DOI:
10.1039/C9SC02835H
(Edge Article)
Chem. Sci., 2019,
10, 9265-9269
Saturated oxygen and nitrogen heterocycles via oxidative coupling of alkyltrifluoroborates with alkenols, alkenoic acids and protected alkenylamines†
Received
11th June 2019
, Accepted 17th August 2019
First published on 19th August 2019
Abstract
Saturated heterocycles are important components of many bioactive compounds. The method disclosed herein enables a general route to a range of 5-, 6- and 7-membered oxygen and nitrogen heterocycles by coupling potassium alkyltrifluoroborates with heteroatom-tethered alkenes, predominantly styrenes, under copper-catalyzed conditions, in the presence of MnO2. The method was applied to the synthesis of the core of the anti-depressant drug citalopram. The reaction scope and observed reactivity is consistent with a polar/radical mechanism involving intermolecular addition of the alkyl radical to the alkene followed by [Cu(III)]-facilitated C–O (or C–N) bond forming reductive elimination.
Introduction
The flexible, convergent synthesis of saturated oxygen and nitrogen heterocycles can be accomplished by the addition of a carbon radical to readily available alkenoic acids, alkenols and alkenyl amines under oxidative conditions (Scheme 1a).1–10
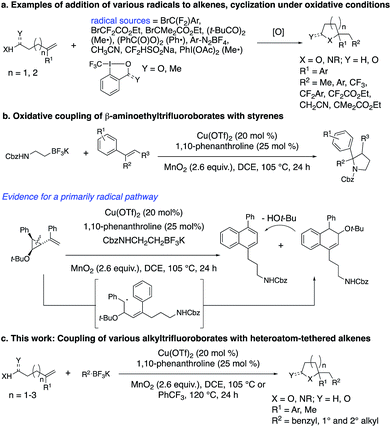 |
| Scheme 1 Radical additions to alkenes and oxidative cyclizations thereof. | |
The carbon radical source is an important variable in these alkene addition processes. Alkylhalides have been applied, in particular when their metal-catalyzed reduction forms stabilized or semi-stabilized carbon radicals, such as those conjugated with carbonyl groups11 or that are perhalogenated.10 Halosulfonate salts that form radicals by oxidative desulfonation have also been used.4,5 Hypervalent iodine reagents that decompose in the presence of metal catalysts to generate alkyl radicals have been used in such alkene coupling reactions.2,3,6–8 Nitriles, whose alpha positions can be deprotonated and oxidized, have been applied as the stabilized alkyl radical component.9 Less stable alkyl radicals, generated by decomposition of peroxides1 and from aryldiazonium salts, have also been used.3 Benzyl and other simple alkyl radicals are generally lacking in these radical addition/cyclization methodologies.
We and others have been exploring the use of alkyltrifluoroborates as radical precursors in oxidative coupling reactions.12 Oxidation of the alkyltrifluoroborates by metal catalysts under either thermal or photochemical activation enables the generation of a range of alkyl radicals that can undergo direct addition or metal-catalyzed coupling with appropriate coupling partners including alkenes. Primary, secondary and benzylic radicals are among the alkyl radicals formed under such oxidative conditions from alkyltrifluoroborates.
We recently reported on the synthesis of 2-arylpyrrolidines via copper-catalyzed oxidative coupling of styrenes with potassium N-carbamolyl-β-aminoethyl trifluoroborates (Scheme 1b).13 In these reactions, the [Cu] salt oxidizes the alkyltrifluoroborate to a primary carbon radical. Addition of the radical to the styrene, followed by cyclization via addition of the pendant carbamate under the oxidative conditions generates the 2-arylpyrrolidine. A radical clock experiment supported a mechanism involving primarily carbon radical intermediates (as opposed to carbocation).13 Herein we present a new approach to the synthesis of a broad range of saturated heterocycles by addition of various potassium alkyltrifluoroborates to heteroatom-functionalized alkenes, primarily styrenes.
Results and discussion
The oxidative coupling/cyclization of 2-(1-phenylvinyl)benzoic acid 1a with potassium benzyltrifluoroborate (limiting reagent) was investigated as illustrated in Table 1. Reaction variables such as substrate and copper loading, solvent, temperature, ligand and oxidant were explored. Coupling 1a (1 equiv.) with BnBF3K in the presence of 20 mol% Cu(OTf)2, 25 mol% 1,10-phenanthroline (3a) and MnO2 (2.6 equiv.) in 1,2-dichloroethane (DCE) at 105 °C gave 78% of lactone 2a (Table 1, entries 1). An increase of 1a loading to 1.5 equiv. resulted in a higher isolated yield of 2a (84%, Table 1, entry 2). A decrease in Cu(OTf)2 loading to 5 and 10 mol% both gave 71% isolated yield of lactone 2a (Table 1, entries 3 and 4). In the absence of [Cu], lactone 2a is still formed, albeit in diminished yield (51%, Table 1, entry 5 and see below Scheme 4 and associated discussion). The reactions in toluene and dioxane were less productive while the reaction in PhCF3 gave a comparable yield to DCE (Table 1, entries 6–8). The use of other ligands, 3b–e and 4a, or no ligand, gave reactions with equivalent or lower yields (Table 1, entries 9–14). When Ag2CO3 or K2S2O8 were used instead of MnO2, lower yields were obtained (Table 1, entries 15 and 16). The reaction scale with respect to limiting BnBF3K could be run on 1 mmol scale (73% isolated 2a, Table 1, entry 17).
Table 1 Reaction optimizationa
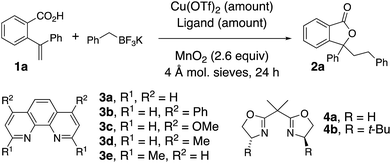
|
Entry |
Mol% Cu(OTf)2 |
Ligand (mol%) |
Solvent |
Temp (°C) |
Yield (%) |
All reactions were run in a sealed tube under argon with 0.125 mmol BnBF3K and 1.5 equiv. of acid 1a in DCE (0.125 mM) unless otherwise noted.
Reaction run with 1 equiv. of acid 1a.
Reaction run with Ag2CO3 (1.3 equiv.) instead of MnO2.
Reaction run with K2S2O8 (2.6 equiv.) instead of MnO2.
Reaction run with 1 mmol BnBF3K.
|
1b |
20 |
3a (25) |
DCE |
105 |
78 |
2 |
20 |
3a (25) |
DCE |
105 |
84 |
3 |
5 |
3a (6) |
DCE |
105 |
71 |
4 |
10 |
3a (12) |
DCE |
105 |
71 |
5 |
— |
— |
DCE |
105 |
51 |
6 |
20 |
3a (25) |
PhCH3 |
120 |
48 |
7 |
20 |
3a (25) |
PhCF3 |
120 |
82 |
8 |
20 |
3a (25) |
Dioxane |
120 |
71 |
9 |
20 |
3b (25) |
DCE |
105 |
62 |
10 |
20 |
3c (25) |
DCE |
105 |
84 |
11 |
20 |
3d (25) |
DCE |
105 |
77 |
12 |
20 |
3e (25) |
DCE |
105 |
66 |
13 |
20 |
4a (25) |
DCE |
105 |
71 |
14 |
20 |
— |
DCE |
105 |
54 |
15c |
20 |
3a (25) |
DCE |
105 |
67 |
16d |
20 |
3a (25) |
DCE |
105 |
35 |
17e |
20 |
3a (25) |
DCE |
105 |
73 |
Using the optimal reaction conditions (Table 1, entry 2), the alkyltrifluoroborate scope was next explored in the coupling/cyclization reaction with alkenoic acids 1 (Table 2). Alkyltrifluoroborates such as benzylic, primary, methyl, ethyl, neopentyl, allylic and secondary, and alkyls functionalized with a nitrile, an acetal and a carbamate all underwent the coupling reaction with varying levels of efficiency. A tert-butyl ester-functionalized alkyltrifluoroborate was also a viable coupling partner [see Table 4 (8g) and Table 5 (12j)]. Neither CF3BF3K nor t-BuOCH2BF3K gave the desired coupling product with 1a.
Table 2 Trifluoroborate scopea
Reaction conditions from Table 1, entry 2 were used unless otherwise noted.
10 mol% Cu(OTf)2 and 12 mol% 1,10-phenanthroline was used.
|
|
Alkenoic acid substituent effects were further explored (Tables 2 and 3). Diaryl alkenes, such as 1a, reacted most efficiently to give phthalide 2a in 84% (Table 2). Replacing the phenyl with methyl (Table 3, 2m, 64%) or H (Table 3, 2n, 39%) gave lower phthalide yields. Substitution on the arene conjugated to the alkene was tolerated, and lactones 5a–f were formed in good yields. A 1,1-dialkyl substituted alkene did undergo addition and cyclization but the desired product was generated in much lower yield (5g, 12%). The remainder of the mass in the lower yielding reactions included unreacted alkenoic acid, (PhCH2)2 (benzyl radical dimerization)14 and benzyl alcohol. Both 6-membered (6a and 6b) and 7-membered ring lactones (7a and 7b) could also be formed. The 2,2′-disubstituted biaryl-backboned alkenoic acid provided 7b more efficiently than the largely aliphatic-backboned heptenoic acid gave 7a.
Table 3 Alkenoic acid scopea
Reaction conditions from Table 1, entry 2 were used unless otherwise noted.
|
|
The scope was next expanded to alkenol coupling/cyclization for the synthesis of saturated oxygen heterocycles (Table 4). The synthesis of tetrahydrofurans (8a–j), phthalans (9a–c), isochromans (10a, 10b), a pyran (10c), morpholines (10d, 10e) and oxepanes (11a, 11b) is enabled by this oxidative coupling reaction. Phthalan 9c, product of coupling of potassium N-Cbz-β-aminoethyltrifluoroborate with the respective 4-cyanophenyl-functionalized alkenol, is a reasonable intermediate for the synthesis of citalopram,9,15 a drug used for the treatment of depression.
Reaction conditions from Table 1, entry 2 were used unless otherwise noted.
Reaction run in PhCF3 at 120 °C.
Reaction run with 10 mol% Cu(OTf)2, 12 mol% 1,10-phenanthroline.
Reaction run for 48 h.
|
|
The reaction was expanded to protected alkenyl amine coupling/cyclization for the synthesis of saturated nitrogen heterocycles (Table 5). In this reaction higher alkenylamine loading (3 equiv., 57% of 12c) gave notably higher isolated yield (32% of 12c was obtained when 2 equiv. of alkenyl amide was used). Variously N-substituted alkenylamines produced pyrrolidines (12a–f, 12h–l), a cyclic urea (12g), an isoindoline (13), a tetrahydroisoquinoline (14) and a tetrahydrobenzoazepine (15).
Table 5 Alkenyl amide scopea
Reaction conditions from Table 1, entry 2 were used except 3 equiv. of alkenylamide was used.
Reaction run in PhCF3 at 120 °C.
Reaction run with 2 equiv. alkenylamide. PG = protecting group.
|
|
Enantioselective conditions
We explored the potential for a chiral ligand to control absolute stereochemistry in our oxidative coupling. A brief substrate and ligand screen revealed that 4-phenylpent-4-enoic acid (1b) can undergo enantioselective coupling/cyclization under minimally modified (lower temperature, t-BuOMe as solvent) reaction conditions using the (S,S)-t-Bu-Box 4b (Scheme 2, 42% yield, 44% ee).2,3 In the absence of [Cu], 15% of lactone 5a was formed as a racemate, indicating some background reaction can occur. In the absence of MnO2, using 50 mol% copper loading and 55 mol% of 4b, 5a was obtained in 22% yield and 44% ee, indicating the potential MnO2 promoted background reaction is unlikely to be affecting the reaction's enantioselectivity.
 |
| Scheme 2 Ligand-induced enantioselectivity. | |
Proposed mechanism
The proposed mechanism is illustrated in Scheme 3. Either copper(II) or MnO2, or a mixture of both, oxidizes the alkyltrifluoroborate to the corresponding radical.14 Addition of the resulting alkyl radical to 1b provides a benzylic radical intermediate. The enantioselective result in Scheme 2 supports the involvement of a chiral ligand-bound copper complex in the C–O bond formation. Thus, formation of an alkyl copper(III) intermediate via addition of the alkyl radical to the [Cu(II)] complex and subsequent C–O bond formation via reductive elimination generates lactone 5a (Scheme 3).3,16 The [Cu(II)] is regenerated by oxidation of [Cu(I)] to [Cu(II)] by MnO2 to continue the catalytic cycle (not shown). The background reaction observed under MnO2-only conditions likely occurs via the alternative benzylic carbocation intermediate, which would be expected to give racemic 5a (Scheme 3).
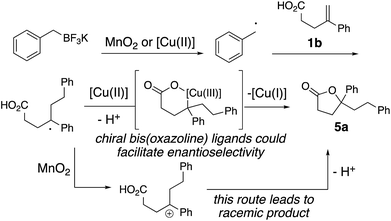 |
| Scheme 3 Proposed mechanism. | |
MnO2-promoted reactions
The possibility of an oxidative coupling involving only MnO2 as oxidant was further investigated (Scheme 4). Manganese salts have previously demonstrated the ability to oxidize alkyltrifluoroborates and boronic acids to radicals, that then undergo oxidative coupling with alkenoic acids17 or heteroarenes (Minisci reaction).18 Under reaction conditions analogous to our alkyltrifluoroborate couplings, but in the absence of any [Cu] salt, we found that alkenoic acids, alkenyl alcohols and alkenyl amines do undergo the oxidative cyclizations, albeit with generally lower efficiency. The secondary cyclopentyl trifluoroborate reacted similarly with alkenoic acid 1a in the presence of MnO2, both with and without [Cu] catalyst (42% vs. 38% of 2d, compare Table 2 to Scheme 4), while benzyltrifluoroborate gave notably higher yield with the catalytic [Cu] conditions (84% vs. 51% of 2a, compare Table 1, entry 3 to Scheme 4). In the absence of [Cu] catalyst, alkenol and alkenyl amide substrates gave considerably lower yields of their corresponding heterocycles 8b (84% with [Cu] vs. 21% without, Table 4 and Scheme 4) and 12l (84% with [Cu] vs. 20% without, Table 5 and Scheme 4). In the MnO2 promoted reactions it is likely the C–O and C–N bond formations involve addition of the heteroatom to a carbocationic intermediate (Scheme 3).17
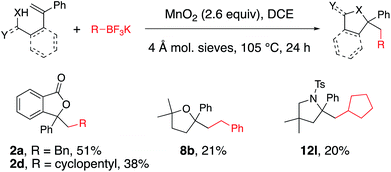 |
| Scheme 4 MnO2-only oxidative cyclization. | |
Conclusions
The copper-catalyzed addition of alkyltrifluoroborates to heteroatom-tethered vinyl arenes under oxidative conditions has been demonstrated to be a general route to saturated oxygen and nitrogen heterocycles. The possibility of asymmetric catalysis has been demonstrated. The observed reactivity is largely consistent with alkyl radical addition to the vinyl arene and [Cu(III)]-facilitated C–O and C–N bond formation. A good range of alkyltrifluoroborates serve as alkyl radical source; these reagents are complementary to radical precursors that generally involve generation of more stabilized radicals.
Conflicts of interest
There are no conflicts to declare.
Acknowledgements
We thank the National Institutes of Health (GM078383) and the Arthur C. Cope Fund for support of this research.
Notes and references
-
(a) X. Bao, T. Yokoe, T. M. Ha, Q. Wang and J. Zhu, Nat. Commun., 2018, 9, 3725 CrossRef PubMed;
(b) Intermolecular: W. Jian, L. Ge, Y. Jiao, B. Qian and H. Bao, Angew. Chem., Int. Ed., 2017, 56, 3650 CrossRef CAS PubMed.
- R. Zhu and S. L. Buchwald, Angew. Chem., Int. Ed., 2013, 52, 12655 CrossRef CAS PubMed.
- R. Zhu and S. L. Buchwald, J. Am. Chem. Soc., 2015, 137, 8069 CrossRef CAS PubMed.
- S. Zhang, L. Li, J. Zhang, J. Zhang, M. Xue and K. Xu, Chem. Sci., 2019, 10, 3181 RSC.
- J.-S. Lin, F.-L. Wang, X.-Y. Dong, W.-W. He, Y. Yuan, S. Chen and X.-Y. Liu, Nat. Commun., 2017, 8, 14841 CrossRef PubMed.
- Y.-F. Cheng, X.-Y. Dong, Q.-S. Gu, Z.-L. Yu and X.-Y. Liu, Angew. Chem., Int. Ed., 2017, 56, 8883 CrossRef CAS PubMed.
- K. Shen and Q. Wang, Org. Chem. Front., 2016, 3, 222 RSC.
- J.-S. Lin, X.-Y. Dong, T.-T. Li, N.-C. Jiang, B. Tan and X.-Y. Liu, J. Am. Chem. Soc., 2016, 138, 9357 CrossRef CAS PubMed.
- T. M. Ha, Q. Wang and J. Zhu, Chem. Commun., 2016, 52, 11100 RSC.
- Y. Da, S. Han, X. Du, S. Liu, L. Liu and J. Li, Org. Lett., 2018, 20, 5149 CrossRef CAS PubMed.
- S. N. Gockel, T. L. Buchanan and K. L. Hull, J. Am. Chem. Soc., 2018, 140, 58 CrossRef CAS PubMed.
-
(a) K. Duan, X. Yan, Y. Liu and Z. Li, Adv. Syn. Cat., 2018, 360, 2781 CrossRef CAS;
(b) J. K. Matsui, S. B. Lang, D. R. Heitz and G. A. Molander, ACS Catal., 2017, 7, 2563 CrossRef CAS PubMed;
(c) D. R. Heitz, K. Rizwan and G. A. Molander, J. Org. Chem., 2016, 81, 7308 CrossRef CAS PubMed;
(d) For related [Cu(II)]-catalyzed reactions with alkyltin reagents, see: M. U. Luescher and J. W. Bode, Synlett, 2019, 30, 464 CrossRef CAS;
(e) Electrochemically promoted intermolecular couplings with vinyl and alkynyltrifluoroborates: P. Xiong, H. Long, J. Song, Y. Wang, J. F. Li and H. C. Xu, J. Am. Chem. Soc., 2018, 140, 16387 CrossRef CAS PubMed.
- C. Um and S. R. Chemler, Org. Lett., 2016, 18, 2515 CrossRef CAS PubMed.
- T. W. Liwosz and S. R. Chemler, Org. Lett., 2013, 15, 3034 CrossRef CAS PubMed.
-
(a) A. Carlsson, K. Fuxe, B. Hamberger and T. Malmfors, Br. J. Pharmacol., 1969, 36, 18 CrossRef CAS PubMed;
(b) J. F. M. Hewitt, L. Williams, P. Aggarwal, C. D. Smith and D. J. France, Chem. Sci., 2013, 4, 3538 RSC and references therein
(c) C. Alamillo-Ferrer, M. Karabourniotis-Sotti, A. R. Kennedy, M. Campbell and N. C. O. Tomkinson, Org. Lett., 2016, 18, 3102 CrossRef CAS PubMed.
- related enantioselective reactions involving Cu(III) intermediates:
(a) M. B. Andrus and Z. Zhou, J. Am. Chem. Soc., 2002, 124, 8806 CrossRef CAS PubMed;
(b) F. Wang, P. Chen and G. Liu, Acc. Chem. Res., 2018, 51, 2036 CrossRef CAS PubMed.
- Y. Gao, J. Xu, P. Zhang, H. Fang, G. Tang and Y. Zhao, RSC Adv., 2015, 5, 36167 RSC.
- G. A. Molander, V. Colombel and V. A. Braz, Org. Lett., 2011, 13, 1852 CrossRef CAS PubMed.
Footnote |
† Electronic supplementary information (ESI) available: Experimental procedures, NMR spectra of all new intermediates and products. See DOI: 10.1039/c9sc02835h |
|
This journal is © The Royal Society of Chemistry 2019 |