy)PF6 |
5 |
6 |
MeCN instead of i-PrOH |
13 |
7 |
DMF instead of i-PrOH |
17 |
8 |
THF instead of i-PrOH |
N.P. |
9 |
DCM instead of i-PrOH |
N.P. |
10 |
Et3N instead of DIPEA |
13 |
11 |
DABSO (1.5 eq.) |
84 |
12 |
DIPEA (3.0 eq.) |
61 |
13 |
Selectfluor™ instead of NFSI |
18 |
14 |
NFSI (3.0 eq.) |
90 |
15 |
Without P.C. |
N.P. |
16 |
No light |
Trace |
17 |
In air |
Trace |
Having established the optimized reaction conditions, we turned our attention to investigate the reaction scope. A variety of primary, secondary and tertiary carboxylic acid-derived NHPI esters were examined, and the results are shown in Table 2. In the case of primary carboxylic acids, we could observe good compatibility toward phenyl (2b, 86%), 4-methoxybenzene (2c, 79%), thienyl (2d, 78%), chloride (2e, 73%), C–C triple bond (2f, 70%) and benzyl (2g, 71%). Secondary acids could also be efficiently converted into the corresponding alkylsulfonyl fluorides in high yields (2h–2l, 78%–92%), including norbornylane (2i, 90%), cyclohexene (2j, 92%), cycloheptane (2k, 88%) and α-methylbenzyl (2l, 78%) substrates. Delightfully, compared with the existing synthetic methods of tertiary alkylsulfonyl fluorides which suffer from synthetic difficulties or moderate yields,17f,19i the most prominent feature of this novel method is that it allows for direct fluorosulfonylation of tertiary aliphatic carboxylic acid NHPI ester to access tertiary alkylsulfonyl fluorides in high yields, including adamantane (2n, 93%). Remarkably, due to mild conditions and excellent compatibility, this method can also be used in the late-stage modification of natural products or drugs to obtain the corresponding fluorosulfonylation products in good to high yields, including 2q (83%, from palmitic acid), 2t (79%, from oxaprozin), 2u (86%, from flubiprofen) and 2v (84%, from abietic acid). As shown in Table 2, primary, secondary and tertiary alkyl carboxylic acid-derived NHPI esters can all be well accommodated under the reaction conditions, providing rapid access to alkyl boronic esters with considerable structural diversity.
Standard conditions: at the 0.2 mmol scale, isolated yields.
|
|
In order to gain a better understanding of the reaction mechanism, 2,2,6,6-tetramethylpiperidinooxy (TEMPO) was added to the reactions under the standard conditions. It was found that the yield of the expected product 2a was dramatically reduced and the TEMPO-trapped product 3 was detected by HRMS analysis (Scheme 2A). In addition, we obtained the corresponding ring-opened fluorosulfonylation product 519i in 66% 19F yield when cyclopropionate was used as the radical probe under the standard conditions (Scheme 2B). The above results showed that the reaction proceeds through an alkyl radical mechanism. According to the above studies and previous work,21 a plausible mechanism for this visible light-mediated decarboxylative radical fluorosulfonylation via SO2 insertion/fluorination is proposed, as shown in Scheme 2C.
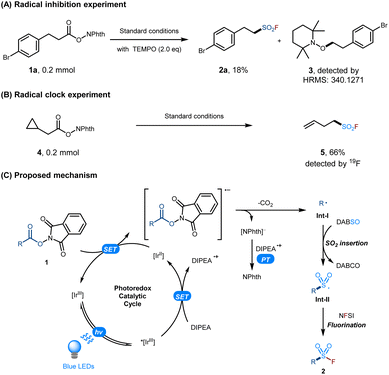 |
| Scheme 2 Mechanistic study and proposed mechanism. | |
Initially, the single electron transfer (SET) between the excited photocatalyst Ir[dF(CF3)ppy]2(dtbbpy)PF6 (P.C., [Ir]) and DIPEA produces DIPEA˙+ and the reductive [IrII] species. Subsequently, the reduction of NHPI esters 1 by the resulting [IrII] species leads to the formation of the alkyl radical Int-I and the regeneration of the photocatalyst [IrIII]. The insertion of SO2 into the alkyl radical Int-I then gives rise to the sulfonyl radical Int-II, which is captured by NFSI through fluorine atom transfer17e,f to afford the final fluorosulfonylation product 2.
To demonstrate the synthetic value of our products, we carried out the derivatization of the obtained product 2b (Scheme 3). We investigated the multifunctional synthesis of various sulfonyl-containing derivatives based on the fluorosulfonyl group. For example, sulfonyl azide 6 was obtained through SuFEx click reactions with trimethylsilyl azide (TMSN3). On the other hand, sulfonate ester 7 was achieved through the reaction of 2b with naphthol. Moreover, the sulfonate derivatives of estrone 8 can also be obtained in 77% yield by the SuFEx click reaction.
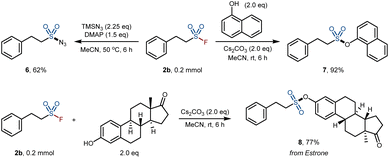 |
| Scheme 3 Derivatization reactions of 2-phenylethane-1-sulfonyl fluoride (2b). | |
Conclusions
In conclusion, by combining photoredox catalysis and SO2 radical insertion, a visible light-mediated decarboxylative radical fluorosulfonylation via SO2 insertion/fluorination of aliphatic carboxylic acid NHPI esters has been successfully developed for the first time. This method is applicable to a variety of alkyl carboxylic acids, including primary, secondary, and tertiary ones, as well as several natural products and drugs, allowing for facile access to various alkylsulfonyl fluorides, which are important compounds in multiple fields of chemistry, biology and materials science. Moreover, the value of the products has been demonstrated once again with a direct transformation of sulfonyl fluorides to sulfonyl azides and sulfonate esters. We anticipate that this radical photocatalytic decarboxylative fluorosulfonylation will provide a useful and complementary new approach for the synthesis of sulfonyl fluorides.
Author contributions
X. Nie conceived this study, developed the reactions, performed the reaction optimization and completed this manuscript; H. Zhang and S. Li completed the scope investigation, mechanistic study and product diversification; and H. Zheng, G. Zhu and S. Liao helped complete this manuscript with feedback from X. Nie. In addition, S. Liao provided financial and experimental material support. We thank the members of the NMR and HRMS departments for their excellent service.
Conflicts of interest
There are no conflicts to declare.
Acknowledgements
We gratefully acknowledge the Recruitment Program of Global Experts (1000plan), the Natural Science Foundation of China (No. 21602028), Beijing National Laboratory for Molecular Sciences (BNLMS201913) and Fuzhou University for the financial support.
Notes and references
-
(a) W. Steinkopf, Über Aromatische Sulfofluoride, J. Prakt. Chem., 1927, 117, 1–82 CrossRef CAS
;
(b) W. Steinkopf and P. Jaeger, Über Aromatische Sulfofluoride. II. Mitteilung, J. Prakt. Chem., 1930, 128, 63–88 CrossRef CAS
.
- J. Dong, L. Krasnova, M. G. Finn and K. B. Sharpless, Sulfur(VI) Fluoride Exchange (SuFEx): Another Good Reaction for Click Chemistry, Angew. Chem., Int. Ed., 2014, 53, 9430–9448 CrossRef CAS PubMed
.
- For selected reviews, see:
(a) P. K. Chinthakindi and P. I. Arvidsson, Sulfonyl Fluorides (SFs): More Than Click Reagents?, Eur. J. Org. Chem., 2018, 3648–3666 CrossRef CAS
;
(b) A. S. Barrow, C. J. Smedley, Q. Zheng, S. Li, J. Dong and J. E. Moses, The Growing Applications of SuFEx Click Chemistry, Chem. Soc. Rev., 2019, 48, 4731–4758 RSC
;
(c) L. Xu and J. Dong, Click Chemistry: Evolving on the Fringe, Chin. J. Chem., 2020, 38, 414–419 CrossRef CAS
;
(d) C. Lee, A. J. Cook, J. E. Elisabeth, N. C. Friede, G. M. Sammis and N. D. Ball, The Emerging Applications of Sulfur(VI) Fluorides in Catalysis, ACS Catal., 2021, 11, 6578–6589 CrossRef CAS PubMed
;
(e) T. Zhong, Z. Chen, J. Yi, G. Lu and J. Weng, Recent Progress in the Synthesis of Sulfonyl Fluorides for SuFEx Click Chemistry, Chin. Chem. Lett., 2021, 32, 2736–2750 CrossRef CAS
.
- For selected reviews, see:
(a) A. Narayanan and L. H. Jones, Sulfonyl Fluorides as Privileged Warheads in Chemical Biology, Chem. Sci., 2015, 6, 2650–2659 RSC
;
(b) L. H. Jones, Emerging Utility of Fluorosulfate Chemical Probes, ACS Med. Chem. Lett., 2018, 9, 584–586 CrossRef CAS PubMed
;
(c) P. Martin-Gago and C. A. Olsen, Arylfluorosulfate-Based Electrophiles for Covalent Protein Labeling: A New Addition to the Arsenal, Angew. Chem., Int. Ed., 2019, 58, 957–966 CrossRef CAS PubMed
;
(d) S. E. Dalton and S. Campos, Covalent Small Molecules as Enabling Platforms for Drug Discovery, ChemBioChem, 2020, 21, 1080–1100 CrossRef CAS PubMed
;
(e) L. H. Jones and J. W. Kelly, Structure-Based Design and Analysis of SuFEx Chemical Probes, RSC Med. Chem., 2020, 11, 10–17 RSC
.
-
(a) R. Artschwager, D. J. Ward, S. Gannon, A. J. Brouwer, H. van de Langemheen, H. Kowalski and R. M. J. Liskamp, Potent and Highly Selective Inhibitors of the Proteasome Trypsin-Like Site by Incorporation of Basic Side Chain Containing Amino Acid Derived Sulfonyl Fluorides, J. Med. Chem., 2018, 61, 5395–5411 CrossRef CAS PubMed
;
(b) Z. Liu, J. Li, S. Li, G. Li, K. B. Sharpless and P. Wu, SufEx Click Chemistry Enabled Late-Stage Drug Functionalization, J. Am. Chem. Soc., 2018, 140, 2919–2925 CrossRef CAS PubMed
;
(c) X. Yang, T. J. M. Michiels, C. de Jong, M. Soethoudt, N. Dekker, E. Gordon, M. van der Stelt, L. H. Heitman, D. van der Es and I. J. AP, An Affinity-Based Probe for the Human Adenosine A2A Receptor, J. Med. Chem., 2018, 61, 7892–7901 CrossRef CAS PubMed
;
(d) C. Baggio, P. Udompholkul, L. Gambini, A. F. Salem, J. Jossart, J. J. P. Perry and M. Pellecchia, Aryl-Fluorosulfate-Based Lysine Covalent Pan-Inhibitors of Apoptosis Protein (Iap) Antagonists with Cellular Efficacy, J. Med. Chem., 2019, 62, 9188–9200 CrossRef CAS PubMed
;
(e) H. Xu, F. Ma, N. Wang, W. Hou, H. Xiong, F. Lu, J. Li, S. Wang, P. Ma, G. Yang and R. A. Lerner, DNA-Encoded Libraries: Aryl Fluorosulfonates as Versatile Electrophiles Enabling Facile on-DNA Suzuki, Sonogashira, and Buchwald Reactions, Adv. Sci., 2019, 6, 1901551 CrossRef CAS PubMed
;
(f) S. Kitamura, Q. Zheng, J. L. Woehl, A. Solania, E. Chen, N. Dillon, M. V. Hull, M. Kotaniguchi, J. R. Cappiello, S. Kitamura, V. Nizet, K. B. Sharpless and D. W. Wolan, Sulfur(VI) Fluoride Exchange (SuFEx)-Enabled High-Throughput Medicinal Chemistry, J. Am. Chem. Soc., 2020, 142, 10899–10904 CrossRef CAS PubMed
;
(g) Q. Li, Q. Chen, P. C. Klauser, M. Li, F. Zheng, N. Wang, X. Li, Q. Zhang, X. Fu, Q. Wang, Y. Xu and L. Wang, Developing Covalent Protein Drugs via Proximity-Enabled Reactive Therapeutics, Cell, 2020, 182, 85–97 CrossRef CAS PubMed
;
(h) C. Liu, Q. Zhou, Y. Li, L. V. Garner, S. P. Watkins, L. J. Carter, J. Smoot, A. C. Gregg, A. D. Daniels, S. Jervey and D. Albaiu, Research and Development on Therapeutic Agents and Vaccines for COVID-19 and Related Human Coronavirus Diseases, ACS Cent. Sci., 2020, 6, 315–331 CrossRef CAS PubMed
;
(i) M. Teng, S. B. Ficarro, H. Yoon, J. Che, J. Zhou, E. S. Fischer, J. A. Marto, T. Zhang and N. S. Gray, Rationally Designed Covalent BCL6 Inhibitor That Targets a Tyrosine Residue in the Homodimer Interface, ACS Med. Chem. Lett., 2020, 11, 1269–1273 CrossRef CAS PubMed
.
-
(a) J. Dong, K. B. Sharpless, L. Kwisnek, J. S. Oakdale and V. V. Fokin, SuFEx-Based Synthesis of Polysulfates, Angew. Chem., Int. Ed., 2014, 53, 9466–9470 CrossRef CAS PubMed
;
(b) B. Gao, L. Zhang, Q. Zheng, F. Zhou, L. M. Klivansky, J. Lu, Y. Liu, J. Dong, P. Wu and K. B. Sharpless, Bifluoride-Catalysed Sulfur(VI) Fluoride Exchange Reaction for the Synthesis of Polysulfates and Polysulfonates, Nat. Chem., 2017, 9, 1083–1088 CrossRef CAS PubMed
;
(c) K. Durie, J. Yatvin, M. Kovaliov, G. H. Crane, J. Horn, S. Averick and J. Locklin, SuFEx Postpolymerization Modification Kinetics and Reactivity in Polymer Brushes, Macromolecules, 2018, 51, 297–305 CrossRef CAS
;
(d) T. Hmissa, X. Zhang, N. R. Dhumal, G. J. McManus, X. Zhou, H. B. Nulwala and A. Mirjafari, Autocatalytic Synthesis of Bifluoride Ionic Liquids by SuFEx Click Chemistry, Angew. Chem., Int. Ed., 2018, 57, 16005–16009 CrossRef CAS PubMed
;
(e) C. Yang, J. P. Flynn and J. Niu, Facile Synthesis of Sequence-Regulated Synthetic Polymers Using Orthogonal SuFEx and CuAAc Click Reactions, Angew. Chem., Int. Ed., 2018, 57, 16194–16199 CrossRef CAS PubMed
;
(f) Z. Cao, F. Zhou, P.-Y. Gu, D. Chen, J. He, J. R. Cappiello, P. Wu, Q. Xu and J. Lu, Preparation of Aryl Polysulfonates via a Highly Efficient SuFEx Click Reaction, Their Controllable Degradation and Functionalized Behavior, Polym. Chem., 2020, 11, 3120–3124 RSC
;
(g) H. Wan, S. Zhou, P. Gu, F. Zhou, D. Lyu, Q. Xu, A. Wang, H. Shi, Q. Xu and J. Lu, Aie-Active Polysulfates via a Sulfur(VI) Fluoride Exchange (SuFEx) Click Reaction and Investigation of Their Two-photon Fluorescence and Cyanide Detection in Water and in Living Cells, Polym. Chem., 2020, 11, 1033–1042 RSC
.
-
(a) G. F. Zha, W. Y. Fang, Y. G. Li, J. Leng, X. Chen and H. L. Qin, SO2F2 Mediated Oxidative Dehydrogenation and Dehydration of Alcohols to Alkynes, J. Am. Chem. Soc., 2018, 140, 17666–17673 CrossRef CAS PubMed
;
(b) P. J. Foth, F. Gu, T. G. Bolduc, S. S. Kanani and G. M. Sammis, New Sulfuryl Fluoride-Derived Alkylating Reagents for the 1,1-Dihydrofluoroalkylation of Thiols, Chem. Sci., 2019, 10, 10331–10335 RSC
;
(c) G. Meng, T. Guo, T. Ma, J. Zhang, Y. Shen, K. B. Sharpless and J. Dong, Modular Click Chemistry Libraries for Functional Screens Using a Diazotizing Reagent, Nature, 2019, 574, 86–89 CrossRef CAS PubMed
;
(d) C. J. Smedley, Q. Zheng, B. Gao, S. Li, A. Molino, H. M. Duivenvoorden, B. S. Parker, D. J. D. Wilson, K. B. Sharpless and J. E. Moses, Bifluoride Ion Mediated SuFEx Trifluoromethylation of Sulfonyl Fluorides and Iminosulfur Oxydifluorides, Angew. Chem., Int. Ed., 2019, 58, 4552–4556 CrossRef CAS PubMed
;
(e) M. C. Giel, C. J. Smedley, E. R. R. Mackie, T. Guo, J. Dong, T. P. Soares da Costa and J. E. Moses, Metal-Free Synthesis of Functional 1-Substituted-1,2,3-Triazoles from Ethenesulfonyl Fluoride and Organic Azides, Angew. Chem., Int. Ed., 2020, 59, 1181–1186 CrossRef CAS PubMed
;
(f) D. D. Liang, D. E. Streefkerk, D. Jordaan, J. Wagemakers, J. Baggerman and H. Zuilhof, Silicon-Free SuFEx Reactions of Sulfonimidoyl Fluorides: Scope, Enantioselectivity, and Mechanism, Angew. Chem., Int. Ed., 2020, 59, 7494–7500 CrossRef CAS PubMed
;
(g) S. Mahapatra, C. P. Woroch, T. W. Butler, S. N. Carneiro, S. C. Kwan, S. R. Khasnavis, J. Gu, J. K. Dutra, B. C. Vetelino, J. Bellenger, C. W. Am Ende and N. D. Ball, SuFEx Activation with Ca(NTf2)2: A Unified Strategy to Access Sulfamides, Sulfamates, and Sulfonamides from S(VI) Fluorides, Org. Lett., 2020, 22, 4389–4394 CrossRef CAS PubMed
;
(h) M. Mendel, I. Kalvet, D. Hupperich, G. Magnin and F. Schoenebeck, Site-Selective, Modular Diversification of Polyhalogenated Aryl Fluorosulfates (ArOSO2F) Enabled by an Air-Stable Pd(I) Dimer, Angew. Chem., Int. Ed., 2020, 59, 2115–2119 CrossRef CAS PubMed
.
- T. S.-B. Lou and M. C. Willis, Sulfonyl Fluorides as Targets and Substrates in the Development of New Synthetic Methods, Nat. Rev. Chem., 2022, 6, 146–162 CrossRef CAS
.
-
(a) T. A. Bianchi and L. A. Cate, Phase Transfer Catalysis. Preparation of Aliphatic and Aromatic Sulfonyl Fluorides, J. Org. Chem., 1977, 42, 2031–2032 CrossRef CAS
;
(b) J. J. Krutak, R. D. Burpitt, W. H. Moore and J. A. Hyatt, Chemistry of Ethenesulfonyl Fluoride. Fluorosulfonylethylation of Organic Compounds, J. Org. Chem., 1979, 44, 3847–3858 CrossRef CAS
.
-
(a) A. Shavnya, S. B. Coffey, K. D. Hesp, S. C. Ross and A. S. Tsai, Reaction of Alkyl Halides with Rongalite: One-Pot and Telescoped Syntheses of Aliphatic Sulfonamides, Sulfonyl Fluorides, and Unsymmetrical Sulfones, Org. Lett., 2016, 18, 5848–5851 CrossRef CAS PubMed
;
(b) L. Tang, Y. Yang, L. Wen, X. Yang and Z. Wang, Catalyst-Free Radical Fluorination of Sulfonyl Hydrazides in Water, Green Chem., 2016, 18, 1224–1228 RSC
;
(c) G. Laudadio, A. A. Bartolomeu, L. Verwijlen, Y. Cao, K. T. de Oliveira and T. Noel, Sulfonyl Fluoride Synthesis through Electrochemical Oxidative Coupling of Thiols and Potassium Fluoride, J. Am. Chem. Soc., 2019, 141, 11832–11836 CrossRef CAS PubMed
;
(d) P. K. T. Lo, Y. Chen and M. C. Willis, Nickel(II)-Catalyzed Synthesis of Sulfinates from Aryl and Heteroaryl Boronic Acids and the Sulfur Dioxide Surrogate Dabso, ACS Catal., 2019, 9, 10668–10673 CrossRef CAS
;
(e) L. Wang and J. Cornella, A Unified Strategy for Arylsulfur(VI) Fluorides from Aryl Halides: Access to Ar-SOF3 Compounds, Angew. Chem., Int. Ed., 2020, 59, 23510–23515 CrossRef CAS PubMed
.
-
(a) P. K. Chinthakindi, H. G. Kruger, T. Govender, T. Naicker and P. I. Arvidsson, On-Water Synthesis of Biaryl Sulfonyl Fluorides, J. Org. Chem., 2016, 81, 2618–2623 CrossRef CAS PubMed
;
(b) J. Leng and H. L. Qin, 1-Bromoethene-1-Sulfonyl Fluoride (1-Br-ESF), a New Sufex Clickable Reagent, and Its Application for Regioselective Construction of 5-Sulfonylfluoro Isoxazoles, Chem. Commun., 2018, 54, 4477–4480 RSC
;
(c) C. J. Smedley, M. C. Giel, A. Molino, A. S. Barrow, D. J. D. Wilson and J. E. Moses, 1-Bromoethene-1-Sulfonyl Fluoride (BESF) Is Another Good Connective Hub for Sufex Click Chemistry, Chem. Commun., 2018, 54, 6020–6023 RSC
;
(d) J. Thomas and V. V. Fokin, Regioselective Synthesis of Fluorosulfonyl 1,2,3-Triazoles from Bromovinylsulfonyl Fluoride, Org. Lett., 2018, 20, 3749–3752 CrossRef CAS PubMed
;
(e) Y.-P. Meng, S.-M. Wang, W.-Y. Fang, Z.-Z. Xie, J. Leng, H. Alsulami and H.-L. Qin, Ethenesulfonyl Fluoride (ESF) and Its Derivatives in Sufex Click Chemistry and More, Synthesis, 2019, 52, 673–687 Search PubMed
;
(f) X. Zhang, B. Moku, J. Leng, K. P. Rakesh and H.-L. Qin, 2-Azidoethane-1-Sulfonylfluoride (ASF): A Versatile Bis-Clickable Reagent for SuFEx and CuAAC Click Reactions, Eur. J. Org. Chem., 2019, 1763–1769 CrossRef CAS
;
(g) C. J. Smedley, G. Li, A. S. Barrow, T. L. Gialelis, M. C. Giel, A. Ottonello, Y. Cheng, S. Kitamura, D. W. Wolan, K. B. Sharpless and J. E. Moses, Diversity Oriented Clicking (DOC): Divergent Synthesis of Sufexable Pharmacophores from 2-Substituted-Alkynyl-1-Sulfonyl Fluoride (SASF) Hubs, Angew. Chem., Int. Ed., 2020, 59, 12460–12469 CrossRef CAS PubMed
.
-
(a) G. P. Roth and C. Sapino, Palladium in Cephalosporin Chemistry: An Inexpensive Triflate Replacement for Palladium Acetate Mediated Coupling Reactions, Tetrahedron Lett., 1991, 32, 4073–4076 CrossRef CAS
;
(b) T. Guo, G. Meng, X. Zhan, Q. Yang, T. Ma, L. Xu, K. B. Sharpless and J. Dong, A New Portal to Sufex Click Chemistry: A Stable Fluorosulfuryl Imidazolium Salt Emerging as an “F-SO2+” Donor of Unprecedented Reactivity, Selectivity, and Scope, Angew. Chem., Int. Ed., 2018, 57, 2605–2610 CrossRef CAS PubMed
;
(c) H. Zhou, P. Mukherjee, R. Liu, E. Evrard, D. Wang, J. M. Humphrey, T. W. Butler, L. R. Hoth, J. B. Sperry, S. K. Sakata, C. J. Helal and C. W. Am Ende, Introduction of a Crystalline, Shelf-Stable Reagent for the Synthesis of Sulfur(VI) Fluorides, Org. Lett., 2018, 20, 812–815 CrossRef CAS PubMed
;
(d) R. Lekkala, R. Lekkala, B. Moku, K. P. Rakesh and H.-L. Qin, Applications of Sulfuryl Fluoride (SO2F2) in Chemical Transformations, Org. Chem. Front., 2019, 6, 3490–3516 RSC
;
(e) X. Nie, T. Xu, Y. Hong, H. Zhang, C. Mao and S. Liao, Introducing a New Class of Sulfonyl Fluoride Hubs via Radical Chloro-Fluorosulfonylation of Alkynes, Angew. Chem., Int. Ed., 2021, 60, 22035–22042 CrossRef CAS PubMed
;
(f) X. Nie, T. Xu, J. Song, A. Devaraj, B. Zhang, Y. Chen and S. Liao, Radical Fluorosulfonylation: Accessing Alkenyl Sulfonyl Fluorides from Alkenes, Angew. Chem., Int. Ed., 2021, 60, 3956–3960 CrossRef CAS PubMed
;
(g) N. L. Frye, C. G. Daniliuc and A. Studer, Radical 1-Fluorosulfonyl-2-Alkynylation of Unactivated Alkenes, Angew. Chem., Int. Ed., 2022, 61, e202115593 CAS
.
- P. S. Santos and M. T. S. Mello, The Raman Spectra of Some Molecular Complexes of 1-Azabicyclo[2.2.2]Octane and 1,4-Diazabicyclo[2.2.2]Octane, J. Mol. Struct., 1988, 178, 121–133 CrossRef CAS
.
- B. Nguyen, E. J. Emmett and M. C. Willis, Palladium-Catalyzed Aminosulfonylation of Aryl Halides, J. Am. Chem. Soc., 2010, 132, 16372–16373 CrossRef CAS PubMed
.
- For selected reviews on DABSO, see:
(a) E. J. Emmett and M. C. Willis, The Development and Application of Sulfur Dioxide Surrogates in Synthetic Organic Chemistry, Asian J. Org. Chem., 2015, 4, 602–611 CrossRef CAS
;
(b) Y. Q. Li and Y. H. Fan, Recent Advances in C-S Bond Construction to Synthesize Sulfone, Synth. Commun., 2019, 49, 3227–3264 CrossRef CAS
;
(c) S. Liang, K. Hofman, M. Friedrich and G. Manolikakes, Recent Advances in the Synthesis and Direct Application of Sulfinate Salts, Eur. J. Org. Chem., 2020, 4664–4676 CrossRef CAS
;
(d) D. Zeng, M. Wang, W.-P. Deng and X. Jiang, The Same Oxygenation-State Introduction of Hypervalent Sulfur under Transition-Metal-Free Conditions, Org. Chem. Front., 2020, 7, 3956–3966 RSC
;
(e) J. A. Andrews and M. C. Willis, DABSO – a Reagent to Revolutionize Organosulfur Chemistry, Synthesis, 2022, 54, 1695–1707 CrossRef CAS
;
(f) M. Seyed Hashtroudi, V. Fathi Vavsari and S. Balalaie, DABSO as a SO2 Gas Surrogate in the Synthesis of Organic Structures, Org. Biomol. Chem., 2022, 20, 2149–2163 RSC
.
-
(a) A. T. Davies, J. M. Curto, S. W. Bagley and M. C. Willis, One-Pot Palladium-Catalyzed Synthesis of Sulfonyl Fluorides from Aryl Bromides, Chem. Sci., 2017, 8, 1233–1237 RSC
;
(b) A. L. Tribby, I. Rodriguez, S. Shariffudin and N. D. Ball, Pd-Catalyzed Conversion of Aryl Iodides to Sulfonyl Fluorides Using SO2 Surrogate DABSO and Selectfluor, J. Org. Chem., 2017, 82, 2294–2299 CrossRef CAS PubMed
;
(c) T. S. Lou, S. W. Bagley and M. C. Willis, Cyclic Alkenylsulfonyl Fluorides: Palladium-Catalyzed Synthesis and Functionalization of Compact Multifunctional Reagents, Angew. Chem., Int. Ed., 2019, 58, 18859–18863 CrossRef CAS PubMed
;
(d) Y. Liu, D. Yu, Y. Guo, J. C. Xiao, Q. Y. Chen and C. Liu, Arenesulfonyl Fluoride Synthesis via Copper-Catalyzed Fluorosulfonylation of Arenediazonium Salts, Org. Lett., 2020, 22, 2281–2286 CrossRef CAS PubMed
;
(e) D. Louvel, A. Chelagha, J. Rouillon, P. A. Payard, L. Khrouz, C. Monnereau and A. Tlili, Metal-Free Visible-Light Synthesis of Arylsulfonyl Fluorides: Scope and Mechanism, Chem. – Eur. J., 2021, 27, 8704–8708 CrossRef CAS PubMed
.
-
(a) Y. Liu, H. Wu, Y. Guo, J. C. Xiao, Q. Y. Chen and C. Liu, Trifluoromethylfluorosulfonylation of Unactivated Alkenes Using Readily Available Ag(O2CCF2SO2F) and N-Fluorobenzenesulfonimide, Angew. Chem., Int. Ed., 2017, 56, 15432–15435 CrossRef CAS PubMed
;
(b) Q. Lin, Y. Liu, Z. Xiao, L. Zheng, X. Zhou, Y. Guo, Q.-Y. Chen, C. Zheng and C. Liu, Intermolecular Oxidative Radical Fluoroalkylfluorosulfonylation of Unactivated Alkenes with (Fluoroalkyl)Trimethylsilane, Silver Fluoride, Sulfur Dioxide and N-fluorobenzenesulfonimide, Org. Chem. Front., 2019, 6, 447–450 RSC
;
(c) Y. Liu, Q. Lin, Z. Xiao, C. Zheng, Y. Guo, Q. Y. Chen and C. Liu, Zinc-Mediated Intermolecular Reductive Radical Fluoroalkylsulfination of Unsaturated Carbon-Carbon Bonds with Fluoroalkyl Bromides and Sulfur Dioxide, Chem. – Eur. J., 2019, 25, 1824–1828 CrossRef CAS PubMed
;
(d) P. J. Sarver, N. B. Bissonnette and D. W. C. MacMillan, Decatungstate-Catalyzed C(sp3)-H Sulfinylation: Rapid Access to Diverse Organosulfur Functionality, J. Am. Chem. Soc., 2021, 143, 9737–9743 CrossRef CAS PubMed
;
(e) T. Zhong, J. T. Yi, Z. D. Chen, Q. C. Zhuang, Y. Z. Li, G. Lu and J. Weng, Photoredox-Catalyzed Aminofluorosulfonylation of Unactivated Olefins, Chem. Sci., 2021, 12, 9359–9365 RSC
;
(f) Z. D. Chen, X. Zhou, J. T. Yi, H. J. Diao, Q. L. Chen, G. Lu and J. Weng, Catalytic Decarboxylative Fluorosulfonylation Enabled by Energy-Transfer-Mediated Photocatalysis, Org. Lett., 2022, 24, 2474–2478 CrossRef CAS PubMed
.
- J. A. Andrews, L. R. E. Pantaine, C. F. Palmer, D. L. Poole and M. C. Willis, Sulfinates from Amines: A Radical Approach to Alkyl Sulfonyl Derivatives via Donor-Acceptor Activation of Pyridinium Salts, Org. Lett., 2021, 23, 8488–8493 CrossRef CAS PubMed
.
-
(a) G. Qiu, L. Lai, J. Cheng and J. Wu, Recent Advances in the Sulfonylation of Alkenes with the Insertion of Sulfur Dioxide via Radical Reactions, Chem. Commun., 2018, 54, 10405–10414 RSC
;
(b) G. Qiu, K. Zhou, L. Gao and J. Wu, Insertion of Sulfur Dioxide via a Radical Process: An Efficient Route to Sulfonyl Compounds, Org. Chem. Front., 2018, 5, 691–705 RSC
;
(c) G. Qiu, K. Zhou and J. Wu, Recent Advances in the Sulfonylation of C-H Bonds with the Insertion of Sulfur Dioxide, Chem. Commun., 2018, 54, 12561–12569 RSC
;
(d) S. Ye, G. Qiu and J. Wu, Inorganic Sulfites as the Sulfur Dioxide Surrogates in Sulfonylation Reactions, Chem. Commun., 2019, 55, 1013–1019 RSC
;
(e) S. Ye, M. Yang and J. Wu, Recent Advances in Sulfonylation Reactions Using Potassium/Sodium Metabisulfite, Chem. Commun., 2020, 56, 4145–4155 RSC
;
(f) Q. Lin, Z. Ma, C. Zheng, X. J. Hu, Y. Guo, Q. Y. Chen and C. Liu, Arenesulfonyl Fluoride Synthesis via Copper–Free Sandmeyer–Type Fluorosulfonylation of Arenediazonium Salts, Chin. J. Chem., 2020, 38, 1107–1110 CrossRef CAS
;
(g) S. Liu, Y. Huang, X.-H. Xu and F.-L. Qing, Fluorosulfonylation of Arenediazonium Tetrafluoroborates with Na2S2O5 and N-fluorobenzenesulfonimide, J. Fluor. Chem., 2020, 240, 109653 CrossRef CAS
;
(h) T. Zhong, M. K. Pang, Z. D. Chen, B. Zhang, J. Weng and G. Lu, Copper-Free Sandmeyer-Type Reaction for the Synthesis of Sulfonyl Fluorides, Org. Lett., 2020, 22, 3072–3078 CrossRef CAS PubMed
;
(i) Z. Ma, Y. Liu, X. Ma, X. Hu, Y. Guo, Q.-Y. Chen and C. Liu, Aliphatic Sulfonyl Fluoride Synthesis via Reductive Decarboxylative Fluorosulfonylation of Aliphatic Carboxylic Acid NHPI Esters, Org. Chem. Front., 2022, 9, 1115–1120 RSC
.
- For selected reviews and articles on NHPI esters, see:
(a) J. Xuan, Z. G. Zhang and W. J. Xiao, Visible-Light-Induced Decarboxylative Functionalization of Carboxylic Acids and Their Derivatives, Angew. Chem., Int. Ed., 2015, 54, 15632–15641 CrossRef CAS PubMed
;
(b) Y. Jin and H. Fu, Visible–Light Photoredox Decarboxylative Couplings, Asian J. Org. Chem., 2017, 6, 368–385 CrossRef CAS
;
(c) S. Murarka,
N-(Acyloxy)Phthalimides as Redox-Active Esters in Cross-Coupling Reactions, Adv. Synth. Catal., 2018, 360, 1735–1753 CrossRef CAS
;
(d) J. Schwarz and B. König, Decarboxylative Reactions with and without Light – a Comparison, Green Chem., 2018, 20, 323–361 RSC
;
(e) J. He, G. Chen, B. Zhang, Y. Li, J.-R. Chen, W.-J. Xiao, F. Liu and C. Li, Catalytic Decarboxylative Radical Sulfonylation, Chem, 2020, 6, 1149–1159 CrossRef CAS
;
(f) S. Karmakar, A. Silamkoti, N. A. Meanwell, A. Mathur and A. K. Gupta, Utilization of C(sp3)–Carboxylic Acids and Their Redox–Active Esters in Decarboxylative Carbon–Carbon Bond Formation, Adv. Synth. Catal., 2021, 363, 3693–3736 CrossRef CAS
;
(g) S. K. Parida, S. K. Hota, R. Kumar and S. Murarka, Late-Stage Alkylation of Heterocycles Using N-(Acyloxy)Phthalimides, Chem. – Asian J., 2021, 16, 879–889 CrossRef CAS PubMed
;
(h) S. K. Parida, T. Mandal, S. Das, S. K. Hota, S. De Sarkar and S. Murarka, Single Electron Transfer-Induced Redox Processes Involving N-(Acyloxy)Phthalimides, ACS Catal., 2021, 11, 1640–1683 CrossRef CAS
.
-
(a) R. Xu, T. Xu, M. Yang, T. Cao and S. Liao, A Rapid Access to Aliphatic Sulfonyl Fluorides, Nat. Commun., 2019, 10, 3752–3758 CrossRef PubMed
;
(b) T. Cao, T. Xu, R. Xu, X. Shu and S. Liao, Decarboxylative Thiolation of Redox-Active Esters to Free Thiols and Further Diversification, Nat. Commun., 2020, 11, 5340–5347 CrossRef CAS PubMed
;
(c) X. Shu, R. Xu, Q. Ma and S. Liao, Accessing Alkyl Boronic Esters via Visible Light-Mediated Decarboxylative Addition Reactions of Redox-Active Esters, Org. Chem. Front., 2020, 7, 2003–2007 RSC
;
(d) X. Shu, R. Xu and S. Liao, Photocatalytic Divergent Decarboxylative Amination: A Metal-Free Access to Aliphatic Amines and Hydrazines, Sci. China: Chem., 2021, 64, 1756–1762 CrossRef CAS
.
|
This journal is © the Partner Organisations 2022 |