DOI:
10.1039/D0MA00302F
(Paper)
Mater. Adv., 2020,
1, 1622-1630
Supramolecular liquid crystals exhibiting a chiral twist-bend nematic phase†
Received
12th May 2020
, Accepted 1st July 2020
First published on 2nd July 2020
Abstract
A selection of novel, chiral supramolecular liquid crystals formed by hydrogen-bonding are reported and their phase properties characterised by polarised optical microscopy, X-ray diffraction and resonant soft X-ray diffraction. Their behaviour is compared to that of their achiral analogues. The binary mixture of the bent-shaped, non-mesogenic H-bond acceptor 1OB6OS and chiral H-bond donor (S)-(2-Me)4OBA exhibits chiral nematic (N*) and chiral smectic A (SmA*) phases. Doping the twist-bend nematogenic (NTB) complex 1OB6OS:4OBA with (S)-(2-Me)4OBA in ternary mixtures induces a transition from the N* phase to the chiral twist-bend nematic phase N*TB, the first example seen in a supramolecular liquid crystal. The binary mixture of CB6OS and (S)-(2-Me)4OBA exhibits N* and N*TB phases, with the helical structure of the latter having a periodicity of approximately 2 complex lengths (∼8 nm). The nature of the hydrogen bond is reversed in a binary mixture of the bent-shaped, twist-bend nematogen CB6OBA with (S)-(2-Me)4OBA, and this exhibits the phase sequence N*–N*TB–SmC*–SmX.
Introduction
The twist-bend nematic phase, NTB, has been a topic of very significant interest for the liquid crystal community since its experimental discovery in 2011,1 many years after its initial prediction by Meyer in 19762 and later independently by Dozov in 2001.3 Unlike the chiral nematic (cholesteric) phase, in which the director twists around an axis perpendicular to the long axes of the molecules, the director in the NTB phase is tilted at a constant angle θ < 90° with respect to the helix axis and precesses on a cone forming an oblique helix of pitch about two orders of magnitude shorter than that typically found in the N* phase, and equal to just a few molecular lengths.4 The NTB phase can be considered as a generalised case of the chiral nematic phase and has been described as the ‘structural link’ between the N and N* phases.4 Molecular curvature is widely considered to be the essential prerequisite for the formation of the NTB phase and the vast majority of twist-bend nematogens are odd-membered liquid crystal dimers (see, for example ref. 5–12). Undoubtedly the most fascinating feature of the NTB phase is that its chiral superstructure is formed despite its constituent molecules being chemically achiral, and indeed the NTB phase represents the first example of such spontaneous chiral symmetry breaking in a fluid system with no long-range positional order. As the induction of chirality is spontaneous, there exists an equal probability of the formation of either handedness of helix and hence the NTB phase consists of doubly degenerate locally chiral domains of opposite handedness, giving a globally achiral (racemic) phase. This raises the intriguing question of how the NTB phase, having spontaneous structural chirality, will respond at a microscopic level to the presence of molecular chirality. This has been theoretically addressed by Meyer, who extended the elastic instability model of the NTB phase to consider the effect of a chiral field introduced by chiral doping.13 This model suggests the removal of the double degeneracy of the chiral domains, with the new ground state of the NTB phase being the domains with the same handedness as the dopant. With increasing chiral doping, the conical angle in this favoured helical state increases, while that in the unfavoured helical state decreases and eventually the more favoured handedness dominates. The helical pitch is essentially unchanged. Thus, chiral doping is predicted to increase the stability of the NTB phase. Another theoretical model including the effects of molecular chirality was described by Longa and Tomczyk, who used minimum coupling Landau–de Gennes theory to reveal the possibility of a polar, chiral NTB phase.14 At present, only a handful of systems exhibiting the transition between chiral nematic and twist-bend nematic phases have been observed experimentally. These have included mixtures of twist-bend nematogens with a chiral dopant15,16 as well as intrinsically chiral materials, with the latter being particularly rare.17,18 Recently, we reported the first comparison between racemic and chiral versions of the same twist-bend nematogens and found that TN(*)TB–N(*) was higher for the chiral dimers than their racemic counterparts, in accord with Meyer's model.18
The preparation of supramolecular mesogens via hydrogen bonding is a flexible and efficient alternative to covalent bonding, and a convenient way of adding functionality to a molecule in an effective and controllable manner.19 This is especially useful for introducing chirality to a system as it avoids the risk of partial racemisation during the synthetic process. An early example of supramolecular liquid crystal systems was reported in the 1950s by Jones and Gray, who described the thermal behaviour of the 4-alkoxybenzoic acids. The mesogenic behaviour was attributed to the formation of calamitic-like, symmetric homomeric complexes of two equivalent benzoic acid fragments with a supramolecular core formed via hydrogen bonding.20 This approach was extended in 2015 to the formation of the first supramolecular twist-bend nematogen CB6OBA (see Table 1), in which two acid dimers hydrogen-bond to form a bent supramolecular trimer.21
Table 1 Hydrogen bond acceptors and hydrogen bond donors used in this work, and the heterocomplexes formed. ‘1OB’ denotes a biphenyl mesogenic unit terminated with a methoxy group; ‘CB’ a cyanobiphenyl moiety; ‘6O’ a hexyloxy flexible spacer; ‘S’ a 4-stilbazole unit; ‘BA’ a benzoic acid moiety; ‘(S)-(2-Me)4O’ a 2-methylbutoxy chain and ‘4O’ a butoxy terminal chain
H-bond acceptors |
H-bond donors |
|
|
|
|
|
|
Heterocomplexes formed by H-bonding |
|
|
We have previously reported the thermal behaviour of equimolar mixtures containing the hydrogen bond acceptors: 1OB6OS, CB6OS and nOS with different lengths of terminal alkoxy chain, n, and the hydrogen bond donors, the extensively studied 4-alkoxybenzoic acids, nOBAs, and CB6OBA, the structures of which are shown in Table 1.22–24 These complexes were the first examples of how molecular recognition between unlike H-bond donor and acceptor fragments can induce spontaneous chirality in a condensed phase, and formed the twist-bend nematic phase despite the components being either non-mesogenic (1OB6OS, CB6OS, nOS) or exhibiting only conventional nematic or smectic phases (nOBAs). The hydrogen bond between pyridyl-based fragments (S) and benzoic acids (BA) is strong enough to promote liquid crystalline complexes25 and the molecular curvature required for NTB phase behaviour is imparted by the flexible hexyloxy (6O) spacer of the longer fragment.26
Here, we combine our investigations on chiral and supramolecular twist-bend nematogens, and report the effect of molecular chirality in hydrogen-bonded mixtures containing a chiral branched terminal chain in place of the alkyl chains (n) in the nOBA and nOS series, specifically (S)-2-methylbutyl, which is denoted herein as (S)-(2-Me)4. The structures of the H-bonded heterocomplexes formed in these mixtures are also illustrated in Table 1.
Experimental
Synthesis and analytical data
1OB6OS,24 CB6OS22 and CB6OBA21,23 were synthesised by methods reported elsewhere. The alcoholic stilbazole 4-[(E)-2-(pyridin-4-yl)ethenyl]phenol (HOS) was prepared through the base-catalysed condensation of 4-hydroxybenzaldehyde and 4-methylpyridine.27 This was combined with the relevant bromoalkane in a Williamson ether synthesis to give the alkoxy-terminated stilbazole compounds 4OS and (S)-(2-Me)4OS.23 Butoxybenzoic acid was purchased from Sigma Aldrich and purified for use in mixtures by recrystallisation from ethanol (10 mL g−1). (S)-2-methylbutoxybenzoic acid ((S)-(2-Me)4OBA) was synthesised by a standard method reported elsewhere.22,28 Analytical data are given in the ESI.†
Polarised optical microscopy (POM)
POM measurements were performed using an Olympus BH2 polarising light microscope equipped with a Linkam TMS 92 hot stage (University of Aberdeen) or a Zeiss Axio Imager A2m polarizing microscope equipped with a Linkam heating stage (University of Warsaw). Glass cells obtained from Warsaw Military University of Technology (WAT) with thicknesses of 1.6 or 3 microns, with ITO transparent electrodes and polymer aligning layers were used.
Differential scanning calorimetry (DSC)
The thermal behaviour of the final products and the binary mixtures was investigated using a Mettler Toledo DSC1 differential scanning calorimeter (University of Aberdeen) equipped with a TSO 801RO sample robot and calibrated using indium and zinc standards. The temperature scan profile in all cases, unless otherwise stated, was heat, cool and reheat at 10 K min−1 under nitrogen, with a 3-minute isotherm between heating and cooling segments. Thermal data were extracted from the second heating trace unless otherwise stated.
X-Ray diffraction (XRD)
Small-angle X-ray diffraction (SAXS) patterns for powder samples were obtained with a Bruker Nanostar system using CuKα radiation and patterns were collected with an area detector VANTEC2000 (University of Warsaw). The temperature of the sample was controlled with a precision of ±0.1 K. Samples were prepared either in thin-walled glass capillaries or as droplets on a heated surface. Wide-angle diffractograms (WAXS) were obtained with a Bruker D8 GADDS system (CuKα line, Goebel mirror, point beam collimator, VANTEC2000 area detector) (University of Warsaw).
Binary/ternary mixtures
Binary and ternary mixtures were prepared by co-dissolving pre-weighed amounts of each compound in dichloromethane or chloroform and allowing the solvent to evaporate slowly at room temperature. The mixtures were further dried in a vacuum oven at 50 °C for ∼16–20 h. Binary phase diagrams were constructed and, where necessary, used to estimate a virtual temperature for transitions precluded from direct observation by crystallisation on cooling, by extrapolation of the relevant trendline.
Results and discussion
4OBA and (S)-(2-Me)4OBA
The chiral alkoxybenzoic acid, (S)-(2-Me)4OBA did not show liquid crystalline behaviour. However, on heating from the crystalline solid a reversible transition to a disordered, or “soft” crystal phase (SCr) was seen prior to clearing. In order to first predict virtual mesogenic behaviour for the chiral acid, and also assess the effects of a chiral branch on the liquid crystallinity of supramolecular systems, binary mixtures of the nematogenic non-chiral 4OBA29,30 and chiral (S)-(2-Me)4OBA were prepared and their thermal behaviour is summarised in Table 2 along with those of the pure acids. For all mixtures containing chiral acid, the chiral nematic phase was seen in place of the conventional nematic, identifiable by its characteristic oily streak texture, shown in Fig. 1. For mixtures containing over 40 mol% of (S)-(2-Me)4OBA, the same soft crystal phase (SCr) is also present, initially visible only by supercooling the chiral nematic phase (40–60 mol% (S)-(2-Me)4OBA) but enantiotropic for >60 mol%. The almost linear dependence of the TSCrN* line on the binary phase diagram, see Fig. 2, indicates that there is good miscibility of the mixture components in the SCr phase, contrary to the behaviour normally observed in conventional crystal phases.
Table 2 Transition temperatures (T, °C) and associated enthalpy changes (ΔH, kJ mol−1) for the 4OBA:(S)-(2-Me)4OBA binary mixtures
mol% (S)-(2-Me)4OBA |
MP |
SCr |
|
N* |
|
I |
[] denotes a virtual temperature. Temperature obtained from the DSC cooling trace. |
100 (S)-(2-Me)4OBA |
105 (3.55) |
● |
108 (12.44) |
● |
[106] |
● |
90 |
96 (18.31) |
● |
110 (12.81) |
● |
110 (3.10) |
● |
80 |
86 (18.18) |
● |
110 (10.51) |
● |
117 (3.07) |
● |
70 |
81 (18.06) |
● |
109 (10.56) |
● |
122 (3.05) |
● |
60 |
114 (12.77) |
● |
111a (10.92) |
● |
130 (3.03) |
● |
50 |
114 (14.05) |
● |
113a (10.85) |
● |
134 (3.01) |
● |
40 |
124 (14.92) |
● |
117a (11.00) |
● |
141 (2.33) |
● |
30 |
133 (11.98) |
● |
|
● |
146 (2.43) |
● |
20 |
138 (15.01) |
● |
|
● |
151 (2.58) |
● |
10 |
144 (17.31) |
● |
|
● |
156 (2.93) |
● |
0 (4OBA) |
147 (17.87) |
● |
|
● |
160 (2.64) |
● |
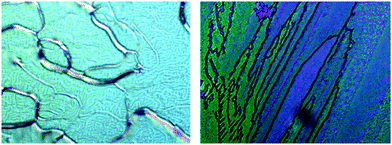 |
| Fig. 1 POM textures obtained for a 40 : 60 4OBA : (S)-(2-Me)4OBA mixture in the chiral nematic phase (fingerprint and oily streak texture) (left) and soft crystal phase (right). | |
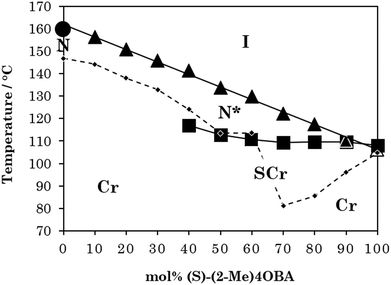 |
| Fig. 2 Phase diagram of binary mixtures of 4OBA and (S)-(2-Me)4OBA, where ◆ represents the melting point, ● TNI, ▲ TN*I, and ■ TSCrN/I. An unfilled triangle represents a virtual transition temperature. | |
These data are collected in a phase diagram in Fig. 2. TN(*)I varies in essentially a linear manner on varying the composition of the mixture, and decreases rather steeply on increasing the concentration of (S)-(2-Me)4OBA – dropping by over 50 K between the two pure acids. The TN(*)I trendline was extrapolated to 100 mol% (S)-(2-Me)4OBA to estimate a virtual TN*I of 106 °C for the chiral acid. This behaviour is in accord with the change in TN(*)I seen on comparing other branched and unbranched molecular pairs and is attributed to the methyl branch disrupting the interactions between the mesogenic units.
1OB6OS:4OBA/(S)-(2-Me)4OBA
The bent-shaped, supramolecular complex formed by 1OB6OS and 4OBA exhibits conventional nematic and twist-bend nematic phases. A third mesophase is observed below the NTB phase, denoted the SmX phase; its monotropic nature precluded detailed analysis but it was suggested to be a highly ordered smectic phase.22,24 An equimolar mixture of the chiral benzoic acid, (S)-(2-Me)4OBA – here considered to be the chiral “analogue” of 4OBA – with 1OB6OS does not form the NTB phase, instead a SmA* phase is observed below the N* phase. These assignments are made based on textural observations, an oily streak texture and focal conic fans in coexistence with homeotropic regions, characteristic of the chiral nematic and smectic A* phases, respectively. In order to assess the effects of the branch on the phases present in the 1OB6OS:4OBA mixture, a series of ternary mixtures were prepared containing the H-bond acceptor 1OB6OS and both H-bond donor acids. The concentration of 1OB6OS was kept constant at 50 mol% and the relative amounts of 4OBA and (S)-(2-Me)4OBA were varied to maintain the remaining 50 mol%, keeping the total numbers of pyridyl and acid groups equal. The transitional properties of these mixtures are given in Table 3.
Table 3 Transition temperatures (T, °C) and associated enthalpy changes (ΔH, kJ mol−1) for the 1OB6OS:4OBA/(S)-(2-Me)4OBA ternary mixtures

|
mol% 4OBA |
MP |
SmX |
|
SmA |
|
N*TB |
|
N* |
|
I |
Temperature obtained from the DSC cooling trace.
Temperature obtained using POM.
|
50 |
122 (49.00) |
● |
87a (7.02) |
|
|
● |
105b |
● |
167a (3.07) |
● |
47.5 |
122 (32.42) |
● |
85a (6.52) |
|
|
● |
104b |
● |
165a (3.10) |
● |
45 |
123 (49.14) |
● |
87a (6.81) |
|
|
● |
103b |
● |
165a (2.72) |
● |
40 |
123 (46.19) |
● |
88a (7.51) |
|
|
● |
99b |
● |
163a (2.75) |
● |
37.5 |
123 (39.39) |
● |
88a (6.84) |
|
|
● |
98b |
● |
162a (2.37) |
● |
30 |
123 (46.56) |
● |
86a (7.48) |
● |
89a (0.45) |
● |
95b |
● |
159a (2.86) |
● |
25 |
123 (38.46) |
● |
85a (4.49) |
● |
92a (0.34) |
● |
93b |
● |
156a (1.77) |
● |
20 |
123 (47.21) |
● |
84a (5.53) |
● |
92a (0.40) |
|
|
● |
154a (2.31) |
● |
10 |
123 (40.86) |
● |
83a (4.22) |
● |
94a (0.26) |
|
|
● |
149a (1.18) |
● |
5 |
122 (49.57) |
● |
80a (3.64) |
● |
92a (0.28) |
|
|
● |
145a (1.85) |
● |
0 |
121 (48.63) |
● |
78a (3.11) |
● |
93a (0.31) |
|
|
● |
142a (1.27) |
● |
Textural features similar to those found in a covalently-bonded N*TB system were also observed in mixtures with less than 50% of the chiral component. Specifically, in a 3 μm planar aligned cell, the oily streak texture of the chiral nematic phase transforms to a stripe pattern some degrees prior to the transition to the N*TB phase, see Fig. 3. This phenomenon was observed in the N* phase of both chirally-doped bent core materials and chiral twist-bend nematogens, and suggested to be a consequence of different modulated equilibrium director structures.18,31 In planar aligned cells, the helical axis of the N* phase is oriented perpendicular to the substrate, and the free energy of this system is minimised when the cell thickness, d, is a multiple of the half pitch, p/2. Since the pitch is temperature dependent, at most temperatures this condition is not fulfilled, and thus to minimise the free energy the helix becomes distorted. In a normal N* system, these deformations typically involve the twist elastic constant K22. However, in the system studied here we presume the bend elastic constant K33 is smaller than K22 and instead we see bend deformations, resulting in this stripe texture. The reason for this is not clear but may reflect the instantaneous formation of short pitch helices which influence the macroscopic bend elastic constant.32 At the N*–N*TB transition, the oily streak pattern undergoes dynamic textural changes before settling into a generally uniform texture of birefringence and pattern akin to that seen in covalently-bonded chiral twist-bend nematogens.18 However, the narrow temperature range of the N*TB phase presumably prevented further development of the more detailed stripe and chain-like textures seen previously in the N*TB phase.18 This is the first observation of the chiral twist-bend nematic phase in a hydrogen-bonded system. In the smectic A* phase, characteristic focal conic fans were clearly observed when the phase was viewed between untreated glass slides (Fig. 3).
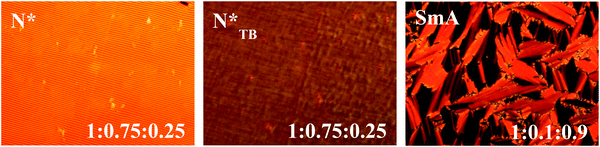 |
| Fig. 3 POM images for 1OB6OS : 4OBA : (S)-(2-Me)4OBA with composition 1 : 0.75 : 0.25 in the N* and N*TB phases (left and middle, respectively) in a 3 micron planar aligned cell, and with composition 1 : 0.1 : 0.9 in the SmA phase (right) in untreated glass slides. | |
The phase diagram constructed from these data is shown in Fig. 4. It is clear that, as with the rod-like acid complexes (Fig. 2), TN(*)I is significantly reduced on switching from a straight to a branched chain acid. The magnitude of this reduction is approximately half that seen for the acid dimers across Table 2, consistent with the presence of one methyl branch in 1OB6OS:(S)-(2-Me)4OBA as opposed to two in the pure acid complex (S)-(2-Me)4OBA. TN(*)TBN(*) also decreases with increasing amount of (S)-(2-Me)4OBA, and at 30 mol% chiral acid in the mixture, the N*TB phase is precluded by the emergence of a SmA* phase. TSmA*N* is reduced upon addition of the unbranched acid, but this is a weaker dependence. The transition temperatures of the SmX phase remain essentially constant across all mixture compositions.
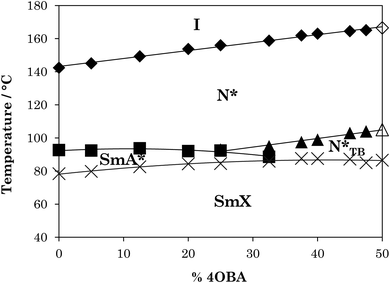 |
| Fig. 4 Dependence of phase behaviour of the 1OB6OS:4OBA:(S)-(2-Me)4OBA series of ternary mixtures on the molar percentage of achiral H-bond donor 4OBA, where ◆ represents TN*I, ■ TSmAN*, ▲ TN*TBN* and × TNTB/SmA-SmX. Unfilled diamond and triangle represent conventional N–I and NTB–N, respectively. Melting points omitted for clarity. | |
CB6OS:(S)-(2-Me)4OBA
The supramolecular complex formed by CB6OS and 4OBA also exhibits conventional nematic and twist-bend nematic phases.22 The CB6OS:(S)-(2-Me)4OBA binary mixture exhibits the chiral nematic phase and, unlike its methoxy analogue 1OB6OS:(S)-(2-Me)4OBA, the chiral twist-bend nematic phase. The ordered smectic phase (SmX) seen for 1OB6OS:(S)-(2-Me)4OBA is absent for CB6OS:(S)-(2-Me)4OBA. In a 3 micron planar aligned cell, the oily streak texture of the N* phase again undergoes a dynamic change at the N*–N*TB phase transition, to a blocky, somewhat striped texture with areas of chain-like defects, see Fig. 5, also similar to textures reported for covalently bonded chiral twist-bend nematogens.18 The X-ray patterns for both the N* and N*TB phases are also shown in Fig. 5 and are very similar: specifically, these contain diffuse wide-angle scattering indicative of the liquid-like ordering in nematic phases. There is no visible small-angle reflection at the short scan times used (∼10 minutes exposure time, as the sample was prone to crystallisation), and hence the local arrangement of the molecules unfortunately could not be determined.
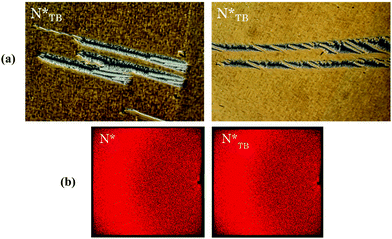 |
| Fig. 5 (a) POM textures seen for the chiral twist-bend nematic phase and (b) X-ray diffraction patterns obtained in the N* and N*TB phases for CB6OS:(S)-(2-Me)4OBA. | |
The transition temperatures and associated enthalpy changes for the mixtures are given in Table 4. The methyl branch lowers the TN(*)I by 22 K, approximately the same as in the 1OB6OS mixtures, and this is accompanied by a reduction in ΔSN(*)I. These observations are consistent with the behaviour of unbranched and branched pairs of covalently bonded dimers, and suggests an increase in biaxiality in the N* phase on branching the complex.33,34TN(*)TBN(*) is less affected, lowered by only 7 K on chain branching, suggesting that a reduction in interaction strength parameter has a lesser effect on the stability of the N*TB phase than on that of the N* phase, and highlighting the importance of molecular curvature for the predominantly shape-driven NTB–N transition.35 In previous studies we have also noted that N*TB–N* transition temperatures for optically pure chiral dimers have been found to be higher than their racemic counterparts,18 in accord with the elastic instability model described by Meyer.13 While we have no racemic counterpart for comparison here, it is possible that such an enhancement in TN*TBN* has somewhat offset the effects of the branch, resulting in a smaller reduction than expected.
Table 4 Transition temperatures (T, °C) and associated enthalpy changes (ΔH, kJ mol−1) for the CB6OS:4OBA and CB6OS:(S)-(2-Me)4OBA supramolecular dimers
|
MP |
N*TB |
|
N* |
|
I |
Temperature obtained from the DSC cooling trace.
Temperature obtained using POM.
|
4OBA |
107 (29.67) |
● |
95b |
● |
167 (2.87) |
● |
(2-Me)4OBA |
103 (44.26) |
● |
88a (0.14) |
● |
145 (1.27) |
● |
Finally, resonant soft X-ray scattering (RSoXS), with incident beam energy tuned to the absorption K-edge of carbon was used to confirm the helical structure of the chiral twist-bend nematic phase. A weak, single resonant signal was found in the N*TB phase (Fig. 6), corresponding to periodicity 8.1–8.4 nm – approximately 2 complex lengths. Interestingly, the observed temperature dependence of the helical pitch near the N*TB–N* transition is much weaker than typically seen for twist-bend nematic phases in covalently-bonded dimers, suggesting a strongly first-order transition, although the enthalpy change associated with the N*TB–N* transition remains characteristically small.
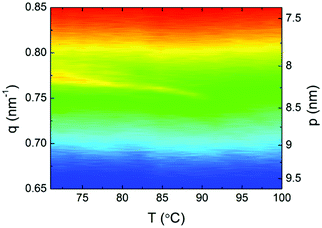 |
| Fig. 6 RSoXS pattern obtained for CB6OS:(S)-(2-Me)4OBA. | |
CB6OBA:(S)-(2-Me)4OS
The supramolecular complex CB6OBA:4OS is an enantiotropic nematogen, and on cooling also exhibits the NTB phase.23 Unlike in the 1OB6OS:4OBA and CB6OS:4OBA systems, this is followed on further cooling by a SmC phase and another more ordered smectic phase, which here we denote SmX2. The use of a chiral molecule as the hydrogen bond acceptor – (S)-(2-Me)4OS, here considered as the chiral “equivalent” of 4OS – to form the chiral complex CB6OBA:(S)-(2-Me)4OS does not alter the phase behaviour; on cooling from the isotropic phase we observe the sequence N*–N*TB–SmC*–SmX2*. The transition temperatures and associated enthalpy changes for both complexes are given in Table 5.
Table 5 Transition temperatures (T, °C) and associated enthalpy changes (ΔH, kJ mol−1) for the CB6OBA:4OS and CB6OBA:(S)-(2-Me)4OS supramolecular dimers
|
MP |
SmX* |
|
SmC* |
|
N*TB |
|
N* |
|
I |
Temperature obtained from the DSC cooling trace.
Temperature obtained using POM.
|
4OS |
108 (36.87) |
● |
70a (2.58) |
● |
86a (0.69) |
● |
113b |
● |
180 (2.65) |
● |
(S)-(2-Me)4OS |
154 (54.92) |
● |
77a (4.16) |
● |
97a (2.68) |
● |
111b |
● |
151a (3.31) |
● |
The highest temperature phase is a conventional chiral nematic phase N*, assigned by observation of a characteristic oily streak texture, see Fig. 7. Cooling from the N* phase led to the dynamic N*–N*TB textural change as seen in previous systems showing the N*TB phase, and a resulting texture containing rope- and chain-like defects (Fig. 7). X-ray diffraction patterns of these higher temperature phases, also shown in Fig. 7, exhibited only diffuse wide- and small-angle signals, consistent with the absence of a lamellar structure and confirm the liquid-like ordering of molecules in the nematic phases. A transition from the N*TB phase to a smectic phase with liquid-like order within the layers is clear from the narrowing of the small-angle X-ray diffraction signal while the high-angle signal remains diffuse. Optically, this phase retains many of the features of the N*TB phase, most significantly the stripe and rope-like pattern, although these “ropes” now appear to have a more braided texture. Homeotropically aligned areas which appear black in the preceding N*TB phase also remain as such in the smectic phase. Given the textural similarities of this phase to the higher temperature smectic phase seen in analogous achiral complexes, it has been tentatively assigned as a SmC* phase. We note the possibility that the SmC phase reported for the achiral complexes may belong to the new class of heliconical smectic phases formed by achiral materials,36,37 but its monotropic nature precluded further investigation.23 It is therefore possible that the helical structure of the SmC* phase seen here is not conventional, and this may be a subject of future work. On further cooling a bright, schlieren-like mosaic texture emerges from the dark regions of the SmC* phase, and this strongly resembles the texture of the lowest temperature phase exhibited by the achiral CB6OBA:nOS complexes with n = 3–10. The X-ray diffraction pattern retains sharp small-angle signals, and the high angle signal is split and narrowed, suggesting a tilted hexatic-type smectic phase. This shows that the SmX phases present in the CB6OBA:nOS series (n = 3–10) and CB6OBA:(S)-(2-Me)4OS are the same, and we have denoted the latter SmX* as it is formed from chiral molecules. Across all these phases, the small angle signals appear at slightly more than the full complex length (d/l ∼ 1.2; d is the periodicity deduced from the position of the lowest angle diffraction signal, l is the estimated length of the molecule in the most stretched conformation), hence the arrangement of the complexes may be partially bilayer with strong interdigitation, see structure in Fig. 8 and data in Table 6.
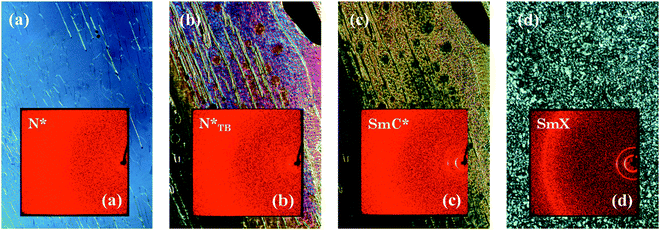 |
| Fig. 7 POM images and associated X-ray diffraction patterns (insets) for CB6OBA:(S)-(2-Me)4OS in the (a) N*, (b) N*TB, (c) SmC* and (d) SmX phases. | |
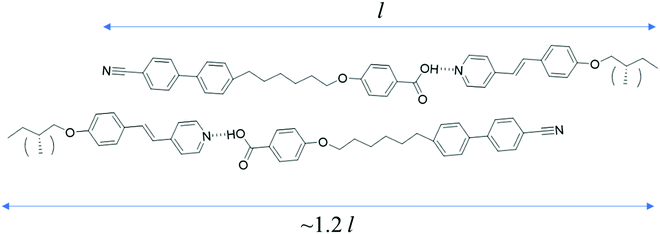 |
| Fig. 8 Proposed molecular arrangement in the lamellar phases of CB6OBA:(S)-(2-Me)4OS. | |
Table 6 Layer thickness in the smectic phases obtained from integrated diffraction signals for the CB6OBA:(S)-(2-Me)4OS complex
|
Phase |
Complex length, l/Å |
Low angle signals/Å |
High angle signals/Å |
d/l |
CB6OBA:(S)-(2-Me)4OS |
SmC* |
40.1 |
47.8, 24.2, 13.0 |
4.3 |
1.2 |
SmX |
46.5, 23.5 |
4.6, 4.2 |
1.2 |
Branching the terminal chain has the expected effect on TN*I, specifically a reduction by 29 K. The branch also decreases TN(*)TBN(*), but as in the previous systems, it is a smaller reduction of only 2 K. The opposite effect is observed for the smectic phases, with both SmC* and SmX* increasing in stability with the branch. This enhancement of the smectic phases may be attributed to the branched group effectively filling void space in the tilted lamellar structure to such an extent that this stabilisation more than offsets the dilution in lateral interactions of the mesogenic cores brought about by the branch. This is also evident in the increased melting point of the branched complex, suggesting a more efficient packing in the crystal phase compared with that for the unbranched one.
Conclusion
We have described the first examples of the chiral twist-bend nematic phase, N*TB, exhibited by supramolecular liquid crystals formed by hydrogen-bonding between unlike H-bonded donors and acceptors. In general, the properties of the N*TB phase strongly resemble those previously observed in covalently bonded materials. In particular, the optical textures observed are distinct and contain unusual features, the physical significance of which remain unclear. In addition, the thermal stability of the N*TB phase is greater than that of the conventional NTB phase exhibited by achiral materials. We have observed a transition between the N*TB phase and a SmC* phase, and we have still to determine whether the structure of this SmC* phase is conventional, or more akin to the heliconical smectic phases recently reported for achiral molecules with a strikingly short helical pitch.36,37
Funding
The work was supported by the National Science Centre (Poland) under the grant no. 2016/22/A/ST5/00319.
Conflicts of interest
The authors declare no conflict of interest.
Acknowledgements
RW gratefully thanks the Carnegie Trust for the Universities of Scotland for the award of a PhD Scholarship (2015–2018).
References
- M. Cestari,
et al., Phase behavior and properties of the liquid-crystal dimer 1′′,7′′-bis(4-cyanobiphenyl-4′-yl) heptane: A twist-bend nematic liquid crystal, Phys. Rev. E: Stat., Nonlinear, Soft Matter Phys., 2011, 84, 031704 CrossRef CAS PubMed.
-
R. B. Meyer, Structural problems in liquid crystal physics, Les Houches Summer School in Theoretical Physics, Molecular Fluids, Gordon and Breach, 1976, pp. 316–320 Search PubMed.
- I. Dozov, On the spontaneous symmetry breaking in the mesophases of achiral banana-shaped molecules, Europhys. Lett., 2001, 56, 247–253 CrossRef CAS.
- V. Borshch,
et al., Nematic twist-bend phase with nanoscale modulation of molecular orientation, Nat. Commun., 2013, 4, 2635 CrossRef CAS PubMed.
- P. A. Henderson and C. T. Imrie, Methylene-linked liquid crystal dimers and the twist-bend nematic phase, Liq. Cryst., 2011, 38, 1407–1414 CrossRef CAS.
- E. Cruickshank,
et al., Sulfur-linked cyanobiphenyl-based liquid crystal dimers and the twist-bend nematic phase, Liq. Cryst., 2019, 46, 1595–1609 CrossRef CAS.
- D. A. Paterson, J. P. Abberley, W. T. A. Harrison, J. Storey and C. T. Imrie, Cyanobiphenyl-based liquid crystal dimers and the twist-bend nematic phase, Liq. Cryst., 2017, 44, 127–146 CAS.
- D. A. Paterson,
et al., The role of a terminal chain in promoting the twist-bend nematic phase: the synthesis and characterisation of the 1-(4-cyanobiphenyl-4′-yl)-6-(4-alkyloxyanilinebenzylidene-4′-oxy)hexanes, Liq. Cryst., 2018, 45, 2341–2351 CrossRef CAS.
- R. J. Mandle, C. T. Archbold, J. P. Sarju, J. L. Andrews and J. W. Goodby, The dependency of nematic and twist-bend mesophase formation on bend angle, Sci. Rep., 2016, 6, 1–12 CrossRef PubMed.
- M. Šepelj,
et al., Intercalated liquid-crystalline phases formed by symmetric dimers with an α,ω-diiminoalkylene spacer, J. Mater. Chem., 2007, 17, 1154–1165 RSC.
- R. J. Mandle, The dependency of twist-bend nematic liquid crystals on molecular structure: A progression from dimers to trimers, oligomers and polymers, Soft Matter, 2016, 12, 7883–7901 RSC.
- W. D. Stevenson,
et al., Dynamic calorimetry and XRD studies of the nematic and twist-bend nematic phase transitions in a series of dimers with increasing spacer length, Phys. Chem. Chem. Phys., 2018, 20, 25268–25274 RSC.
- C. Meyer, Nematic twist-bend phase under external constraints, Liq. Cryst., 2016, 43, 2144–2162 CrossRef CAS.
- L. Longa and W. Tomczyk, Twist-bend nematic phase in the presence of molecular chirality, Liq. Cryst., 2018, 45, 2074–2085 CrossRef CAS.
- R. J. Mandle and J. W. Goodby, A novel nematic-like mesophase induced in dimers, trimers and tetramers doped with a high helical twisting power additive, Soft Matter, 2018, 14, 8846–8852 RSC.
- C. T. Archbold, E. J. Davis, R. J. Mandle, S. J. Cowling and J. W. Goodby, Chiral dopants and the twist-bend nematic phase-induction of novel mesomorphic behaviour in an apolar bimesogen, Soft Matter, 2015, 11, 7547–7557 RSC.
- E. Gorecka,
et al., A Twist-Bend Nematic (NTB) Phase of Chiral Materials, Angew. Chem., Int. Ed., 2015, 54, 10155–10159 CrossRef CAS PubMed.
- R. Walker, J. M. D. Storey, C. T. Imrie, D. Pociecha and E. Gorecka, The Chiral Twist-Bend Nematic Phase (N*TB), Chem. – Eur. J., 2019, 25, 13329–13335 CrossRef CAS PubMed.
- C. M. Paleos and D. Tsiourvas, Supramolecular hydrogen-bonded liquid crystals, Liq. Cryst., 2001, 28, 1127–1161 CrossRef CAS.
- G. W. Gray and B. Jones, Mesomorphism of some Alkoxynaphthoic Acids, Nature, 1951, 167, 83–84 CrossRef CAS PubMed.
- S. M. Jansze, A. Martínez-Felipe, J. M. D. Storey, A. T. M. Marcelis and C. T. Imrie, A twist-bend nematic phase driven by hydrogen bonding, Angew. Chem., Int. Ed., 2015, 54, 643–646 CAS.
- R. Walker,
et al., Hydrogen bonding and the design of twist-bend nematogens, J. Mol. Liq., 2020, 303, 112630 CrossRef.
- R. Walker,
et al., Twist-Bend Nematogenic Supramolecular Dimers and Trimers Formed by Hydrogen Bonding, Crystals, 2020, 10, 175 CrossRef CAS.
- R. Walker,
et al., Spontaneous chirality
through mixing achiral components: A twist-bend nematic phase driven by hydrogen-bonding between unlike components, Chem. Commun., 2018, 54, 3383–3386 RSC.
- T. Kato and J. M. J. Fréchet, New Approach to Mesophase Stabilization through Hydrogen-Bonding Molecular Interactions in Binary Mixtures, J. Am. Chem. Soc., 1989, 111, 8533–8534 CrossRef CAS.
- D. A. Paterson,
et al., Understanding the twist-bend nematic phase: The characterisation of 1-(4-cyanobiphenyl-4′-yloxy)-6-(4-cyanobiphenyl-4′-yl)hexane (CB6OCB) and comparison with CB7CB, Soft Matter, 2016, 12, 6827–6840 RSC.
- S. El Sayed,
et al., 2,4-Dinitrophenyl Ether-Containing Chemodosimeters for the Selective and Sensitive in Vitro and in Vivo Detection of Hydrogen Sulfide, Supramol. Chem., 2015, 27, 244–254 CrossRef CAS.
- J. Jensen, S. C. Grundy, S. L. Bretz and C. S. Hartley, Synthesis and characterization of self-assembled liquid crystals: P-alkoxybenzoic acids, J. Chem. Educ., 2011, 88, 1133–1136 CrossRef CAS.
- P. Painter, C. Cleveland and M. Coleman, An Infrared Spectroscopic Study of p-n-Alkoxybenzoic Acids, Mol. Cryst. Liq. Cryst. Sci. Technol., Sect. A, 2000, 348, 269–293 CrossRef CAS.
- A. Martínez-Felipe, A. G. Cook, M. J. Wallage and C. T. Imrie, Hydrogen bonding and liquid crystallinity of low molar mass and polymeric mesogens containing benzoic acids: A variable temperature Fourier transform infrared spectroscopic study, Phase Transitions, 2014, 87, 1191–1210 CrossRef.
- S. M. Salili,
et al., Spontaneously modulated chiral nematic structures of flexible bent-core liquid crystal dimers, Liq. Cryst., 2017, 44, 160–167 CAS.
- D. Pociecha,
et al., Critical behavior of the optical birefringence at the nematic to twist-bend nematic phase transition, Phys. Rev. E, 2018, 98, 052706 CrossRef CAS.
- C. T. Imrie, Laterally substituted dimeric liquid crystals, Liq. Cryst., 1989, 6, 391–396 CrossRef CAS.
- J. P. Abberley,
et al., Structure–property relationships in twist-bend nematogens: the influence of terminal groups, Liq. Cryst., 2017, 44, 68–83 CAS.
- R. Walker,
et al., Molecular curvature, specific intermolecular interactions and the twist-bend nematic phase: the synthesis and characterisation of the 1-(4-cyanobiphenyl-4′-yl)-6-(4-alkylanilinebenzylidene-4′-oxy)hexanes (CB6O.m), Soft Matter, 2019, 15, 3188–3197 RSC.
- M. Salamończyk,
et al., Multi-level chirality in liquid crystals formed by achiral molecules, Nat. Commun., 2019, 10, 1922 CrossRef PubMed.
- J. P. Abberley,
et al., Heliconical smectic phases formed by achiral molecules, Nat. Commun., 2018, 9, 228 CrossRef PubMed.
Footnote |
† Electronic supplementary information (ESI) available. See DOI: 10.1039/d0ma00302f |
|
This journal is © The Royal Society of Chemistry 2020 |