DOI:
10.1039/C5FO00714C
(Paper)
Food Funct., 2016,
7, 171-182
Polyphenolic extract from Hibiscus sabdariffa reduces body fat by inhibiting hepatic lipogenesis and preadipocyte adipogenesis
Received
14th June 2015
, Accepted 4th October 2015
First published on 8th October 2015
Abstract
Diets high in fat lead to excess lipid accumulation in adipose tissue, which is a crucial factor in the development of obesity, hepatitis, and hyperlipidemia. In this study, we investigated the anti-obesity effect of Hibiscus sabdariffa extract (HSE) in vivo. Hamsters fed a high-fat diet (HFD) develop symptoms of obesity, which were determined based on body weight changes and changes in plasma and serum triglycerides, free fatty acid concentrations, total cholesterol levels, LDL-C levels, HDL-C levels, and adipocyte tissue weight. HFD-fed hamsters were used to investigate the effects of HSE on symptoms of obesity such as adipogenesis and fatty liver, loss of blood glucose regulation, and serum ion imbalance. Interestingly, HSE treatment effectively reduced the effects of the HFD in hamsters in a dose-dependent manner. Further, after inducing maturation of preadipocytes, Hibiscus sabdariffa polyphenolic extract (HPE) was shown to suppress the adipogenesis of adipocytes. However, HPE does not affect the viability of preadipocytes. Therefore, both HSE and HPE are effective and viable treatment strategies for preventing the development and treating the symptoms of obesity.
1. Introduction
Obesity is one of the major public health problems in developed countries. Obesity is a metabolic disease characterized by an increase in fat mass and body weight.1 The metabolic disorders associated with obesity show symptoms of insulin resistance, nonalcoholic fatty liver disease (NAFLD), atherosclerosis, degenerative disorders (e.g., dementia), immune-mediated disorders, and cancers.1,2 Extensive adipose tissue remodeling occurs during the development of obesity in the form of adipocyte hypertrophy, adipocyte hyperplasia, and angiogenesis.3
During adulthood, changes in the adipocyte number result from a complex interplay between the proliferation of preadipocytes and the differentiation of preadipocytes to adipocytes.4 Under normal conditions, adipocyte tissue development begins during gestation and proceeds until adolescence through increased proliferation of preadipocytes and their subsequent differentiation into adipocytes.5 Progenitor preadipocytes are committed to differentiate into white adipocytes of the adipose and also comprise a significant portion of fat tissue (15–50%).4,6 Differentiated adipocytes store fatty acids in the cytoplasm in the form of triglycerides (TGs) with the involvement of various enzymes such as fatty acid synthase (FASN) and acetyl-CoA carboxylase (ACC). The sterol regulatory element binding protein 1(SREBP-1) is a master transcriptional regulator that plays a critical role in regulating fatty acid synthesis and has two types of patterns: a precursor segment and a mature segment. ALK 7 plays an important role in maintaining the balance between energy expenditure and fat accumulation by suppressing adipocyte mitochondrial biogenesis, fatty acid oxidation, and β-AR mediated-lipolysis.7,8
Several studies have demonstrated the efficiency of various natural compounds to suppress adipogenesis and thereby prevent obesity in animal models.9–12
Polyphenols are a large and ubiquitous group of bio-active phytochemicals,13 which are well known antioxidant agents that exhibit effective anti-inflammatory, anti-allergy, hepatoprotective, antithrombotic, antiviral, and anti-carcinogenic activities.14
Serum total cholesterol (TC), TG, free fatty acids (FFA), high density lipoproteins (HDL), low density lipoproteins (LDL), and blood glucose levels of high-fat diet (HFD)-fed rats can be regulated by treating with polyphenols obtained from various sources.15–17 In addition, various polyphenols have shown promising hepatoprotective effects against drug-induced hepatic necrosis and cholestatic liver injury in rodents.18
Hibiscus sabdariffa, a tropical herb that has been used worldwide in cold and hot beverages, is known for its potential protective effects against many diseases such as hypertension, inflammation, and liver disease.19,20 In our previous work, HSE inhibited LDL oxidation in vitro and decreased serum cholesterol levels in cholesterol-fed rats and rabbits. Further, polyphenol-rich HSE has demonstrated chemopreventive properties by acting through the mitogen-activated protein kinase (MAPK) signaling pathway.21–23 HSE capsules are also known to reduce serum cholesterol in human subjects.19 Our previous study also showed that HSE can act as an adjuvant for preventing obesity in humans.24
In this study, HSE and the HSE polyphenol fraction (HPE) were tested for anti-obesity activities in the HFD-induced obesity animal model. The inhibitory effects of HSE on 3T3-L1 adipocytes were examined to determine the influence of HSE on 3T3-L1 preadipocyte differentiation. We also monitored improvements in metabolic syndrome and symptoms of liver damage following HSE anti-obesity treatment.
2. Materials and methods
2.1. Preparation of HSE and HPE
HSE was prepared from H. sabdariffa (Malvaceae) and analyzed according to our previous report.21 Briefly, H. sabdariffa L. (150 g) was macerated with hot water (95 °C, 6000 mL) for 2 h, and the aqueous extract was evaporated under vacuum at −85 °C. The extracted solution was filtered, lyophilized to obtain 75 g of HSE, and stored at 4 °C before use. To prepare the HPE, 100 g of H. sabdariffa L. was extracted three times with 300 mL of methanol at 50 °C for 3 h. The extracts were filtered and the solvent was removed using a vacuum rotary evaporator. The residue was dissolved in 500 mL of water (50 °C) and extracted with 200 mL of hexane to remove some of the pigments. The aqueous phase was extracted three times with 180 mL of ethyl acetate, which was then evaporated under reduced pressure. The residue was redissolved in 250 mL of water, lyophilized to yield approximately 2 g of HPE, and stored at −20 °C before use. HPLC analysis was performed on a Hitachi system series L-6200A (Tokyo, Japan) equipped with a Hitachi D-7000 chromatography data station software, a Hitachi L-4250 UV-visible detector and a column. Wavelength monitoring was performed at 260 nm. Separation of polyphenols was carried out on a Mightysil RP-18 (250 × 4.6 mm) column from Kanto (Kanto Chemical, Tokyo, Japan). The elution solvents consisted of acetic acid/water (2
:
98, v/v, solvent A) and 0.5% in water/acetonitrile (50
:
50, v/v, solvent B). All solvents were filtered with a 0.22 μm membrane filter. The flow rate was 1.0 mL min−1 and the injection volume was 20 μL. Quantification of polyphenols was performed by the external standard method. Triplicate tests were conducted for each sample. The HPE extract was analyzed by HPLC as protocatechuic acid (24%), catechin (3%), gallocatechins (2%), caffeic acid (20%), and gallocatechin gallates (28%) and with 74% polyphenol content, and HSE also analyzed by HPLC as protocatechuic acid (9%), catechin (10%), caffeic acid (18%), gallocatechin gallates (20%) and with 2% polyphenol content. The biochemical constituents and characteristics of HPE and HSE have been published in our previous study.25
2.2. Animals and experimental design
All animal experiments were performed according to the protocols approved by the Institutional Animal Care and Use Committee of Chung Shan Medical University in Taichung, Taiwan (no. 513). Male Syrian hamsters, aged 7 weeks and weighing 120 g, were purchased from the National Laboratory Animal Breeding and Research Center (Taipei, Taiwan) and housed under standard laboratory conditions (18–23 °C, 55–60% humidity, and a 12 h light/dark cycle) for 1 week before the experiments were performed to allow the animals to adapt to their environment. All Syrian hamsters were randomly divided into six groups (n = 8 per group) as follows: control (normal meals); HFD (normal meals containing 10% coconut oil and 0.5% cholesterol); and HFD supplemented with 0.5%, 1.0%, or 2.0% HSE. After 10 weeks of feeding on the aforementioned diets, the Syrian hamsters were fasted for 12–14 h and then sacrificed. Whole blood and livers were then collected from the Syrian hamsters.
2.3. Blood sample analysis
Blood samples from the Syrian hamsters were collected in EDTA tubes and immediately centrifuged at 1500g for 10 min. Then, the serum was decanted, and the samples were stored at 4 °C. Biochemical examinations were performed within 1 h of specimen collection. Serum levels of TG, TC, HCL-C, LDL-C, alanine transaminase (ALT), and aspartate aminotransferase (AST) were measured using clinical chemistry reagent kits (Randox Laboratories, Antrim, UK). Free fatty acids were assayed using a free fatty acid quantification kit (BioVision, Mountain View, CA) according to the manufacturer's protocol. Briefly, the free fatty acids in the plasma of Syrian hamsters were converted to their CoA derivatives, which were subsequently oxidized with the concomitant generation of color. FFA was then easily quantified using a colorimetric (spectrophotometrically at λ = 570 nm) method.
2.4. Determination of TC and TG in the liver
Portions of fresh livers from the Syrian hamsters were collected for liver lipid extraction. Liver tissues (1.25 g) were homogenized with chloroform
:
methanol (1
:
2, 3.75 mL). Then, chloroform (1.25 mL) and distilled water (1.25 mL) were added to the homogenate and mixed well. After centrifugation (1500g for 10 min), the lower clear organic phase solution was transferred into a new tube and lyophilized. The lyophilized powder was dissolved in chloroform
:
methanol (1
:
2) to prepare the liver lipid extract and stored at −20 °C for less than 3 days. The liver TGs and liver cholesterol in the lipid extracts were measured by enzymatic colorimetric methods using commercial kits (HUMAN, Wiesbaden, Germany).
2.5. Cell culture
Mouse embryo derived 3T3-L1 cells (BCRC 60159) were obtained from the Bioresource Collection and Research Center (BCRC, Food Industry Research and Development Institute, Hsinchu, Taiwan). The culture medium included DMEM, 10% calf serum, 1.5 g per L sodium bicarbonate, and 100 U per mL penicillin–streptomycin. The cells were maintained at 37 °C in a humidified 5% CO2 incubator.
2.6. MTT assay
The 3T3-L1 pre-adipocytes were plated into 24-well plates at a density of 1 × 104 cells per well. After 24 h, the culture medium was replaced with the indicated concentration of MLE or MLPE, and the cells were incubated for 2–14 days. Culture solutions were removed and replaced with new culture medium. The sterile filtered MTT solution (5 mg mL−1) in phosphate buffered saline (PBS, 0.85% NaCl, 2.68 mM KCl, 10 mM Na2HPO4, and 1.76 mM KH2 PO4 were dissolved in distilled water, pH 7.4) was added to each well at a final concentration of 0.5 mg MTT per mL. Unreacted dye was removed after 4 h. The insoluble formazan crystals were dissolved in 1000 mL per well of isopropanol and measured spectrophotometrically using a Hitachi U2900 spectrophotometer (Hitachi, Ltd, Tokyo, Japan) at 563 nm. Inhibition (%) was expressed as the percentage of cell growth compared to non-treated control cells.
2.7. Adipocyte maturation assay
Mature adipocyte were seeded in a 6-well plate (3 × 106 cells) and treated with the indicated concentration of HSE for 14 days. After being washed twice with PBS, the cells were fixed with 4% formaldehyde for 30 min and then stained with 0.05 g per mL Oil Red O or 1 μg per mL Nile red for 30 min at room temperature. The red lipid droplets in the Oil Red O were visualized using a light microscope. Lipid-bound Nile red fluorescence was detected, and the lipid content in the cells was immediately analyzed and quantified by using a flow cytometer (Becton Dickinson, Mountain View, CA, USA).
2.7. Annexin V-FITC/PI double staining analysis
Annexin V-FITC/PI double staining of the cells was determined using an Annexin V-FITC kit (Vybrant™ Apoptosis Assay, V-13242, Molecular Probes, Eugene, OR, USA) to detect early apoptosis, late apoptosis, and necrosis induced by HSE or HPE. Mature 3T3-L1 adipocytes (1 × 106 cells per dish) were added to each well of a 6 cm dish at 37 °C in culture medium containing the indicated concentration of HSE or HPE for 72 h. Approximately 1 × 105 cells were then stained for 10 min at room temperature with Annexin V-FITC and PI in a Ca2+-enriched binding buffer (Annexin V-FITC kit) and analyzed by FACScan flow cytometry. Annexin V-FITC and PI emissions were detected in the FL1 and FL2 channels of an FACScan flow cytometer using the emission filters of 525 and 575 nm, respectively. Approximately 1 × 104 counts were made for each sample. The percentages of normal (Annexin V-FITC−/PI−), early apoptotic (Annexin V-FITC+/PI−), late apoptotic (Annexin V-FITC+/PI+), and necrotic cells (Annexin V-FITC−/PI+) were calculated using CELL Quest software.
2.8. Western blot analysis
Equal amounts of protein samples (50 μg) were subjected to SDS−polyacrylamide gel electrophoresis and transferred onto nitrocellulose membranes (Millipore, Bedford, MA, USA). The membranes were blocked with 5% nonfat milk powder containing 0.05% Tween 20 in PBS and then incubated with the primary antibody at 4 °C overnight. Next, the membranes were washed three times with 0.05% Tween 20 in PBS and incubated with an anti-mouse secondary antibody conjugated to horseradish peroxidase (GE Healthcare, Little Chalfont, Buckinghamshire, UK). The bands were detected by enhanced chemiluminescence using ECL western blotting detection reagents and exposed on an ECL hyperfilm using a FUJFILM Las-3000 (Tokyo, Japan). Protein quantitation was determined by densitometry using FUJFILM MultiGauge V2.2 software.
2.9. Statistical analysis
The data were analyzed using an unpaired t-test and represented as the means (standard deviation (SD)). Significant differences were evaluated using an unpaired t-test and considered significant at the level p < 0.05. All data collected were analyzed using an unpaired t-test after one-way ANOVA showed a significant difference among all the groups (p < 0.05).
3. Results
3.1. The effect of HSE on body weight and food efficiency
To verify the effects of HSE in vivo, male Syrian hamsters were fed a high-fat diet feed containing 10% coconut oil and 0.2% cholesterol for 10 weeks. Consumption of the HFD for 10 weeks resulted in a significant increase in body weight gain and feeding efficiency of the hamster. The body weight gain of the HFD group significantly increased compared with that of the control group. HSE supplementation did not cause any change in the food intake of the HFD-fed hamsters. However, supplementation of HSE in the HFD group resulted in a significant decrease in body weight gain compared with the HFD group (Table 1). HPE showed similar effects to HSE.
Table 1 A comparison of body weight and adipose tissue content in HFD-fed hamsters
|
Control |
HFD |
HFD + 0.5% HSE |
HFD + 1.0% HSE |
HFD + 2.0% HSE |
Each value is expressed as the mean ± SD (n = 8 per group). Statistical significance was analyzed with ANOVA. #, p < 0.05 as compared to the control group. *, p < 0.05 as compared to the HFD group. |
Body weight change |
24.26 ± 4.66 |
32.48 ± 10.96# |
23.72 ± 6.31* |
20.17 ± 13.01* |
20.85 ± 9.52* |
Feeding efficiency |
0.67 ± 1.69 |
0.48 ± 1.24 |
−0.04 ± 1.13 |
−0.82 ± 3.15 |
−1.08 ± 2.87 |
Gonadal fat tissue/body weight |
0.17 ± 0.01 |
0.22 ± 0.03# |
0.19 ± 0.02* |
0.18 ± 0.03* |
0.17 ± 0.02* |
Pararenal fat tissue/body weight |
0.12 ± 0.01 |
0.17 ± 0.03# |
0.14 ± 0.02 |
0.14 ± 0.03 |
0.12 ± 0.04* |
Total fat tissue weight/body weight |
0.30 ± 0.02 |
0.39 ± 0.07# |
0.34 ± 0.04* |
0.33 ± 0.07* |
0.31 ± 0.07* |
3.2. Analysis of plasma and liver lipid levels
The results from the analysis of plasma lipid levels are shown in Fig. 1. Hamsters fed a normal diet were used as a control. The following groups were analyzed: HSE-0%, hamsters fed a high-fat diet without HSE; HSE 0.5%, hamsters fed a high-fat diet with 0.5% HSE; HSE 1%, hamsters fed a high-fat diet with 1% HSE; and HSE 2%, hamsters fed a high-fat diet with 2% HSE. Triglycerides (Fig. 1A), fatty acid concentrations (Fig. 1B), total cholesterol content (Fig. 1C), LDL-C (Fig. 1D), HDL-C (Fig. 1E), and LDL-C/HDL-C (Fig. 1F) were decreased in the serum from hamsters treated with HSE. The results from the analysis of liver lipid levels are shown in Fig. 2. Hamsters fed a normal diet were used as a control. The following groups were analyzed: HSE-0%, hamsters fed a high-fat diet without HSE; HSE 0.5%, hamsters fed a high-fat diet with 0.5% HSE; HSE 1%, hamsters fed a high-fat diet with 1% HSE; and HSE 2%, hamsters fed a high-fat diet with 2% HSE. The liver cholesterol content (Fig. 2A) and liver triglyceride levels (Fig. 2B) decreased in a dose-dependent manner following HSE treatment.
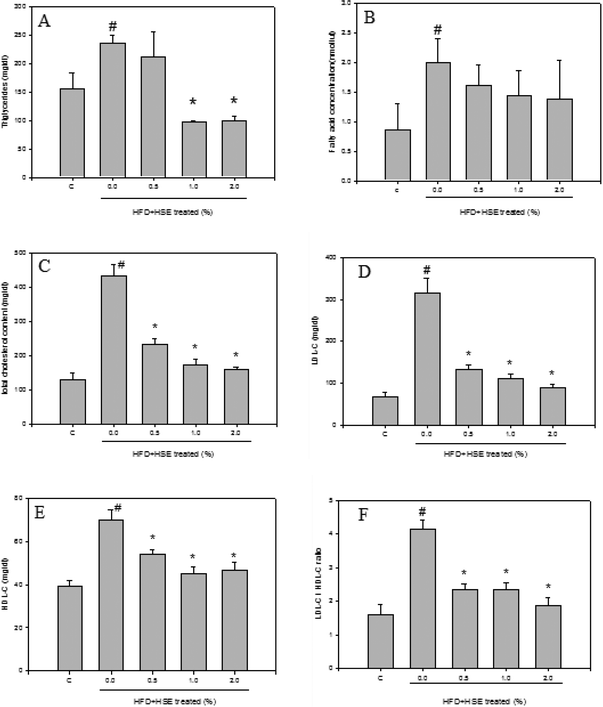 |
| Fig. 1 The effect of HSE supplementation on plasma lipid levels in HFD-fed hamsters. Male Syrian (7 weeks old) hamsters were individually housed and maintained at 25 °C with a 12 h light/dark cycle. The induced group of Syrian hamsters was maintained on a HFD containing 10% coconut oil and 0.2% cholesterol for 10 weeks. The levels of triglycerides (A), fatty acids (B), total cholesterol (C), LDL-C (D), HDL-C (E), and LDL-C/HDL-C (F) were measured in HFD-fed hamsters that had been supplemented with or without various concentrations of HSE. Corresponding levels in hamsters fed a normal diet (control, C) were used as the control. The data are shown as the mean ± SD: *, p < 0.05 as compared to the HFD group. #, p < 0.05 as compared to the normal group. | |
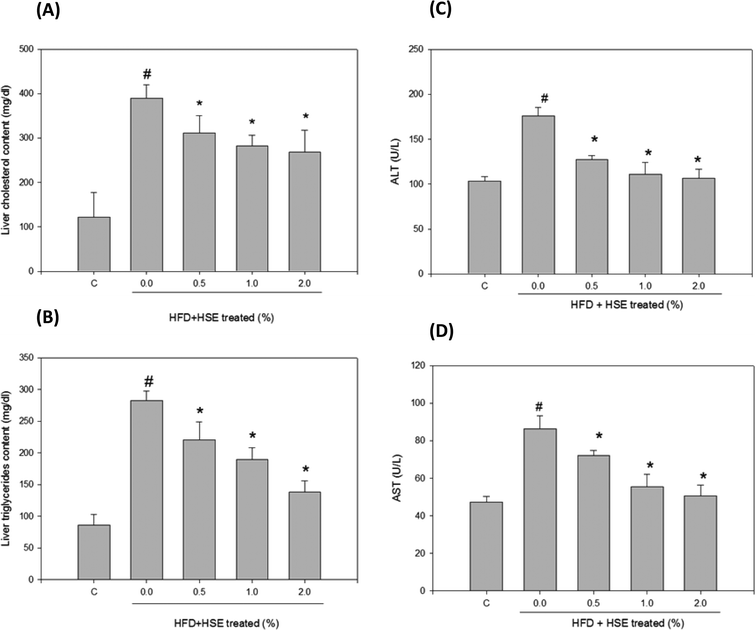 |
| Fig. 2 The effect of HSE supplementation on liver lipid and serum levels in HFD-fed hamsters. Male Syrian (7 weeks old) hamsters were individually housed and maintained at 25 °C with a 12 h light/dark cycle. The induced group of Syrian hamsters was maintained on a HFD containing 10% coconut oil and 0.2% cholesterol for 10 weeks. The liver cholesterol content (A), the levels of liver triglycerides (B), serum ALT (C) and serum AST (D) activities were measured in HFD-fed hamsters that had been supplemented with or without various concentrations of HSE. Corresponding levels in hamsters fed a normal diet (control, C) were used as the control. The data are shown as the mean ± SD: *, p < 0.05 as compared to the HFD group. #, p < 0.05 as compared to the normal group. | |
3.3. Effects of HSE on the liver function and blood biochemical parameters
Plasma ALT and AST levels were analyzed in hamsters fed HFD or HFD containing 0.5% HSE, 1.0% HSE, or 2.0% HSE. ALT (Fig. 2C) and AST (Fig. 2D) values decreased in a dose-dependent manner following HSE treatment for 10 weeks (n = 8/group). BUN and CRE levels did not significantly change compared with the control (Table 2). Glucose and ketone body values did not significantly change compared with the control (Table 2). After 10 weeks of being fed a HFD or a HFD containing 0.5% HSE, 1.0% HSE, or 2.0% HSE, serum ion levels were analyzed in the male hamsters (n = 8/group). Potassium and sodium levels did not significantly change compared with the control (Table 2).
Table 2 Effects of HSE on the serum biochemical parameters in HFD-fed hamsters
|
ND |
HFD |
HFD + HSE 0.5% |
HFD + HSE 1.0% |
HFD + HSE 2.0% |
Each value is expressed as the mean ± SD (n = 8 per group). Statistical significance was analyzed with ANOVA. |
BUN (mg dL−1) |
13.32 ± 1.81 |
15.09 ± 2.58 |
14.56 ± 1.72 |
13.42 ± 4.47 |
13.36 ± 1.86 |
UA (mg dL−1) |
1.00 ± 0.16 |
1.01 ± 0.25 |
1.02 ± 0.33 |
1.00 ± 0.18 |
1.00 ± 0.13 |
Creatinine (mg dL−1) |
0.77 ± 0.05 |
0.82 ± 0.06 |
0.82 ± 0.04 |
0.79 ± 0.03 |
0.78 ± 0.05 |
Ketone body (mmol L−1) |
0.19 ± 0.07 |
0.19 ± 0.07 |
0.19 ± 0.05 |
0.19 ± 0.06 |
0.19 ± 0.07 |
Sodium (mmol L−1) |
146.92 ± 1.56 |
149.33 ± 3.60 |
148.92 ± 1.44 |
147.83 ± 1.47 |
147.17 ± 1.47 |
Potassium (mmol L−1) |
6.61 ± 0.48 |
6.98 ± 0.42 |
6.90 ± 0.52 |
6.73 ± 0.47 |
6.72 ± 0.46 |
Glucose (mg dL−1) |
16.42 ± 9.07 |
28.58 ± 8.34 |
23.75 ± 5.34 |
23.08 ± 7.59 |
21.50 ± 6.02 |
3.4. The effect of HSE and HPE on premature adipocyte cell viability
The MTT assay performed on 3T3-L1 cells at different time points (2, 4, 6, 8, 10, 12, or 14 days) showed that HSE (1 or 2 mg mL−1) and HPE (0.25 or 0.5 mg mL−1) did not alter the cell viability (Fig. 3). Therefore, a concentration of 2 mg mL−1 of HSE was considered to be a non-lethal dose for HSE treatment. Similarly, concentrations of HPE up to 0.5 mg mL−1 did not affect the cell viability (Fig. 3).
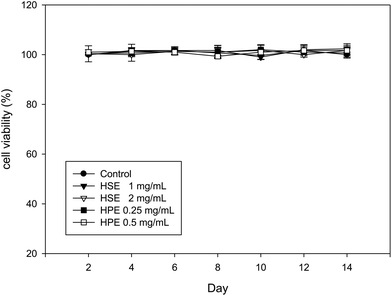 |
| Fig. 3 HSE is not cytotoxic to 3T3-L1 preadipocyte cells. 3T3-L1 preadipocyte cells were incubated with various concentrations of HSE (1 or 2 mg mL−1) or HPE (0.5 or 1 mg mL−1) at 37 °C for the indicated times. 3T3-L1 preadipocyte cells treated without any extract served as the negative control. Cell viability was measured using an MTT assay. The data are shown as the means ± SD from three replicates per treatment. | |
3.5. The effect of HSE and HPE on the adipogenesis of preadipocytes
Oil Red O was used to stain 3T3-L1 cells. Then, after differentiation of 3T3-L1 cells, a strong reduction in fatty molecules was observed at 1 mg mL−1 and 2 mg mL−1 of HSE when compared with the completely differentiated adipocytes (Fig. 4). The loss of fat molecules was due to the suppression of preadipocyte differentiation by HSE treatment (Fig. 4). HPE was more efficient in suppressing adipogenesis than HSE (Fig. 4). The markers of adipocyte differentiation, such as SREBP 1 and ALK7, and the markers of free fatty acid synthesis, such as FASN, were found decrease in a concentration-dependent manner following treatment with HSE. The phosphorylation of ACC and AMPK was also increased on treating with HSE. The results show a decrease in adipocyte differentiation and fatty acid synthesis, which reflects an ameliorating effect of HSE on obesity. A similar trend was observed in the 3T3-L1 pre-adipocytes when treated with HPE. HPE was more efficient in regulating the markers of adipocyte differentiation and free fatty acid synthesis (Fig. 5).
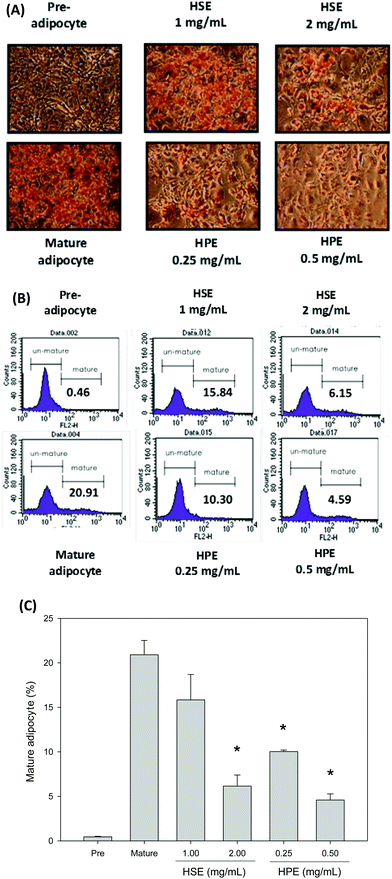 |
| Fig. 4 HSE and HPE inhibited adipogenesis of mature adipocyte cells. Post-confluent 3T3-L1 pre-adipocytes were treated with HSE or HPE at the indicated concentrations for 14 days. Then, the cells were stained with Oil Red O (A), and flow cytometry was performed (B). The fluorescence was quantified by flow cytometry (C). The data were shown from three replicates per treatment. *, p < 0.05, compared to the Mature group. In the Pre group, 3T3-L1 preadipocytes treated without any extract served as the negative control. In the Mature group, 3T3-L1 preadipocytes were induced to become mature adipocytes and were treated without any extract. | |
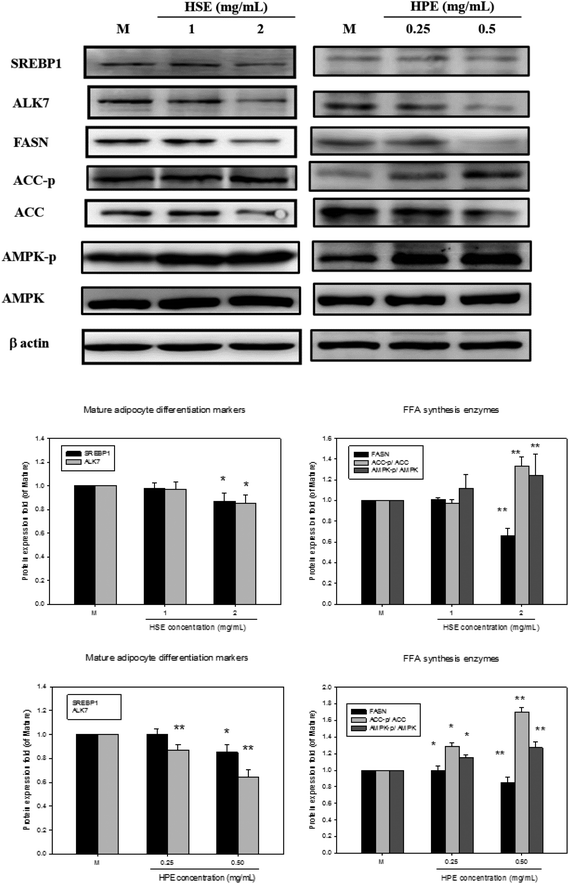 |
| Fig. 5 HSE and HPE inhibited adipocyte differentiation. HSE and HPE reduced the expression of the adipocyte differentiation markers SREBP1 and ALK7. Post-confluent 3T3-L1 pre-adipocytes were treated with HSE or HPE at the indicated concentrations for 14 days. Proteins isolated from the cells were analyzed by western blot using the appropriate antibody. All data are expressed as the fold change relative to the untreated control cells. The data were shown as the means ± SD from three replicates per treatment. In the Mature group, 3T3-L1 preadipocytes were induced to become mature adipocytes and were treated without any extract. | |
3.6. The effect of HSE and HPE on mature adipocytes
Flow cytometry was used to determine the number of apoptotic cells following HSE and HPE treatment. HSE and HPE were found to be effective in reducing the number of mature adipocytes. The percentage of apoptotic cells among the total mature adipocytes increased following treatment with HSE or HPE in a dose dependent manner (Fig. 6). HPE was more efficient in stimulating cell apoptosis of adipocytes. Similar concentrations of HSE and HPE did not affect preadipocyte cell viability.
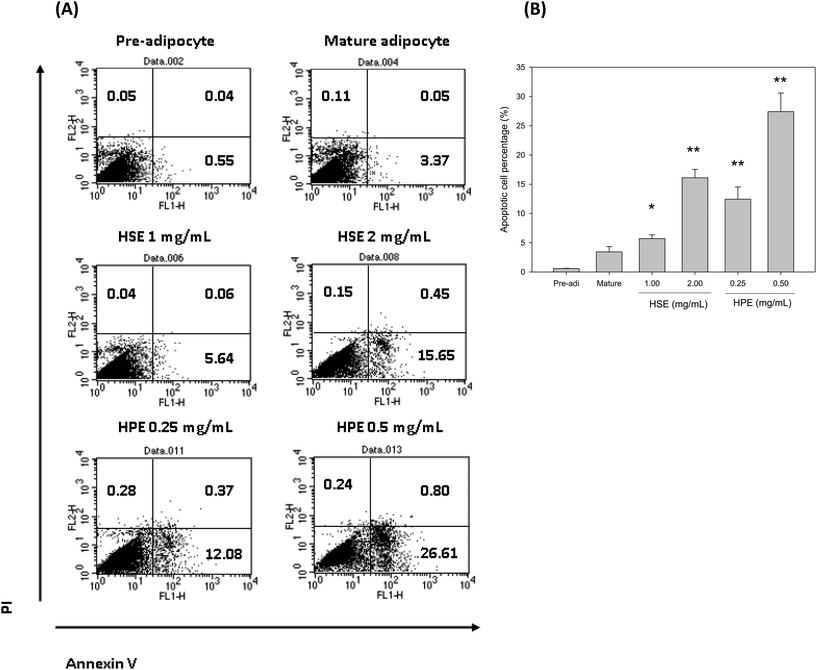 |
| Fig. 6 HSE and HPE induced apoptosis of mature adipocytes. Mature adipocytes were treated with various concentrations of HSE or HPE for 72 h. Then, the cells were stained with Annexin V/PI and analyzed by flow cytometry (A). The lower-right quadrant shows the early apoptotic cells, and the upper-right quadrant shows the late apoptotic cells. Fluorescence was quantified by flow cytometry. The percentage of apoptotic cells is the sum of the early and late apoptotic cells (B). The data shown were from three replicates per treatment. *, p < 0.05, compared with the Mature group. **, p < 0.01, compared with the Mature group. In the Pre group, 3T3-L1 preadipocytes were treated without any extract and served as the negative control. In the Mature group, 3T3-L1 preadipocytes were induced to become mature adipocytes and were treated without any extract. | |
4. Discussion
An accumulation of excess adipose tissue causes obesity and is a major risk factor for type 2 diabetes and cardiovascular disease.26 Adipocytes are the primary sites of energy storage, and an accumulation of triglycerides occurs during nutritional excess. Adipocyte dysfunction is a critical phenomenon that plays an important role in the development and progression of obesity. It is therefore considered to be a risk factor for the development of various diseases, including coronary heart disease, hypertension, type 2 diabetes mellitus, cancer, respiratory complications, and osteoarthritis.1 Recent reports have outlined that administration of an efficient anti-obesity drug may decrease energy/food intake and increase energy expenditure, as well as decrease preadipocyte differentiation and proliferation, decrease lipogenesis and increase lipolysis, and decrease fat oxidation.27
The prevalence of both obesity and type-2 diabetes has increased sharply in Western countries. Therefore, therapeutic strategies for treating these diseases are being actively pursued at various levels. Our results highlight the promising anti-obesity potential of HSE. Specifically, our results show that HSE and its polyphenolic fraction HPE can inhibit the lipid accumulation that results from fat feeding. HSE is already known to be rich in antioxidants such as polyphenolic and flavonoids, which can decrease oxysterols in bile acid metabolism and block lipid accumulation in the liver that results from the bile salt system.28 Therefore, the effect of the HSE could be due to the presence of polyphenols in the HSE, and HSE treatment can thereby effectively decrease the cholesterol in plasma and organs. Daily intake of HSE decreased body weight, BMI and body fat, and reduced abdominal fat distribution in humans.24 The anti-obesity effects of HSE and HPE were also reflected by the effective adipolytic activity demonstrated against post-confluent 3T3-L1 mature adipocytes. Interestingly, neither HSE nor HPE showed any cytotoxic effects against 3T3-L1 preadipocyte cells. However, the anti-obesity effects of HSE are more desirable. An analysis of the molecular markers of fatty acid synthesis and accumulation also reveal that HSE and HPE have similar effects in regulating fatty acid accumulation. Polyphenols are known to activate the AMPK pathway, which mediates FASN and ACC activities29 and regulates fatty acid accumulation. HSE exhibits activities against atherosclerosis, liver disease, cancer, diabetes and metabolic syndromes.30 A comparative analysis of the effects of HSE and HPE further proves that the active metabolites of HSE are also present in HPE. A previous study showed that H. sabdariffa polyphenol-rich extracts (HPE) more effectively decrease plasma cholesterol and LDL-C than crude HSEs.31 Active polyphenols such as protocatechuic acid, GCG, and caffeic acid, which are present in HPE, are known to potentially reduce total cholesterol levels in the plasma in various animal models.25
Additionally, the anti-obesity effects of HSE and its polyphenol content were determined in animal models by analyzing the body weight, fat mass, feed intake, plasma lipid profiles, and biochemical examination of liver and renal function in HFD-fed hamsters. Our results showed that supplementation with either HSE or HPE significantly reduced body weight gain, fat mass, and plasma TG, TC, and LDL cholesterol concentrations. High levels of cholesterol in the plasma are commonly observed in obese patients. In our HFD-fed animal model, not only were the levels of cholesterol elevated, but the levels of TG and LDL were also increased in the plasma.32
Obesity also causes chronic inflammation in the liver, which can result in liver damage.33 Our results showed that a HFD increases ALT and AST levels in the plasma, which indicates a damaged liver in the HFD-fed hamsters.34 To better understand the effects of a HFD on liver function, the enzyme activities of ALT and AST were measured.35 ALT and AST measurements in the HFD-fed hamsters collectively reflected the severity of liver damage caused by the HFD. Both ALT and AST are leakage enzymes, and their elevation in the circulation indicates significant hepatocellular damage. Toxicity, inflammation, hypoxia, and tissue trauma may be the underlying reasons for their elevation.36 Liver abnormalities are often characterized by an increase in the liver weight, an increase in ALT and AST activities, and the occurrence of inflammatory infiltrates in rats. Meanwhile, renal abnormalities are characterized by an increase in the kidney weight and pararenal fat accumulation when a HFD is consumed.35 However, AST is also found in the heart, kidneys, brain, and skeletal muscles and has been used as a nonspecific marker for other organ damage.36,37 Our results reveal that treatment with HSE significantly reduced ALT and AST levels in the circulation. Therefore, HSE ameliorated the potential liver damage caused by the HFD. The reduction of liver enzymes following HSE treatment indicates a decrease in necrosis and fat deposition in liver cells.38
Further analysis shows that the HSE treatment did not cause any marked change in renal functional markers such as BUN and CRE, indicating that HSE administration does not affect the renal function.39 Insulin resistance is usually accompanied by unregulated blood glucose levels, ion levels, and ketone bodies in the plasma, which increase the risk for diabetic ketoacidosis.40 HSE supplementation in HFD-fed hamsters did not change blood glucose levels, ion levels, or ketone body levels in the plasma. Therefore, HSE does not affect insulin function. The polyphenol and flavonoid composition of HSE contributes to its hepatic protective activities and protective effects against hypolipidemia.25,41 Additionally, our previous work showed that HSE effectively reduces serum cholesterol levels in 42 men and women volunteers after 4 weeks of administration.42 A HFD has been known to cause an increase in liver mitochondrial ROS production.43 It is widely recognized that ROS can cause cell damage via the mechanisms involved in lipid peroxidation, which results in tissue injury, particularly in the liver.44 Dietary polyphenols contain a number of phenolic hydroxyl groups and have been shown to have various beneficial effects, which are primarily due to their ROS scavenging activities.45 Polyphenols are widely distributed in vegetables, fruits, and beverages and are present as an integral part of the human diet.46,47 Various polyphenol-rich sources, such as tea, pomegranates, grape juice, apples, and pecan nuts, have demonstrated hepatic protection against many insults.45,47–50 Various herbal extracts, such as the Chrysanthemum morifolium extract, Morinda citrifolia L. extract, and Coix lachryma-jobi L. extract, that are abundant in polyphenols have also shown to provide protection against obesity-related liver damage.51–54
In conclusion, HSE treatment improves HFD-induced obesity and lipid accumulation-induced damage in the liver in an animal model of obesity and inhibits adipogenesis in preadipocytes. The major active components responsible for the anti-obesity effects of HSE are polyphenols; therefore, polyphenolic-rich HPE treatment is a safe therapy for treating HFD-induced obesity and liver disease.
Conflict of interest
The authors declare no competing financial interest.
Acknowledgements
This work was financially supported by Ministry of Science and Technology, Taiwan (MOST 99-2632-B-040-001-MY3) and Chienkuo Technology University, Taiwan (CTU-104-RP-BS-001-016-A.
References
- P. G. Kopelman, Obesity as a medical problem, Nature, 2000, 404, 635–643 CAS.
- S. Yoshimoto, T. M. Loo, K. Atarashi, H. Kanda, S. Sato, S. Oyadomari, Y. Iwakura, K. Oshima, H. Morita, M. Attori, K. Honda, Y. Ishikawa, E. Hara and N. Ohtani, Obesity-induced gut microbial metabolite promotes liver cancer through senescence secretome, Nature, 2013, 499, 97–101 CrossRef CAS PubMed.
- M. M. Avram, A. S. Avram and W. D. James, Subcutaneous fat in normal and diseased states - 3. Adipogenesis: From stem cell to fat cell, J. Am. Acad. Dermatol., 2007, 56, 472–492 CrossRef PubMed.
- T. Tchkonia, D. E. Morbeck, T. von Zglinicki, J. van Deursen, J. Lustgarten, H. Scrable, S. Khosla, M. D. Jensen and J. L. Kirkland, Fat tissue, aging, and cellular senescence, Aging Cell, 2010, 9, 667–684 CrossRef CAS PubMed.
- W. P. Cawthorn, E. L. Scheller and O. A. MacDougald, Adipose tissue stem cells: the great WAT hope, Trends Endocrinol. Metab., 2012, 23, 270–277 CrossRef CAS PubMed.
- S. Cinti, The adipose organ at a glance, Dis. Models & Mech., 2012, 5, 588–594 CAS.
- T. Guo, P. Marmol, A. Moliner, M. Bjornholm, C. Zhang, K. M. Shokat and C. F. Ibanez, Adipocyte ALK7 links nutrient overload to catecholamine resistance in obesity, eLife, 2014, 3, e03245 Search PubMed.
- P. A. Edwards, D. Tabor, H. R. Kast and A. Venkateswaran, Regulation of gene expression by SREBP and SCAP, Biochim. Biophys. Acta, 2000, 1529, 103–113 CrossRef CAS.
- B. H. Choi, I. S. Ahn, Y. H. Kim, J. W. Park, S. Y. Lee, C. K. Hyun and M. S. Do, Berberine reduces the expression of adipogenic enzymes and inflammatory molecules of 3T3-L1 adipocyte, Exp. Mol. Med., 2006, 38, 599–605 CrossRef CAS PubMed.
- A. Ejaz, D. Wu, P. Kwan and M. Meydani, Curcumin inhibits adipogenesis in 3T3-L1 adipocytes and angiogenesis and obesity in C57/BL mice, J. Nutr., 2009, 139, 919–925 CrossRef CAS PubMed.
- W. Shao, Z. Yu, Y. Chiang, Y. Yang, T. Chai, W. Foltz, H. Lu, I. G. Fantus and T. Jin, Curcumin prevents high fat diet induced insulin resistance and obesity via attenuating lipogenesis in liver and inflammatory pathway in adipocytes, PLoS One, 2012, 7, e28784 CAS.
- J. T. Hwang, I. J. Park, J. I. Shin, Y. K. Lee, S. K. Lee, H. W. Baik, J. Ha and O. J. Park, Genistein, EGCG, and capsaicin inhibit adipocyte differentiation process via activating AMP-activated protein kinase, Biochem. Biophys. Res. Commun., 2005, 338, 694–699 CrossRef CAS PubMed.
- H. de Groot and U. Rauen, Tissue injury by reactive oxygen species and the protective effects of flavonoids, Fundam. Clin. Pharmacol., 1998, 12, 249–255 CrossRef CAS PubMed.
- A. R. Tapas, D. M. Sakarkar and R. B. Kakde, Flavonoids as Nutraceuticals: A Review, Trop. J. Pharm. Res., 2008, 7, 1089–1099 Search PubMed.
- S. Zhang, L. Zheng, D. Dong, L. Xu, L. Yin, Y. Qi, X. Han, Y. Lin, K. Liu and J. Peng, Effects of flavonoids from Rosa laevigata Michx fruit against high-fat diet-induced non-alcoholic fatty liver disease in rats, Food Chem., 2013, 141, 2108–2116 CrossRef CAS PubMed.
- C. H. Jung, I. Cho, J. Ahn, T. I. Jeon and T. Y. Ha, Quercetin reduces high-fat diet-induced fat accumulation in the liver by regulating lipid metabolism genes, Phytother. Res., 2013, 27, 139–143 CrossRef CAS PubMed.
- L. X. Yu, H. X. Yan, Q. Liu, W. Yang, H. P. Wu, W. Dong, L. Tang, Y. Lin, Y. Q. He, S. S. Zou, C. Wang, H. L. Zhang, G. W. Cao, M. C. Wu and H. Y. Wang, Endotoxin accumulation prevents carcinogen-induced apoptosis and promotes liver tumorigenesis in rodents, Hepatology, 2010, 52, 1322–1333 CrossRef CAS PubMed.
- C. D. Fernando and P. Soysa, Total phenolic, flavonoid contents, in vitro antioxidant activities and hepatoprotective effect of aqueous leaf extract of Atalantia ceylanica, BMC Complementary Altern. Med., 2014, 14, 395 CrossRef PubMed.
- A. Herrera-Arellano, S. Flores-Romero, M. A. Chavez-Soto and J. Tortoriello, Effectiveness and tolerability of a standardized extract from Hibiscus sabdariffa in patients with mild to moderate hypertension: a controlled and randomized clinical trial, Phytomedicine, 2004, 11, 375–382 CrossRef CAS PubMed.
- B. H. Ali, H. M. Mousa and S. El-Mougy, The effect of a water extract and anthocyanins of hibiscus sabdariffa L on paracetamol-induced hepatoxicity in rats, Phytother. Res., 2003, 17, 56–59 CrossRef CAS PubMed.
- H. H. Lin, H. P. Huang, C. C. Huang, J. H. Chen and C. J. Wang, Hibiscus polyphenol-rich extract induces apoptosis in human gastric carcinoma cells via p53 phosphorylation and p38 MAPK/FasL cascade pathway, Mol. Carcinogen, 2005, 43, 86–99 CrossRef CAS PubMed.
- C. C. Chen, J. D. Hsu, S. F. Wang, H. C. Chiang, M. Y. Yang, E. S. Kao, Y. C. Ho and C. J. Wang, Hibiscus sabdariffa extract inhibits the development of atherosclerosis in cholesterol-fed rabbits, J. Agric. Food Chem., 2003, 51, 5472–5477 CrossRef CAS PubMed.
- C. H. Peng, C. C. Chyau, K. C. Chan, T. H. Chan, C. J. Wang and C. N. Huang, Hibiscus sabdariffa polyphenolic extract inhibits hyperglycemia, hyperlipidemia, and glycation-oxidative stress while improving insulin resistance, J. Agric. Food Chem., 2011, 59, 9901–9909 CrossRef CAS PubMed.
- H. C. Chang, C. H. Peng, D. M. Yeh, E. S. Kao and C. J. Wang, Hibiscus sabdariffa extract inhibits obesity and fat accumulation, and improves liver steatosis in humans, Food Funct., 2014, 5, 734–739 CAS.
- M. Y. Yang, C. H. Peng, K. C. Chan, Y. S. Yang, C. N. Huang and C. J. Wang, The hypolipidemic effect of Hibiscus sabdariffa polyphenols via inhibiting lipogenesis and promoting hepatic lipid clearance, J. Agric. Food Chem., 2010, 58, 850–859 CrossRef CAS PubMed.
- A. Must, J. Spadano, E. H. Coakley, A. E. Field, G. M. Colditz and W. H. Dietz, The disease burden associated with overweight and obesity, JAMA, J. Am. Med. Assoc., 1999, 282, 1523–1529 CrossRef CAS PubMed.
- Y. W. Wang and P. J. H. Jones, Conjugated linoleic acid and obesity control: efficacy and mechanisms, Int. J. Obes., 2004, 28, 941–955 CrossRef CAS PubMed.
- A. Crosignani, M. Zuin, M. M. Allocca and M. Del Puppo, Oxysterols in bile acid metabolism, Clin. Chim. Acta, 2011, 412, 2037–2045 CrossRef CAS PubMed.
- C. H. Wu, M. Y. Yang, K. C. Chan, P. J. Chung, T. T. Ou and C. J. Wang, Improvement in high-fat diet-induced obesity and body fat accumulation by a Nelumbo nucifera leaf flavonoid-rich extract in mice, J. Agric. Food Chem., 2010, 58, 7075–7081 CrossRef CAS PubMed.
- H. H. Lin, J. H. Chen and C. J. Wang, Chemopreventive Properties and Molecular Mechanisms of the Bioactive Compounds in Hibiscus Sabdariffa Linne, Curr. Med. Chem., 2011, 18, 1245–1254 CrossRef CAS.
- L. C. Liu, C. J. Wang, C. C. Lee, S. C. Su, H. L. Chen, J. D. Hsu and H. J. Lee, Aqueous extract of Hibiscus sabdariffa L. decelerates acetaminophen-induced acute liver damage by reducing cell death and oxidative stress in mouse experimental models, J. Sci. Food Agric., 2010, 90, 329–337 CrossRef CAS PubMed.
- T. Obama, S. Nagaoka, K. Akagi, R. Kato, N. Horiuchi, Y. Horai, T. Aiuchi, S. Arata, T. Yamaguchi, M. Watanabe and H. Itabe, Dietary Cholesterol Reduces Plasma Triacylglycerol in Apolipoprotein E-Null Mice: Suppression of Lipin-1 and-2 in the Glycerol-3-Phosphate Pathway, PLoS One, 2011, 6, e22917 CAS.
- N. Lanthier, O. Molendi-Coste, P. D. Cani, N. van Rooijen, Y. Horsmans and I. A. Leclercq, Kupffer cell depletion prevents but has no therapeutic effect on metabolic and inflammatory changes induced by a high-fat diet, FASEB J., 2011, 25, 4301–4311 CrossRef CAS PubMed.
- Y. Jiang, M. Y. Zhao and W. An, Increased hepatic apoptosis in high-fat diet-induced NASH in rats may be associated with downregulation of hepatic stimulator substance, J. Mol. Med., 2011, 89, 1207–1217 CrossRef CAS PubMed.
- U. G. M. de Castro, R. A. S. dos Santos, M. E. Silva, W. G. de Lima, M. J. Campagnole-Santos and A. C. Alzamora, Age-dependent effect of high-fructose and high-fat diets on lipid metabolism and lipid accumulation in liver and kidney of rats, Lipids Health Dis., 2013, 12, 136 CrossRef PubMed.
- S. E. Chapman and R. A. Hostutler, A laboratory diagnostic approach to hepatobiliary disease in small animals, Vet. Clin. N. Am-Small, 2013, 43, 1209–1225 CrossRef PubMed.
- N. S. Ramli, L. Brown, P. Ismail and A. Rahmat, Effects of red pitaya juice supplementation on cardiovascular and hepatic changes in high-carbohydrate, high-fat diet-induced metabolic syndrome rats, BMC Complement. Altern. Med., 2014, 14, 189 CrossRef PubMed.
- L. L. Wang, X. J. Meng and F. Q. Zhang, Raspberry Ketone Protects Rats Fed High-Fat Diets Against Nonalcoholic Steatohepatitis, J. Med. Food, 2012, 15, 495–503 CrossRef CAS PubMed.
- M. Ostermann, Diagnosis of acute kidney injury: Kidney Disease Improving Global Outcomes criteria and beyond, Curr. Opin. Crit. Care, 2014, 20, 581–587 CrossRef PubMed.
- N. S. Ramli, L. Brown, P. Ismail and A. Rahmat, Effects of red pitaya juice supplementation on cardiovascular and hepatic changes in high-carbohydrate, high-fat diet-induced metabolic syndrome rats, BMC Complement. Altern. Med., 2014, 14, 189 CrossRef PubMed.
- M. J. Yoon, G. Y. Lee, J. J. Chung, Y. H. Ahn, S. H. Hong and J. B. Kim, Adiponectin increases fatty acid oxidation in skeletal muscle cells by sequential activation of AMP-activated protein kinase, p38 mitogen-activated protein kinase, and peroxisome proliferator-activated receptor alpha, Diabetes, 2006, 55, 2562–2570 CrossRef CAS PubMed.
- T. L. Lin, H. H. Lin, C. C. Chen, M. C. Lin, M. C. Chou and C. J. Wang, Hibiscus sabdariffa extract reduces serum cholesterol in men and women, Nutr. Res., 2007, 27, 140–145 CrossRef CAS PubMed.
- G. Vial, H. Dubouchaud, K. Couturier, C. Cottet-Rousselle, N. Taleux, A. Athias, A. Galinier, L. Casteilla and X. M. Leverve, Effects of a high-fat diet on energy metabolism and ROS production in rat liver, J. Hepatol., 2011, 54, 348–356 CrossRef CAS PubMed.
- L. Tian, X. Shi, L. Yu, J. Zhu, R. Ma and X. Yang, Chemical composition and hepatoprotective effects of polyphenol-rich extract from Houttuynia cordata tea, J. Agric. Food Chem., 2012, 60, 4641–4648 CrossRef CAS PubMed.
- Y. Cui, X. Yang, X. Lu, J. Chen and Y. Zhao, Protective effects of polyphenols-enriched extract from Huangshan Maofeng green tea against CCl4-induced liver injury in mice, Chem.-Biol. Interact., 2014, 220C, 75–83 CrossRef PubMed.
- N. P. Seeram, M. Aviram, Y. Zhang, S. M. Henning, L. Feng, M. Dreher and D. Heber, Comparison of antioxidant potency of commonly consumed polyphenol-rich beverages in the United States, J. Agric. Food Chem., 2008, 56, 1415–1422 CrossRef CAS PubMed.
- S. Mukherjee, S. Ghosh, S. M. Choudhury, A. Adhikary, K. Manna, S. Dey, G. Sa, T. Das and S. Chattopadhyay, Pomegranate reverses methotrexate-induced oxidative stress and apoptosis in hepatocytes by modulating Nrf2-NF-kappaB pathways, J. Nutr. Biochem., 2013, 24, 2040–2050 CrossRef CAS PubMed.
- O. Aprikian, J. Busserolles, C. Manach, A. Mazur, C. Morand, M. J. Davicco, C. Besson, Y. Rayssiguier, C. Remesy and C. Demigne, Lyophilized apple counteracts the development of hypercholesterolemia, oxidative stress, and renal dysfunction in obese Zucker rats, J. Nutr., 2002, 132, 1969–1976 CAS.
- A. D. Rodrigues, T. B. Scheffel, G. Scola, M. T. Dos Santos, B. Fank, C. Dani, R. Vanderlinde, J. A. Henriques, A. S. Coitinho and M. Salvador, Purple grape juices prevent pentylenetetrazol-induced oxidative damage in the liver and serum of Wistar rats, Nutr. Res., 2013, 33, 120–125 CrossRef CAS PubMed.
- D. Heber, Y. Zhang, J. Yang, J. E. Ma, S. M. Henning and Z. Li, Green tea, black tea, and oolong tea polyphenols reduce visceral fat and inflammation in mice fed high-fat, high-sucrose obesogenic diets, J. Nutr., 2014, 144, 1385–1393 CrossRef CAS PubMed.
- Y. Cui, X. L. Wang, J. Xue, J. Y. Liu and M. L. Xie, Chrysanthemum morifolium extract attenuates high-fat milk-induced fatty liver through peroxisome proliferator-activated receptor alpha-mediated mechanism in mice, Nutr. Res., 2014, 34, 268–275 CrossRef CAS PubMed.
- Y. L. Lin, Y. Y. Chang, D. J. Yang, B. S. Tzang and Y. C. Chen, Beneficial effects of noni (Morinda citrifolia L.) juice on livers of high-fat dietary hamsters, Food Chem., 2013, 140, 31–38 CrossRef CAS PubMed.
- L. F. Wang, J. Sun, Q. D. Yi, X. F. Wang and X. R. Ju, Protective Effect of Polyphenols Extract of Adlay (Coix lachryma-jobi L. var. ma-yuen Stapf) on Hypercholesterolemia-Induced Oxidative Stress in Rats, Molecules, 2012, 17, 8886–8897 CrossRef CAS PubMed.
- Y. P. Zou, Y. H. Lu and D. Z. Wei, Hypocholesterolemic effects of a flavonoid-rich extract of Hypericum perforatum L. in rats fed a cholesterol-rich diet, J. Agric. Food Chem., 2005, 53, 2462–2466 CrossRef CAS PubMed.
Footnotes |
† These authors contributed equally to this work and therefore share first authorship. |
‡ Present address: no.110, Sec. 1, Jianguo N. Rd., South District, Taichung, Taiwan 402. |
|
This journal is © The Royal Society of Chemistry 2016 |
Click here to see how this site uses Cookies. View our privacy policy here.