DOI:
10.1039/D3SC03909A
(Edge Article)
Chem. Sci., 2024,
15, 2443-2455
New sustainable polymers and oligomers for Cultural Heritage conservation†
Received
28th July 2023
, Accepted 9th January 2024
First published on 17th January 2024
Abstract
The development of “green” chemistry materials with enhanced properties is a central topic in numerous applicative fields, including the design of polymeric systems for the conservation of works of art. Traditional approaches in art restoration comprise polymer thickeners and viscous dispersions to partially control solvents in the removal of soil or aged varnishes/coatings from artifacts. Alternatively, polymeric gel networks can be specifically designed to grant full control of the cleaning action, yielding safe, time- and cost-effective restorations. The selection of polymers and oligomers in gel design is crucial to tune solvent upload, retention, and controlled release over the sensitive artistic surfaces. Starting from an overview of traditional polymer formulations and state-of-the-art gel systems for cleaning works of art, we provide here the design of a new class of gels, focusing on the selection of oligomers to achieve gels with tailored hydrophilicity/hydrophobicity. We evaluated the oligomers Hydrophilic–Lipophilic Balance (HLB) by developing, for the first time, a novel methodology combining SEC and DOSY NMR analysis, which was tested on a library of “green” oligoesters synthesized by polycondensation and poorly explored in the literature. Oligomers with moderate polydispersity were chosen to validate the new protocol as a robust tool for designing polymeric gels even on industrial scale. The methodology is more time-effective than traditional methods, and gives additional insights on the oligomers physico-chemical nature, evaluating their compatibility with different solvents. Then, we used the selected oligoesters with castor oil to obtain a new class of organogels able to upload solvents with varying polarity, which effectively removed different types of unwanted layers typically found in painting restoration. These results validate the oligomers screening approach and the new class of gels as promising chemical processes/materials in art preservation. The methodology can potentially allow evaluation of HLB also for small molecules (e.g., surfactants), opening for the formulation of polymers solutions/gels beyond Cultural Heritage conservation, as in pharmaceutics, cosmetics, food industry, tissue engineering, agriculture, and others.
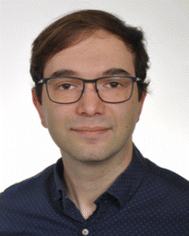 Damiano Bandelli | Damiano Bandelli received his Master's Degree at the University of Florence, Italy, in 2014 and obtained his Ph.D. degree in Macromolecular and Organic Chemistry at the Friedrich-Schiller University in Jena, Germany, in 2022. In 2020 he joined the CSGI centre in Florence. He is currently a researcher at the University of Florence, Department of Chemistry, in the field of Chemistry for Cultural Heritage preservation. He has been involved in European and national projects in the fields of pharmaceutics and Cultural Heritage preservation. His main research interest is in the development of tailor-made polymeric materials for advanced applications in conservation science. |
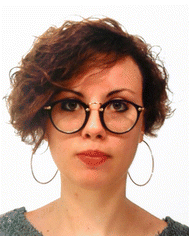 Rosangela Mastrangelo | Rosangela Mastrangelo received her Master's Degree at the University of Florence, Italy, in 2016 and pursued a Ph.D. in Chemistry – Science for Cultural Heritage Conservation in 2021. She is currently a researcher at the University of Florence (Department of Chemistry and CSGI). She joined National and International Projects, involving the collaboration with international companies and research centres for the formulation of innovative materials, in the field of Cultural Heritage conservation and cosmetics. Her research is mainly focused on gels for the cleaning of painted surfaces and the relevant physico-chemical aspects involved in the cleaning mechanism. |
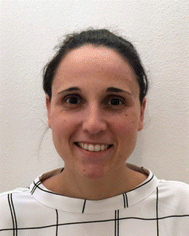 Giovanna Poggi | Giovanna Poggi holds a Ph.D. in Science for Cultural Heritage Conservation from the University of Florence under the mentorship of Prof. Piero Baglioni. She worked as a postdoctoral fellow at National Center for Colloids and Nanosciences (CSGI) in the framework of the European Projects FP7 NANOFORART, H202 NANORESTART, H2020 APACHE and ERC GraphenART. She is currently a fixed-term researcher in physical chemistry at the Chemistry Department of the University of Florence. Her research mainly deals with the synthesis and characterization of nanoparticles and their application for paper and wood deacidification and for carbonate-based matrices consolidation. Recently, she has started working on the development and characterization of hydrogels and organogels for the cleaning of works of art and on the development and use of biocompatible polymers in several applicative fields. |
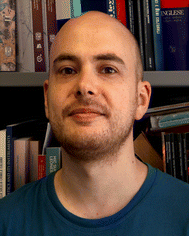 David Chelazzi | David Chelazzi received his Master's Degree in Chemistry from the University of Florence (Italy) in 2003 and obtained his Ph.D. degree in Science for Cultural Heritage Conservation in 2007. He is currently a researcher at the University of Florence (Department of Chemistry and CSGI). His main research interest is in advanced and nanomaterials for the conservation of works of art. He is involved in European and national projects on the development of advanced materials and solutions for the preservation of Cultural Heritage (FP7, H2020, HORIZON EUROPE). He is author and co-author of more than 95 papers in the field of physical-chemistry, conservation science, and environmental science. |
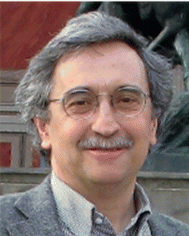 Piero Baglioni | Piero Baglioni is Emeritus Professor of Physical Chemistry in the Department of Chemistry at the University of Florence. He is on the editorial/advisory boards of several international journals and a member of the scientific board of several national and international institutions and societies. He is the author of more than 550 publications and 27 patents in the field of colloids and interfaces, and a pioneer in the application of soft matter to the conservation of Cultural Heritage. |
Introduction
Sustainability is a key concept in today's society, and “green” chemical processes are being adopted in different sectors looking for efficient, safe materials and reactions with reduced ecotoxicological impact and decreased waste.1 In general, sustainability is implemented by minimizing the raw materials needed in processes, reducing waste or hazardous products, and employing as much as possible low-energy pathways and renewable sources to foster cost-effective, circular economy approaches.2–7
These trends are becoming progressively adopted also in the field of Cultural Heritage preservation, which gathers scientists and industrial actors along with end-users (art curators, conservators, museums, collections, etc.) and social science and humanities. Cultural Heritage, when well maintained and accessible to citizens, can act as a driver of socioeconomic welfare, but there is the urgent necessity of implementing “green” materials and sustainable methodologies to be used for remedial and preventive conservation8–12 of movable and immovable works of art. Indeed, even though it does not involve large production volumes as compared to other sectors, Cultural Heritage preservation is a field with high social impact, and part of a framework aimed at providing resilience to society, inspiring sustainable practices. In addition, art preservation is carried out by professionals (restorers, curators) who are exposed to safety risks when traditional chemicals are used to restore the artefacts, e.g., some petroleum-based solvents, siloxanes, or cocoamine surfactants.13 Finally, the innovative green solutions devised for art conservation in the framework of materials science and colloids, such as gels, films, foams, emulsions, particles, and composites, can be useful to other industrial/technological sectors where the employment of green chemistry solutions is strictly required, e.g., detergency, cosmetics, pharmaceutics, food industry, packaging, coatings, etc.
Advanced restoration materials have included, over the decades, inorganic nanoparticles,14–17 hybrid systems,18–21 microemulsions and gels,13,22 protective coatings,23–25 and films.26–28 Such diversity responds to the complexity of physico-chemical processes and interactions at play in the artifacts' degradation processes that must be counteracted. While some of these advanced materials have already acceptable sustainability, there is wide room, and need, for their conversion into fully “green” approaches that address, as much as possible, all the requirements of the European Green Deal.2,3,29 This is not a straightforward task, considering that advanced remedial and preventive conservation tools are often derived in the framework of materials science, colloids, and soft matter,30–32 where subtle changes in compositions or formulation processes can produce structural or functional alterations of the final products. Therefore, the chemical design of new “green” solutions must be carefully carried out taking into accounts different factors.
Because polymers and oligomers are central in the formulation of advanced solutions for Cultural Heritage preservation, it is fundamental to highlight sustainable raw materials, chemical processes, and applicative protocols. In particular, a recurrent and delicate task in remedial conservation is the cleaning of artifacts, i.e., the removal of unwanted layers such as soil, dust, and aged varnishes or coatings from past restorations. Most artistic surfaces, like iconic modern/contemporary canvas paintings, can be highly sensitive to water and solvents,33–35 thus cleaning tools must be selective, removing target layers without affecting the original binders, pigments, dyes, etc. Polymers have been widely employed to devise confining networks to upload solvents and control their release on works of art. Namely, chemical and physical polymeric gels allow high cleaning effectiveness and safe applications, surpassing the limitations of traditional solvent thickeners that grant only limited control of the cleaning action or tend to leave residues on treated surfaces.13,36 However, while several formulations of hydrogels (able to upload aqueous fluids) have already passed from design to extensive assessment, and are now available to conservators worldwide,37–40 the development of polymer gel networks to confine organic solvents (organogels) is still in its infancy in conservation science.41–46 This open task must be addressed, and new robust systems proposed, since conservators still rely on organic solvents as complementary tools when artistic surfaces do not tolerate even the minimal contact with aqueous phases.
Starting from a critical overview of traditional polymer formulations and state-of-the-art gel systems for cleaning works of art, we report in this contribution on the design of a new class of organogels, which started from the selection of specific oligoesters in view of building networks with tailored hydrophilicity/hydrophobicity to clean works of art. These oligomers are “green” compounds that have been poorly explored in the literature but have great potential in gel synthesis. Their Hydrophilic–Lipophilic Balance (HLB) was here evaluated by developing, for the first time, a novel methodology that combines Size Exclusion Chromatography (SEC) and diffusion-ordered spectroscopy (DOSY) NMR analysis. The new method is more time-effective than traditional ones and provides additional insights on the physico-chemical nature of the oligomers, evaluating their compatibility with different solvents. Such information can in principle guide phase separations in pre-gel oligomers' solutions and during gelation processes. Oligomers with moderate polydispersity were selected to validate the new protocol as a robust approach in the design of polymeric gels even on industrial scale. Then, the oligoesters were reacted with castor oil, a valuable renewable resource in chemical industry47 but still almost unexplored in art conservation,21,43,48 to yield a new class of organogels. In particular, the HLB evaluation protocol that we developed grants the possibility of introducing alternated domains with varying hydrophobicity/hydrophilicity in the castor oil polyurethane network, producing a completely new class of gels as compared to standard polymer networks with a homogeneous hydrophobic phase. One of the practical advantages of this new feature is the enhanced capability to upload solvents with varying polarity, simply by switching the oligomer inserted in the castor oil network, which is expected to make the new gels highly versatile tools in art cleaning interventions.
The gels were assessed on two different types of unwanted layers found in painting restoration, i.e., a synthetic polymer varnish and wax. Our final goal was to validate the oligomers screening approach and the new class of castor oil–oligoester gels as novel promising chemical processes/materials in art preservation. In addition, the methodology could potentially allow the evaluation of HLB for small molecules (e.g., surfactants), overall supporting the formulation of polymers solutions and gels in fields even beyond Cultural Heritage conservation, such as pharmaceutics, cosmetics, food industry, tissue engineering, agriculture, and others.
Polymers solutions and gels in art preservation – an overview
Starting from the postwar boom, polymers have permeated through all the range of mankind activities, and many different classes of polymeric compounds have been continuously developed and applied,49 including functional polymers from the 1960s on.50 Following these trends, synthetic polymers were enthusiastically adopted also in the restoration of Cultural Heritage51 (besides their direct use as artistic materials),52,53 where they added to the vast plethora of natural materials used for centuries, as glues/adhesives or consolidants, fixatives, varnishes, and protective coatings. The widespread use of polymeric materials in Cultural Heritage restoration was fostered by their advantageous practical features, such as availability, low cost, ease of application, high adhesive power, and short-term appealing effects like colour saturation when applied on painted surfaces. However, scientific awareness and rigorous design of restoration materials came only later, beginning around the end of the 1980s,54 to replace serendipity and trial-and-error in the common practice. In fact, the diffusion of synthetic polymers was not well supported by full awareness of their physico-chemical transformations under the action of UV-Vis light, temperature, relative humidity, and pollutants, which promote numerous degradation pathways of works of art.
A first bulk of synthetic formulations, applied either as solutions or aqueous emulsions, comprises polyester, ketone, epoxy or silicone resins, siloxanes, polyolefins, polyamides, as well as acrylics or vinyl polymers, largely used as consolidants or water repellents since the 1970s on different types of works of art.23,55 Products like Paraloid B72, polyvinyl acetate, and Primal AC-33, have been, and still are, extensively applied on wall paintings, stone, metal, and glass. However, these polymers are degraded by photo-oxidation processes promoted by light and enhanced by temperature and pollutants, which can cause yellowing, cracking, change in molecular weight/solubility, and loss of adhesive power of the coatings.23,56 Moreover, these products can also be attacked by microorganisms, contrary to what was initially believed,57,58 or degrade emitting volatile acids.59 Finally, polymer coatings typically block the pores at the surface of wall paintings, causing salt solutions, coming from the wall matrix or the ground, to crystallize in constrained spaces at the mural-coating interface. The resulting high pressure disrupts the mural surface layers and the coating, leading to significant degradation. All these alterations can jeopardize the surface of the treated works of art and pose significant risks to their maintenance. In response, several advanced, nanostructured cleaning fluids have been developed starting from a pioneering application in the 1980s,60e.g., surfactant micelles and oil-in-water (o/w) microemulsions that are able to swell, detach or dewet aged polymer coatings from artistic surfaces,13,22,30,32 like canvas or mural paintings, stone, paper, wood, and other substrates. It has been shown that the combination, in the nanostructured cleaning fluids, of surfactants and good solvents for the polymer coating, is pivotal to promote chain mobility and the formation of interfaces, which overall boosts the kinetics of dewetting processes.61,62 This leads to enhanced efficacy and selectivity in the removal of unwanted layers from works of art.
Parallel to the development of the nanostructured cleaning fluids, however, research has focused on the design of polymer networks able to confine the fluids, as well as simple aqueous solutions or solvent blends, granting controlled release on water- or solvent-sensitive surfaces that are frequently met in art conservation.13,22,54,63 This is a crucial task that has led to the development of different families of polymer formulations. Traditionally, polyacrylic acid (PAA)54 and cellulose ethers64 have been employed to thicken solvents into viscous dispersions that grant only partial release control in the removal of aged varnishes from paintings. PAA was combined with cocoamine surfactants in the so-called “solvent gels”, polymer dispersions that can thicken a vast range of solvents.54,65 Even though they are versatile systems, these thickeners have poor retentiveness while leaving polymer or surfactant residues on the treated surfaces, and thus requiring clearing steps that can be invasive to the paintings' original layers.36
Alternatively, polyacrylamide,66 poly(2-hydroxyethyl methacrylate) (p(HEMA)), poly(vinylpyrrolidone) (PVP), and polyvinyl alcohol (PVA), have been used to formulate hydrogels to clean works of art. For instance, HEMA can be crosslinked into pHEMA networks in the presence of linear PVP, yielding semi-interpenetrated gel networks (SIPNs) that combine the mechanical properties of pHEMA with the high hydrophilicity of PVP, i.e., hydrogels with high viscoelasticity and water retentiveness.67 These SIPNs have been used to safely clean water-sensitive substrates like watercolours or poorly bound painted layers,67,68 improving on traditional gellan or agar gels, or even to remove scotch tape adhesives from inked paper,69 or graffiti70 and vandalism from murals, without affecting the original painted layers. PVA has been recently used to formulate “twin-chain” polymer networks (TC-PNs), where one type of PVA (“H-PVA”, with higher molecular weight and hydrolysis degree) is blended with an “L-PVA” (lower molecular weight and hydrolysis degree) before gelation.37 Phase separation and demixing in the pre-gel blend leads to the formation of L-PVA blobs in a continuous phase of H-PVA. When the demixed blend is gelled by freeze-thawing (FT), the blobs deform and partially merge. The following washing procedure causes the partial release of L-PVA, leaving a spongy, disordered and interconnected porous network, as opposed to the aligned, stacked channel-like pores obtained by FT solutions of H-PVA. Some of the L-PVA is retained in the pores' walls, acting as a plasticizer, and making the network compliant to mechanical stress. As a result, the TC-PNs are able to adapt to rough painted surfaces much better than the pHEMA/PVP SIPNs or rigid gellan sheets.37 In addition, the disordered, interconnected network likely favours dirt pick-up and its capture into the gel. These features have made the TC-PNs ideal tools for the cleaning of masterpieces by Pablo Picasso,38 Jackson Pollock,37 Roy Lichtenstein,39 and others. Both the pHEMA/PVP SIPNs and the PVA TC-PNs have been loaded with aqueous solutions or o/w microemulsions and widely used in restoration interventions, recently becoming standard polymer hydrogels for art cleaning. PVA has also been used with borax to formulate highly viscous polymer dispersions that can load some organic solvents in addition to aqueous systems,71–73 or combined with a polyamine chelator to obtain dispersions able to film on bronze sculptures, solubilize and extract copper corrosion products, and then be peeled off the surface.74
While these hydrogels and highly viscous dispersions overcome the limitations of traditional thickeners, there is still the need to design organogels, i.e., polymer networks able to confine organic solvents as complementary cleaning tools to aqueous systems. Poly ethyl or methyl acrylate organogels (PEMA, PMMA) have showed promising results in the removal of adhesives or aged varnishes from artworks,41,42,75,76 but currently the focus is on bio- or natural polymers, derived from non-petrochemical feedstocks, and renewable sources to formulate gels coping with the requirements of the European Green Deal and sustainability.12,77 Accordingly, organogels have been recently formulated using either polyhydroxy butyrate,9 thiol-ene photopolymerization,45 or castor oil,43 while PVA has been combined with starch to yield hydrogels with similar cleaning capability to the TC-PNs but enhanced “green” character.10 In addition, pectin, an ionic biopolymer from renewable resources, has been used as a thickener to stabilize Pickering emulsions made by halloysite nanotubes, formulated to clean marble.11
Castor oil, in particular, is a renewable resource that, being nonedible, does not impact on the food chain, and has large potential in the formulation of organogels for Cultural Heritage preservation or adhesives.43,78 The following sections will be dedicated to the design of a new class of castor oil–oligoester organogels, reported in this contribution for the first time.
Finally, it is worth mentioning the use of polymers in advanced coating formulations for the protection or consolidation of works of art. Even in this case, while several synthetic polymers have been proposed in the last decades to formulate hybrid superhydrophobic and other advanced protective coatings,23,32 there is a shift towards the use of biopolymers and sustainable formulations. Examples include polylactic acid,23 chitosan,8,23 starch,79 and even geopolymers.20
Overall, these reports show how the formulation of polymer systems for the preservation of Cultural Heritage is a vibrant field, where the need for time- and cost-effective tools joins with recent sustainability requirements to create open challenges that need novel solutions, possibly linking with transversal industrial and technological sectors.
Design of a new class of organogels
Nowadays, new challenges related to climate changes demand for “greener” strategies in materials design.3 To this purpose, the full understanding of structure–property relationships in novel materials is crucial. In particular, the hydrophilic lipophilic balance (HLB) of polymeric compounds is a fundamental parameter connected to their degradation, solvent compatibility and processability.80–83 However, despite its importance, there are no protocols enabling its clear evaluation for hydrophobic polymers, while it has been reported for polymeric nanoparticles.84–86 In particular, a crucial issue aspect involves the effect on HLB of variations in chemical composition and molar mass.
The octanol/water partition coefficient (log
P) is commonly employed for HLB evaluation, and can be experimentally obtained or calculated (“c
log
P”) for small organic molecules and nonpolar surfactants.87 However, its evaluation for hydrophobic macromolecules is complex, and, to the best of our knowledge, there is no protocol available. In this sense, gaining in-depth information on polymer morphological features and solution dynamics could be beneficial. Separation techniques such as size exclusion chromatography (SEC) do not only provide molar mass estimation, but, when a viscosimeter detector is employed, they also enable the estimation of macromolecular morphology, which is related to polymer–solvent interactions.88,89 As a complementary tool, diffusion ordered spectroscopy nuclear magnetic resonance (DOSY NMR) could also be employed to evaluate the dynamics of polymer solution and estimate molar mass.90,91
Herein, we demonstrate the evaluation of log
P for a library of oligoesters by combining SEC and DOSY NMR analysis. Aiming at characterizing oligomeric species with similar molar mass, an easily accessible polycondensation reaction of selected dicarboxylic acid and diols was performed. To this aim, the selected reactants (sebacic, adipic and succinic acid as well as hexane- and butanediol) can be produced avoiding the use of petrochemical resources and, according to the European Chemical Agency, these compounds are commonly employed in fertilizers, cleaning products and pharmaceuticals. No solvents nor catalysts were employed during the oligoester synthesis.
The synthetic pathway enabled to vary repetition units and screen a wide range of chemical composition. The final materials were preliminarily analysed by means of SEC to evaluate their molar mass and their morphology in solution, according to absolute multiangle light scattering-refractive index (MALS-RI) analysis and universal calibration. Subsequently, DOSY NMR analysis was performed with a selection of six deuterated solvents with varied log
P to determine the oligoesters' diffusion coefficient and hydrodynamic radii.
Finally, to provide a proof of concept of the devised approach, the analysed oligoesters were used to formulate a new class of castor oil–polyurethane organogels. The gels' swelling capability was tested in solvents commonly employed in Cultural Heritage practices, and the swollen organogels were then used to remove wax or an acrylate copolymer coating from model surfaces, mimicking art cleaning interventions.
Development of a robust protocol for the log
P estimation of hydrophobic oligomers and polymers
Our first goal was to screen a library of oligoesters featuring similar molar masses but varied chemical composition. Therefore, a two-step polycondensation reaction of selected dicarboxylic acids (sebacic acid, adipic acid or succinic acid) and diols (hexanediol or butanediol) was performed. Despite being usually conducted at temperatures above 200 °C with the addition of metal catalyst, a polycondensation reaction can be performed at lower temperatures if oligomeric species are wanted.92,93 To this aim, equimolar amounts of the selected dicarboxylic acid and diol were reacted at 180 °C to obtain the oligomeric species named as O1 to O5 (Table 1, see ESI†).
Table 1 Selected structural characterization data of the synthesized oligoesters
Code |
Repeating unit |
M
n,MALS
[kg mol−1] |
Đ
MALS
|
M
n,univ. cal.
[kg mol−1] |
Đ
univ. cal.
|
R
h
[nm] |
[η]d [mL g−1] |
α
|
c log Pf |
Number-average molar mass and dispersity index obtained with MALS-RI detection.
Number-average molar mass and dispersity index obtained with absolute calibration (Viscosimeter-RI detection).
Hydrodynamic radius obtained from SEC-Viscosimeter analysis. For all samples the error was found to be ±0.01 nm.
Intrinsic viscosity obtained from SEC-Viscosimeter analysis. For all samples the error lied between ±0.05 and 0.06 mL g−1.
Parameter α of Mark–Houwink obtained according to MALS estimation of molar masses. For all samples the error was found to be ±0.001.
Calculated partition coefficient for the meric structure of each oligoester, O1 to O5.
|
O1 |
Hexylene sebaceate |
3.0 |
1.36 |
2.8 |
1.79 |
1.42 |
6.22 |
0.750 |
4.57 |
O2 |
Hexylene adipate |
2.8 |
1.48 |
2.8 |
1.66 |
1.32 |
5.47 |
0.685 |
2.75 |
O3 |
Hexylene succinate |
3.0 |
1.42 |
2.9 |
1.78 |
1.30 |
4.93 |
0.649 |
1.84 |
O4 |
Butylene sebaceate |
3.0 |
1.37 |
2.9 |
1.54 |
1.35 |
5.54 |
0.720 |
3.66 |
O5 |
Butylene adipate |
2.8 |
1.36 |
2.8 |
1.72 |
1.29 |
4.94 |
0.654 |
1.84 |
The oligoesters were preliminarily analysed by means of size exclusion chromatography (SEC) in tetrahydrofuran (THF) eluent employing a system equipped with triple detection (MALS-Viscosimeter-RI) that enabled the calculations of molar masses according to both light scattering and universal calibration approaches.
Independently on the method adopted, similar values of number-average molar mass (Mn, ca. 3 kg mol−1) were obtained (Table 1). In contrast, molar mass dispersity obtained from MALS were lower in comparison to universal calibration due to the MALS overcounting of larger molar masses, making universal calibration data more reliable. Interestingly, overlaying the chromatograms for the series O1 to O5 revealed a slight shift of the elution volume (Fig. 1). Since SEC relies on the volumetric separation of oligo- and polymeric species, the chromatograms of samples with similar molar mass would differentiate if solvation were different, resulting in a slight variation of polymer–solvent interactions. Because the aliphatic oligoesters differ in the mer's (monomeric unit) chemical composition, a variation in solvation properties could be ascribed to their different hydrophobicity. To evaluate this behavior, c
log
P was calculated from the structure of each repetition unit of the oligoester series (Table 1). Then, the plot of the hydrodynamic radius and intrinsic viscosity vs. c
log
P revealed a linear correlation (Fig. 1), suggesting that polymer–solvent interactions increase with hydrophobicity.
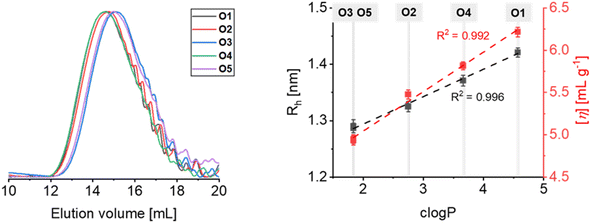 |
| Fig. 1 Size exclusion chromatographic analysis of the oligoesters O1 to O5. Left: refractive index chromatograms. Right: evolution and linear fitting of hydrodynamic radius, Rh, and intrinsic viscosity, [η], vs. c log P for the oligoesters O1 to O5. | |
Additional information was obtained fitting plots of the intrinsic viscosity vs. molar mass to the Mark–Houwink equation (eqn (1)), which enabled calculating the parameter α, related to conformational changes in solution:
where [
η] is the intrinsic viscosity,
i.e. the viscosity of a solution ideally containing one macromolecule,
M is the molar mass of the oligomer, while
k and
α are the Mark–Houwink parameters describing oligomer–solvent interactions, and thus the oligomer morphology in solution.
The fittings are shown in Fig. 2. Even though the obtained α values differ for each oligomer, owing to the different approaches for molar mass estimation, a linear increase of α vs. c
log
P is clearly evident in all cases. The trend indicates good solvent behavior for all the oligoesters in THF. In addition, chain elongation increases with the mers' hydrophobicity (O3/O5 < O2 < O4 < O1), due to increased polymer–solvent interactions. To further evaluate the hydrophobicity of the oligomeric species, diffusion experiments were performed via DOSY NMR in selected deuterated solvents. Despite being often used to gain information on synthetic features, DOSY NMR represents an intriguing technique capable of providing additional insights. Recently, Voorter et al. implemented its applicability for polymer molar mass estimation employing a variety of deuterated solvents as an alternative to SEC analysis.90 However, while these authors analyzed monodisperse polymers and stated that variations in diffusion coefficients depend only on the solvent viscosity, we consider here polydisperse species and show that diffusion is also affected by solvent–polymer interactions. Six deuterated solvents with increased log
P were used to gain information on the oligoester hydrophobicity, i.e., acetone, tetrahydrofuran, chloroform, dichloromethane, benzene, and toluene. Indeed, oligomers with similar molar mass exhibit varying diffusion coefficients in different solvents according to the T1/T2 relaxation time calculation approach (Fig. 3a).
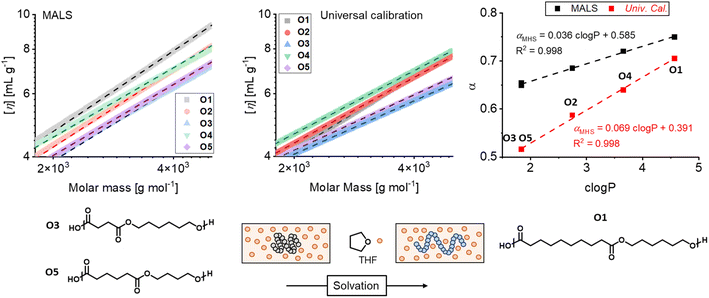 |
| Fig. 2 Mark–Houwink interpretation from SEC analysis in THF of the oligoesters O1 to O5. Left: intrinsic viscosity, [η], over molar mass plot from MALS-RI analysis (markers) and fitting (dotted line). Middle: intrinsic viscosity, [η], over molar mass plot from Viscosimeter-RI analysis (colored markers) and fitting (dotted line). Right: plot and linear fitting of the parameter α of Mark–Houwink of the oligoesters O1 to O5 vs. c log P. | |
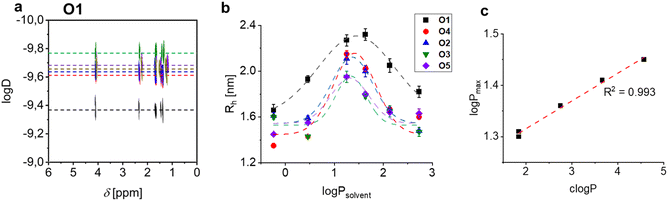 |
| Fig. 3 Evaluation of hydrodynamic radii (Rh) via NMR experiments on the Ox library with deuterated solvents. Left (a): DOSY NMR plot for O1, oligo(exylene sebaceate). Middle (b): evolution of Rh and related errors (calculated from T1/T2 method) vs. solvent log P, for the library O1 to O5. The lines are fitting to a Gaussian-like distribution (eqn (3)); where not indicated, error bars are within markers. Right (c): plot of the estimated log P vs. the calculated oligoester c log P for each repetition unit. | |
In principle, variations of diffusion coefficients in different solvents could be ascribed both to variations of the solvent viscosity and of the hydrodynamic volumes and radii of the diffusing species. To estimate the effect of polymer–solvent interactions, ruling out viscosity, diffusion data were translated to hydrodynamic radii according to the Stokes–Einstein equation (eqn (2)):
|  | (2) |
where
Rh is the hydrodynamic radius,
kb is the Boltzmann constant,
T is the absolute temperature,
η is the viscosity of the solvent at temperature
T, and
D is the diffusion coefficient calculated from
T1/
T2 relaxation times data.
Independently on the oligomer species, the calculated Rh shifts in different solvents, with maximum Rh values in chloroform or dichloromethane (Fig. 3b).
The two solvents have average log
P values in the solvent series, thus the plot of Rhvs. solvent's log
P showed a distribution pattern as highlighted in Fig. 3b. In principle, the capability of a liquid to dissolve a target compound follows the principle of “similia similibus solvuntur”, and the condition of highest solvation directly gives the estimation of the macromolecule log
P. To precisely calculate such parameter, we hypothesized a symmetrical distribution of Rh values, in other words changes of Rh seem to mainly depend on the log
P difference from the best solvent. Accordingly, we fitted the data to a Gaussian-like distribution (eqn (3)):
| 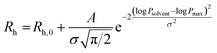 | (3) |
where
Rh is the hydrodynamic radius,
Rh,0 is the minimum
Rh value of the distribution,
A is a pre-exponential factor,
σ is the distribution variance, log
Psolvent is the partition coefficient water/octanol of the solvents, and log
Pmax is the mode of the curve corresponding to the actual log
P of the oligomeric species. The fitting revealed a trend of log
P in the oligoester series, as follows: O1 > O4 > O2 > O3 ≈ O5.
In addition, the newly estimated log
P values are linearly correlated with the c
log
P of the oligomers meric structures (Fig. 3c), proving the consistency of the method. Interestingly, the log
P values were found to be lower than the meric c
log
P, which can be reasonably explained considering that c
log
P is representative of the sole repetition unit and does not consider the oligomer chain capability to arrange in a three-dimensional space.
In conclusion, the proposed approach provides a robust and reliable methodology to evaluate the hydrophobicity of oligomers with slight differences in structure and polydisperse molar mass values. The evaluation served as a crucial step preliminary to the use of the oligoesters in the formulation of the new castor oil gels.
A new class of castor oil–oligoester gels
Each of the characterized oligoesters was reacted with castor and poly(hexamethylene diisocyanate) in a 10/72/18 (oligoester/castor oil/isocyanate) m/m% ratio at 80 °C (see ESI†), yielding dry organogels shaped as sheets. The organogels were named G1–5, following the same nomenclature as their parent oligoesters.
The gels were then swollen with three different solvents, i.e., acetone, methyl ethyl ketone (MEK), and p-xylene. Acetone is one of the most recurring solvents used in the cleaning of works of art, and, along with MEK, exhibits good green chemistry metrics.13 MEK is a versatile solvent that proved effective in the removal of synthetic polymer coatings.61 Finally, p-xylene has good cleaning capability versus wax, which is a common non-polar coating found as finishing layer or contaminant on the surface of works of art.60,94 Even though they were not directly used in the NMR evaluation of the oligoesters, MEK and p-xylene fall either within or very close to the Gaussian distribution of log
P showed in Fig. 3.
The maximum swelling of the organogels in the three selected solvents is showed in Fig. 4 (detailed swelling curves are reported in ESI†). As expected, swelling in acetone was faster (see ESI†) and reached higher solvent contents for the gels containing the less lipophilic additives (G3 and G5) with a relative weight increase (Δm/m0) plateu of 1.2. The remaining gels exhibit lesser uptake (G2 ≈ G4 > G1). A similar swelling behavior was monitored when MEK was employed. In this case, higher values of relative weight increase (c.a. 2.0) were reached, as expected since MEK has a log
P (0.37), closer to those of the oligoesters. The gels formulated with the most hydrophobic oligoesters (G1, G4) exhibited the largest swelling in p-xylene (log
P 3.10). In all cases, swelling in p-xylene is lower than in MEK, and closer to the values of acetone, as predicted by the log
P distribution showed in Fig. 3. These results confirmed the reliability of the novel screening approach to predict the affinity of oligomers, and their derived organogels, to solvents with different polarity. It must be noticed that the swelling capacity of the new castor oil–oligoester organogels is larger than that of castor oil gels previously reported for art cleaning applications.43
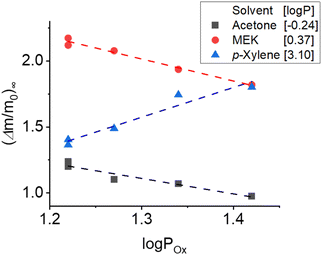 |
| Fig. 4 Evolution of the castor oil organogels' maximum swelling capability (Δm/m0)∞ in acetone (black), MEK (red) and p-xylene (blue) over the log P of oligoesters O1 to O5 obtained from swelling experiments (see ESI†). Dotted lines are simply guidelines to highlight trends. | |
Finally, two of the organogels, G1 and G5, were swollen with, respectively, p-xylene and acetone, and then used for the removal of Paraloid B72 and wax from frosted glass slides that simulate hydrophilic substrates. It was previously verified that the two solvents have TEAS solubility parameters that fall within the swelling areas of the two target coatings (see Fig. 5).
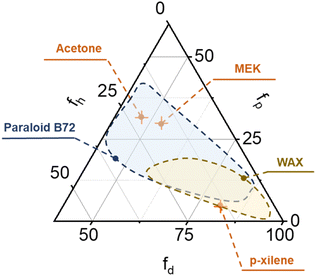 |
| Fig. 5 TEAS swelling areas of Paraloid B72 and wax, and representation of the three solvents (acetone, MEK and p-xylene) employed for the castor oil organogels' swelling experiments. | |
As showed in Fig. 6, the application of the swollen organogels resulted in the complete removal of the coatings after two applications (10 minutes each) without the use of any additional mechanical action. Interestingly, during the removal of Paraloid B72, the acrylate polymer layer adhered to the G5 gel, allowing the coating removal simply as a swollen film attached to the gel.
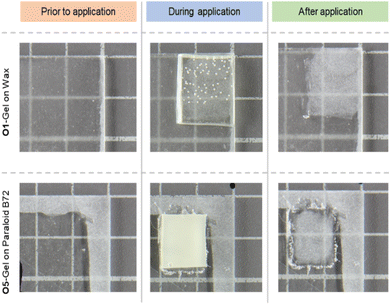 |
| Fig. 6 Cleaning test experiments employing O1 and O5 containing castor oil gels; from left to right: surface prior to gel application, during gel application and cleaned surface after 2 gel applications of 10 minutes each. Top row: application of the O1-gel swollen in p-xylene for 1 h on a frosted glass surface covered with wax. Bottom row: application of the O5-gel swollen in acetone for 1 h on a frosted glass surface covered with Paraloid B72. The coatings make the frosted glass transparent, and their removal by the gels restored the original opaque appearance. | |
Overall, the development of a new evaluation method for the estimation of the log
P of oligomers/polymers was established by means of diffusion analysis. The proposed approach allows a robust and reliable evaluation based on DOSY NMR analysis for (i) polydisperse samples and (ii) also for macromolecules differing for a single methyl unit in the meric structure. The obtained data follow the same trend depicted from calculated data on the repetition unit composition. In addition, this approach grants a more comprehensive insight on the entire polymer behavior in solution. Finally, the evaluation method allowed the design of a new class of organogels (castor oil–oligoester) tailored for the confinement of solvents in a wide range of polarity. The gel–solvent affinity can be simply tuned by selecting the proper oligomer from the screening step. In principle, this design approach can also allow the formulation of gel networks where domains with tailored hydrophobicity/hydrophilicity are created into the 3D gel polymer network.
Conclusions and future perspectives
This contribution depicts novel approaches toward Cultural Heritage preservation based upon (i) an in-depth overview of novel strategies in restorative practices based on the use of polymeric materials and (ii) the development of a new approach for the estimation of log
P and, consequently, HLB, for oligomers and polymers. Overall, the reported experimental data rely on the study of polymer solution dynamics according to both SEC and DOSY NMR. In a first stage, SEC analysis was fundamental to estimate the molar mass of the formulated oligomer library according to absolute and equivalent methods. Moreover, the good correlation of the Mark–Howink parameter α versus the calculated partition coefficient (c
log
P) of the meric units, indicated a variation in polymer–solvent interactions related to the oligomer chemical composition. DOSY NMR further showed that tuning the solvent log
P resulted in the variation of diffusion coefficients and hydrodynamic radii. The obtained data were employed for log
P estimation for each oligomer. We found excellent correlation of the so-obtained log
P with the calculated c
log
P. We also showed how this robust approach can be used to design new organogels based on castor oil polyurethanes modified with the oligoester library. The new gels have optimal swelling capability in organic solvents with a wide range of polarity, making the castor oil–oligoester organogels versatile and promising tools for the removal of undesired/aged coatings and contaminants from the surface of works of art. In addition, our design approach could be used to formulate gel networks where domains with tailored hydrophobicity/hydrophilicity are created into the 3D gel polymer network. For instance, gels with alternated hydrophilic and hydrophobic domains, able to uptake aqueous solutions and organic solvents, would allow addressing simultaneously diverse types of hydrophilic and hydrophobic soil/coatings in a unique, one-step cleaning intervention. In addition, the novel materials described in this contribution fit well into material groups that are relevant to different industrial applications: oligo- and polyesters are widely employed in common goods, from textile to 3D printings, and are building blocks to produce materials in automobiles, nautical sector, and others.95,96 At the same time, polyurethanes have high volume production for applications as insulating foams in the building construction industry. Therefore, the new materials described in this manuscript for Cultural Heritage conservation, could also be of interest for a broader range of scientific and technological applications where green, sustainable solutions are required.
Data availability
All the relevant experimental data are found in the main article text and in the ESI.†
Author contributions
D. B., D. C., G. P. and P. B. conceptualized the project D. B., R. M. and G. P. performed the experimental studies. D. B., D. C. and P. B. prepared the manuscript.
Conflicts of interest
There are no conflicts to declare.
Acknowledgements
CSGI and the European Union (GREENART project, Horizon Europe research and innovation program under grant agreement no. 101060941) are gratefully acknowledged for financial support. Views and opinions expressed are however those of the author(s) only and do not necessarily reflect those of the European Union or the European Research Executive Agency (REA). Neither the European Union nor the granting authority can be held responsible for them. The publication was made by a researcher (DB) with a research contract funded by the European Union-PNRR NextGenerationEU in accordance with Article 24, paragraph 3a of Law No. 240 of December 30, 2010, as amended and Ministerial Decree No. 1365 of November 8, 2022 and by (RM) with a research contract co-funded by the European Union – PON Research and Innovation 2014–2020 in accordance with Article 24, paragraph 3a of Law No. 240 of December 30, 2010, as amended and Ministerial Decree No. 1062 of August 10, 2021.
References
- M. J. Mulvihill, E. S. Beach, J. B. Zimmerman and P. T. Anastas, Green Chemistry and Green Engineering: A Framework for Sustainable Technology Development, Annual Review of Environment and Resources, 2011, 36, 271–293 CrossRef.
- J. B. Zimmerman, P. T. Anastas, H. C. Erythropel and W. Leitner, Designing for a green chemistry future, Science, 2020, 367, 397–400 CrossRef CAS PubMed.
- K. L. Mulholland, R. W. Sylvester and J. A. Dyer, Sustainability: waste minimization, green chemistry and inherently safer processing, Environ. Prog., 2000, 19, 260–268 CrossRef CAS.
- C. L. Kelly, Addressing the sustainability challenges for polymers in liquid formulations, Chem. Sci., 2023, 14, 6820–6825 RSC.
- X. Wang, Y. Qian, H. Gao, C. W. Coley, Y. Mo, R. Barzilay and K. F. Jensen, Towards efficient discovery of green synthetic pathways with Monte Carlo tree search and reinforcement learning, Chem. Sci., 2020, 11, 10959–10972 RSC.
- S. A. Matlin, S. E. Cornell, A. Krief, H. Hopf and G. Mehta, Chemistry must respond to the crisis of transgression of planetary boundaries, Chem. Sci., 2022, 13, 11710–11720 RSC.
- N. Brun, P. Hesemann and D. Esposito, Expanding the biomass derived chemical space, Chem. Sci., 2017, 8, 4724–4738 RSC.
- F. Boccaccini, C. Giuliani, M. Pascucci, C. Riccucci, E. Messina, M. P. Staccioli, G. M. Ingo and G. Di Carlo, Toward a Green and Sustainable Silver Conservation: Development and Validation of Chitosan-Based Protective Coatings, Int. J. Mol. Sci., 2022, 23, 14454 CrossRef CAS PubMed.
- C. Samorì, P. Galletti, L. Giorgini, R. Mazzeo, L. Mazzocchetti, S. Prati, G. Sciutto, F. Volpi and E. Tagliavini, The Green Attitude in Art Conservation: Polyhydroxybutyrate–Based Gels for the Cleaning of Oil Paintings, ChemistrySelect, 2016, 1, 4502–4508 CrossRef.
- V. Rosciardi, D. Chelazzi and P. Baglioni, “Green” biocomposite poly(vinyl alcohol)/starch cryogels as new advanced tools for the cleaning of artifacts, J. Colloid Interface Sci., 2022, 613, 697–708 CrossRef CAS PubMed.
- G. Cavallaro, S. Milioto, L. Nigamatzyanova, F. Akhatova, R. Fakhrullin and G. Lazzara, Pickering Emulsion Gels Based on Halloysite Nanotubes and Ionic Biopolymers: Properties and Cleaning Action on Marble Surface, ACS Appl. Nano Mater., 2019, 2, 3169–3176 CrossRef CAS.
- A. Passaretti, L. Cuvillier, G. Sciutto, E. Guilminot and E. Joseph, Biologically Derived Gels for the Cleaning of Historical and Artistic Metal Heritage, Appl. Sci., 2021, 11, 3405 CrossRef CAS.
- A. Casini, D. Chelazzi and P. Baglioni, Advanced methodologies for the cleaning of works of art, Sci. China: Technol. Sci., 2023, 66, 2162–2182 CrossRef.
- P. I. Girginova, C. Galacho, R. Veiga, A. Santos Silva and A. Candeias, Inorganic Nanomaterials for Restoration of Cultural Heritage: Synthesis Approaches towards Nanoconsolidants for Stone and Wall Paintings, ChemSusChem, 2018, 11, 4168–4182 CrossRef CAS PubMed.
- D. Chelazzi, G. Poggi, Y. Jaidar, N. Toccafondi, R. Giorgi and P. Baglioni, Hydroxide nanoparticles for cultural heritage: consolidation and protection of wall paintings and carbonate materials, J. Colloid Interface Sci., 2013, 392, 42–49 CrossRef CAS PubMed.
- R. Giorgi, D. Chelazzi, E. Fratini, S. Langer, A. Niklasson, M. Rådemar, J.-E. Svensson and P. Baglioni, Nanoparticles of calcium hydroxide for wood deacidification: decreasing the emissions of organic acid vapors in church organ environments, Journal of Cultural Heritage, 2009, 10, 206–213 CrossRef.
- C. Rodriguez-Navarro and E. Ruiz-Agudo, Nanolimes: from
synthesis to application, Pure Appl. Chem., 2018, 90, 523–550 CrossRef CAS.
- M. Frigione and M. Lettieri, Novel Attribute of Organic–Inorganic Hybrid Coatings for Protection and Preservation of Materials (Stone and Wood) Belonging to Cultural Heritage, Coatings, 2018, 8, 319 CrossRef.
- C. Cianci, D. Chelazzi, G. Poggi, F. Modi, R. Giorgi and M. Laurati, Hybrid fibroin-nanocellulose composites for the consolidation of aged and historical silk, Colloids Surf., A, 2022, 634, 127944 CrossRef CAS.
- F. Giacobello, I. Ielo, H. Belhamdi and M. R. Plutino, Geopolymers and Functionalization Strategies for the Development of Sustainable Materials in Construction Industry and Cultural Heritage Applications: A Review, Materials, 2022, 15, 1725 CrossRef CAS PubMed.
- A. Zuliani, D. Chelazzi, R. Mastrangelo, R. Giorgi and P. Baglioni, Adsorption kinetics of acetic acid into ZnO/castor oil-derived polyurethanes, J. Colloid Interface Sci., 2023, 632, 74–86 CrossRef CAS PubMed.
- D. Chelazzi, R. Giorgi and P. Baglioni, Microemulsions, Micelles, and Functional Gels: How Colloids and Soft Matter Preserve Works of Art, Angew. Chem., Int. Ed., 2018, 57, 7296–7303 CrossRef CAS PubMed.
- A. Artesani, F. Di Turo, M. Zucchelli and A. Traviglia, Recent Advances in Protective Coatings for Cultural Heritage–An Overview, Coatings, 2020, 10, 217 CrossRef CAS.
- D. K. Silva da Conceição, K. Nunes de Almeida, E. Nhuch, M. G. Raucci, C. Santillo, M. Salzano de Luna, L. Ambrosio, M. Lavorgna, C. Giuliani, G. Di Carlo, M. P. Staccioli, T. Falcade and H. S. Schrekker, The synergistic effect of an imidazolium salt and benzotriazole on the protection of bronze surfaces with chitosan-based coatings, Heritage Sci., 2020, 8, 40 CrossRef.
- C. H. M. Camargos, G. Poggi, D. Chelazzi, P. Baglioni and C. A. Rezende, Protective Coatings Based on Cellulose Nanofibrils, Cellulose Nanocrystals, and Lignin Nanoparticles for the Conservation of Cellulosic Artifacts, ACS Appl. Nano Mater., 2022, 5, 13245–13259 CrossRef CAS.
- P. N. Manoudis, I. Karapanagiotis, A. Tsakalof, I. Zuburtikudis, B. Kolinkeová and C. Panayiotou, Superhydrophobic films for the protection of outdoor cultural heritage assets, Appl. Phys. A: Mater. Sci. Process., 2009, 97, 351–360 CrossRef CAS.
- G. Infurna, G. Cavallaro, G. Lazzara, S. Milioto and N. T. Dintcheva, Bionanocomposite Films Containing Halloysite Nanotubes and Natural Antioxidants with Enhanced Performance and Durability as Promising Materials for Cultural Heritage Protection, Polymers, 2020, 12, 1973 CrossRef CAS PubMed.
- G. Infurna, G. Cavallaro, G. Lazzara, S. Milioto and N. T. Dintcheva, Understanding the Effects of Crosslinking and Reinforcement Agents on the Performance and Durability of Biopolymer Films for Cultural Heritage Protection, Molecules, 2021, 26, 3468 CrossRef CAS.
- S. Filipović, N. Lior and M. Radovanović, The green deal – just transition and sustainable development goals Nexus, Renewable Sustainable Energy Rev., 2022, 168, 112759 CrossRef.
- P. Baglioni, E. Carretti and D. Chelazzi, Nanomaterials in art conservation, Nat. Nanotechnol., 2015, 10, 287–290 CrossRef CAS PubMed.
- P. Baglioni and D. Chelazzi, How Science Can Contribute to the Remedial Conservation of Cultural Heritage, Chem.–Eur. J., 2021, 27, 10798–10806 CrossRef CAS PubMed.
- M. Baglioni, G. Poggi, D. Chelazzi and P. Baglioni, Advanced Materials in Cultural Heritage Conservation, Molecules, 2021, 26, 3967 CrossRef CAS PubMed.
- C. E. Dillon, A. F. Lagalante and R. C. Wolbers, Acrylic emulsion paint films: the effect of solution pH, conductivity, and ionic strength on film swelling and surfactant removal, Stud. Conserv., 2014, 59, 52–62 CrossRef CAS.
- L. Baij, J. Hermans, B. Ormsby, P. Noble, P. Iedema and K. Keune, A review of solvent action on oil paint, Heritage Sci., 2020, 8, 43 CrossRef.
- B. Ormsby and T. Learner, The effects of wet surface cleaning treatments on acrylic emulsion artists' paints – a review of recent scientific research, Stud. Conserv., 2009, 54, 29–41 CrossRef.
- A. Casoli, Z. Di Diego and C. Isca, Cleaning painted surfaces: evaluation of leaching phenomenon induced by solvents applied for the removal of gel residues, Environ. Sci. Pollut. Res., 2014, 21, 13252–13263 CrossRef CAS PubMed.
- R. Mastrangelo, D. Chelazzi, G. Poggi, E. Fratini, L. Pensabene Buemi, M. L. Petruzzellis and P. Baglioni, Twin-chain polymer hydrogels based on poly(vinyl alcohol) as new advanced tool for the cleaning of modern and contemporary art, Proc. Natl. Acad. Sci. U. S. A., 2020, 117, 7011–7020 CrossRef CAS PubMed.
- L. Pensabene Buemi, M. L. Petruzzellis, D. Chelazzi, M. Baglioni, R. Mastrangelo, R. Giorgi and P. Baglioni, Twin-chain polymer networks loaded with nanostructured fluids for the selective removal of a non-original varnish from Picasso's “L'Atelier” at the Peggy Guggenheim Collection, Venice, Heritage Sci., 2020, 8, 77 CrossRef CAS.
- A. Bartoletti, R. Barker, D. Chelazzi, N. Bonelli, P. Baglioni, J. Lee, L. V. Angelova and B. Ormsby, Reviving WHAAM! a comparative evaluation of cleaning systems for the conservation treatment of Roy Lichtenstein's iconic painting, Heritage Sci., 2020, 8, 9 CrossRef.
- A. Bartoletti, T. Maor, D. Chelazzi, N. Bonelli, P. Baglioni, L. V. Angelova and B. A. Ormsby, Facilitating the conservation treatment of Eva Hesse's Addendum through practice-based research, including a comparative evaluation of novel cleaning systems, Heritage Sci., 2020, 8, 35 CrossRef CAS.
- P. Ferrari, D. Chelazzi, N. Bonelli, A. Mirabile, R. Giorgi and P. Baglioni, Alkyl carbonate solvents confined in poly(ethyl methacrylate) organogels for the removal of pressure sensitive tapes (PSTs) from contemporary drawings, Journal of Cultural Heritage, 2018, 34, 227–236 CrossRef.
- M. D. Pianorsi, M. Raudino, N. Bonelli, D. Chelazzi, R. Giorgi, E. Fratini and P. Baglioni, Organogels for the cleaning of artifacts, Pure Appl. Chem., 2017, 89, 3–17 CrossRef CAS.
- G. Poggi, H. D. Santan, J. Smets, D. Chelazzi, D. Noferini, M. L. Petruzzellis, L. Pensabene Buemi, E. Fratini and P. Baglioni, Nanostructured bio-based castor oil organogels for the cleaning of artworks, J. Colloid Interface Sci., 2023, 638, 363–374 CrossRef CAS PubMed.
- S. Prati, F. Volpi, R. Fontana, P. Galletti, L. Giorgini, R. Mazzeo, L. Mazzocchetti, C. Samorì, G. Sciutto and E. Tagliavini, Sustainability in art conservation: a novel bio-based organogel for the cleaning of water sensitive works of art, Pure Appl. Chem., 2018, 90, 239–251 CrossRef CAS.
- Y. Çakmak, E. Çakmakçi, N. K. Apohan and R. Karadag, Isosorbide, pyrogallol, and limonene-containing thiol-ene photocured bio-based organogels for the cleaning of artworks, Journal of Cultural Heritage, 2022, 55, 391–398 CrossRef.
- T. T. Duncan, B. H. Berrie and R. G. Weiss, Soft, Peelable Organogels from Partially Hydrolyzed Poly(vinyl acetate) and Benzene-1,4-diboronic Acid: Applications to Clean Works of Art, ACS Appl. Mater. Interfaces, 2017, 9, 28069–28078 CrossRef CAS PubMed.
- H. Mutlu and M. A. R. Meier, Castor oil as a renewable resource for the chemical industry, Eur. J. Lipid Sci. Technol., 2010, 112, 10–30 CrossRef CAS.
- A. Zuliani, D. Bandelli, D. Chelazzi, R. Giorgi and P. Baglioni, Environmentally friendly ZnO/castor oil polyurethane composites for the gas-phase adsorption of acetic acid, J. Colloid Interface Sci., 2022, 614, 451–459 CrossRef CAS PubMed.
- D. Feldman, Polymer History, Des. Monomers Polym., 2008, 11, 1–15 CrossRef CAS.
- K. Wang, K. Amin, Z. An, Z. Cai, H. Chen, H. Chen, Y. Dong, X. Feng, W. Fu, J. Gu, Y. Han, D. Hu, R. Hu, D. Huang, F. Huang, F. Huang, Y. Huang, J. Jin, X. Jin, Q. Li, T. Li, Z. Li, Z. Li, J. Liu, J. Liu, S. Liu, H. Peng, A. Qin, X. Qing, Y. Shen, J. Shi, X. Sun, B. Tong, B. Wang, H. Wang, L. Wang, S. Wang, Z. Wei, T. Xie, C. Xu, H. Xu, Z.-K. Xu, B. Yang, Y. Yu, X. Zeng, X. Zhan, G. Zhang, J. Zhang, M. Q. Zhang, X.-Z. Zhang, X. Zhang, Y. Zhang, Y. Zhang, C. Zhao, W. Zhao, Y. Zhou, Z. Zhou, J. Zhu, X. Zhu and B. Z. Tang, Advanced functional polymer materials, Mater. Chem. Front., 2020, 4, 1803–1915 RSC.
- R. Chércoles Asensio, M. San Andrés Moya, J. M. de la Roja and M. Gómez, Analytical characterization of polymers used in conservation and restoration by ATR-FTIR spectroscopy, Anal. Bioanal. Chem., 2009, 395, 2081–2096 CrossRef PubMed.
- M. Lazzari, A. Ledo-Suárez, T. López, D. Scalarone and M. A. López-Quintela, Plastic matters: an analytical procedure to evaluate the degradability of contemporary works of art, Anal. Bioanal. Chem., 2011, 399, 2939–2948 CrossRef CAS PubMed.
- T. Learner, A review of synthetic binding media in twentieth-century paints, The Conservator, 2000, 24, 96–103 CrossRef.
-
R. Wolbers, Cleaning painted surfaces: aqueous methods, 2000 Search PubMed.
- D. Quintero Balbas, A. Dal Fovo, D. Porcu, A. Chaban, S. Porcinai, R. Fontana and J. Striova, Non-Invasive Evaluation of Polymeric Protective Coatings for Metal Surfaces of Cultural Heritage Objects: Comparison of Optical and Electromagnetic Methods, Appl. Sci., 2022, 12, 7532 CrossRef CAS.
- O. Chiantore and M. Lazzari, Photo-oxidative stability of paraloid acrylic protective polymers, Polymer, 2001, 42, 17–27 CrossRef CAS.
- F. Cappitelli, F. Villa and P. Sanmartín, Interactions of microorganisms and synthetic polymers in cultural heritage conservation, Int. Biodeterior. Biodegrad., 2021, 163, 105282 CrossRef CAS.
- F. Cappitelli and C. Sorlini, Microorganisms Attack Synthetic Polymers in Items Representing Our Cultural Heritage, Appl. Environ. Microbiol., 2008, 74, 564–569 CrossRef CAS.
- D. Chelazzi, A. Chevalier, G. Pizzorusso, R. Giorgi, M. Menu and P. Baglioni, Characterization and degradation of poly(vinyl acetate)-based adhesives for canvas paintings, Polym. Degrad. Stab., 2014, 107, 314–320 CrossRef CAS.
- P. Baglioni, D. Chelazzi, R. Giorgi and G. Poggi, Colloid and Materials Science for the Conservation of Cultural Heritage: Cleaning, Consolidation, and Deacidification, Langmuir, 2013, 29, 5110–5122 CrossRef CAS PubMed.
- M. Baglioni, C. Montis, D. Chelazzi, R. Giorgi, D. Berti and P. Baglioni, Polymer Film Dewetting by Water/Surfactant/Good-Solvent Mixtures: A Mechanistic Insight and Its Implications for the Conservation of Cultural Heritage, Angew. Chem., Int. Ed., 2018, 57, 7355–7359 CrossRef CAS PubMed.
- M. Baglioni, F. H. Sekine, T. Ogura, S.-H. Chen and P. Baglioni, Nanostructured fluids for polymeric
coatings removal: surfactants affect the polymer glass transition temperature, J. Colloid Interface Sci., 2022, 606, 124–134 CrossRef CAS PubMed.
-
L. V. Angelova, B. Ormsby, J. Townsend and R. Wolbers, Gels in the Conservation of Art, Archetype Publications, 2017 Search PubMed.
-
D. Chelazzi, E. Fratini, R. Giorgi, R. Mastrangelo, M. Rossi and P. Baglioni, in Gels and Other Soft Amorphous Solids, American Chemical Society, 2018, ch. 15, vol. 1296, pp. 291–314 Search PubMed.
-
R. C. Wolbers, C. Stavroudis and M. Cushman, Aqueous methods for the cleaning of paintings, in Conservation of Easel Paintings, 2020, pp. 526–548 Search PubMed.
- G. Pizzorusso, E. Fratini, J. Eiblmeier, R. Giorgi, D. Chelazzi, A. Chevalier and P. Baglioni, Physicochemical Characterization of Acrylamide/Bisacrylamide Hydrogels and Their Application for the Conservation of Easel Paintings, Langmuir, 2012, 28, 3952–3961 CrossRef CAS PubMed.
- J. A. L. Domingues, N. Bonelli, R. Giorgi, E. Fratini, F. Gorel and P. Baglioni, Innovative Hydrogels Based on Semi-Interpenetrating p(HEMA)/PVP Networks for the Cleaning of Water-Sensitive Cultural Heritage Artifacts, Langmuir, 2013, 29, 2746–2755 CrossRef CAS PubMed.
- M. Baglioni, J. A. L. Domingues, E. Carretti, E. Fratini, D. Chelazzi, R. Giorgi and P. Baglioni, Complex Fluids Confined into Semi-interpenetrated Chemical Hydrogels for the Cleaning of Classic Art: A Rheological and SAXS Study, ACS Appl. Mater. Interfaces, 2018, 10, 19162–19172 CrossRef CAS PubMed.
- N. Bonelli, C. Montis, A. Mirabile, D. Berti and P. Baglioni, Restoration of paper artworks with microemulsions confined in hydrogels for safe and efficient removal of adhesive tapes, Proc. Natl. Acad. Sci. U. S. A., 2018, 115, 5932–5937 CrossRef CAS PubMed.
- M. Baglioni, G. Poggi, R. Giorgi, P. Rivella, T. Ogura and P. Baglioni, Selective removal of over-paintings from “Street Art” using an environmentally friendly nanostructured fluid loaded in highly retentive hydrogels, J. Colloid Interface Sci., 2021, 595, 187–201 CrossRef CAS PubMed.
- L. V. Angelova, P. Terech, I. Natali, L. Dei, E. Carretti and R. G. Weiss, Cosolvent Gel-like Materials from Partially Hydrolyzed Poly(vinyl acetate)s and Borax, Langmuir, 2011, 27, 11671–11682 CrossRef CAS PubMed.
- E. Carretti, C. Matarrese, E. Fratini, P. Baglioni and L. Dei, Physicochemical characterization of partially hydrolyzed poly(vinyl acetate)–borate aqueous dispersions, Soft Matter, 2014, 10, 4443–4450 RSC.
- E. Al-Emam, H. Soenen, J. Caen and K. Janssens, Characterization of polyvinyl alcohol-borax/agarose (PVA-B/AG) double network hydrogel utilized for the cleaning of works of art, Heritage Sci., 2020, 8, 106 CrossRef CAS.
- T. Guaragnone, A. Casini, D. Chelazzi and R. Giorgi, PVA-based peelable films loaded with tetraethylenepentamine for the removal of corrosion products from bronze, Appl. Mater. Today, 2020, 19, 100549 CrossRef.
- P. Baglioni, N. Bonelli, D. Chelazzi, A. Chevalier, L. Dei, J. Domingues, E. Fratini, R. Giorgi and M. Martin, Organogel formulations for the cleaning of easel paintings, Appl. Phys. A: Mater. Sci. Process., 2015, 121, 857–868 CrossRef CAS.
- A. Mirabile, D. Chelazzi, P. Ferrari, C. Montis, D. Berti, N. Bonelli, R. Giorgi and P. Baglioni, Innovative methods for the removal, and occasionally care, of pressure sensitive adhesive tapes from contemporary drawings, Heritage Sci., 2020, 8, 42 CrossRef CAS.
- C. K. Williams and M. A. Hillmyer, Polymers from Renewable Resources: A Perspective for a Special Issue of Polymer Reviews, Polym. Rev., 2008, 48, 1–10 CrossRef CAS.
- H. D. Santan, C. James, E. Fratini, I. Martínez, C. Valencia, M. C. Sánchez and J. M. Franco, Structure-property relationships in solvent free adhesives derived from castor oil, Ind. Crops Prod., 2018, 121, 90–98 CrossRef CAS.
- A. Casini, D. Chelazzi and R. Giorgi, Jin Shofu Starch Nanoparticles for the Consolidation of Modern Paintings, ACS Appl. Mater. Interfaces, 2021, 13, 37924–37936 CrossRef CAS.
- M. a. A. Longo and D. Combes, Influence of surface hydrophilic/hydrophobic balance on enzyme properties, J. Biotechnol., 1997, 58, 21–32 CrossRef CAS.
- Y. Xia, J. Wang, S. Xu, Q. Liao, X. Zhu, Y. Wang and Y. Wang, Dually stimuli-responsive hyperbranched polyethylenimine with LCST transition based on hydrophilic–hydrophobic balance, J. Appl. Polym. Sci., 2013, 127, 3249–3255 CrossRef CAS.
- A. Vollrath, C. Kretzer, B. Beringer-Siemers, B. Shkodra, J. A. Czaplewska, D. Bandelli, S. Stumpf, S. Hoeppener, C. Weber, O. Werz and U. S. Schubert, Effect of Crystallinity on the Properties of Polycaprolactone Nanoparticles Containing the Dual FLAP/mPEGS-1 Inhibitor BRP-187, Polymers, 2021, 13, 2557 CrossRef CAS PubMed.
- K. Scheuer, D. Bandelli, C. Helbing, C. Weber, J. Alex, J. B. Max, A. Hocken, O. Stranik, L. Seiler, F. Gladigau, U. Neugebauer, F. H. Schacher, U. S. Schubert and K. D. Jandt, Self-Assembly of Copolyesters into Stereocomplex Crystallites Tunes the Properties of Polyester Nanoparticles, Macromolecules, 2020, 53, 8340–8351 CrossRef CAS.
- D. Bandelli, J. Alex, C. Helbing, N. Ueberschaar, H. Görls, P. Bellstedt, C. Weber, K. D. Jandt and U. S. Schubert, Poly(3-ethylglycolide): a well-defined polyester matching the hydrophilic hydrophobic balance of PLA, Polym. Chem., 2019, 10, 5440–5451 RSC.
- D. Bandelli, C. Helbing, C. Weber, M. Seifert, I. Muljajew, K. D. Jandt and U. S. Schubert, Maintaining the Hydrophilic–Hydrophobic Balance of Polyesters with Adjustable Crystallinity for Tailor-Made Nanoparticles, Macromolecules, 2018, 51, 5567–5576 CrossRef CAS.
- D. Bandelli, I. Muljajew, K. Scheuer, J. B. Max, C. Weber, F. H. Schacher, K. D. Jandt and U. S. Schubert, Copolymerization of Caprolactone Isomers to Obtain Nanoparticles with Constant Hydrophobicity and Tunable Crystallinity, Macromolecules, 2020, 53, 5208–5217 CrossRef CAS.
- H. Schott, Hydrophilic-Lipophilic Balance, Solubility Parameter, and Oil-Water Partition Coefficient as Universal Parameters of Nonionic Surfactants, J. Pharm. Sci., 1995, 84, 1215–1222 CrossRef CAS PubMed.
-
J. M. Goldwasser, in Chromatography of Polymers, American Chemical Society, 1993, ch. 16, vol. 521, pp. 243–251 Search PubMed.
- M. Gaborieau and P. Castignolles, Size-exclusion chromatography (SEC) of branched polymers and polysaccharides, Anal. Bioanal. Chem., 2011, 399, 1413–1423 CrossRef CAS PubMed.
- P.-J. Voorter, A. McKay, J. Dai, O. Paravagna, N. R. Cameron and T. Junkers, Solvent-Independent Molecular Weight Determination of Polymers Based on a Truly Universal Calibration, Angew. Chem., Int. Ed., 2022, 61, e202114536 CrossRef CAS PubMed.
- E. Ruzicka, P. Pellechia and B. C. Benicewicz, Polymer Molecular Weights via DOSY NMR, Anal. Chem., 2023, 95, 7849–7854 CrossRef CAS PubMed.
- H. R. Kricheldorf, M. G. Zolotukhin and J. Cárdenas, Non-Stoichiometric Polycondensations and the Synthesis of High Molar Mass Polycondensates, Macromol. Rapid Commun., 2012, 33, 1814–1832 CrossRef CAS PubMed.
- Q. Cai, T. Bai, H. Zhang, X. Yao, J. Ling and W. Zhu, Catalyst-free synthesis of polyesters
via conventional melt polycondensation, Mater. Today, 2021, 51, 155–164 CrossRef CAS.
- T. Kosec, A. Legat and I. Milošev, The comparison of organic protective layers on bronze and copper, Prog. Org. Coat., 2010, 69, 199–206 CrossRef CAS.
- K. Amulya, R. Katakojwala, S. Ramakrishna and S. Venkata Mohan, Low carbon biodegradable polymer matrices for sustainable future, Compos., Part C: Open Access, 2021, 4, 100111 CAS.
- K. Subramanian, M. K. Sarkar, H. Wang, Z.-H. Qin, S. S. Chopra, M. Jin, V. Kumar, C. Chen, C.-W. Tsang and C. S. K. Lin, An overview of cotton and polyester, and their blended waste textile valorisation to value-added products: a circular economy approach – research trends, opportunities and challenges, Crit. Rev. Environ. Sci. Technol., 2022, 52, 3921–3942 CrossRef.
|
This journal is © The Royal Society of Chemistry 2024 |