DOI:
10.1039/D3MA00406F
(Review Article)
Mater. Adv., 2024,
5, 2719-2735
Modifications of polyalkenoic acid and its effect on glass ionomer cement
Received
13th July 2023
, Accepted 13th February 2024
First published on 14th February 2024
Abstract
Over the past few decades, glass ionomer cement (GIC) has played a pivotal role in dental restoration. GIC has many advantages over other restorative materials including biocompatibility, dental adhesion, aesthetic features, and anticariogenic activity. In addition, GIC can favour remineralisation and reduce the resistance of enamel and dentine towards demineralisation. However, many limitations of conventional GIC including reduced compression and diametrical tensile strength, reduced surface characteristics, brittleness and sensitivity towards moisture when placed first in the mouth retarded the wide applications of GIC in clinical dentistry. Active research is still ongoing to overcome the limitations of conventional GIC. The current review focuses on different modifications of polyalkenoic acid and its effects on improving the physicochemical properties of GIC.
1. Introduction
Glass ionomer cement (GIC) is a dental restorative material formed by an acid–base setting reaction of ion leachable glass (e.g., calcium fluoroalumino silicate) and a weak polyalkenoic acid (polyacid).1 The ability of ionized polyacid to form cross-linked salts with di- and trivalent metal ions liberated from the glass powder results in the formation of a hard setting gel or a matrix of the cement. Here the unreacted glass powder after the setting reaction acts as the filler to reinforce the set cement. Polyalkenoate cement is widely accepted among dentists for multifactorial applications that include core build-up, class III and V carious lesions, cavity linings, replacing carious dentin, root surface sealing during root canal treatment, and sealants for fissures and pits in the tooth.2,3 Importantly, no coupling agents or etching techniques are needed for the strong adhesion of GIC to dentin and enamel. GIC shows thermal compatibility with natural teeth, anticariogenic effects and increased biocompatibility compared to other dental restorations. In addition, GIC can favour remineralisation and reduce the resistance of enamel and dentine towards demineralisation. At the same time, many limitations of conventional GIC including reduced compression and diametrical tensile strength, reduced surface characteristics, brittleness and sensitivity towards moisture when placed first in the mouth retarded the wide applications of GIC in clinical dentistry. Advantages and disadvantages of conventional glass ionomer cement are depicted in Table 1.
Table 1 Advantages and disadvantages of conventional glass ionomer cement
Advantages |
Disadvantages |
Excellent biocompatibility, low toxicity, and marginal adaptation |
Sensitive to moisture and can be affected by acidic environments |
Strong adhesion to dentin and enamel, no need for a bonding agent |
Adhesion may be weakened by saliva contamination |
GIC can favour remineralisation and reduce the resistance of enamel and dentine towards demineralisation |
Bonding may be affected by moisture and erroneous mixing |
Releases fluoride ions, which help to remineralize tooth enamel and prevent decay (caries preventive action) |
Fluoride release may be reduced by acidic environments |
Almost similar thermal diffusivity to dentin which provides thermal insulation to the pulp |
Translucency may not be the same as the natural tooth structure |
Tooth-coloured material, offering good aesthetic results |
Poor mechanical properties, e.g. low compressive strength, low abrasion resistance, and fracture resistance, restricted use to only in low stress-bearing areas |
Easy to mix and manipulate, can last for several years with appropriate care |
Prone to chipping and wear in high-stress areas |
Many studies showed that all the carboxyl groups of polyalkenoic acid do not participate in the cement formation.4–9 Inter/intramolecular hydrogen bonding in polyalkenoic acid leads to the formation of a rigid matrix which causes steric hindrance and limits the formation of salt bridges. Reduced salt bridge formation along with other restrictions reduce the mechanical properties of conventional GIC. Much effort for improving conventional GIC has been reported since its invention by Wilson and Kent in 1968. For example, resin-modified GIC (RMGIC),10–12 metal-modified GIC (MMGIC),13 fiber-reinforced GIC (FRGIC),14 giomers,15 hainomers,16 amalgomers,17 zirconium-modified GIC,18 nanofiber-reinforced GIC,19etc.
Metal-modified GIC is introduced as an attempt to improve the physicochemical characteristics of the conventional GIC. Due to high-density, MMGIC has significant mechanical strength, fracture resistance, and abrasion resistance. However, MMGIC shows less adhesion to the dental tissues.20,21 RMGIC is another key development in the area of dental restoration. RMGIC contains organic monomers, typically 2-hydroxyethyl methacrylate (HEMA) and an associated initiator along with a basic ion-leachable glass powder and water-soluble polyalkenoic acid. RMGIC undergoes two types of setting reactions, an acid–base reaction and a light or chemically activated polymerization reaction. RMGIC is widely accepted by dentists as it has the upper hand regarding several properties such as working time, setting time, reduced water sensitivity, early strength, and no need for etching compared to the conventional GIC. However, RMGIC has shown an increase in polymeric shrinkage, microleakage, low wear resistance and unstable colour.22–24 Giomers show higher optical properties than RMGIC.25 Giomers contain pre-reacted glass-ionomer fillers in the resin matrix. The incorporation of hydroxyapatite into glass powder may lead to the development of a hainomer. The presence of natural apatite in the dental hard tissues and skeletal system may influence the addition of hydroxyapatite to the glass ionomer as well as the bone cement. Nano hydroxyapatite incorporated GIC shows a significant improvement in mechanical strength and remineralization of the dental enamel.26 Zirconomers provide high strength and durability without any toxicity, and are also called white amalgam as they show mechanical properties similar to those of amalgam. Here zirconium pillars are incorporated into the glass ionomer cement to achieve superior mechanical properties.27 Zirconomers can be used for core build-up, sandwich restoration, fractured amalgam restoration, atraumatic restorative techniques, etc.28 Many studies that include nanoparticles in GIC show a significant improvement in the physicochemical properties compared to conventional GIC. Both polyalkenoic acid and glass powder can be altered using cellulose nanofibers, titanium oxide nanoparticles, nano clays and nanoceramics.4,18,19 However, conventional GIC is not obsolete from clinical dentistry yet. There is a progressive growth in the number of manuscripts published on glass ionomer cement and its modifications every year. A graphical representation of the number of publications for the last 20 years is shown in Fig. 1. The current review focuses on different modifications of polyalkenoic acid to improve the physicochemical, antimicrobial, aesthetic and handling characteristics of GIC. This review also touches upon computational studies of GIC reported so far.
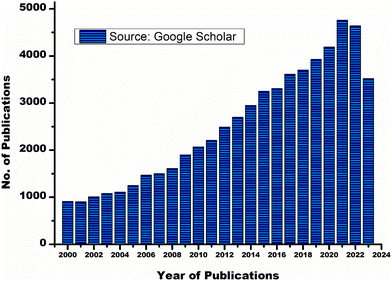 |
| Fig. 1 Number of publications reporting on glass ionomer cement in the last 20 years found using the platform of google scholar. Different keywords were used for collecting manuscripts (e.g. glass ionomer cement, GIC and polyalkenoic acid). | |
1.1. History of GIC
In 1908 Black et al. demonstrated the use of amalgam and gold in dental restoration mainly for cavity filling. But less adhesion of gold and amalgam to the dental tissues affected their manipulation and efficiency.29 Wilson and co-workers resolved the issue using glass ionomer cement. Several modifications of GIC were introduced thereafter.30 Adding additives such as tartaric acid to polyalkenoic acid led to improved rheological properties and accelerated the setting speed of aluminosilicate polyacrylate (ASPA II).31 Crisp and Wilson tried the combination of methyl alcohol and polyacrylic acid in the liquid composition (ASPA III).32 The extended setting time due to the esterification of carboxylic acid in polyacrylic acid was the major advantage of ASPA III. However, discoloration happened during the clinical trial and the system was abandoned.33 Later copolymers of acrylic acid and itaconic acid with 5–15% tartaric acid were identified as an improved liquid composition as they reduced stereoregularity of a polymer and prevented the gelation (ASPA IV). ASPA IV was the first marketed product.34 During 1970 silver reinforced GIC or miracle mix was marketed by GC America and 3M ESPE. The dental product division of 3M company launched the first resin modified GIC named Vitre bond™ in 1990.35 In the early 1990s light cured resin modified GIC was introduced into the market. The next year a tricure system named Vitremer™ was developed by 3M ESPE.36 The nano ionomer technology based RMGIC was introduced in the market in 2007 (Ketac™). The evolution of GIC in the market still continues in the form of modifications and new formulations to improve the strength and toughness of GIC. Significant innovations have been made in dental restoration materials, each of them represented as patented technologies. Novel polymer modified glasses for the GIC were developed by Alireza et al. using click chemistry and reverse addition fragmentation transfer polymerisation (RAFT) method.37 In 2013 Marcel Marchiori Farret et al. were granted a patent for antimicrobial orthodontic glass ionomer cement, which is designed to prevent microbial growth during different orthodontic procedures.38 The silver nanoparticle incorporated GIC developed in 2018 can enhance the antimicrobial properties of the GIC developed by Meledandri and Carla Joy.39 Early tooth decay can be prevented by bioactive glass facilitating remineralization invented in 2020.40 Amino acid integrated RMGIC was invented in 2023, in which L-arginine was incorporated into the RMGIC to inhibit microbial growth.41 These innovations highlight the active research on sustained oral health and effective patient-centric treatments. A schematic diagram showing the history and advancement of glass ionomer cement in the last few decades is presented in Fig. 2.
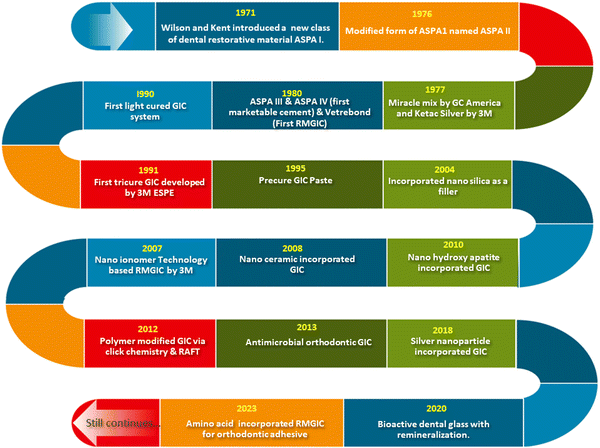 |
| Fig. 2 Schematic diagram representing the history and advances of glass ionomer cement. | |
1.2. Mechanism of cement formation in GIC
Polyalkenoic acid (PAA) plays a significant role in the formation of GIC. The cement-forming reaction consists of several stages that include (a) attack of protons of PAA by the glass powder, (b) release and migration of the liberated ions from the glass to the aqueous phase, (c) unwinding, or relaxation of the “twisted” polymer chains due to neutralization or ionisation of polyalkenoic acid, (d) ion binding between the charged polyalkenoic acid chains and glass powder, (e) gelation and (f) continuous ion binding that leads to the hardening of the cement.42 Different mechanisms of GIC formation are shown in Fig. 3.
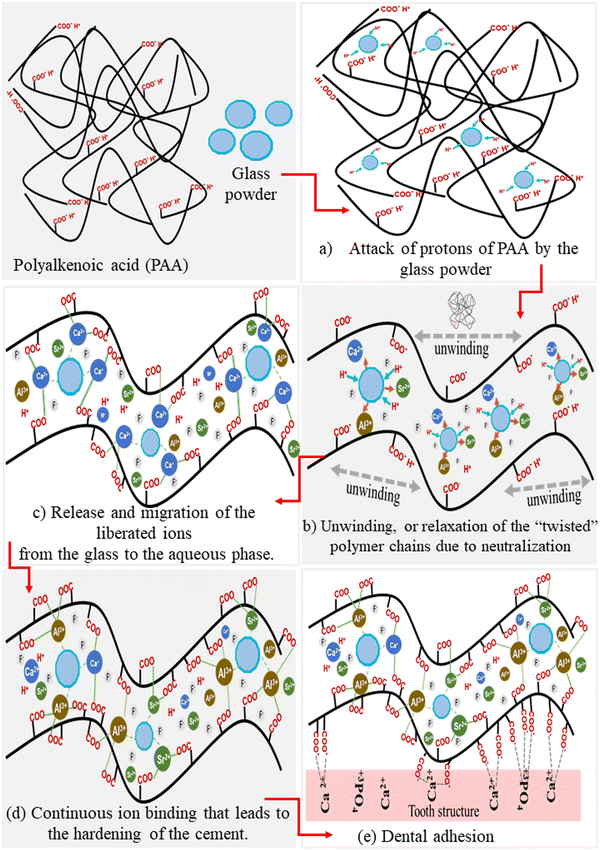 |
| Fig. 3 Different mechanisms for the formation of glass ionomer cement. | |
2. Polyalkenoic acid
Polymers rich in carboxylate ions that are used as liquid components of GIC are called polyalkenoic acids or polyacids. Polyalkenoic acids are either homopolymers of acrylic acid or copolymers of acrylic acid with unsaturated di- or tri-carboxylic acid (e.g. maleic acid, itaconic acid, and 2-butene-1,2,3-tricarboxylic acid) at different molar ratios.43 Chemical structures of these acids are shown in Fig. 4. Polyalkenoic acid played an important role in providing the strength and adhesion of GIC to the tooth structure. A homopolymer of acrylic acid (i.e., polyacrylic acid) with molecular weight ≥30 kDa results in gelation at high concentration.44 It also showed that inter/intramolecular hydrogen bonding at high concentration of polyacrylic acid leads to a very rigid matrix, which causes steric hindrance and limits the formation of salt bridges in GIC subsequently reducing its mechanical properties. Copolymerization of acrylic acid resolves this issue to a large extent as irregularly arranged copolymers of acrylic acid reduce inter/intramolecular hydrogen bonding within the polymer matrix.44–46 Atai et al.47 prepared poly(acrylic acid-co-itaconic acid) via a precipitation photopolymerization technique and studied the effect of its molecular weight and copolymer composition on the mechanical properties of GIC. The result showed that increasing the amount of itaconic acid improved the mechanical properties of GIC by providing more free spaces and carboxylic groups for reaction with calcium fluoroalumino silicate glass powder (A). In another study, Dandan zhou et al.48 demonstrated the effect of 4-pentenoic acid (PA) in the copolymer of acrylic acid and itaconic acid (AA-co-IA) in improving the mechanical properties of GIC. The result showed that 5 wt% PA in the polyalkenoic acid composition improved the flexural strength and compressive strength of GIC and the results were better than those of the commercial control (Fuji IX) and the experimental control (AA-co-IA).
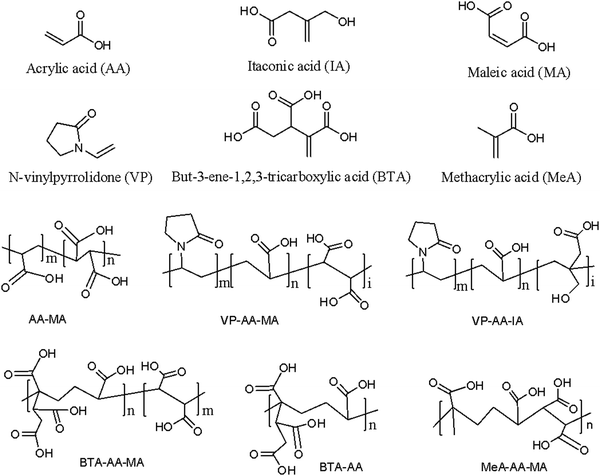 |
| Fig. 4 Chemical structures of common monomers and copolymers of different combinations of monomers used for the synthesis of glass ionomer cement. | |
2.1. Polymerisation techniques used for the synthesis of polyalkenoic acid
Different polymerisation techniques were used for the synthesis of polyalkenoic acid including conventional free radical polymerization (e.g. photopolymerisation and thermal polymerisation), living radical polymerization e.g. reversible addition–fragmentation transfer polymerization (RAFT) and atom transfer radical polymerisation (ATRP).49–51 Microwave-assisted polymerization is also effective for the synthesis of polyalkenoic acid.52,53 Compared to conventional free radical polymerisation, living radical polymerization techniques help to achieve proper control over the molecular weight and the molecular weight distribution of the polymer.54 Here polymer radicals do not terminate via an inherent termination reaction and polymerization continued anytime by the addition of monomers. The ability to control the polymerization reaction can result in in the synthesis of polymers having desired characteristics that are specific to particular applications, which are crucial for various applications in dentistry.
The molecular weight and molecular weight distribution of polyalkenoic acid play a major role in the properties of glass ionomer cement. The molecular weight of polyalkenoic acid influences a wide range of clinically relevant parameters, including setting time, working time, solubility, acid erosion, compressive strength, flexural strength, toughness, fracture toughness, and abrasive wear rate of GIC.44 For example, increasing the molecular weight of polyalkenoic acid increases solution viscosity that reduces the working time of GIC. However, varying the molecular weight of polyalkenoic acid in GIC has no effect on the chemistry of the setting process as the setting reaction takes place with individual acid groups on the polymer chain. A positive correlation between the molecular weight of polyalkenoic acid and the mechanical properties of GIC was reported in many studies. However, the molecular weight has no significant influence on Young's modulus or on hardness at higher molecular weights (>105). At low molecular weights, free volume considerations become important, and the molecular weight of the polymer influences the hardness and modulus via a reduction in the glass transition temperature. Wilson et al. demonstrated the effect of molecular weight of polyalkenoic acid on different characteristics of GIC using commercially available polyalkenoate acids of molecular weight ranging from 11.5 kDa to 1080 kDa.45 The study demonstrated that high molecular weight polyalkenoic acid increases the mechanical properties of GIC.45 Fleming et al. demonstrated the influence of the number average molecular weight of polyalkenoic acid on the compressive fracture strength (σ) and modulus (E) of GIC using polyacrylic acid with molecular weights ranging from 5000 to 200
000. The result showed that as the number average molecular weight increased, σ the E value of GIC also increased and peaked at 40
000–80
000 Da. Both σ and E values decreased as the number average molecular weight increased further to 200
000 Da.55
2.1.1. Conventional free radical polymerisation for the synthesis of polyalkenoic acid.
Free radical polymerization is one of the simple and most useful chemistries widely used in the production of dental biomaterials.49 In free radical polymerization, successive addition of free radicals (initiator free radicals/monomer free radicals/oligomer free radicals/prepolymer free radicals) takes place forming polymer chains. During the beginning stage of GIC, gelation of polyacrylic acid at higher concentration was a major challenge. Different techniques were used to resolve the gelation issue of polyacrylic acid that include copolymerisation of acrylic acid. A free radical solution polymerisation technique is a simple and widely used technique for the copolymerisation of acrylic acid using different monomers such as itaconic acid,49 maleic acid,56 vinyl pyrrolidine57etc. Initially, Crips et al. developed a composition of poly(acrylic acid-co-itaconic acid) and reported improved GIC characteristics.32 In the study, poly(acrylic acid-co-itaconic acid) was synthesised via a free radical solution polymerisation technique using propan-2-ol as the chain transfer agent. Culbertson et al. demonstrated the effect of N-vinyl pyrrolidone on the composition of polyalkenoic acid and showed that low levels of N-vinylpyrrolidone in polyalkenoic acid elevate the mechanical strength of GIC via reducing inter/intra molecular interactions between polyalkenoic acid chains.57 Dong et al. synthesised polyalkenoic acid via free radical polymerisation using a multiarm chain transfer agent.58 The study demonstrated that low viscous multi-arm polyalkenoic acid at high molecular weight leads to enhanced mechanical properties of GIC. As reported in many studies, molecular weight of polyalkenoic acid plays a very crucial role in the mechanical properties of GIC.30,44,59 However, it is difficult to control the molecular weight of polyalkenoic acid via conventional free radical polymerisation techniques.60,61
2.1.2. Living radical polymerisation (LRP) for the synthesis of polyalkenoic acid.
Living radical polymerization (controlled polymerization) techniques were introduced for the synthesis of polyalkenoic acid with controlled molecular weight and molecular weight distribution.62–64 Examples of LRP include atom transfer radical polymerisation (ATRP) and reverse addition fragmentation transfer polymerisation (RAFT). ATRP allows facile synthesis of styrenic and (meth)acrylate homopolymers and copolymers with predetermined degrees of polymerisation and narrow polydispersity. Dong Xie et al. developed 4 arm star shaped poly(acrylic acid) via the ATRP technique.58 The GIC formulated with the multi-arm polyacrylic acids showed significantly lower viscosities in water as compared to those formulated with their linear counterparts. Due to the lower viscosities, the molecular weight of the polymer can be significantly increased for achieving enhanced mechanical strength while maintaining the ease of mixing and handling. The results showed significantly improved compressive strength for GIC prepared using optimised multi-arm polyacrylic acid as compared to Fuji II after aging in water for 3 months. Xie et al.51 formulated resin-modified GIC using photo curable methacrylate tethered hyperbranched polyacrylic acid (HBPA). Here HBPA was synthesised via the ATRP technique using 2-(2-bromopropionyloxyl)ethyl acrylate as the initiator. The study demonstrated improved mechanical properties for the GIC formulated using hyperbranched polyacrylic acid compared to that of Fuji II LC as the control. The result showed that the compressive strength and diametrical tensile strength of experimental GIC were 53% and 125% higher than those of Fuji II LC. In another study Xie et al. demonstrated the effect of glycidyl methacrylate tethered 4-arm star-shape poly(acrylic acid) through ATRP for light-cured glass-ionomer cement (LCGIC).65 The LCGIC system showed 13% and 86% higher compressive strength, and diametrical tensile strength compared to Fuji II LC. RAFT polymerization is one of the most powerful techniques for preparing complex polymer architectures with low molecular weight distribution, predictable molecular weights, and high chain end-groups.51 One of the many benefits of RAFT polymerization is that it can be conducted in an aqueous solvent. The use of aqueous solvents allows fast polymerization kinetics as well as comes under the umbrella of green chemistry. However, an aqueous solvent can cause potential harm to the polymer, such as hydrolysis and aminolysis. Hence careful consideration of polymerization conditions is required for optimum control of the polymerization process. The application of RAFT agents thiocarbonylthio compounds for mediating radical polymerizations has occupied the forefront of precision polymer development across both industry and academia.66 Alireza et al. combined the RAFT polymerisation technique and click chemistry for the preparation of RMGIC.67 The study demonstrated that poly(acrylic acid-co-itaconic acid) containing alkyne groups was synthesised via the RAFT polymerisation technique and coupled with an azide terminated silane group modified glass powder via click chemistry. The results of working and setting times demonstrated that the combination of RAFT and click chemistry for the preparation GIC can be used successfully. However, the study did not show favourable mechanical properties in comparison to the control group. The RAFT-mediated self-condensing vinyl polymerization technique is a promising tool that gives hyper branched polymers with precise control over the branching structure of the polymers. Besides, it is easy to incorporate additional functionalities at the branch points and the chain ends. Furthermore, this technique allows for the additional decoration via post-polymerization modifications, and the tuneable branching frequency will be adjusted to yield desired polymers.68 Wetzel et al. investigated the impact of polyacrylic acid characteristics such as linear and branched polymers on the setting and mechanical properties of glass ionomer cement. The study showed that branched polyacrylic acid with higher molecular weight resulted in fast setting properties and increased compressive strength, similar to the behaviour of linear polyacrylic acid.69
2.2. Green chemistry route for the synthesis of polyalkenoic acid
In addition to the development of GIC, polyalkenoic acid has become an integral part of various industries associated with dentistry due to their biocompatibility and adhesive properties. The current focus on sustainability has encouraged the scientific community to explore more green pathways for the synthesis of polymers or other biomaterials of interest, which can reduce the environmental hazards without affecting the quality or inherent properties of the system. Not much work has been reported specifically on green chemistry routes for the synthesis of polyalkenoic acid. However, conventional polyalkenoic acid synthesis involved water based reactions and in most cases high reaction efficacy is ensured within 4 to 6 h. The purification step is also very simple as it involved rotary evaporation of the reaction mixture followed by freeze drying. Green chemistry route is followed in the synthesis of polyalkenoic acid since its invention. Recent studies showed that researchers around the globe have claimed green synthesis routes for GIC using different additives such as carbohydrates,70,71 organic acids72–74 and other plant-based75–77 materials to enhance its properties. For example, Dola Sundeep et al. investigated the effect of silver nanoparticles synthesized from the Mangifera indica leaves and studied the antimicrobial effect against Escherichia coli and Staphylococcus aureus at different concentrations in GIC.78 According to their study, this greener approach to synthesize silver nanoparticles containing GIC showed effective antimicrobial activities. David Aguilar-Perez et al.79 studied the effect of green synthesized copper nanoparticles in GIC on potential microbials such as Streptococcus mutants and Streptococcus sanguine and human dental pulp fibroblasts (HDPFs).
2.3. Effects of the concentration of polyalkenoic acid on GIC
The concentration of polyalkenoic acid has a major role in determining the strength of GIC. The theory of reptation model considers individual polymer chains and states. Each polymer chain is forced to wriggle in an anisotropic curvilinear motion, called “reptation” at high concentration of the polymer solution that leads to dense chain entanglements. Crips et al. studied the effect of working time and mechanical strength of GIC with respect to the concentration of polyalkenoic acid.32 The study showed that increased polyalkenoic acid concentration accelerates the cement forming reaction rate. It was also reported that an increase in the concentration of polyalkenoic acid increases its viscosity thereby restricting the appropriate mixing of glass powder and polyalkenoic acid solution. Viscosity increase will adversely affect the cement forming reaction. Most of the commercial GIC formulations use ∼45–50 wt% solutions of polyalkenoic acid. The viscosity of polyalkenoic acid can be controlled by optimising the concentration of the solution based on its molecular weight or by optimising the molecular weight of the polymer based on the required concentration. In both the cases, the effect of water during the preparation of GIC should be considered. An optimum amount of water is needed for the proper setting reaction and to attain desired end properties. In general, the increased concentration of polyalkenoic acid resulted in improved matrix formation through the assimilation of metallic ions and formation of salt bridges. It was also postulated that increased polymer concentration leads to increased intra- and intermolecular interactions resulting in compressed macromolecular chains and hence decreased formation of salt bridges.80–82
2.4. Effects of molecular interactions of polyalkenoic acid on GIC
It was reported that inter/intramolecular interactions of polyalkenoic acid reduce the extent of formation of salt bridges in GIC. Carboxylic acid groups are very closely attached in the base composition of polyalkenoic acid in the conventional GIC e.g. polyacrylic acid or poly(acrylic acid-co-itaconic acid/maleic acid).83 During the cement forming reaction, carboxyl groups of polyalkenoic acids are converted to carboxylate ions to form inter- or intramolecular Al3+ tricarboxylate/Ca2+ dicarboxylate complexes. The negative charge on the polyalkenoic acid chain drastically increased while most of the carboxylic acid groups were ionised. It leads to strong bonding of hydrogen atoms in the remaining un-ionised carboxyl groups and is not replaced by multivalent metal ions. In effect, the inter or intra molecular hydrogen bonding between polyalkenoic acid chains introduces steric hindrance to metal ions to react at carboxyl groups. This strong steric hindrance of metal ions to react with carboxyl groups thereby reduces the Ca2+/Al3+ di- and tri-carboxylate salt-bridges being formed in the set cement and reduces its mechanical properties.2,84,85
2.5. Effects of additives in polyalkenoic acid in improving the properties of GIC
The presence of different additives in polyalkenoic acid formulations plays a major role in tailoring different properties of GIC via interfering with different cement forming mechanisms. Chelating agents extract metal ions from glass powder and thereby suppress the ionization and unwinding of polyalkenoic acid which prolongs the working time and ensures the build-up of metal ions in polyalkenoic acid solutions for crosslinking. Different studies showed that the acidic chelating agents have multiple advantages. Commonly used chelating agents are tartaric acid,86,87 oxalic acids,88 phosphoric acid and citric acids.89 Stereochemistry of tartaric acid plays a major role in the chelation process. Different types of tartaric acids (e.g. (+)-tartaric acid, (−)-tartaric acid and (±)-tartaric acid) being used as chelating agents in GIC. Among them (+)-tartaric acid is most effective in manipulating the working time and setting time of GIC. Chemical structures of different stereo isomers of tartaric acid are shown in Fig. 5. Although there are many complexing agents used to manipulate the setting reaction of GIC, none has the unique combination effects exhibited by (+)-tartaric acid. As (+)-tartaric acid is a stronger acid than polyalkenoic acid, it enhances the decomposition of the glass powder and subsequently chelates the metal ions released. After a certain point in the reaction, (+)-tartaric acid can no longer bind with more metal ions, which then become available for the crosslinking of polyalkenoic acid. Hill et al. studied the effects of various additives on the setting of the glass-ionomer cement by means of cone and plate viscometry.90 It was demonstrated that low concentrations of (+)-tartaric acid accelerated the development of viscosity of the cement paste, while high concentrations retarded it. At intermediate concentrations, (+)-tartaric acid had an interesting, uniquely favourable effect on setting characteristics. Tartaric acid induces a lag period in the setting process at the beginning, during which the viscosity of the cement paste remains constant. This lag period is followed by a sharp, almost exponential, increase in viscosity. Thus, (+)-tartaric acid is found to have a dual effect on the setting reaction, i.e., first inhibits the gelation and then accelerates the setting time. The sharpening of setting curves also helps the hardening of the cement which reflects in its mechanical properties such as compressive strength and diametrical tensile strength.
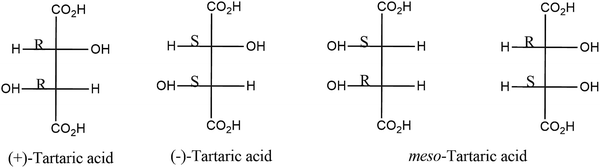 |
| Fig. 5 Different stereoisomers of tartaric acid. | |
Nicolson et al. studied the role of tartaric acid in GIC using Fourier transform infra-red (FT-IR) spectroscopy.91 The study supports the initial reaction of (+)-tartaric acid with the glass powder. Prosser et al. used C13 nuclear magnetic resonance (NMR) spectra to study the effect of tartaric acid on GIC.92 The study demonstrated that metal cations can be complexed faster with tartaric acid than polyalkenoic acid. These metal cations may either come directly from the glass powder or may originate from metal tartrate. One of the important findings of the study which is contradictory to previous reports/literature is that pK1 and pK2 values for the meso-tartaric acid are greater than those of (+)-tartaric acid and (−)-tartaric acid. Therefore, meso-tartaric acid would be expected to enhance the setting characteristics of GIC to some extent. In conclusion, chelating agents in the cement matrix can facilitate the extraction of metal ions from the glass powder and introduce a small delay in the crosslinking of polyalkenoic acid chains with cations present in the glass powder that subsequently helps in increasing the working time and attaining a sharp setting time for the conventional GIC. In another study Permana et al. demonstrated the effects of dicarboxylic acids such as tartaric acid and oxalic acid on the compressive strength of GIC.86 The study reported an optimum compressive strength for GIC in the presence of 10% (w/w) of both the dicarboxylic acids. However, compared to oxalic acid containing GIC, significant enhancement in compression strength was seen in tartaric acid added GIC. Prentice et al. investigated the influence of oxalic acid on different parameters of GIC such as the initial setting time, working time, hardness and compressive strength.93 At a concentration greater than 1% (w/w), oxalic acid showed a significant effect on the working and initial setting time of GIC. However, the study also showed that more than 7% (w/w) oxalic acid significantly reduced the hardness of the corresponding GIC samples. Another study investigated the influence of different concentrations of phosphoric acid on GIC (0–7% (w/w)). The study demonstrated a significant improvement in mechanical properties by the addition of 2% (w/w) phosphoric acid and after that there was a reduction in its mechanical properties.94
2.6. Effects of chemical compositions of polyalkenoic acid in improving the properties of GIC
The chemical composition of polyalkenoic acid plays a major role in different properties of GIC. As shown in the GIC forming mechanism, carboxylic acid groups in polyalkenoic acid facilitate cement forming reactions and dental adhesions. Higher concentrations of free carboxyl groups in polyalkenoic acid lead to improved mechanical and biological properties. However, higher concentrations of carboxyl groups also led to gelation via inter or intramolecular hydrogen bonding of polyalkenoic acid chains, thereby interfering with cement forming reactions and subsequently modulating different properties of GIC. This can be avoided by adding a short spacer molecule in each polymer chains. Towards this strategy different modifications of polyalkenoic acid chains were investigated. Kao et al. incorporated N-acryl glutamic acid into poly(acrylic acid-co-itaconic acid).95 The polymeric chain with a copolymer of acrylic acid, itaconic acid and N-acryl glutamic acid resulted in a significant improvement in mechanical properties such as compressive strength, diametrical tensile strength, and surface hardness. Amino acid modifications of polyalkenoic acid were reported with improved mechanical properties of GIC. Xie et al. demonstrated the use of methacrylate and acrylate modified photocurable amino acid derivatives of glutamic acid and beta-alanine for the synthesis of polyalkenoic acid.96 Among different derivatives of amino acid modified polyalkenoic acid investigated, poly(methacryloyl glutamic acid-co-acryloyl beta-alanine) showed a higher mechanical strength. Wu et al. modified polyalkenoic acid with six different amino acids and investigated their surface and mechanical properties.97 Amino acid modified GIC showed higher mechanical properties than commercially available control. Xie et al. developed a biocompatible monomer, methacryloyl-beta alanine, and used it as a replacement for 2-hydroxyethyl methacrylate (HEMA) in RMGIC.98 The study demonstrated an improvement in the mechanical properties of methacryloyl-beta alanine modified GIC compared to the control.
2.7. Biocompatibility
Biocompatibility of GIC has been studied extensively. Brodbeck et al. demonstrated that polyalkenoic acid could prevent the failure of implanted biomedical devices by limiting macrophage fusion and monocyte adhesion in vivo using the rat cage implant system.99 No studies reported the systemic toxicity of GIC. However, a freshly mixed GIC can be toxic. It may be attributed to the high acidity of freshly prepared GIC (pH 1.6–3.7) vs. completely set GIC (pH 5.4–7.3) and the release of metal ions such as aluminium and fluoride and/or free polyalkenoic acid at the initial stage. In vitro cytocompatibility of conventional GIC (CGIC) has been investigated by different research groups. It was shown that cytotoxic behaviour of CGIC depends on its setting reactions. Sultan Gulce Iz et al. studied the cytocompatibility of GIC with and without chlorhexidine and those coated with varnish.100 The results showed that among the tested GIC, Fuji IX covered with varnish was the most biocompatible. The addition of chlorhexidine lowered the cell viability considerably. The study also supports the use of varnish to prevent water loss and reduce the cytotoxicity of GIC. The biocompatibility of commercially available glass ionomer cement reinforced with cellulose microfibers or cellulose nanocrystals in rats was investigated. Three test specimens of each cement were implanted in the dorsal subcutaneous tissue of 15 rats. After 7, 30, and 60 days, the animals were slaughtered and histopathological analyses were done. All the samples tested positive for biocompatibility. Among them GIC with cellulose nanocrystals demonstrated tissue regeneration potential with more fibroblasts and less macrophages and mononuclear cells at 7 days and a thinner fibrous tissue capsule at 60 days. However, instances of negative clinical feedback were also reported. For example, Steinbrunner et al. revealed that very thin mixtures of CGIC, when used as a pit and fissure sealant, generated more pronounced tissue reactions than a thick mixture of the same product when used as a filling material.101 Their study discussed the exposure of pulp to CGIC and severe pulp reactions including abscess formation following the exposure. CGIC used as luting agents caused severe pain in certain cases. The possible causes of these clinical reactions can be incorrect handling (e.g. pronounced drying of the prepared tooth prior to cementation), insufficient dentin thickness, excessive pressure during cementation or increased solubility resulting from the inhibited setting reaction.
2.8. Dental tissue adhesion
Glass ionomer cement is a self-adhesive material that adheres to dental tissues such as dentin and enamel without prior treatment due to the formation of ionic bonds (linkages formed by electrostatic attraction between oppositely charged ions) between the carboxyl groups (COO−) of polyalkenoic acid in GIC and the Ca2+ ions present in the hydroxyapatite crystals of the tooth. It was also reported that GIC bonds better in enamel compared to dentine because of the higher inorganic content in enamel. Many studies demonstrated the mechanism of adhesion via different methods.102,103 Smith et al. used infra-red spectroscopy to demonstrate enhanced dental adhesion of GIC and showed that carboxylate groups of polyalkenoic acid chelate with Ca2+ ions in hydroxyapatite to improve adhesion.104 Ionic interactions of carboxylate groups of polyalkenoic acid and the tooth surface were also studied using spectroscopic methods. Wilson et al. demonstrated the ability of metal ions to form a salt bridge between pendent carboxylate groups in polyalkenoic acid and negatively charged appetite in the surface of enamel for enhanced dental adhesion.105 Another study investigated the presence of excess phosphate and calcium ions in the surface layer of adhering cement that diffuse through the enamel surface. It can be attributed to the ability of polyalkenoic acid to cross the interface and interact with the surface layer of the enamel. Van Meerbeek et al.106 illustrated an interlocking mechanism between the self-etching effect of polyalkenoic acid and the hydroxyl apatite coated collagen fibril network of dentine to explain the enhanced adhesion of GIC. Different factors must be considered for improving the adhesion of GIC including surface wetting, micromechanical interlocking and improved ionic bonding between the carboxylate group of polyalkenoic acid and calcium ions in the hydroxyapatite surface, and the material must have comparable strength to enamel or dentine it replaces to prevent material failure and proper mixing of the cement to avoid the formation of air voids as they act as point of stress and result in reduced adhesive strength of GIC to the tooth surface.
3. Effects of antimicrobial activity of polyalkenoic acids on GIC
The chemical compositions of polyalkenoaic acid play a major role in the antimicrobial activities of GIC. Polyalkenoic acids show distinctive ion exchange characteristics and alter the setting properties of the GIC significantly to prevent the fostering environment which is hostile to microbial proliferation. Polyalkenoic acid enhances the dental adhesion to the tooth structure and facilitates the controlled release of different ions such as fluoride, aluminium, etc., which provide potential antimicrobial effects. This controlled ion-releasing mechanism maintained the cement matrix disrupting the bacterial metabolic pathways effectively and hindering the formation of biofilms. Márjully Eduardo et al. studied the antimicrobial effects of GIC that contains sodium trimetaphosphate (TMP) nanoparticles and chlorhexidine diacetate.107 The study showed that 1.25% of chlorhexidine diacetate and 14% of TMP increased the antibiofilm activity of GIC against S. mutans. In another study, Zahra Jowkar et al. investigated the antimicrobial effects of different nanoparticles such as silver, titanium dioxide, zinc oxide, etc. in GIC.108 The study showed the use of nanoparticles after the conditioner increased the micro shear bond strength (μSBS) values for the clinical practice because of the enhanced antibacterial properties. E. A. Elshenawy et al. demonstrated the effect of quaternized chitosan-coated mesoporous silica nanoparticles on GIC.109 An increased bacterial inhibition zone and fluoride release kinetics of GIC were reported. Table 2 summarises different modifications of polyalkenoic acid and glass powder to improve the mechanical and antimicrobial properties of GIC.
Table 2 Different modifications of polyalkenoic acid and glass powder to improve the mechanical and antimicrobial properties of GIC
Study details |
Antimicrobial activities |
Ref. |
Polyalkenoic acid was modified using cinnamon and thyme essential oils. GIC prepared using the modified polyalkenoic acid formulations was evaluated for its mechanical, fluoride release and antimicrobial activities |
The modified polyalkenoic acid in GIC introduced significantly higher inhibitory effects against both Streptococcus mutans and Candida albicans growth. Compressive strength (CS) of 5% cinnamon-modified GIC (MPa = 160.32 ± 6.66) showed no significant difference when compared to unmodified GIC (MPa = 165.7 ± 5.769) |
D. I. Sherief et al.110 |
N-Vinylcaprolactam (NVC) modified poly alkenoic acid and glass powder modified with yttria-stabilized nano zirconia were studied |
Microhardness and flexuaral strength of the GIC were improved in the presence of zirconia and NVC |
S. Aslani et al.111 |
The effects of different essential oils (2.5%) such as bergamot, peppermint, lemongrass and fennel incorporated polyalkenoic acid formulations on GIC were evaluated |
Mechanical properties of GIC were reduced in the presence of essencial oil modified polyalkenoic acid. However, antimicrobial properties were improved. Bergamot modified GIC recorded the highest mean inhibition zone |
M. E. Elkorashy et al.112 |
α-Lipoic acid-capped silver nanoparticles (AgNPs) in polyalkenoic acid formulations were prepared and their effect on the corresponding GIC were evaluated |
Polyalkenoate-AgNP-modified GIC exhibited significant antibiofilm activities and mechanical properties |
G. C. Porter et al.113 |
The antimicrobial activities of GIC (Fuji IX, Ketac Molar, and d-tech) in different bacterial strains were evaluated |
The zones of inhibition for Fuji IX, Ketac Molar, and d-tech on S. mutans were found to be 10.84 ± 0.22 mm, 10.23 ± 0.15 mm, and 15.65 ± 0.31 mm, respectively. Whereas, those for L. acidophilus were found to be 10.43 ± 0.12 mm, 10.16 ± 0.11 mm, and 15.57 ± 0.13 mm, respectively. |
R. G. Naik et al.114 |
The anti-microbial activities of GIC (Fuji IX) with different additives such as chlorhexidine, propolis and chitosan were studied |
All the formulations showed antimicrobial activities. However, GIC with chlorhexidine sowed higher antimicrobial activities for both S. mutans and L. acidophilus strains |
B. Neelima et al.115 |
Quaternary ammonium salt (QAS) modified polyalkenoic acid in acid GIC were evaluated |
The effects of the substitute chain length, and the grafting ratio of QAS on polyalkenoic acid for their antibacterial and mechanical properties were investigated |
D. Xie et al.116 |
Effects of quaternized chitosan coated nanoparticles on GIC |
Bacterial inhibition zones and fluoride release increased proportionally to the amount of filler added. Mechanical properties were improved |
E. A. Elshenawy et al.109 |
The study aimed to develop antibacterial GIC containing a quaternary ammonium salt (dimethylaminododecyl methacrylate, DMADDM) to improve the antimicrobial properties |
GIC containing DMADDM improved the physicochemical and antibacterial properties. Modified GIC improved the management of secondary caries |
S.-P. Wang et al.117 |
Evaluation of the effect of magnesium oxide nanoparticles (MgO) on the antibacterial and antibiofilm formation of GIC (3M ESPE Ketac Molar) |
The study showed promising antibacterial effect of MgO nanoparticles (MIC = 500 μg ml−1 and MBC = 1000 μg ml−1) on cariogenic microorganisms such as Streptococcus mutans and Streptococcus sobrinus |
A. J. Noori et al.118 |
The copper nanoparticle (CuNP) incorporated GIC (Fuji IX, GC) was prepared and evaluated for its antibacterial activity against oral cavity strains |
2–4% of CuNPs in GIC showed antimicrobial potential against Streptococcus mutans and Streptococcus sanguinis |
Aguilar-Perez et al.119 |
3.1. Computational studies and polyalkenoic acid
The development of glass ionomer cement with improved physicochemical properties is critical for the potential outcomes of dental restorations. Computational studies are a promising tool for predicting and designing novel formulations with intended properties. Computer simulations, modelling, and data analysis are promising tools to better understand the chemical and physical interactions of different components of GIC and their behaviour under various circumstances. These studies can speed up the discovery of different polyalkenoic acid formulations and glass powder without the need for intensive laboratory testing, which can be time-consuming and expensive. Gaviria et al. conducted a computational study of polyalkenoic acid formulation in glass-ionomer cement using three different components including acrylic acid and itaconic acid, as well as a spacer molecule.120 The study demonstrated the behaviour of 15 distinct spacer molecules and optimised the structural arrangement to improve the stability of polyalkenoic acid via the Gaussian 90 computational package using density functional theory. The results demonstrated that in addition to acting as spacer molecules, amino acid derivatives can establish energy interactions with itaconic acid and acrylic acid present in polyalkenoic acid formulation and lead to compaction and energetic stabilisation of the structure. Multiple computational studies have elucidated the multifaceted effects of polyalkenoic acids on glass ionomer cement as detailed in Table 3.
Table 3 Details of different computational studies that demonstrated the multifaceted effects of polyalkenoic acids on glass ionomer cement
Name of the computational study |
Details of the study |
Ref. |
Ab initio molecular dynamics simulations |
The study aims to understand the atomic scale structural and dynamic contributions of different elements in bioactive glass ionomer cement. It also identifies multiple factors affecting the mechanical shortcomings of GIC. Understanding the structural and microscopic details of the cement lead to potential improvements in its design and properties |
K. V. Tian et al.121 |
Density functional theory (DFT) |
The study focuses on computational modelling of the behaviour of polyalkenoic acids in GIC. It examines 15 different polyalkenoic acid formulations which contain acrylic acid, itaconic acid, amino acid derivatives and various spacer molecules to standardize the optimal composition. This is the first computational study that provides insight into the position of polyalkenoic acid and its interactions within the cement forming network |
J. Gaviria et al.122 |
Density functional theory (DFT) |
The research focuses on finding suitable molecules for glass-ionomer materials in three stages. It involves adding glycidyl methacrylate (GM) to polyacids and emphasizes the importance of this step in understanding thermodynamic feasibility. The study optimizes basic glass-ionomer structures using the most stable GM-added molecules and metal ions like Ca2+, Zn2+, and Al3+. Data showed that aluminium contributes to creating more compact and robust glass-ionomer cement |
J. Gaviria et al.123 |
Ab initio molecular dynamics, Density functional theory (DFT) |
The study evaluated the setting dynamics of two commercial glass ionomer cement over 24 hours using Terahertz (THz) spectroscopy and neutron scattering. It uncovered key physical interactions in cement setting, highlighting the importance of THz vibrations and interfacial aluminium atoms. Also studied the effect of disordered structure throughout for tetra, penta and hexa coordinated aluminium in glass ionomer cement. THz spectroscopy demonstrated its utility for real-time monitoring and assessing of THz dielectric parameter changes |
F. V. Song et al.124 |
3.2. Efficacy of GIC in clinical scenario
As discussed above glass ionomer cement has many advantages compared to other dental restorative materials because of its high biocompatibility and remineralisation potential. However, low mechanical properties exclude its use as long-term restorations in extensive lesions and restorations in load bearing areas. Many studies discussed the clinical performance of glass ionomer cement with modifications. In one of the studies, Olczak-Kowalczyk et al. performed a clinical assessment of GIC (Equia Fil from Ivoclar Vivadent) and a composite material (Tetric EvoCeram from Ivoclar Vivadent) in permanent teeth of young patients. A total of 100 cavities on approximal surfaces were filled with the composite material or GIC in 49 patients aged from 12.08 to 19.58 years. The clinical efficacy of Equia Fil and Tetric EvoCeram after two years were assessed to be 95.83%, and 100%, respectively. However, the difference was not statistically significant (P = 0.145).125 In another study, Heintze et al. evaluated the longevity of different materials for Class I and Class II restorations including glass ionomer cement. Multiple clinical trials having a total of 8181 restorations were tested. Among them 2121 and 238 restorations were of GIC and compomers, respectively. The study demonstrated a mean overall survival rate of 80% after 6 years for GIC and compomers together. According to the study, the significantly higher rate of replacement for RMGIC was mainly due to fracture, excessive wear or retention loss. The study also stated that the bulk fracture or chipping of class II restorations for RMGIC was mainly because of its low flexural strength (>80
MPa), which is the minimum value required by the ISO standard 1949 for posterior restorations. In addition, low compressive strength and microhardness also contributed to the high replacement rate.126
3.3. Life cycle assessment of GIC
Life cycle assessment (LCA) provides a robust methodology to determine the environmental impact of a material, product or service supported by the standard BS EN ISO 14040:2006. LCA is increasingly being used in dental healthcare to assess environmental impacts of dental equipment, clinical procedures and oral hygiene devices. There are only a few studies reported on life cycle assessment of dental restorative materials. In one of the studies, Smith et al. demonstrated the LCA of three different restorative materials (e.g. dental amalgam, resin-based composite and GIC) and their associated packaging.127 The study prefixed multiple inclusion and exclusion criteria. For example, patient travel, staff travel, procurement, energy, water, waste, and nitrous oxide were included in the study. However, the impact of capital items was excluded from the study. The results showed that a dental amalgam restoration had a carbon footprint of 14.8 kg CO2-eq, compared to 14.75 kg CO2-eq for a composite filling, while a GIC filling has a carbon footprint of only 8.6 kg CO2-eq.
4. Conclusion
Glass ionomer cement has a long and intriguing history in the field of dental materials because of unique features such as fluoride release, biocompatibility, and adherence to oral tissues. GIC creation includes an interaction between powder and liquid components, in which liquid components consist of concentrated polyalkenoic acid solution. Polyalkenoic acid plays an important role in GIC where its characteristics can be altered by varying the chemical compositions of polyalkenoic acid formulations. Different polymerisation techniques such as free radical polymerisation, live radical polymerisation, atom transfer radical polymerisation (ATRP), and reverse addition fragmentation transfer (RAFT) were tried to control the molecular weight and polydispersity index of polyalkenoic acid. Various parameters including molecular weight, molecular weight distribution, polyalkenoic acid concentration, molecular interactions and the presence of additives/chelating agents influence different characteristics of polyalkenoic acid formulations (liquid compositions) and thereby the physicochemical properties of GIC. Different modifications of polyalkenoic acid were implemented in multiple studies to improve the properties of GIC such as working time, setting time, compressive strength and diametrical tensile strength. The chemical structure of polyalkenoic acid also plays an important role in the biocompatibility and dental tissue adhesion of GIC. Computational studies for developing different structures of polyalkenoic acid to improve different properties of GIC are also promising.
5. Future scope
Glass ionomer cement has come a long way since its development in the 1970s, and its characteristics, performance, and clinical applications have improved with time. To fully realise the potential of GIC in dentistry, additional research and development are required in various areas. One of the most promising applications for glass ionomer cement in the future is as a bioactive material that actively drives tissue regeneration and repair. Incorporating growth factors, stem cells, or other biologically active substances into the cement matrix can accomplish this. This material has the potential to revolutionise the area of regenerative dentistry by providing novel treatment options for problems like periodontal disease, caries, and pulpitis. Glass ionomer cement may also be utilised in minimally invasive dentistry to rebuild and reinforce weakened or broken teeth without the need for major drilling or removal of healthy tissue in the future. This can be accomplished through inventing novel bonding and shaping processes for cement, as well as increasing its mechanical and aesthetic features. The potential application of glass ionomer cement in orthodontics, prosthodontics, and endodontics must be investigated. GIC has the potential to play an even larger role in the future of dentistry with continuous research and development activities.
Conflicts of interest
There are no conflicts to declare.
Acknowledgements
The authors gratefully acknowledge the Science and Engineering Research Board (SERB), Department of Science and Technology, Govt. of India (SRG/2020/001600 dated 4/12/2020) for the financial support.
References
- A. Alaohali, D. S. Brauer, E. Gentleman and P. T. Sharpe, A modified glass ionomer cement to mediate dentine repair, Dent. Mater., 2021, 37, 1307–1315, DOI:10.1016/j.dental.2021.05.003.
- J. W. Nicholson and B. Czarnecka, Review paper: Role of aluminium in glass-ionomer dental cements and its biological effects, J. Biomater. Appl., 2009, 24, 293–308, DOI:10.1177/0885328209344441.
- L. F. Francisconi, P. M. Scaffa, V. R. de Barros, M. Coutinho and P. A. Francisconi, Glass ionomer cements and their role in the restoration of non-carious cervical lesions, J Appl Oral Sci, 2009, 17, 364–369, DOI:10.1590/s1678-77572009000500003.
- S. Najeeb, Z. Khurshid, M. S. Zafar, A. S. Khan, S. Zohaib, J. M. N. Martí, S. Sauro, J. P. Matinlinna and I. U. Rehman, Modifications in glass ionomer cements: Nano-sized fillers and bioactive nanoceramics, Int. J. Mol. Sci., 2016, 17, 1134, DOI:10.3390/ijms17071134.
- A. D. Wilson, The chemistry of dental cements, Chem. Soc. Rev., 1978, 1978(7), 265, 10.1039/CS9780700265.
- J. W. McLean, Glass-ionomer cements, Br. Dent. J., 1988, 164(9), 293–300, DOI:10.1038/sj.bdj.4806434.
- J. Makanjuola and S. Deb, Chemically activated glass-ionomer cements as bioactive materials in dentistry: a review, Prosthesis, 2023, 5(1), 327–345, DOI:10.3390/prosthesis5010024.
- J. W. Nicholson, Adhesion of glass-ionomer cements to teeth: A review, Int. J. Adhes. Adhes., 2016, 69, 33–38, DOI:10.1016/j.ijadhadh.2016.03.012.
-
W. N. John
Glass-Ionomers in Dentistry (Book); The History and Background to Glass-Ionomer Dental Cements, 2016, pp. 1–24 Search PubMed.
- J. F. McCabe, Resin-modified glass-ionomers, Biomaterials, 1998, 19, 521–527, DOI:10.1016/S0142-9612(98)00132-X.
- Z. Malik, D. Qasim Butt, Z. Qasim Butt, N. Muhammad, M. Kaleem, S. Liaqat, M. Adnan Khan and A. Samad Khan, Evolution of Anticariogenic Resin-Modified Glass Ionomer Cements, ChemBioEng Rev., 2021, 8, 326–336, DOI:10.1002/cben.202100005.
- S. M. Mousavinasab, M. Khoroushi, F. Keshani and S. Hashemi, Flexural strength and morphological characteristics of resin-modified glass-ionomer containing bioactive glass, J. Contemp. Dent. Pract., 2011, 12, 41–46, DOI:10.5005/jp-journals-10024-1008.
- J. A. Williams, R. W. Billington and G. J. Pearson, A long term study of fluoride release from metal-containing conventional and resin-modified glass-ionomer cements, J. Oral Rehabil., 2001, 28, 41–47, DOI:10.1046/j.1365-2842.2001.00628.
- S. Garoushi, J. He, J. Obradovic, P. Fardim, P. K. Vallittu and L. Lassila, Incorporation of cellulose fiber in glass ionomer cement, Eur. J. Oral Sci., 2020, 128, 81–88, DOI:10.1111/eos.12668.
- M. E. Rusnac, C. Gasparik, A. I. Irimie, A. G. Grecu, A. Ş. Mesaroş and D. Dudea, Giomers in dentistry - at the boundary between dental composites and glass-ionomers, Med. Pharm. Rep., 2019, 92, 1–6, DOI:10.15386/mpr-1169.
- S. Sing, Advances in Glass Ionomer Cement: A Review, Indian J. Contemp. Dent., 2019, 7, 35–37, DOI:10.5958/2320-5962.2019.00021.4.
- S. M. Hugar, P. Kukreja, H. G. Assudani, V. Patil and P. Thakkar, Comparative Evaluation of the Antibacterial Efficacy of Type II Glass Ionomer Cement, Type IX Glass Ionomer Cement, and AMALGOMERTM Ceramic Reinforcement by Modified “Direct Contact Test”: An in vitro Study, Int. J. Clin. Pediatr. Dent., 2016, 9, 114–117, DOI:10.5005/jp-journals-10005-1345.
- A. Ivanišević, V. B. Rajić, A. Pilipović, M. Par, H. Ivanković and A. Baraba, Compressive Strength of Conventional Glass Ionomer Cement Modified with TiO2 Nano-Powder and Marine-Derived HAp Micro-Powder, Materials, 2021, 14, 4964, DOI:10.3390/ma14174964.
- T. Nishimura, Y. Shinonaga, C. Nagaishi, R. Imataki, M. Takemura, K. Kagami, Y. Abe, K. Harada and K. Arita, Effects of Powdery Cellulose Nanofiber Addition on the Properties of Glass Ionomer Cement, Materials, 2019, 12, 3077, DOI:10.3390/ma12193077.
- S. K. Sidhu, Glass-ionomer cement restorative materials: A sticky subject?, Aust. Dent. J., 2011, 56, 23–30, DOI:10.1111/j.1834-7819.2010.01293.x.
- T. Guo, M. Yang, D. Wang, J. Zheng and S. S. Gao, Antibiofilm and mechanical properties of silver nanowire-modified glass ionomer cement, J. Dent., 2023, 135, 104569, DOI:10.1016/j.jdent.2023.104569.
- K. Rabelo and A. Ellakwa, Mechanical properties of a resin-modified glass-ionomer cement reinforced with short-glass fiber flowable resin composites, Am. J. Dent., 2023, 36, 177–182 Search PubMed , PMID: 37587027.
- W. Potiprapanpong, P. Naruphontjirakul, C. Khamsuk, S. Channasanon, A. Toneluck, S. Tanodekaew, N. Monmaturapoj, A. M. Young and P. Panpisut, Assessment of Mechanical/Chemical Properties and Cytotoxicity of Resin-Modified Glass Ionomer Cements Containing Sr/F-Bioactive Glass Nanoparticles and Methacrylate Functionalized Polyacids, Int. J. Mol. Sci., 2023, 24, 10231, DOI:10.3390/ijms241210231.
- W. Thepveera, W. Potiprapanpong, A. Toneluck, S. Channasanon, C. Khamsuk, N. Monmaturapoj, S. Tanodekaew and P. Panpisut, Rheological Properties, Surface Microhardness, and Dentin Shear Bond Strength of Resin-Modified Glass Ionomer Cements Containing Methacrylate-Functionalized Polyacids and Spherical Pre-Reacted Glass Fillers, J. Funct. Biomater., 2021, 12, 42, DOI:10.3390/jfb12030042.
- F. Antonio Piola Rizzante, R. Schlögel Cunali, J. Fraga Soares Bombonatti, G. Maria Correr, C. Castiglia Gonzaga and A. Yoshio Furuse, Indications and restorative techniques for glass ionomer cement, RSBO Revista Sul-Brasileira de Odontologia, 2015, 12, 79–87, DOI:10.21726/RSBO.V12I1.183.
- V. V. Gordan, P. K. Blaser, R. E. Watson, I. A. Mjör, D. L. McEdward, L. G. Sensi and J. L. Riley, A clinical evaluation of a giomer restorative system containing surface prereacted glass ionomer filler: Results from a 13-year recall examination, J. Am. Dent. Assoc., 2014, 145, 1036–1043, DOI:10.14219/jada.2014.57.
- S. Ub, S. Ramesh and S. Pradeep, Fracture resistance of mesio-occlusal-distal cavities restored with composite, resin modified glass ionomer cement and zirconomer - An in vitro study, Int. J. Dent. Oral Sci., 2020, 7, 44–49, DOI:10.19070/2377-8075-SI02-010008.
- A. M. Saber, A. A. El-Housseiny and N. M. Alamoudi, Atraumatic restorative treatment and interim therapeutic restoration: A review of the literature, Dent. J., 2019, 7, 28, DOI:10.3390/dj7010028.
-
G. V. Black, A work on operative dentistry, Medico-Dental Publishing Company, NORTH WESTERN UNIVERSITY DENTAL SCHOOL, 1917, pp. 1836–1915. https://archive.org/details/workonoperativ01blac/page/n9/mode/2up Search PubMed.
- A. D. Wilson, S. Crisp and A. J. Ferner, Reactions in Glass-Ionomer Cements: IV. Effect of Chelating Comonomers on Setting Behavior, J. Dent. Res., 1976, 55, 489–495, DOI:10.1177/00220345760550033101.
- J. Makanjuola and S. Deb, Chemically Activated Glass-Ionomer Cements as Bioactive Materials in Dentistry: A Review, Prosthesis, 2023, 5, 327–345, DOI:10.3390/prosthesis5010024.
- S. Crisp, B. G. Lewis and A. D. Wilson, Characterization of glass-ionomer cements, J. Dent., 1980, 8, 68–74, DOI:10.1016/s0300-5712(80)80046-7.
- D. Xie, J. G. Park and J. Zhao, Synthesis and preparation of novel 4-arm star-shape poly (carboxylic acid) s for improved light-cured glass-ionomer cements, Dent. Mater., 2007, 23, 395–403 CrossRef CAS PubMed.
-
F. H. Wilson, C. Fails, T. G. Tire and R. Company, US Pat., TO 1C6, 1977, pp. 1–6. https://patents.google.com/patent/US4003160A/en Search PubMed.
- Z. Khurshid, M. Zafar, S. Qasim, S. Shahab, M. Naseem and A. AbuReqaiba, Advances in Nanotechnology for Restorative Dentistry, Materials, 2015, 8, 717–731, DOI:10.3390/ma8020717.
- G. K. Leung, A. W. Y. Wong, C. H. Chu and O. Y. Yu, Update on dental luting materials., Dentistry Journal, 2022, 10, 208 CrossRef PubMed.
- A. Moshaverinia, B. T. S. Thirumamagal and S. R. Schricker, Click Chemistry: A Potential Platform for Development of Novel Dental Restorative Materials, J. Macromol. Sci., Part A, 2012, 49(4), 288–292, DOI:10.1080/10601325.2012.662027.
-
M. Marchiori Farret, E. M. Santayanna de Lima, H. M. S. Oshima and E. G. MotaAqueous anti-microbial solution containing chlorhexidine digluconate and tartaric acid, method for preparing same, and anti-microbial, orthodontic cement, Patent application filing No. US2013/0303622A1, United States Patent and Trademark Office, 2012 Search PubMed.
- G. C. Porter, G. R. Tompkins, D. R. Schwass, K. C Li, J. N. Waddell and G. C. Meledandri, Anti-biofilm activity of silver nanoparticle-containing glass ionomer cements., Dent. Mater., 2020, 36, 1096–1107, DOI:10.1016/j.dental.2020.05.001.
-
A. Moshaverinia, M. M. Hasani-Sadrabadi and T. L. Aghaloo, Bioactive Dental Restotrative Material with Remineralization Properties, 2019, https://patents.google.com/patent/US10667994B2/en?oq=US+10%2c667%2c994+B2 Search PubMed.
-
Y. Ma, Y. Bai, N. Zhang, H. Gao, X. Wu and H. Yang, Application of L-arginine in Preparation of Orthodontic Adhesive Composition, 2023, https://patents.google.com/patent/CN116747143A/en?oq=CN+116747143+A Search PubMed.
- S. Vasiliu, S. Racovita, I. A. Gugoasa, M.-A. Lungan, M. Popa and J. Desbrieres, The Benefits of Smart Nanoparticles in Dental Applications, Int. J. Mol. Sci., 2021, 22, 2585, DOI:10.3390/ijms22052585.
- A. M. F. Alhalawani, D. J. Curran, D. Boyd and M. R. Towler, The role of poly(acrylic acid) in conventional glass polyalkenoate cements, J. Polym. Eng., 2016, 36, 221–237, DOI:10.1515/polyeng-2015-0079.
- A. D. Wilson, R. G. Hill, C. P. Warrens and B. G. Lewis, The Influence of Polyacid Molecular Weight on Some Properties of Glass-ionomer Cements, J. Dent. Res., 1989, 68, 89–94, DOI:10.1177/00220345890680021401.
- A. D. Wilson, S. Crisp and G. Abel, Characterization of glass-ionomer cements 4. Effect of molecular weight on physical properties, J. Dent., 1977, 5, 117–120, DOI:10.1016/0300-5712(77)90070-7.
- R. G. Hill, A. D. Wilson and C. P. Warrens, The influence of poly(acrylic acid) molecular weight on the fracture toughness of glass-ionomer cements, J. Mater. Sci., 1989, 24, 363–371, DOI:10.1007/BF00660982.
- R. Abbasi, A. Nodehi and M. Atai, Synthesis of poly(acrylic-co-itaconic acid) through precipitation photopolymerization for glass-ionomer cements: Characterization and properties of the cements, Dent. Mater., 2020, 36, e169–e183, DOI:10.1016/j.dental.2020.03.006.
- D. Zhou, F. Liu and J. He., Improving the Mechanical Properties of Conventional Glass Ionomer Cement Through Using 4-Pentenoic Acid Modified Polycarboxylic Acid (PCA), J. Macromol. Sci., Part B, 2022, 61, 584–594, DOI:10.1080/00222348.2022.2078142.
- L. Sollka and K. Lienkamp, Progress in the Free and Controlled Radical Homo- and Co-Polymerization of Itaconic Acid Derivatives: Toward Functional Polymers with Controlled Molar Mass Distribution and Architecture., Macromol. Rapid Commun., 2021, 42, 2000546, DOI:10.1002/marc.202000546.
- J. Ji, L. Jia, L. Yan and P. R. Bangal, Efficient synthesis of poly(acrylic acid) in aqueous solution via a RAFT process, J. Macromol. Sci., Part A: Pure Appl.Chem., 2010, 47, 445–451, DOI:10.1080/10601321003659705.
- J. Zhao and D. Xie, A novel hyperbranched poly(acrylic acid) for improved resin-modified glass-ionomer restoratives, Dent. Mater., 2011, 27, 478–486, DOI:10.1016/j.dental.2011.02.005.
- P. N. Upadhya, K.K. Srinivasan, A. V. Adhikari and L. N. Satapathy, Evaluation of Calcium Fluoroaluminosilicate Based Glass Ionomer Luting Cements Processed Both by Conventional and Microwave Assisted Methods, Technologies, 2015, 3, 58–73, DOI:10.3390/technologies3020058.
- S. Sözügeçer and N. P. Bayramgil, Preparation and characterization of polyacrylic acid-hydroxyapatite nanocomposite by microwave-assisted synthesis method, Heliyon, 2021, 7, e07226, DOI:10.1016/j.heliyon.2021.e07226.
- F. H. Isikgor and C. R. Becer, Lignocellulosic biomass: a sustainable platform for the production of bio-based chemicals and polymers, Polym. Chem., 2015, 6, 4497–4559, 10.1039/c5py00263j.
- A. H. Dowling and G. J. Fleming, The influence of poly(acrylic) acid number average molecular weight and concentration in solution on the compressive fracture strength and modulus of a glass-ionomer restorative, Dent. Mater., 2011, 27, 535–543, DOI:10.1016/j.dental.2011.02.003.
- John W. Nicholson and Fareesa Abiden, Changes in compressive strength on ageing in glass polyalkenoate (glass-ionomer) cements prepared from acrylic/maleic acid copolymers, Biomaterials, 1997, 18, 59–62, DOI:10.1016/S0142-9612(96)00109-3.
- B. M. Culbertson, New polymeric materials for use in glass-ionomer cements, J. Dent., 2006, 34, 556–565, DOI:10.1016/j.jdent.2005.08.008.
- D. Xie, J. Zhao and Y. Weng, Synthesis and application of novel multi-arm poly(carboxylic acid)s for glass-ionomer restoratives, J. Biomater. Appl., 2010, 24, 419–436, DOI:10.1177/0885328208098593.
- J. W. Nicholson, Maturation processes in glass-ionomer dental cements, Acta Biomater. Odontol. Scand., 2018, 4, 063–071, DOI:10.1080/23337931.2018.1497492.
- L. Brocken, P. D. Price, J. Whittaker and I. R. Baxendale, Continuous flow synthesis of poly(acrylic acid) via free radical polymerisation, React. Chem. Eng., 2017, 2, 662–668, 10.1039/c7re00063d.
- A. M. F. Alhalawani, D. J. Curran, D. Boyd and M. R. Towler, The role of poly(acrylic acid) in conventional glass polyalkenoate cements, J. Polym. Eng., 2016, 36, 221–237, DOI:10.1515/polyeng-2015-0079.
- N. P. Truong, G. R. Jones, K. G. E. Bradford, D. Konkolewicz and A. Anastasaki, A comparison of RAFT and ATRP methods for controlled radical polymerization, Nat. Rev. Chem., 2021, 5, 859–869, DOI:10.1038/s41570-021-00328-8.
- R. Abbasi, A. Nodehi and M. Atai, Synthesis of poly(acrylic-co-itaconic acid) through precipitation photopolymerization for glass-ionomer cements: Characterization and properties of the cements, Dent. Mater., 2020, 36, e169–e183, DOI:10.1016/j.dental.2020.03.006.
- C. Ladaviere, N. Dörr and J. Claverie, Controlled Radical Polymerization of Acrylic Acid in Protic Media, Macromolecules, 2001, 34, 5370–5372, DOI:10.1021/ma010358v.
- D. Xie, J. Zhao and J. G. Park, A novel light-cured glass-ionomer system for improved dental restoratives, J. Mater. Sci.: Mater. Med., 2007, 18, 1907–1916, DOI:10.1007/s10856-007-3100-z.
- M. D. Nothling, Q. Fu, A. Reyhani, S. Allison-Logan, K. Jung, J. Zhu, M. Kamigaito, C. Boyer and G. G. Qiao, Progress and Perspectives Beyond Traditional RAFT Polymerization, Adv. Sci., 2020, 7, 2001656, DOI:10.1002/advs.202001656.
- A. Moshaverinia, B. T. S. Thirumamagal and S. R. Schricker, Click chemistry: A potential platform for development of novel dental restorative materials, J. Macromol. Sci., Part A: Pure Appl.Chem., 2012, 49, 288–292, DOI:10.1080/10601325.2012.662027.
- J. A. Alfurhood, P. R. Bachler and B. S. Sumerlin, Hyperbranched polymers via RAFT self-condensing vinyl polymerization, Polym. Chem., 2016, 3361–3369, 10.1039/C6PY00571C.
- R. Wetzel, O. Eckardt, P. Biehl, D. S. Brauer and F. H. Schacher, Effect of poly(acrylic acid) architecture on setting and mechanical properties of glass ionomer cements, Dent. Mater., 2020, 36, 77–386, DOI:10.1016/j.dental.2020.01.001.
- S. Al-Mofty, N. Elghazawy and H. Azzazy, A one-step facile process for extraction of cellulose from rice husk and its use for mechanical reinforcement of dental glass ionomer cement, RSC Sustainability, 2023, 1, 1743–1750 RSC.
- da S. Freitas, D. de França, S. P. Cestari and L. C. Mendes, Natural and synthetic fillers for reaching high performance and sustainable hybrid polymer composites., Hybrid Polymer Composite Materials Processing (WoodHead Publishing), 2017, 157–171, DOI:10.1016/B978-0-08-100789-1.00007-1.
- J. O. Makanjuola, E. R. Essien, B. O. Bolasodun, D. C. Umesi, O. H. Oderinu, L. A. Adams and W. L. Adeyemo, A new hydrolytic route to an experimental glass for use in bioactive glass-ionomer cement, J. Mater. Res. Technol., 2018, 18, 2013–2024, DOI:10.1016/j.jmrt.2022.03.094.
- M. D. Moda, P. H. Dos Santos, N. I. P. Pini, L. N. Furini, A. L. F. Briso, A. Assmann and T. C. Fagundes, Effects of Different Toothpastes on the Nanomechanical Properties and Chemical Composition of Resin-Modified Glass Ionomer Cement and Composite Resin Restorations, Dent. J., 2023, 11, 173, DOI:10.3390/dj11070173.
- B. M. B. de Oliveira, I. E. Agostini, M. L. Baesso, R. Menezes-Silva, A. F. S. Borges, M. F. L. Navarro, J. W. Nicholson, S. K. Sidhu and R. C. Pascotto, Influence of external energy sources on the dynamic setting process of glass-ionomer cements, Dent. Mater., 2019, 35, 450–456, DOI:10.1016/j.dental.2019.01.003.
- C. H. C. Saraceni, F. Kabadayan, B. Lima, R. Braga, B. Cunha and I. Suffredini, Mechanical properties of a glass ionomer cement incorporated with Amazon plant extract, Dent. Mater. J., 2019, 38, 411–417, DOI:10.4012/dmj.2018-064.
- I. C. Pinto, J. B. Seibert and L. S. Pinto,
et al., Preparation of glass-ionomer cement containing ethanolic Brazilian pepper extract (Schinus terebinthifolius Raddi) fruits: chemical and biological assays, Sci. Rep., 2020, 10, 22312, DOI:10.1038/s41598-020-79257-3.
- L. Singer, G. Bierbaum, K. Kehl and C. Bourauel, Evaluation of the antimicrobial activity and compressive strength of a dental cement modified using plant extract mixture, J. Mater. Sci.: Mater. Med., 2020, 31, 116, DOI:10.1007/s10856-020-06455-w.
- D. Sundeep, T. Vijaya Kumar, P. S. S. Rao, R. V. S. S. N. Ravikumar and A. Gopala Krishna, Green synthesis and characterization of Ag nanoparticles from Mangifera indica leaves for dental restoration and antibacterial applications, Prog. Biomater., 2017, 6, 57–66, DOI:10.1007/s40204-017-0067-9.
- D. Aguilar-Perez, R. Vargas-Coronado, J. M. Cervantes-Uc, N. Rodriguez-Fuentes, C. Aparicio, C. Covarrubias, M. Alvarez-Perez, V. Garcia-Perez, M. Martinez-Hernandez and J. V. Cauich-Rodriguez, Antibacterial activity of a glass ionomer cement doped with copper nanoparticles, Dent. Mater. J., 2020, 39, 389–396, DOI:10.4012/dmj.2019-046.
- W. D. Cook, Dental polyelectrolyte cements III. Effect of additives on their rheology, Biomaterials, 1983, 4, 85–88, DOI:10.1016/0142-9612(83)90045-5.
- R. G. Riley, J. D. Smart, J. Tsibouklis, P. W. Dettmar, F. Hampson, J. A. Davis, G. Kelly and W. R. Wilber, An investigation of mucus/polymer rheological synergism using synthesised and characterised poly(acrylic acid), Int. J. Pharm., 2001, 217, 87–100, DOI:10.1016/S0378-5173(01)00592-0.
- Y. Wang, R. A. Pethrick, N. E. Hudson and C. J. Schaschke, Rheology of poly(acrylic acid): A model study, Ind. Eng. Chem. Res., 2012, 51, 16196–16208, DOI:10.1021/ie302313a.
- R. Abbasi, A. Nodehi and M. Atai, Synthesis of poly(acrylic-co-itaconic acid) through precipitation photopolymerization for glass-ionomer cements: Characterization and properties of the cements, Dent. Mater., 2020, 36, e169–e183, DOI:10.1016/j.dental.2020.03.006.
- T. De Caluwé, C. W. J. Vercruysse, S. Fraeyman and R. M. H. Verbeeck, The influence of particle size and fluorine content of aluminosilicate glass on the glass ionomer cement properties, Dent. Mater., 2014, 30, 1029–1038, DOI:10.1016/j.dental.2014.06.003.
- A. D. Wilson, Alumino-silicate polyacrylic acid and related cements, Br. Polym. J., 1974, 6, 165–179, DOI:10.1002/pi.4980060303.
- A. J. Permana, H. Setyawati, H. Hamami and I. K. Murwani, The influence of dicarboxylic acids: Oxalic acid and tartaric acid on the compressive strength of glass ionomer cements, AIP Conf. Proc., 2016, 1718, 1–7, DOI:10.1063/1.4943317.
- S. Crisp, B. G. Lewis and A. D. Wilson, Characterization of glass-ionomer cements 5. The effect of the tartaric acid concentration in the liquid component, J. Dent., 1979, 7, 304–312, DOI:10.1016/0300-5712(79)90143-X.
- L. H. Prentice and M. Tyas, The effect of oxalic acid incorporation on the setting time and strength of a glass-ionomer cement, Acta Biomater., 2006, 2, 109–112, DOI:10.1016/j.actbio.2005.08.007.
- A. D. Wilson, B. E. Kent and R. F. Batchelor, Dental Silicate Cements: IV. Phosphoric Acid Modifiers, J. Dent. Res., 1968, 47, 233–243, DOI:10.1177/00220345680470020801.
- E. De Barra and R. Hill, Influence of poly(acrylic acid) content on the fracture behaviour of glass polyalkenoate cements, J. Mater. Sci., 1998, 33, 5487–5497, DOI:10.1023/A:1004479108367.
- J. W. Nicholson, P. J. Brookman, O. M. Lacy and A. D. Wilson, Fourier Transform Infrared Spectroscopic Study of the Role of Tartaric Acid in Glass-ionomer Dental Cements, J. Dent. Res., 1988, 67, 1451–1454 CrossRef CAS PubMed.
- H. J. Prosser, C. P. Richards and A. D. Wilson, NMR spectroscopy of dental materials. II. The role of tartaric acid in glass–ionomer cements, J. Biomed. Mater. Res., 1982, 16, 431–445, DOI:10.1002/jbm.820160411.
- L. H. Prentice, M. Tyas and M. F. Burrow, The effect of oxalic acid incorporation on the setting time and strength of a glass-ionomer cement, Acta Biomater., 2006, 2, 109–112, DOI:10.1016/j.actbio.2005.08.007.
- L. H. Prentice, M. J. Tyas and M. F. Burrow, The effects of boric acid and phosphoric acid on the compressive strength of glass-ionomer cements, Dent. Mater., 2006, 22, 94–97, DOI:10.1016/j.dental.2005.04.004.
- E. C. Kao, B. M. Culbertson and D. Xie, Preparation of glass ionomer cement using N-acryloyl-substituted amino acid monomers--evaluation of physical properties, Dent. Mater., 1996, 12, 44–51, DOI:10.1016/S0109-5641(96)80063-7.
- D. Xie, J. G. Park, M. Faddah and J. Zhao, Preparation, formulation and evaluation of novel photo-cured glass ionomers based on co-polymers of (meth)acrylated amino acids, J. Biomater. Sci., Polym. Ed., 2006, 17, 303–322, DOI:10.1163/156856206775997304.
- W. Wu, D. Xie, A. Puckett and J. W. Mays, Synthesis of amino acid-containing polyacids and their application in self-cured glass-ionomer cement, Eur. Polym. J., 2003, 39, 959–968, DOI:10.1016/S0014-3057(02)00308-7.
- D. Xie, I. D. Chung, W. Wu, J. Lemons, A. Puckett and J. Mays, An amino acid-modified and non-HEMA containing glass-ionomer cement, Biomaterials, 2004, 25, 1825–1830, DOI:10.1016/j.biomaterials.2003.08.033.
- W. G. Brodbeck, J. Patel, G. Voskerician, E. Christenson, M. S. Shive, Y. Nakayama, T. Matsuda, N. P. Ziats and J. M. Anderson, Biomaterial adherent macrophage apoptosis is increased by hydrophilic and anionic substrates in vivo, Appl. Biol. Sci., 2002, 99, 10287–10292 CAS.
- S. G. Iz, F. Ertugrul, E. Eden and S. I. D. Gurhan, Biocompatibility of glass ionomer cements with and without chlorhexidine, Eur. J. Dent., 2013, 7, 89–93, DOI:10.4103/1305-7456.119083.
- R. Steinbrunner, J. Setcos and A. Kafrawy, Connective tissue reactions to glass ionomer cements and resin composites, Am. J. Den., 1992, 4, 281–284, DOI:10.1073/pnas.162124199.
- D. R. Powis, T. Follerås, S. A. Merson and A. D. Wilson, Improved adhesion of a glass ionomer cement to dentin and enamel, J. Dent. Res., 1982, 61, 1416–1422, DOI:10.1177/00220345820610120801.
- L. Cury and J. A. Tenuta, Enamel remineralization: controlling the caries disease or treating early caries lesions? Dental caries: the disease and its signals Enamel remineralization, Braz. Oral Res., 2009, 23, 23–30 CrossRef PubMed.
- D. C. Smith, Medical and dental applications of nanomedicines, J. Biomed. Res. Sympos., 1971, 1, 189–205, DOI:10.1111/apm.12890.
- J. Ellis, M. Anstice and A. D. Wilson, The glass polyphosphonate cement: A novel glass-ionomer cement based on poly(vinyl phosphonic acid), Clin. Mater., 1991, 7, 341–346, DOI:10.1016/0267-6605(91)90079-U.
- J. Perdigão, Current perspectives on dental adhesion:(1) Dentin adhesion–not there yet., 56(1), 190-207., Japanese Dental Science Review, 2020, 56, 190–207, DOI:10.1016/j.jdsr.2020.08.004.
- M. E. R. da Silva, M. Danelon, J. A. Santos Souza, D. F. Silva, J. A. Pereira, D. Pedrini, E. R. de Camargo, A. C. Botazzo Delbem and C. Duque, Incorporation of chlorhexidine and nano-sized sodium trimetaphosphate into a glass-ionomer cement: Effect on mechanical and microbiological properties and inhibition of enamel demineralization, J. Dent., 2019, 84, 81–88, DOI:10.1016/j.jdent.2019.04.001.
- Z. Jowkar, Z. Fattah, S. Ghanbarian and F. Shafiei, The Effects of Silver, Zinc Oxide, and Titanium Dioxide Nanoparticles Used as Dentin Pretreatments on the Microshear Bond Strength of a Conventional Glass Ionomer Cement to Dentin, Int. J. Nanomed., 2020, 15, 4755–4762, DOI:10.2147/IJN.S262664.
- E. A. Elshenawy, M. A. El-Ebiary, E. R. Kenawy and G. A. El-Olimy, Modification of glass-ionomer cement properties by quaternized chitosan-coated nanoparticles, Odontology, 2023, 11, 328–341, DOI:10.1007/s10266-022-00738-0.
- D. I. Sherief, M. S. Fathi and R. K. Abou El Fadl, Antimicrobial properties, compressive strength and fluoride release capacity of essential oil-modified glass ionomer cements-an in vitro study, Clin. Oral Investig., 2021, 25, 1879–1888, DOI:10.1007/s00784-020-03493-0.
- S. Aslani, H. Rezaie and A. Khavandi, Effects of N-Vinylcaprolactam Containing Polyacids and Zirconia on Mechanical Properties of Commercial Glass Ionomer Cements, J. Dent. Mater. Techn., 2020, 9, 43–50, DOI:10.22038/jdmt.2020.43842.1316.
- M. Elkorashy, Essential Oil Modification of Glass Ionomer Cement: Antibacterial Activity and Compressive Strength, Egypt. Dent. J., 2022, 68, 12, DOI:10.21608/edj.2022.140174.2118.
- G. C. Porter, G. R. Tompkins, D. R. Schwass, K. C. Li, J. N. Waddell and C. J. Meledandri, Anti-biofilm activity of silver nanoparticle-containing glass ionomer cements, Dent. Mater., 2020, 36, 1096–1107, DOI:10.1016/j.dental.2020.05.001.
- R. G. Naik, A. S. Dodamani, M. R. Khairnar, H. C. Jadhav and M. A. Deshmukh, Comparative assessment of antibacterial activity of different glass ionomer cements on cariogenic bacteria, Restor. Dent. Endod., 2016, 41, 278–282, DOI:10.5395/rde.2016.41.4.278.
- B. Neelima, J. S. Reddy, P. T. Singh, K. Suhasini, I. Hemachandrika and S. Hasanuddin, Comparative evaluation of antimicrobial efficacy of glass ionomer cement added with propolis, chitosan, and chlorhexidine against Streptococcus mutans and Lactobacillus acidophilus: An in vitro study, J. Indian Soc. Pedod. Prev. Dent., 2020, 38, 367–373, DOI:10.4103/JISPPD.JISPPD_322_20.
- D. Xie, Y. Weng, X. Guo, J. Zhao, R. L. Gregory and C. Zheng, Preparation and evaluation of a novel glass-ionomer cement with antibacterial functions, Dent. Mater., 2011, 27, 487–496, DOI:10.1016/j.dental.2011.02.006.
- S. P. Wang, Y. Ge, X. D. Zhou, H. H. Xu, M. D. Weir, K. K. Zhang, H. H. Wang, M. Hannig, S. Rupf, Q. Li and L. Cheng, Effect of anti-biofilm glass-ionomer cement on Streptococcus mutans biofilms, Int. J. Oral Sci., 2016, 8, 76–83, DOI:10.1038/ijos.2015.55.
- A. J. Noori and F. A. Kareem, The effect of magnesium oxide nanoparticles on the antibacterial and antibiofilm properties of glass-ionomer cement, Heliyon, 2019, 5, e02568, DOI:10.1016/j.heliyon.2019.e02568.
- D. Aguilar-Perez, R. Vargas-Coronado, J. M. Cervantes-Uc, N. Rodriguez-Fuentes, C. Aparicio, C. Covarrubias and J. V. Cauich-Rodriguez, Antibacterial activity of a glass ionomer cement doped with copper nanoparticles, Dent. Mater. J., 2020, 39, 389–396, DOI:10.4012/dmj.2019-046.
- J. Gaviria, J. Quijano and P. Ruiz, Computational Study of the Formation Reaction of Polyacid Precursors of Glass-Ionomer Materials, J. Mater. Sci. Chem. Eng., 2021, 9, 1–9, DOI:10.4236/msce.2021.98001.
- K. V. Tian, G. A. Chass and D. Di Tommaso, Simulations reveal the role of composition into the atomic-level flexibility of bioactive glass cements, Phys. Chem. Chem. Phys., 2016, 18, 837–845, 10.1039/c5cp05650k.
- J. Gaviria, J. Quijano and P. Ruiz, Computational Study of the Formation Reaction of Polyacid Pre-cursors of Glass-Ionomer Materials, J. Mater. Sci. Chem. Eng., 2021, 9, 1–9, DOI:10.4236/msce.2021.98001.
- J. Gaviria, S. Quijano, J. Quijano and P. Ruiz, Computational Study of the Relative Stability of Some Glass-Ionomer Cement-Forming Molecules, J. Mol. Model., 2022, 28, 333, DOI:10.1007/s00894-022-05211-x.
- F. Song, B. Yang, D. T. Devis, R. Donnan, G. Chass, R. Yada, D. Farrar and K. Tian, Resolving nanoscopic structuring and interfacial THz dynamics in setting cements, Mater. Adv., 2022, 3, 4982–4990, 10.1039/d1ma01002f.
- P. Rożniatowski, E. Korporowicz, D. Gozdowski and D. Olczak-Kowalczyk, Clinical study on resin composite and glass ionomer materials in II class restorations in permanent teeth, J. Clin. Exp. Dent., 2021, 13(2), e165–e171, DOI:10.4317/jced.57572.
- S. D. Heintze, A. D. Loguercio, T. A. Hanzen, A. Reis and V. Rousson, Clinical efficacy of resin-based direct posterior restorations and glass-ionomer restorations–An updated meta-analysis of clinical outcome parameters, Dent. Mater., 2022, 38, e109–e135, DOI:10.1016/j.dental.2021.10.018.
- L. Smith, M. Ali, M. Agrissais, S. Mulligan, L. Koh and N. Martin, A comparative life cycle assessment of dental restorative materials, Dent. Mater., 2023, 39, 13–24, DOI:10.1016/j.dental.2022.11.007.
|
This journal is © The Royal Society of Chemistry 2024 |