DOI:
10.1039/C7SC00790F
(Edge Article)
Chem. Sci., 2017,
8, 3898-3904
Reversible mechanical protection: building a 3D “suit” around a T-shaped benzimidazole axle†
Received
20th February 2017
, Accepted 14th March 2017
First published on 28th March 2017
Abstract
The T-shaped benzimidazolium/crown ether recognition motif was used to prepare suit[1]anes. These novel mechanically interlocked molecules (MIMs) were fully characterized by 1H and 13C NMR spectroscopy, single-crystal X-ray diffraction, UV-vis absorption and fluorescence spectroscopy. By conversion to a suit[1]ane, a simple benzimidazole was shown to be protected from deprotonation by strong base. Moreover, it was demonstrated that this unique three-dimensional encapsulation can be made reversible, thus introducing the concept of “reversible mechanical protection”; a protecting methodology that may have potential applications in synthetic organic chemistry and the design of molecular machinery.
Introduction
Mechanically interlocked molecules (MIMs) are assemblies of molecular components or entanglements that cannot be separated without breaking a covalent bond.1 The combination of template-directed synthesis2 and mechanically interlocked topologies3 has allowed the synthesis of a variety of MIMs such as rotaxanes,4 catenanes,5 daisy chains,6 knots7 and Borromean rings.8 The dynamic nature of MIMs has also been exploited as the basis for developing artificial molecular machines by manipulating the relative positions of their constituent components, especially for rotaxanes and catenanes.9
An underappreciated consequence of mechanically interlocking two molecular components is that the permanence of their intimacy can dramatically affect the chemical properties of the individual components.10 Indeed, it is possible to not only stabilize vulnerable molecules,11 but also develop functional materials such as ‘molecular wires’ by virtue of the interpenetrated structure of rotaxanes.12 In a previous study, we showed how this protecting methodology could be used to alter reactivity, by wrapping a polyether macrocycle around the NH centre of a secondary amine. In this way, a simple Lewis base was converted into a sterically encumbered one, which when combined with a bulky Lewis acid, B(C6F5)3, created a Frustrated Lewis Pair (FLP) capable of inducing the heterolytic activation of hydrogen gas.13 More recently, Leigh has shown that switching of the selectivity of a rotaxane catalyst can be achieved by controlling the position of the macrocyclic wheel on the axle.14a,b Moreover, Berna found that a rotaxane structure can promote the regioselectivity of an intramolecular ring closure reaction.14c
Despite these recent developments, the scope of using MIM formation as a protecting methodology is limited. Herein, we demonstrate that a T-shaped axle can be incorporated into a [2]pseudorotaxane by penetrating a macrocycle and then the macrocycle converted into a cryptand to yield a mechanically interlocked molecule known as a suit[1]ane; Fig. 1.15 Furthermore, we show that this “suit” can protect the structure of the axle from external reagents and then subsequently be removed to re-expose the axle, making this 3D protection strategy wholly reversible.
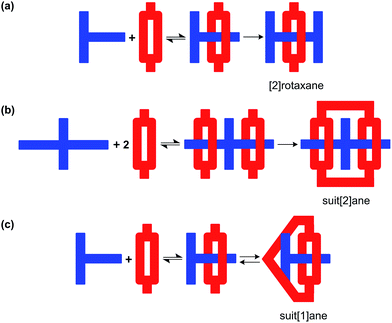 |
| Fig. 1 (a) Conversion of an axle to a [2]pseudorotaxane followed by kinetic trapping as a [2]rotaxane, (b) conversion of an axle with two limbs to a [3]pseudorotaxane followed by trapping as a suit[2]ane and (c) conversion of an axle with one limb to a [2]pseudorotaxane followed by trapping as a suit[1]ane – and the reverse. For suit[n]anes, the value of n represents the number of “limbs” on the axle which are then suited.15a This is different from the common designation of n as the number of components of an interlocked molecule as in [n]rotaxane and [n]catenane. | |
In addition to secondary ammoniums and pyridiniums, it is now well established that imidazolium and benzimidazolium cations also complex well with crown ether hosts.16 In this regard, we developed a T-shaped benzimidazolium cation [1 − H]+ which can act as an axle for the formation of [2]pseudorotaxanes with a wide variety of crown ethers including DB24C8 and BMP26C8.17 In the X-ray structure of [2]pseudorotaxane [1-H⊂DB24C8]+, the crown ether was found to be clamped around the T-shaped axle using ion-dipole, hydrogen-bonding and π-stacking interactions; Scheme 1. This interpenetrated structure could then be trapped by stoppering the end of the axle to form a [2]rotaxane; Fig. 1a.18
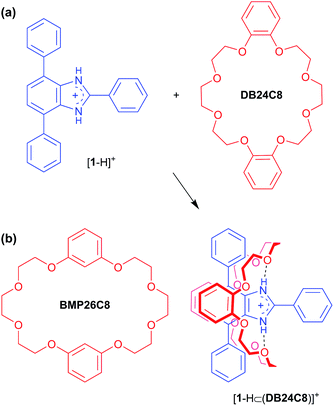 |
| Scheme 1 (a) The [2]pseudorotaxane, [1-H⊂DB24C8]+ can be formed via supramolecular interactions (ion-dipole, hydrogen-bonding and π-stacking) between the T-shaped benzimidazolium cation, [1 − H]+ and the 24-membered crown ether DB24C8.17,18a (b) Of relevance to this work, the crown ether BMP26C8 can also form a similar [2]pseudorotaxane, [1-H⊂BMP26C8]+ with [1 − H]+.18b | |
We propose that this clamped structure could be further employed to construct a suit[1]ane by bridging the two aromatic rings of the crown ether with a third linking chain; Fig. 1c.19 To this end, we report herein: (1) the synthesis and properties of suit[1]anes based on the crown ether/benzimidazolium recognition motif, (2) evidence that this type of encapsulation can be used to protect the axle unit from the interaction with external reagents; in this case, strong base and (3) that the formation of the protective three-dimensional cage can be made reversible using ring opening metathesis – i.e. the suit can be removed when the protection is no longer required.
Results and discussion
The crown ether BMP26C8 – rather than DB24C8 – was chosen as the parent macrocycle for fabrication of the suit[1]ane. Although, the initial association constant for [2]pseudorotaxane formation was lower for BMP26C8 (210 vs. 1970 M−1 for DB24C8 in CD3CN),18b the meta substitution pattern greatly simplified the incorporation of the third chain from a synthetic point-of-view. The target suit[1]ane 6a was prepared as outlined in Scheme 2. The T-shaped benzimidazolium axle [1 − H][BF4] was readily prepared using a previously reported method17 and the appended crown ethers 4 and 5 were constructed via multiple-step syntheses. The bis(hydroxymethyl) substituted crown ether 2 was prepared according to a literature method20 and brominated to afford the bis(bromomethyl) crown ether 3 which was alkylated to afford macrocycles 4 and 5 in yields of 91% and 71%, respectively. This was followed by a Grubbs' I catalysed ring closing metathesis (RCM) reaction21 to construct the suit[1]ane. No evidence of suitane formation was observed when macrocycle 4 was used indicating the appendages were not long enough to fully encapsulated the benzimidazolium axle, however, macrocycle 5 with longer appendages yielded the desired product. Subsequent hydrogenation with H2(g) and neutralization with triethylamine afforded the target suit[1]ane 6a in 42% yield; isomeric suitane 6b (7%) was isolated as a by-product, along with a small amount of free cryptand 7 (<10% by NMR). A comparison of the 1H NMR spectra of suit[1]anes 6a, 6b and free components 1 and 7 is shown in Fig. 2. The spectrum of 6a (Fig. 2a) is very different from an equimolar mixture of the non-interlocked components 1 and 7 (Fig. 2b). The downfield chemical shifts observed for the NH and a protons of the benzimidazole axle at 10.77 and 8.31 ppm respectively are attributed to hydrogen-bonding between these protons and crown ether O-atoms. The large upfield chemical shifts of the aromatic protons h, i, d and d′ indicate the crown ether is clamped around the axle in a fashion similar to that observed previously for [2]pseudorotaxanes and [2]rotaxanes involving a benzimidazolium axle.18b In the suit[1]ane 6a, the newly formed covalent link makes this clamped structure permanent even after the axle has been neutralized. Interestingly, due to the unsymmetrical nature of neutral 1, the aromatic proton i is split into two different signals at 5.95 and 5.58 ppm in the interlocked structure as compared to the 1
:
1 mixture of 1 and 7. The 1H NMR spectrum of 6b is shown in Fig. 1c. Although a similar chemical shift change is observed for the NH proton, the other protons show very different signals. For example, compared to the singlet at 3.89 ppm in 6a, the methylene proton signal j in 6b is split into two coupled doublets at 4.19 ppm and 3.90 ppm, clearly indicating different chemical environments for the two linkages.
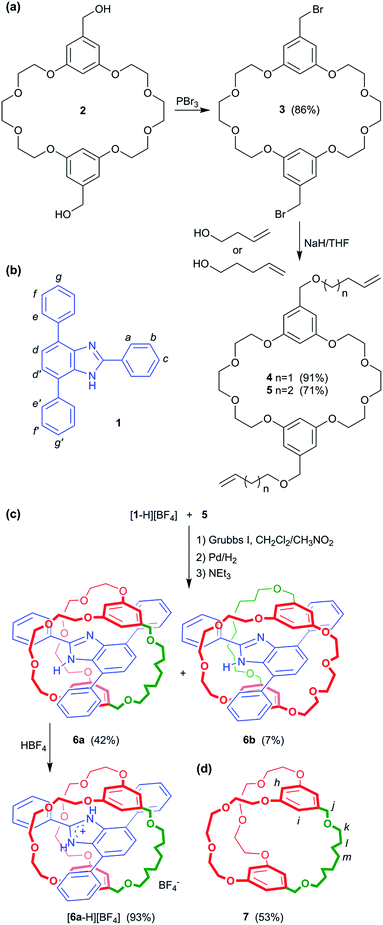 |
| Scheme 2 (a) Preparation of macrocycles 4 and 5 appended with olefinic groups. (b) T-shaped benzimidazole 1 with labelling scheme for 1H NMR spectra. (c) Template-directed synthesis of isomeric suit[1]anes 6a and 6b from the [2]pseudorotaxane [1-H⊂5]+ under ring-closing metathesis conditions and subsequent protonation of 6a to give [6a − H][BF4]. (d) Free macrobicycle 7 can be prepared under the same conditions in the absence of axle template [1 − H]+; labelling scheme for 1H NMR spectra is shown. | |
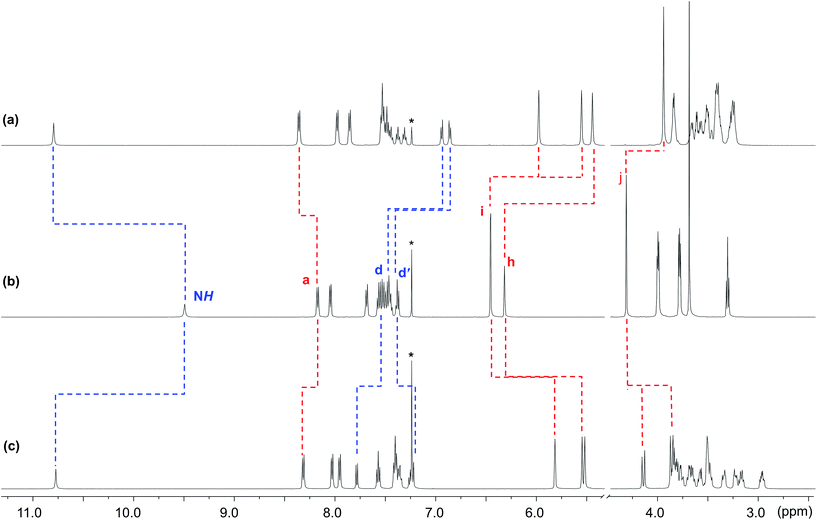 |
| Fig. 2 Comparison of the partial 1H NMR spectra (500 MHz, CDCl3) of (a) suit[1]ane 6a, (b) an equimolar solution of 1 and 7 and (c) suit[1]ane 6b (* = CHCl3). Labelling scheme is shown in Scheme 2 for 1 and 7. | |
The existence of two isomeric suit[1]anes, 6a and 6b, results from macrocycle 5 threading over, not only the phenyl ring substituted at the 2-position of the benzimidazolium cation as predicted, but also the phenyl ring substituted at the 4- (and 7-) position – albeit to a much lower extent.
Surprisingly, only one set of signals was observed for 6b. Commonly, two structures are observed for these types of neutral axles due to slow tautomerization on the NMR time scale,22 but in this case, after formation of the interlocked suit[1]ane, the NH proton is immobilized due to hydrogen-bonding to the cryptand.
The isomeric structures 6a and 6b were further identified by 2D NOESY experiments which allowed determination of the different spatial arrangements of the axle inside the cryptand; Fig. S1.† Cross peaks between alkane proton m of the cryptand and aromatic protons d and d′ of the axle were observed for 6a, while for 6b, proton m was found to be spatially closer to aromatic protons e and f. This is consistent with the structural assignments made from the 1D 1H NMR spectra shown in Fig. 2.
The structures of suit[1]anes 6a and 6b were further confirmed by single-crystal X-ray diffraction;† representations of the solid state structures are shown in Fig. 3. For the structure of 6a, three hydrogen bonds were found to be the main residual interactions between the two independent components. The benzimidazole NH is clearly hydrogen bonded to an O-atom of the cryptand with a N⋯O distance of 2.99 Å and an N–H⋯O angle of 163°. In addition one of the polyether methylene protons forms a C–H⋯N interaction with the basic N-atom of the benzimidazole moiety. The aromatic group of the benzimidazole and one of the aromatic rings of the cryptand were found to be approximately parallel with a distance of ca. 3.53 Å indicative of π-stacking. This π-stacking is also observed in the structure of 6b, but only two hydrogen bonds between the benzimidazole NH proton and cryptand O-atoms were observed. This is presumably due to the different orientation of the T-shaped axle with respect to the polyether chains of the cryptand.
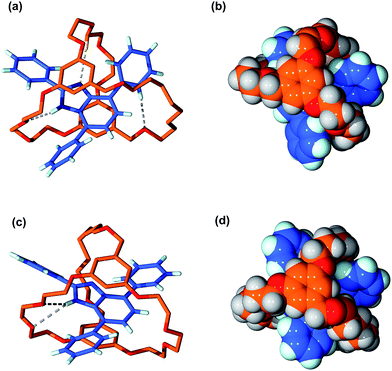 |
| Fig. 3 Single-crystal X-ray structure of suit[1]ane 6a, (a) wire-stick representation and (b) space-filling model. Single-crystal X-ray structure of suit[1]ane 6b, (c) wire-stick representation and (d) space-filling model. Colour code: O = red, N = dark blue, C of axle = blue, C of cryptand = orange, H = white. For wire-stick models, hydrogens not involved in H-bonding are omitted for clarity. | |
It is clear from the space filling models, that for both structures, the NH protons are completely buried inside the cryptand. This is an important observation and provides preliminary evidence that the benzimidazole axles of the suit[1]anes may have different reactivity when compared to the free benzimidazole 1.
In order to further prove the suit[1]anes are truly mechanically interlocked molecules, both 6a and 6b were dissolved in DMSO-d6 and heated to 100 °C for 24 h; conditions that are known to result in unthreading via slippage for some purported [2]rotaxanes.23 No evidence for disassociation of the assemblies was observed in the 1H NMR spectra under these relatively harsh conditions when compared to an equimolar solution of 1 and 7; Fig. S2.†
Benzimidazole has potential for the fabrication of fluorescent polymers due to its versatile electronic structure;24 the anionic form is especially useful and can be accessed by deprotonation with strong base.25 It was therefore of interest to compare the optical properties of the free T-shaped benzimidazole 1 with that of the same molecule encapsulated inside the suit[1]ane, 6a.
When 1 was treated with excess potassium tert-butoxide in THF, the maximum absorption band, λmax, shifted from 322 to 381 nm; Fig. 4a. Neutral 1 also has a fluorescence emission band at 389 nm when excited at 320 nm; Fig. 3c. After deprotonation, the anionic 1 exhibits strong emission bands at 424 and 449 nm when excited at 381 nm; Fig. 3d. This large wavelength shift can be attributed to the release of the steric repulsion between the N–H and the adjacent C–H from the substituted phenyl group.26 In addition, complete disappearance of the NH signal from the 1H NMR spectrum provides further evidence for deprotonation of the benzimidazole unit; Fig. S3.†
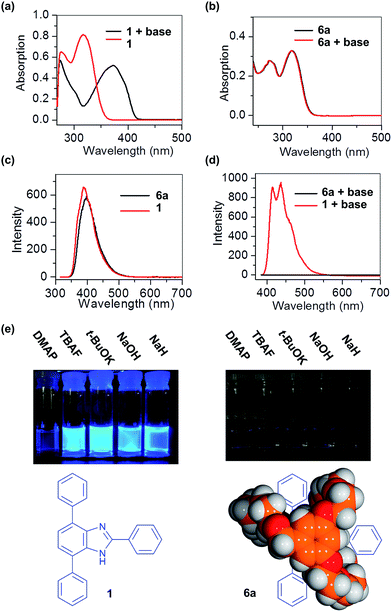 |
| Fig. 4 (a) UV/vis spectra of neutral and anionic 1, (b) UV/vis spectra of 6a and 6a after treatment with 2.0 equiv. of potassium tert-butoxide, (c) fluorescence spectra of 1 and 6a when excited at 320 nm, (d) fluorescence spectra of 1 and 6a after treatment with 2.0 equiv. of potassium tert-butoxide when excited at 381 nm, (e) deprotonation of 1 and 6a with various strong bases and then irradiated at 365 nm using a UV lamp (DMAP = 4-dimethylaminopyridine, TBAF = tetrabutylammonium fluoride, solvent = THF). | |
The suitane 6a has a maximum absorption band λmax at 328 nm in THF which is very similar to that of the free T-shaped benzimidazole 1; Fig. 3b. It also has a fluorescence emission band at 395 nm when excited at 320 nm; Fig. 3c. However, after adding excess potassium tert-butoxide to the solution of 6a, no significant change in the UV/vis or fluorescence spectra were observed. This clearly indicates that deprotonation of the benzimidazole unit inside 6a does not occur under these strongly basic conditions. A series of other moderate and strong bases (DMAP, TBAF, NaOH and NaH) were also screened, but again, no deprotonation was observed.27 The best explanation for these result is that the encapsulation of the benzimidazole by the cryptand can sterically protect the NH group and prevent deprotonation.28 This lack of reactivity was also observed by monitoring the reactions by 1H NMR spectroscopy; no changes were observed for the 1H NMR spectra of a sample of 6a when treated with excess base; Fig. S4.†
Although the protection offered by the cryptand to the benzimidazole group inside suit[1]ane 6a is interesting, traditional protecting groups used in organic synthesis can be installed and then readily removed after the synthetic transformation of interest has been achieved. As such, we were encouraged to find a way to make the “suiting up” of axle 1 demonstrated herein reversible as well. To this end, it occurred to us that for the neutral suit[1]ane, loss of the templating charge should result in a repulsion between the two neutral components – axle and cryptand – thus making the resulting [2]pseudorotaxane thermodynamically unfavourable should one of the chains of the cryptand be broken; Fig. 5. We thus used RCM to prepare the unsaturated version of the suitane 6a, 6a′ (cis/trans = 1
:
2.1) and used this as the protected species, with the idea of being able to remove the “suit” via ring opening metathesis (ROM) chemistry.29
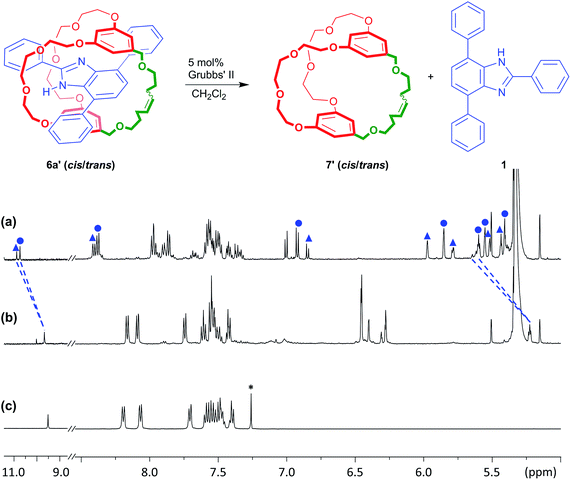 |
| Fig. 5 Removing the ‘suit’ by Ru(II) catalysed ring opening metathesis (ROM). Comparison of the partial 1H NMR spectra of: (a) 2.0 mM solution of suit[1]ane 6a′ in CD2Cl2, (b) solution from (a) with 5 mol% Grubbs II cat. added and (c) free thread 1 in CDCl3 ( = trans, = cis, = CHCl3). | |
A sample of suitane 6a′ was reacted with 5 mol% Grubbs' II catalyst and after stirring at 25 °C for 10 min, the 1H NMR spectrum was recorded. As shown in Fig. 5b, both the cis/trans isomers of 6a′ have disappeared and merged to one set of signals which are very similar in chemical shift to that observed for the free T-shaped benzimidazole axle 1. It was also noted that aromatic protons h, i, and j show similar chemical shifts to those observed for the reduced cryptand 7. This infers that the ROM catalysis produces mostly cryptand rather than oligomers or polymers; this may be due to the relatively low concentration (2.0 mM) used for the reaction.
Conclusions
Utilising the benzimidazolium/crown ether recognition motif, we have templated the synthesis of a unique MIM – a suit[1]ane – comprised of a T-shaped axle encapsulated inside a cryptand. It was further demonstrated that surrounding the benzimidazole axle with the cryptand “suit” protects the axle from the effects of a reagent such as a strong base. Moreover, this novel three-dimensional protecting group could be easily removed making this a reversible process facilitated by catalytic RCM and ROM. This concept of reversible mechanical protection may have potential applications in synthetic organic chemistry, and the synthesis of complicated systems such as molecular machines in which the rigid interlocked nature of the suit[1]ane might be coupled to the dynamics of rotaxanes and catenanes.
Acknowledgements
SJL is grateful for the awarding of a Canada Research Chair and a NSERC of Canada Discovery grant in support of this research. SJL also thanks the Canadian Foundation for Innovation, the Ontario Innovation Trust and the University of Windsor for support of the X-ray Diffraction Centre at the University of Windsor.
Notes and references
-
(a)
G. Schill, Catenanes, Rotaxanes and Knots, Academic Press, New York, NY, 1971 Search PubMed;
(b)
J. P. Sauvage, and C. Dietrich-Buchecker, Molecular Catenanes, Rotaxanes and Knots: A Journey Through the World of Molecular Topology, Wiley-VCH, Weinheim, 1999 Search PubMed;
(c) D. B. Amabilino and J. F. Stoddart, Chem. Rev., 1995, 95, 2725–2828 CrossRef CAS;
(d) J. F. Stoddart, Chem. Soc. Rev., 2009, 38, 1802 RSC;
(e) K. Zhu and S. J. Loeb, Top. Curr. Chem., 2014, 354, 213 CrossRef PubMed;
(f) J. F. Stoddart, Angew. Chem., Int. Ed., 2014, 53, 11102 CrossRef CAS PubMed;
(g) S. Erbas-Cakmak, D. A. Leigh, C. T. McTernan and A. L. Nussbaumer, Chem. Rev., 2015, 115, 10081 CrossRef CAS PubMed.
-
(a)
Templated Organic Synthesis, ed. F. Diederich and P. J. Stang, Wiley-VCH, Weinheim, 1999 Search PubMed;
(b) C. A. Schalley, T. Weilandt, J. Brüggemann and F. Vögtle, Top. Curr. Chem., 2004, 248, 141 CrossRef;
(c) F. Aricó, J. D. Badjic, S. J. Cantrill, A. H. Flood, K. C. F. Leung, Y. Liu and J. F. Stoddart, Top. Curr. Chem., 2005, 249, 203 Search PubMed;
(d) J. D. Crowley, S. M. Goldup, A. L. Lee, D. A. Leigh and R. T. McBurney, Chem. Soc. Rev., 2009, 38, 1530 RSC;
(e) J. E. Beves, B. A. Blight, C. J. Campbell, D. A. Leigh and R. T. McBurney, Angew. Chem., Int. Ed., 2011, 50, 9260 CrossRef CAS PubMed.
- R. S. Forgan, J. P. Sauvage and J. F. Stoddart, Chem. Rev., 2011, 111, 5434 CrossRef CAS PubMed.
-
(a) P. L. Anelli, N. Spencer and J. F. Stoddart, J. Am. Chem. Soc., 1991, 113, 5131 CrossRef CAS PubMed;
(b) R. A. Bissell, E. Cordova, A. E. Kaifer and J. F. Stoddart, Nature, 1994, 369, 133 CrossRef CAS;
(c) S. J. Loeb and J. A. Wisner, Chem. Commun., 2000, 1939 RSC;
(d) G. J. E. Davidson, S. Sharma and S. J. Loeb, Angew. Chem., Int. Ed., 2010, 49, 4938 CrossRef CAS PubMed;
(e) S. J. Loeb, J. Tiburcio and S. J. Vella, Chem. Commun., 2006, 1598 RSC;
(f) N. D. Suhan, L. Allen, M. T. Gharib, E. Viljoen, S. J. Vella and S. J. Loeb, Chem. Commun., 2011, 47, 5991 RSC;
(g) Y. Yamada, N. Mihara, S. Shibano, K. Sugimoto and K. Tanaka, J. Am. Chem. Soc., 2013, 135, 11505 CrossRef CAS PubMed;
(h) S. Dong, J. Yuan and F. Huang, Chem. Sci., 2014, 5, 247 RSC;
(i) J. E. M. Lewis, J. Winn, L. Cera and S. M. Goldup, J. Am. Chem. Soc., 2016, 138, 16329 CrossRef CAS PubMed.
-
(a) M. Asakawa, P. R. Ashton, V. Balzani, A. Credi, C. Hamers, G. Mattersteig, M. Montali, A. N. Shipway, N. Spencer, J. F. Stoddart, M. S. Tolley, M. Venturi, A. J. P. White and D. J. Williams, Angew. Chem., Int. Ed., 1998, 37, 333 CrossRef CAS;
(b) A. L. Hubbard, G. J. E. Davidson, R. H. Patel, J. A. Wisner and S. J. Loeb, Chem. Commun., 2004, 138 RSC;
(c) L. Wang, M. O. Vysotsky, A. Bogdan, M. Bolte and V. Bohmer, Science, 2004, 304, 1312 CrossRef CAS PubMed;
(d) Z. Meng, Y. Han, L. Wang, J. Xiang, S. He and C. Chen, J. Am. Chem. Soc., 2015, 137, 9739 CrossRef CAS PubMed;
(e) H. Li, H. Zhang, A. D. Lammer, M. Wang, X. Li, V. M. Lynch and J. L. Sessler, Nat. Chem., 2015, 7, 1003 CrossRef CAS PubMed;
(f) S. Li, H. Zhang, X. Xu and Y. Liu, Nat. Commun., 2015, 6, 7590 CrossRef PubMed;
(g) I. R. Fernando, M. Frasconi, Y. Wu, W. Liu, M. R. Wasielewski, W. A. Goddard III and J. F. Stoddart, J. Am. Chem. Soc., 2016, 138, 10214 CrossRef CAS PubMed.
-
(a) J. Rotzler and M. Mayor, Chem. Soc. Rev., 2013, 42, 44 RSC;
(b) C. J. Bruns and J. F. Stoddart, Nat. Nanotechnol., 2013, 8, 9 CrossRef CAS PubMed;
(c) L. Gao, Z. Zhang, B. Zheng and F. Huang, Polym. Chem., 2014, 5, 5734 RSC;
(d) X. Fu, Q. Zhang, S. Rao, D. Qu and H. Tian, Chem. Sci., 2016, 7, 1696 RSC.
-
(a) J.-F. Ayme, J. E. Beves, C. J. Campbell and D. A. Leigh, Chem. Soc. Rev., 2013, 42, 1700 RSC;
(b) J.-F. Ayme, J. E. Beves, D. A. Leigh, R. T. McBurney, K. Rissanen and D. Schultz, Nat. Chem., 2012, 4, 15 CrossRef CAS PubMed;
(c) N. Ponnuswamy, F. B. L. Cougnon, J. M. Clough, G. Dan Pantoş and J. K. M. Sanders, Science, 2012, 338, 783 CrossRef CAS PubMed;
(d) G. Gil-Ramírez, S. Hoekman, M. O. Kitching, D. A. Leigh, I. Vitorica-Yrezabal and G. Zhang, J. Am. Chem. Soc., 2016, 138, 13159 CrossRef PubMed;
(e) G. Zhang, G. Gil-Ramírez, A. Markevicius, C. Browne, I. J. Vitorica-Yrezabal and D. A. Leigh, J. Am. Chem. Soc., 2015, 137, 10437 CrossRef CAS PubMed;
(f) J. J. Danon, A. Krüger, D. A. Leigh, J.-F. Lemonnier, A. J. Stephens, I. J. Vitorica-Yrezabal and S. L. Woltering, Science, 2017, 355, 159 CrossRef CAS PubMed.
-
(a) K. S. Chichak, S. J. Cantrill, A. R. Pease, S.-H. Chiu, G. W. V. Cave, J. L. Atwood and J. F. Stoddart, Science, 2004, 304, 1308 CrossRef CAS PubMed;
(b) C. D. Meyer, R. S. Forgan, K. S. Chichak, A. J. Peters, N. Tangchaivang, G. W. V. Cave, S. I. Khan, S. J. Cantrill and J. F. Stoddart, Chem.–Eur. J., 2010, 16, 12570 CrossRef CAS PubMed;
(c) S.-L. Huang, Y.-J. Lin, Z.-H. Li and G.-X. Jin, Angew. Chem., Int. Ed., 2014, 53, 11218 CrossRef CAS PubMed;
(d) T. Kim, N. Singh, J. Oh, E. Kim, J. Jung, H. Kim and K. Chi, J. Am. Chem. Soc., 2016, 138, 8368 CrossRef CAS PubMed;
(e) N. Singh, D. Kim, D. H. Kim, E.-H. Kim, H. Kim, M. S. Lah and K.-W. Chi, Dalton Trans., 2017, 46, 571 RSC.
-
(a) V. Balzani, A. Credi, F. Raymo and J. F. Stoddart, Angew. Chem., Int. Ed., 2000, 39, 3348 CrossRef CAS;
(b) J. D. Badjić, V. Balzani, A. Credi, S. Silvi and J. F. Stoddart, Science, 2004, 303, 1845 CrossRef PubMed;
(c) W. R. Browne and B. L. Feringa, Nat. Nanotechnol., 2006, 1, 25 CrossRef CAS PubMed;
(d) E. Kay, D. Leigh and F. Zerbetto, Angew. Chem., Int. Ed., 2007, 46, 72 CrossRef CAS PubMed;
(e)
V. Balzani, A. Credi and M. Venturi, Molecular Devices and Machines: Concepts and Perspectives for the Nanoworld, Wiley-VCH, 2008 Search PubMed;
(f) S. Erbas-Cakmak, D. A. Leigh, C. T. McTernan and A. L. Nussbaumer, Chem. Rev., 2015, 115, 10081 CrossRef CAS PubMed;
(g) C. Cheng and J. F. Stoddart, ChemPhysChem, 2016, 17, 1780 CrossRef CAS PubMed;
(h) D. A. Leigh, Angew. Chem., Int. Ed., 2016, 55, 14506 CrossRef CAS PubMed.
- E. A. Neal and S. M. Goldup, Chem. Commun., 2014, 50, 5128 RSC.
-
(a) E. H. Buston, J. R. Young and H. L. Anderson, Chem. Commun., 2000, 905 RSC;
(b) R. Eelkema, K. Maeda, B. Odell and H. L. Anderson, J. Am. Chem. Soc., 2007, 129, 12384 CrossRef CAS PubMed;
(c) E. Arunkumar, C. C. Forbes, B. C. Noll and B. D. Smith, J. Am. Chem. Soc., 2005, 127, 3288 CrossRef CAS PubMed;
(d) J. J. Gassensmith, J. M. Baumes and B. D. Smith, Chem. Commun., 2009, 6329 RSC;
(e) G. T. Spence, G. V. Hartland and B. D. Smith, Chem. Sci., 2013, 4, 4240 RSC;
(f) M. Franz, J. A. Januszewski, D. Wendinger, C. Neiss, L. D. Movsisyan, F. Hampel, H. L. Anderson, A. Görling and R. R. Tykwinski, Angew. Chem., Int. Ed., 2015, 54, 6645 CrossRef CAS PubMed;
(g) L. D. Movsisyan, M. Franz, F. Hampel, A. L. Thompson, R. R. Tykwinski and H. L. Anderson, J. Am. Chem. Soc., 2016, 138, 1366 CrossRef CAS PubMed.
-
(a) M. J. Frampton and H. L. Anderson, Angew. Chem., Int. Ed., 2007, 46, 1028 CrossRef CAS PubMed;
(b) K. Sugiyasu, Y. Honsho, R. M. Harrison, A. Sato, T. Yasuda, S. Seki and M. Takeuchi, J. Am. Chem. Soc., 2010, 132, 14754 CrossRef CAS PubMed;
(c) J. Terao, Y. Tanaka, S. Tsuda, N. Kambe, M. Taniguchi, T. Kawai, A. Saeki and S. Seki, J. Am. Chem. Soc., 2009, 131, 18046 CrossRef CAS PubMed.
- C. B. Caputo, K. Zhu, V. N. Vukotic, S. J. Loeb and D. W. Stephan, Angew. Chem., Int. Ed., 2012, 52, 960 CrossRef PubMed.
-
(a) D. A. Leigh, V. Marcos and M. R. Wilson, ACS Catal., 2014, 4, 4490 CrossRef CAS;
(b) V. Blanco, D. A. Leigh and V. Marcos, Chem. Soc. Rev., 2015, 44, 5341 RSC;
(c) A. Martinez-Cuezva, C. Lopez-Leonardo, D. Bautista, M. Alajarin and J. Berna, J. Am. Chem. Soc., 2016, 138, 8726 CrossRef CAS PubMed.
-
(a) A. R. Williams, B. H. Northrop, T. Chang, J. F. Stoddart, A. J. P. White and D. J. Williams, Angew. Chem., Int. Ed., 2006, 45, 6665 CrossRef CAS PubMed;
(b) H. Hou, K. C.-F. Leung, D. Lanari, A. Nelson, J. F. Stoddart and R. H. Grubbs, J. Am. Chem. Soc., 2006, 128, 15358 CrossRef CAS PubMed;
(c) B. H. Northrop, F. Aricó, N. Tangchiavang, J. D. Badjić and J. F. Stoddart, Org. Lett., 2006, 8, 3899 CrossRef CAS PubMed.
-
(a) D. Castillo, P. Astudillo, J. Mares, F. J. Gonzlez, A. Vela and J. Tiburcio, Org. Biomol. Chem., 2007, 5, 2252 RSC;
(b) L. Li and G. J. Clarkson, Org. Lett., 2007, 9, 497 CrossRef CAS PubMed;
(c) N. Farahani, K. Zhu, N. Noujeim and S. J. Loeb, Org. Biomol. Chem., 2014, 12, 4824 RSC.
- N. Noujeim, K. Zhu, V. N. Vukotic and S. J. Loeb, Org. Lett., 2012, 14, 2484 CrossRef CAS PubMed.
-
(a) K. Zhu, V. N. Vukotic and S. J. Loeb, Angew. Chem., Int. Ed., 2012, 51, 2168 CrossRef CAS PubMed;
(b) K. Zhu, V. N. Vukotic, N. Noujeim and S. J. Loeb, Chem. Sci., 2012, 3, 3265 RSC;
(c) N. Farahani, K. Zhu and S. J. Loeb, ChemPhysChem, 2016, 17, 1875 CrossRef CAS PubMed.
- For related structures see:
(a) W. S. Bryant, J. W. Jones, P. E. Mason, I. Guzei, A. L. Rheingold, F. R. Fronczek, D. S. Nagvekar and H. W. Gibson, Org. Lett., 1999, 1, 1001 CrossRef CAS PubMed;
(b) S. Li, M. Liu, B. Zheng, K. Zhu, F. Wang, N. Li, X. Zhao and F. Huang, Org. Lett., 2009, 11, 3350 CrossRef CAS PubMed;
(c) A. Pun, D. A. Hanifi, G. Kiel, E. O'Brien and Y. Liu, Angew. Chem., Int. Ed., 2012, 51, 13119 CrossRef CAS PubMed;
(d) M. Zhang, X. Yan, F. Huang, Z. Niu and H. W. Gibson, Acc. Chem. Res., 2014, 47, 1995 CrossRef CAS PubMed.
- F. Wang, Q. Zhou, K. Zhu, S. Li, C. Wang, M. Liu, N. Li, F. R. Fronczek and F. Huang, Tetrahedron, 2009, 65, 1488 CrossRef CAS.
-
(a) A. F. M. Kilbinger, S. J. Cantrill, A. W. Waltman, M. W. Day and R. H. Grubbs, Angew. Chem., Int. Ed., 2003, 42, 3281 CrossRef CAS PubMed;
(b) E. N. Guidry, S. J. Cantrill, J. F. Stoddart and R. H. Grubbs, Org. Lett., 2005, 7, 2129 CrossRef CAS PubMed;
(c) S. Dasgupta and J. S. Wu, Chem. Sci., 2012, 3, 425 RSC.
- I. Elguraish, K. Zhu, L. A. Hernandez, H. Amarne, J. Luo, V. N. Vukotic and S. J. Loeb, Dalton Trans., 2015, 44, 898 RSC.
- M. Bolla, J. Tiburcio and S. J. Loeb, Tetrahedron, 2008, 64, 8423 CrossRef CAS.
- J. Kulhánek and F. Bureš, Beilstein J. Org. Chem., 2012, 8, 25 CrossRef PubMed.
- J. D. Harris, C. Mallet, C. Mueller, C. Fischer and K. R. Carter, Macromolecules, 2014, 47, 2915 CrossRef CAS.
- I. Nurulla, A. Tanimoto, K. Shiraishi, S. Sasaki and T. Yamamoto, Polymer, 2002, 43, 1287 CrossRef CAS.
-
(a) Y. Tachibana, H. Kawasaki, N. Kihara and T. Takata, J. Org. Chem., 2006, 71, 5093 CrossRef CAS PubMed;
(b) J. Berná, A. M. Brouwer, S. M. Fazio, N. Haraszkiewicz, D. A. Leigh and C. M. Lennon, Chem. Commun., 2007, 1910 RSC;
(c) S. Lee, C.-H. Chen and A. H. Flood, Nat. Chem., 2013, 5, 704 CrossRef CAS PubMed.
- The benzimidazolium [1 − H]+ has a pKa of 4.48 (J. Kempf, N. Noujeim, A. R. Schmitzer, RSC Adv., 2014, 4, 42293) which is fairly acidic compared to secondary ammonium ions most often used as axles in rotaxanes. Thus, the first deprotonation of [1 − H]+ of suit[1]ane is fairly facile. However deprotonation of the NH of the neutral rotaxane is quite difficult; not only because of the steric effort of wearing the suit, but also because of the thermodynamically unfavourable product – i.e. the complex of an anionic axle inside an electron rich cryptand.
- M. Scholl, S. Ding, C. W. Lee and R. H. Grubbs, Org. Lett., 1999, 1, 953 CrossRef CAS PubMed.
Footnote |
† Electronic supplementary information (ESI) available: Synthetic details and full characterisation of all new compounds. CCDC 1533271 and 1533272. For ESI and crystallographic data in CIF or other electronic format see DOI: 10.1039/c7sc00790f |
|
This journal is © The Royal Society of Chemistry 2017 |