DOI:
10.1039/D3QO01610B
(Review Article)
Org. Chem. Front., 2024,
11, 217-235
Recent advances in photochemical and electrochemical strategies for the synthesis of sulfonyl fluorides
Received
1st October 2023
, Accepted 13th November 2023
First published on 14th November 2023
Abstract
Sulfonyl fluorides display unique reactivity in organic synthesis and have wide applications in drug discovery, chemical biology, and materials science. Against the backdrop of increasing demands for environmental friendliness and sustainability, photochemical and electrochemical strategies have recently emerged as alternative methods for synthesizing diverse sulfonyl fluorides. This review highlights the recent advancements in these strategies, emphasizing their novel features and proposed mechanisms. We expect this review to inspire additional novel approaches to access diverse sulfonyl fluorides.
1. Introduction
It has been more than a century since the first sulfonyl fluoride was synthesized.1 Since then, this class of compounds has continued to garner considerable attention from organic chemists and biochemists, particularly after sulfur(VI) fluoride exchange (SuFEx) came to be regarded as the second-generation click reaction introduced by Sharpless and colleagues in 2014.2 Sulfonyl fluorides are used to synthesize3 sulfonamides,4 sulfones,5 and sulfonate esters,6 and in 18F radiolabelling,7 and have applications8 in chemical biology,9 drug discovery,10 and materials science.11 Consequently, numerous methods have been developed for the synthesis of sulfonyl fluorides. The classical synthetic route involves chloride–fluoride exchange with a parent sulfonyl chloride,12 but the low accessibility and stability of sulfonyl chlorides limit their utility. Afterward, various alternative methods were developed using more readily available starting materials such as sulfonic acid, sulfinates, sulfonamides, and aryl halides.13 However, these approaches often require stoichiometric oxidants, harsh reaction conditions, or transition metals, leading to high costs, poor chemo-selectivity, and toxicity. Therefore, environmentally benign and sustainable methodologies are highly desirable for the efficient synthesis of sulfonyl fluorides.
Photocatalysis, which harnesses light to form new chemical bonds under mild conditions, typically enables reactions with exceptional functional group compatibility and avoids the use of toxic and hazardous reagents.14 Electrosynthesis has also emerged as an efficient and sustainable platform for selective molecular transformations.15 The direct use of traceless and clean electrons as redox reagents obviates the need for stoichiometric chemical redox reagents, making such reactions more efficient and environmentally friendly. Although several reviews have been published on synthetic methodologies to access sulfonyl fluorides,16 none have focused solely on photochemical and electrochemical strategies. Thus, this review exclusively summarizes the achievements in the construction of sulfonyl fluorides via photocatalysis and electrocatalysis in the past several years, with emphasis on their novel features and proposed mechanisms (Scheme 1). A comprehensive overview of the current challenges and future prospects for advancing the practical applications of photo- and electro-reactions for sulfonyl fluoride synthesis is also provided.
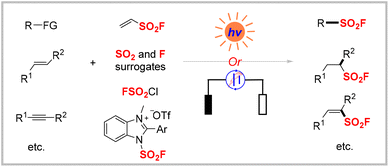 |
| Scheme 1 Photochemical and electrochemical strategies towards diverse sulfonyl fluorides. | |
2. Photochemical synthesis of sulfonyl fluorides
As photocatalysis has matured over the past several decades, many photoreactions have been accomplished, but this practical and operationally simple technology is seldom implemented for sulfonyl fluorides. In this section, we will present the progress in the synthesis of sulfonyl fluorides by photocatalysis using different sulfonyl fluoride sources.
2.1 Direct use of sulfonyl fluoride hubs
Ethylene sulfonyl fluoride (ESF) is a readily available and versatile compound that has been widely utilized as a sulfonyl fluoride hub to access various alkyl sulfonyl fluorides.17 On the one hand, owing to their highly electrophilic C
C double bond, ESF and other alkenylsulfonyl fluorides are strong Michel acceptors and readily react with various nucleophiles, such as amines, alcohols, and 1,3-dicarbonyl compounds.2,17a–c On the other hand, the strong electron-withdrawing property of the SO2F group also makes ESF act as a dienophile in Diels–Alder reactions.17h In 2018, Mykhailiuk et al. reported one example of a photocatalytic [2 + 2] cycloaddition reaction of ESF with N-benzylmaleimide to access the corresponding adduct in 29% yield (Scheme 2, top).18 In 2020, the Qin group reported another efficient photocatalytic [2 + 2] cycloaddition reaction between ESF and pyridine or isoquinolone (Scheme 2, bottom).19 In this photo-induced cycloaddition reaction, various pyridones and isoquinolones were transformed into unique cyclobutane-fused pyridinyl sulfonyl fluorides in good to excellent yields. In addition, 1-bromoethene-1-sulfonyl fluoride, as another sulfonyl fluoride hub prepared from ESF, was also applied to this cycloaddition reaction under the same conditions and afforded the desired products in moderate to good yields. Besides, upon the reliable SuFEx reaction, adduct products were smoothly converted into sulfonates and sulphonamides, which have potential applications in drug discovery and development.
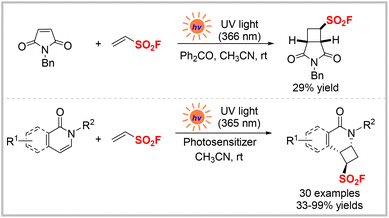 |
| Scheme 2 Photocatalytic cycloaddition reactions of ethylene sulfonyl fluoride (ESF). | |
Apart from being involved in cycloaddition reactions, ESF can also serve as a radical acceptor for the photochemical synthesis of aliphatic sulfonyl fluorides. In 2019, Liao and co-workers demonstrated a photocatalytic strategy for the preparation of a series of sulfonyl fluorides from redox-active N-hydroxyphthalimide (NHPI) esters derived from naturally abundant carboxylic acids and ESF (Scheme 3).20 This method employed Eosin Y-Na2 as a metal-free photocatalyst and a Hantzsch ester as the reductant to produce various aliphatic sulfonyl fluorides in moderate to high yields. Notably, amino acids, peptides, and several drugs were also applicable to this transformation. Derivatization to pharmaceutically relevant motifs such as sultams, sulfonate, and sulfonyl azide demonstrated the potential application of SO2F-containing products. A plausible mechanism for this reaction is shown in Scheme 3, in which Eosin Y-Na2 is initially excited to Eosin Y-Na2*, which is then reduced by the Hantzsch ester. The resultant Eosin Y-Na2 radical anion is oxidized by the NHPI ester to regenerate the catalyst and afford the NHPI ester radical anion, which undergoes decarboxylation to release CO2 and give an alkyl radical. The latter is added to ESF and subsequently abstracts a hydrogen atom from the Hantzsch ester radical to generate the desired product.
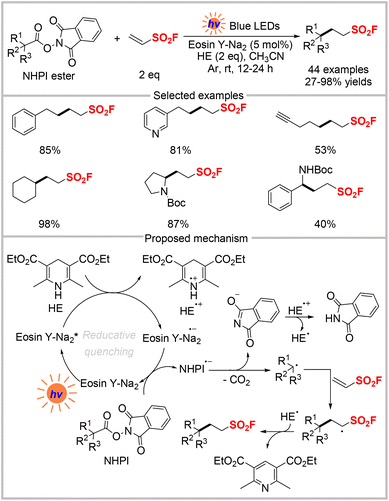 |
| Scheme 3 Photocatalytic radical addition reaction of N-hydroxyphthalimide (NHPI) esters and ESF. | |
In 2020, Qin and co-workers developed a pre-activation-free method for synthesizing sulfonyl fluorides from readily-available alkyl iodides and ESF. In this approach, Mn2(CO)10 was used as the photocatalyst under visible-light irradiation (Scheme 4).21 This methodology converted a wide range of 1°, 2°, and 3° alkyl iodides, many of which were derived from natural products, into aliphatic sulfonyl fluorides in the presence of a Hantzsch ester as the hydrogen source. Several large-scale experiments also proceeded smoothly. The proposed mechanism of this reaction is shown in Scheme 4, in which Mn2(CO)10 first undergoes homolytic cleavage of its Mn–Mn bond to generate a Mn(CO)5 radical. The Mn(CO)5 radical reacts with an alkyl iodide to produce alkyl radical A and MnI(CO)5. The radical addition of alkyl radical A to ESF affords a new alkyl radical B, which then traps hydrogen from the Hantzsch ester to generate the final product and dienyl radical C. Finally, the resulting dienyl radical C undergoes aromatization in the presence of MnI(CO)5 to produce the pyridine-HI salt D. The Mn(CO)5 radical is regenerated, thus completing the catalytic cycle.
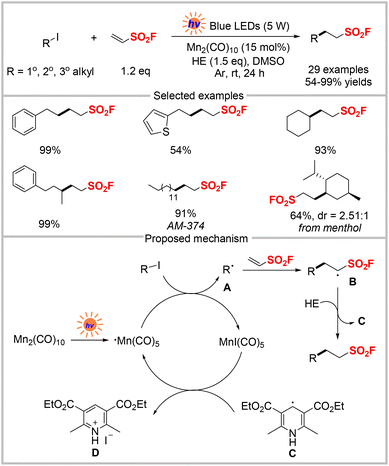 |
| Scheme 4 Photocatalytic radical addition reaction of alkyl iodides with ESF. | |
Su, Li, and collaborators recently disclosed the conversion of vinyl fluorosulfates, as FSO2-containing compounds, into β-keto sulfonyl fluorides through visible light-induced catalytic radical rearrangement (Scheme 5).22 Under the optimal conditions, a variety of aromatic vinyl fluorosulfates with different electronic and steric properties were tolerated, generating the corresponding products in 33–90% yields. However, acyclic or aliphatic ketones were unsuitable for this transformation. The authors proposed a mechanism based on the control experiments, crossover experiments, and DFT calculations, as outlined in Scheme 5. In this mechanism, an excited-state species [IrIII]* is generated from [IrIII] under visible light irradiation. There are two pathways by which [IrIII]* can interact with the vinyl fluorosulfate substrate. In pathway A, vinyl fluorosulfate (ET = 134 kJ mol−1) undergoes homolytic cleavage via energy transfer from the excited state [IrIII]* (ET = 251 kJ mol−1) to produce an enol radical and FSO2 radical. Subsequent recombination of these two radicals generates the final β-keto sulfonyl fluoride. In pathway B, the excited-state [IrIII]*
is oxidized by vinyl fluorosulfate (Ered1/2 = +1.30 V vs. SCE) to produce the [IrIV] species and a radical anion. Upon subsequent radical fragmentation/reconstruction and oxidation by [IrIV], the final product is formed, and the catalyst is regenerated.
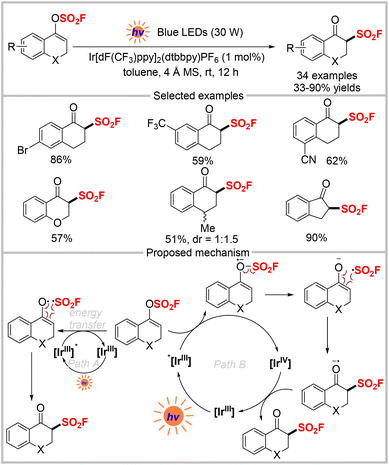 |
| Scheme 5 Photocatalytic rearrangement of vinyl fluorosulfates. | |
Glorius and colleagues very recently developed a bench-stable bifunctional reagent, (diphenylmethylene)sulfamoyl fluoride. This compound undergoes σ-bond homolysis via the inherent weak N–S bond (≈70 kcal mol−1) through energy transfer catalysis to construct protected β-amino sulfonyl fluorides from alkenes (Scheme 6).23 This novel approach featured a wide substrate scope with good functional group tolerance. Gaseous ethylene and propylene afforded the target sulfonate esters from SuFEx and sulfonyl fluoride, respectively. The reaction could also be controllably switched to yield aliphatic sulfonyl fluorides in the presence of a thiol-based hydrogen atom donor. Under the optimal conditions, terminal alkenes, unactivated 1,2-substituted alkenes, and 1,1-disubstituted alkenes were all tolerated in this hydro-fluorosulfonylation reaction. Additionally, the synthetic scope of β-imino sulfonyl fluorides was showcased by transforming them into biologically-active β-lactams. The proposed mechanism of this divergent reaction is shown in Scheme 6, in which the photocatalyst is excited by a blue LED and undergoes energy transfer with (diphenylmethylene)sulfamoyl fluoride to form the corresponding triplet state. After homolysis of the N–S σ-bond, a sulfonyl fluoride radical and an iminyl radical are formed. The former is then added to the alkene to form a carbon-centered radical that recombines with the iminyl radical to give the imino-fluorosulfonylated product. It may also abstract a hydrogen atom from a hydrogen donor to yield an aliphatic sulfonyl fluoride.
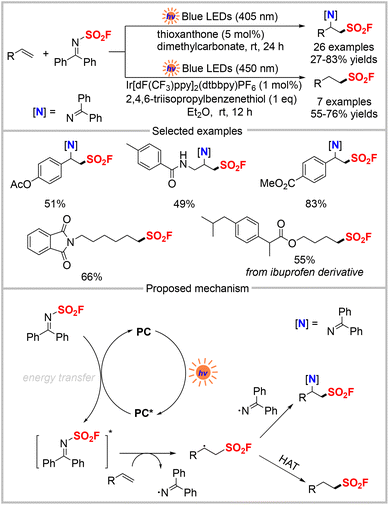 |
| Scheme 6 Chemodivergent fluorosulfonylation of alkenes with a bifunctional reagent via energy transfer. | |
2.2 Radical SO2 insertion and fluorination strategy
Another important strategy for directly installing the sulfonyl fluoride motif involves sulfonylation with SO2 surrogates (DABSO, Na2S2O5, etc.), followed by oxidation and fluorination using F surrogates (NFSI, Selectfluor, etc.). This strategy typically involves transition-metal catalyzed cross-coupling and produces only aryl and alkenyl sulfonyl fluorides.24 A similar radical SO2 insertion and fluorination strategy has also emerged for the same purpose, which typically involves the generation of alkyl radicals.25 Conventional approaches require the use of excess oxidants or transition metals such as Ag and Cu.
Photocatalysis is one of the most effective strategies for generating reactive radicals under mild conditions. Accordingly, the radical SO2 insertion and fluorination strategy has been used in several examples of decarboxylative fluorosulfonylation through visible-light photocatalysis. In 2022, Weng and co-workers developed the first three-component decarboxylative fluorosulfonylation of aldoxime esters to prepare sulfonyl fluorides via photocatalysis. In this approach, DABSO and N-fluorobenzenesulfonimide (NFSI) were used as the sulfonyl source and fluorination reagent, respectively (Scheme 7).26 The reaction was driven by energy transfer-mediated photocatalysis, which produced diverse aliphatic sulfonyl fluorides under mild conditions with high functional group compatibility. The proposed mechanism is shown in Scheme 7, in which the triplet state [IrIII]* is generated upon irradiation with visible light. Energy transfer between [IrIII]* and the aldoxime ester produces the catalyst and the excited-state aldoxime ester, which then undergoes N–O bond homolysis to produce an iminyl radical and an alkyl radical after expelling CO2. The alkyl radical is trapped by SO2 from DABSO to afford the alkylsulfonyl radical, which then reacts with NFSI to form the final fluorosulfonylated product.
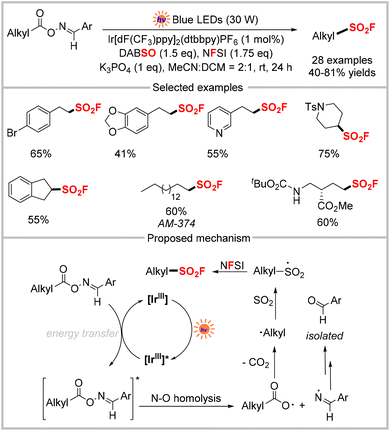 |
| Scheme 7 Catalytic decarboxylative fluorosulfonylation of aldoxime esters through energy-transfer-mediated photocatalysis. | |
Beyond the energy-transfer-mediated reaction pathway, the radical SO2 insertion/fluorination strategy was also realized via a photo-redox process. Nie and co-workers reported the photoredox decarboxylative fluorosulfonylation of various NHPI esters derived from aliphatic carboxylic acids using DABSO and NFSI (Scheme 8).27 This method enabled the construction of primary, secondary, and tertiary aliphatic sulfonyl fluorides in good to excellent yields under mild conditions. The proposed mechanism is shown in Scheme 8, in which the excited photocatalyst Ir[dF(CF3)ppy]2(dtbbpy)PF6 and DIPEA first undergo single-electron transfer (SET), which forms DIPEA˙+ and the reducing [IrII] species. Then, the reduction of NHPI esters by [IrII] produces an alkyl radical after releasing CO2, and then the catalyst is regenerated. Upon capturing SO2 from DABSO, the alkyl radical is transformed into the sulfonyl radical. Finally, the fluorosulfonylation product is generated from the reaction of an alkyl radical and NFSI via fluorine atom transfer.
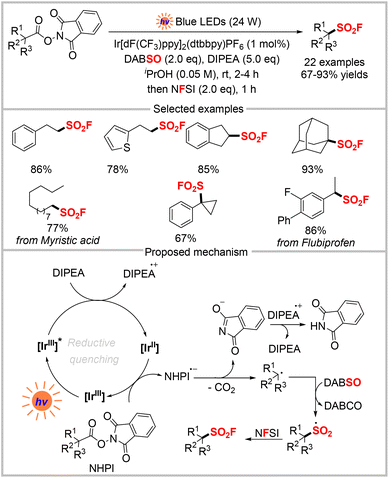 |
| Scheme 8 Catalytic photo-redox decarboxylative fluorosulfonylation of NHPI esters. | |
The Liao,28 Larionov,29 Weng,30 and Liu31 groups independently reported the construction of sulfonyl fluorides under blue-light irradiation using the same decarboxylative fluorosulfonylation approach but different radical precursors, fluoride sources, and reaction conditions. Their approaches are briefly summarized in Scheme 9.
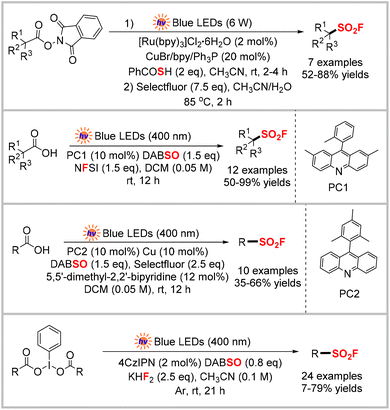 |
| Scheme 9 Catalytic photo-redox decarboxylative fluorosulfonylation to form sulfonyl fluorides. | |
In addition to using widely available carboxylic acids and their redox-active derivatives as radical precursors to synthesize sulfonyl fluorides, commonly used organotrifluoroborate salts and boronic acid pinacol esters have also been converted into alkyl sulfonyl fluorides using photocatalysis. In 2023, the Tambar group reported a method (Scheme 10)32 that enabled the synthesis of primary, secondary, and tertiary sulfonyl fluorides under mild conditions with broad functional group tolerance. Mechanistic studies, including UV-visible absorption, steady-state and time-resolved spectroscopy, and control experiments, were used to obtain the mechanism outlined in Scheme 10. An alkyl radical was generated from the oxidation of the trifluoroborate salt by the photo-excited catalyst PC3*. After capturing SO2 from DABSO, an alkyl sulfonyl radical was formed, which then oxidized the reduced acridinium species PC3−1 to regenerate the catalyst and afford the alkyl sulfonyl anion. Finally, the reaction of the alkyl sulfonyl anion with Selectfluor produced the desired sulfonyl fluoride.
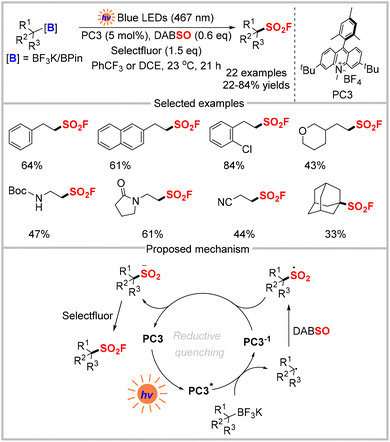 |
| Scheme 10 Catalytic photo-redox fluorosulfonylation of alkyl organoboron substrates. | |
β-Amino sulfonyl fluorides are key intermediates in the synthesis of peptide sulfonyl fluorides, which are promising covalent inhibitors.33 However, these compounds require tedious procedures that typically need 6–8 steps. Recently, Weng and co-workers reported the photoredox catalytic aminofluorosulfonylation of unactivated olefins to rapidly construct β-amino sulfonyl fluorides (Scheme 11).34 The catalytic system involved the generation of an amidyl radical, 5-exo-trig cyclization, SO2 insertion, and subsequent fluorine atom transfer. This strategy was used to obtain various aminofluorosulfonylated products in moderate to good yields under mild conditions. The authors proposed a mechanism for this three-component cascade reaction, as shown in Scheme 11. First, an amidyl radical and [IrII] are produced via the excited state [IrIII]* with the assistance of a base via proton-coupled electron transfer (PCET) under visible-light irradiation. Subsequently, 5-exo-trig cyclization of the amidyl radical generates an alkyl radical, which is then captured by SO2 from DABSO to produce an alkylsulfonyl radical. Then, the sulfonyl fluoride product and N(SO2Ph)2 radical are produced by subsequent fluorine atom transfer between the alkylsulfonyl radical and NFSI. Finally, the N(SO2Ph)2 radical is reduced by [IrII] to complete the catalytic cycle.
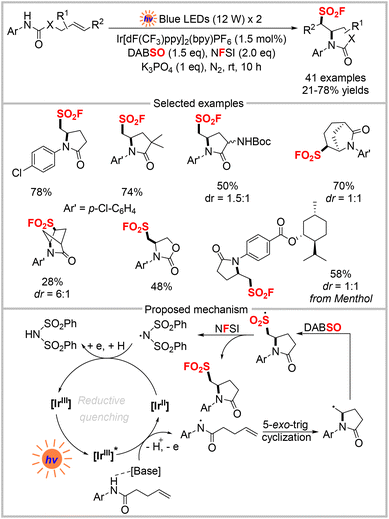 |
| Scheme 11 Catalytic photo-redox fluorosulfonylation of unactivated alkenes. | |
Four types of activated substrates, including Katritzky pyridinium salts,35 aryl diazonium salts,36,37 arylazo sulfones,38 and thianthrenium salts,39 and unactivated strained cycloalkanols,40 have also been used in this radical SO2 insertion and fluorination strategy under visible-light irradiation to synthesize aromatic and aliphatic sulfonyl fluorides (Scheme 12). For their mechanisms, radicals are typically generated through single electron transfer to the activated substrates, whereas the strained cycloalkanols are oxidized by the excited catalyst to initiate the reaction in these examples.
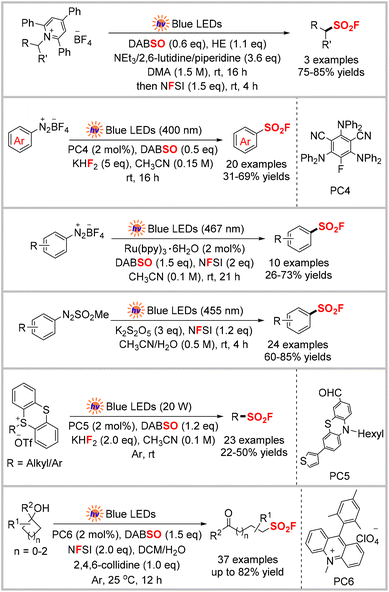 |
| Scheme 12 Catalytic photo-redox fluorosulfonylation of different substrates for the construction of sulfonyl fluorides. | |
2.3 FSO2Cl as a fluorosulfonyl radical precursor
In comparison with the classical synthetic route through chloride–fluoride exchange from sulfonyl chlorides and the above two strategies, direct one-step fluorosulfonylation to introduce the FSO2 group is an attractive alternative to access sulfonyl fluorides. Gaseous sulfuryl fluoride (SO2F2) is inexpensive and abundant but inert towards hydrolysis and is a relatively inert electrophile, which is attributed to the homolytic bond dissociation energy of its S–F bonds (81 ± 2 kcal mol−1).41 In addition, this is also exemplified by sulfuryl chloride fluoride (FSO2Cl), in which the homolytic bond dissociation energy of the S–F bond (84 ± 10 kcal mol−1) is considerably higher than that of the S–Cl bond (43 ± 10 kcal mol−1).42 In 2014, the Sharpless group disclosed that a proton can activate the exchange of S–F bonds for S–O bonds by using SO2F2 as electrophilic “FSO2+” synthons.2 Besides, other fluorosulfonylating reagents including the fluorosulfuryl imidazolium triflate salt (FDIT)43 and [4-(acetylamino)phenyl]imidodisulfuryl difluoride (AISF)44 have been widely used as electrophilic “FSO2+” synthons to synthesize sulfonyl fluorides.45 In contrast, the use of the fluorosulfonyl radical (˙SO2F) from an appropriate reagent for the radical fluorosulfonylation reaction was unknown until the first seminal example was disclosed by Liao and colleagues in 2020 (Scheme 13).46 This approach utilizes FSO2Cl as the fluorosulfonyl radical precursor and [Ir(dFCF3ppy)2dtbbpy]PF6 as the photocatalyst to obtain diverse alkenyl sulfonyl fluorides with excellent functional group compatibility. Several natural products could also be fluorosulfonylated for late-stage modification. The proposed mechanism of this photocatalytic fluorosulfonylation reaction is shown in Scheme 14. First, the excited state [IrIII]* reduces FSO2Cl via single-electron transfer to produce a fluorosulfonyl radical, which then attacks the alkene to form a carbon-centered radical. This radical reacts with another molecule of FSO2Cl to create a chlorinated product via a radical chain process. Subsequently, the fluorosulfonylation product is obtained after losing HCl. Alternatively, the redox pathway may also involve the single-electron oxidation of the carbon radical by [IrIV] and deprotonation.
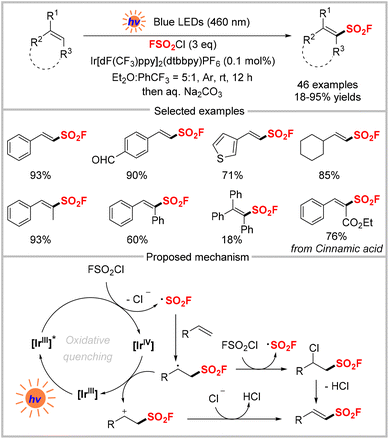 |
| Scheme 13 Fluorosulfonylation of alkenes with FSO2Cl as a radical precursor. | |
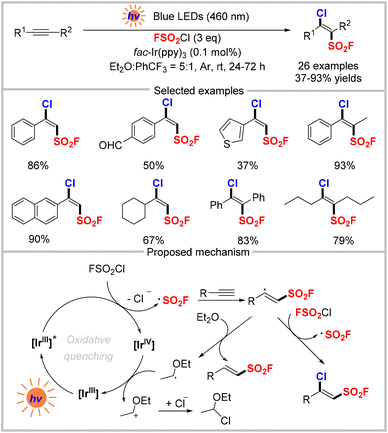 |
| Scheme 14 Chloro-fluorosulfonylation of alkynes with FSO2Cl as the radical precursor. | |
Soon after, the same group developed a similar photo-redox catalytic system to synthesize β-chloro alkenylsulfonyl fluorides (BCASFs) from alkynes under mild conditions (Scheme 14).47 Terminal and internal alkynes were transformed into the corresponding sulfonyl fluorides. As a new class of sulfonyl fluoride hubs, the BCASFs could be used in many reactions including Suzuki coupling, Sonogashira coupling, selective reduction, and nucleophilic substitution reactions with nitrogen, oxygen, and sulfur nucleophiles based on the β-chloro site. The SuFEx reactions were also applied for the late-stage modification of peptides and drugs, highlighting the potential applications of BCASFs for constructing more complex compounds. In the mechanism proposed by the authors, FSO2Cl is first reduced by the excited state [IrIII]* to produce the fluorosulfonyl radical, which undergoes radical addition to an alkyne to generate a vinyl radical. The vinyl radical further reacts with FSO2Cl to give the final product and then initiates a radical chain process. The vinyl radical might also be converted into alkenylsulfonyl fluoride via hydrogen-atom transfer from Et2O. The resulting ether-derived radical is then oxidized by [IrIV] to regenerate the catalyst and α-chlorinated diethyl ether upon addition of the chloride anion.
The above work showed that the FSO2Cl radical precursor was reduced by an excited photocatalyst to form a fluorosulfonyl radical. Our recent study disclosed a facile visible light-mediated method to access 6-keto alkenylsulfonyl fluorides (Scheme 15).48 This was based on the formation of an electron donor–acceptor (EDA) complex between FSO2Cl and propargyl alcohol. This reaction is catalyst-free, base-free, and additive-free. It can also be performed in open-air and represents the first example of an EDA complex that uses FSO2Cl as the acceptor. Radical trapping experiments indicated the participation of FSO2 radicals, and on–off light experiments supported a chain radical pathway. Based on these findings and previous literature reports, the mechanism, shown in Scheme 15, was proposed. In this mechanism, FSO2Cl and propargyl alcohol initially form an EDA complex in the group state. Upon irradiation of this EDA complex by blue LEDs, SET from propargyl alcohol to FSO2Cl occurs, which forms a ˙SO2F radical. The radical addition of ˙SO2F to alkyne affords a vinyl radical that undergoes 1,5-HAT to generate an alkyl radical. After 5-exo-trig cyclization, a secondary alkyl radical is formed, and cleavage of the cyclic C–C bond produces a ketyl radical. Subsequent SET from the ketyl radical to FSO2Cl yields the corresponding carbon cation intermediate, which finally loses a proton to give the desired product. Subsequently, this EDA complex photoactivation strategy was used to design a three-component reaction that furnished a wide range of FSO2-functionalized tetrahydropyridines.49
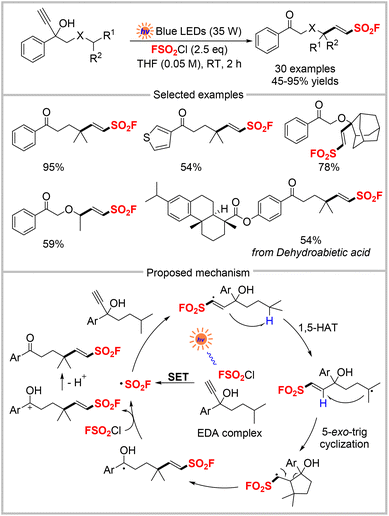 |
| Scheme 15 Fluorosulfonylation of alkenes with FSO2Cl as a radical precursor. | |
2.4 Imidazolium sulfonyl fluoride salt as a fluorosulfonyl radical precursor
Although FSO2Cl has been used as a fluorosulfonyl radical precursor under photochemical conditions, it has inherent drawbacks. First, it is a low-boiling point (7 °C) compound, making it inconvenient to prepare and handle. Second, the highly electrophilic nature of the chloride causes it to easily react with electron-rich substrates to form chlorinated byproducts, resulting in a limited substrate scope.
To tackle these limitations, Liao and co-workers recently discovered that the solid-state imidazolium sulfonyl fluoride salt (FABI) could serve as a redox-active fluorosulfonyl radical precursor under photo-redox conditions (Scheme 16).50 Compared with the previously-used FSO2Cl, this newly-developed reagent is bench-stable and compatible with various olefins, especially electron-rich alkenes and triaryl ethylenes. This radical fluorosulfonylation was used to obtain several vinyl sulfonyl fluorides in good to excellent yields. The reaction could also switch to yield β-alkoxyl sulfonyl fluorides in the presence of alcohols or acids. As shown in Scheme 16, the authors proposed a mechanism for this fluorosulfonylation reaction based on their experimental findings. First, the excited state [IrIII]*
transfers an electron to the redox-active radical precursor FABI (Ered1/2 = −1.07 V vs. SCE). Upon the homolytic cleavage of the N–S bond, the ˙SO2F radical is generated, which undergoes addition to the alkene to produce the key alkyl radical. This radical is then oxidized by the highly-oxidative [IrIV] to provide the corresponding carbon cation, which is either deprotonated to yield the vinyl sulfonyl fluoride or captured by alcohols/acids to give the difunctionalization product.
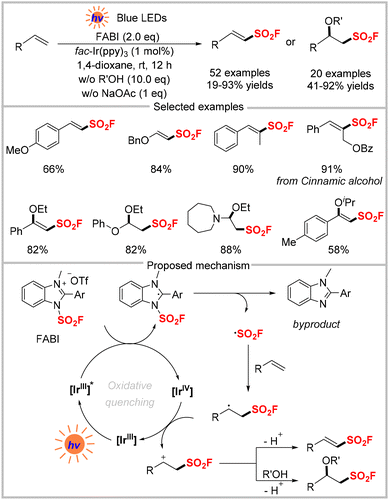 |
| Scheme 16 Photocatalytic fluorosulfonylation of alkenes with FABI as a radical precursor. | |
Continuing their previous work on the fluorocarboborylation of unsaturated hydrocarbons with the imidazolium sulfonate reagent,51 Wang and colleagues developed the same imidazolium sulfonyl fluoride salt for a radical fluorosulfonylation reaction (Scheme 17).52 Under their optimized conditions, this photocatalysis enabled the synthesis of a wide range of alkenyl sulfonyl fluorides and alkyl sulfonyl fluorides, as well as other functionalities in moderate to good yields with high regio- and stereoselectivity. Radical trapping and radical clock experiments supported the involvement of the fluorosulfonyl radical.
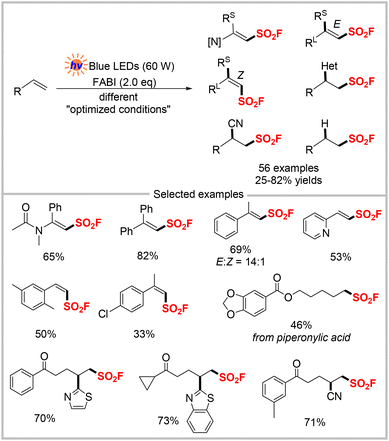 |
| Scheme 17 Divergent fluorosulfonylation of alkenes with FABI as a radical precursor. | |
Using the same 1-fluorosulfonyl benzoimidazolium triflate salt as the fluorosulfonyl radical precursor, the Liao group developed a radical hydro-fluorosulfonylation procedure for alkenes using oxygen-doped anthanthrene (ODA) as the metal-free photocatalyst and 1,4-cyclohexadiene (1,4-CHD) as the hydrogen donor (Scheme 18).53 This method constructed aliphatic sulfonyl fluorides under mild and metal-free conditions and enabled convenient later-stage modification of natural products and drugs in excellent yields in most cases. Interestingly, when (TMS)3SiH instead of 1,4-CHD was used as the hydrogen donor, this photocatalytic system could be applied for the radical hydrofluorosulfonylation of alkynes, producing the desired alkenylsulfonyl fluorides with high Z-selectivity. Mechanistically, the excited photocatalyst ODA (E1/2(ODA*/ODA˙+) = −1.76 V vs. SCE) is sufficient to reduce the FABI (E1/2 = −1.07 V vs. SCE). Then, homolytic cleavage of the N–S bond produces the ˙SO2F radical, which undergoes addition to an alkene to generate the key alkyl radical. HAT then occurs between the alkyl radical and 1,4-CHD, resulting in the formation of the desired product and a cyclohexadienyl radical. The latter can be oxidized by ODA˙+ (E1/2(ODA˙+/ODA) = +0.82 V vs. SCE) to regenerate the photocatalyst and complete the catalytic cycle.
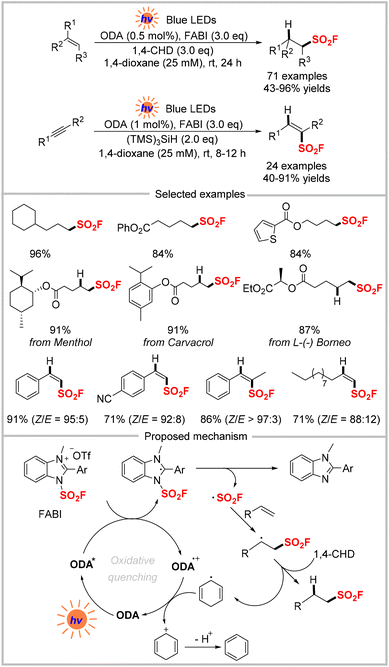 |
| Scheme 18 Photocatalytic fluorosulfonylation of alkenes/alkynes with FABI as the radical precursor. | |
Liao and colleagues reported several radical fluorosulfonylation reactions to construct diverse sulfonyl fluorides (Scheme 19) using the same redox-active FABI as the sulfonyl fluoride radical precursor and a photocatalyst. These reactions included radical fluorosulfonylation of alkenes/intramolecular arylation via a cascade reaction to access FSO2-functionalized chromanes,54 radical fluorosulfonylation of vinyl acetates,55 radical fluorosulfonyl heteroarylation of unactivated alkenes with quinoxalin-2(1H)-ones,56 and a FSO2 radical-initiated tandem addition reaction of two different olefins.57
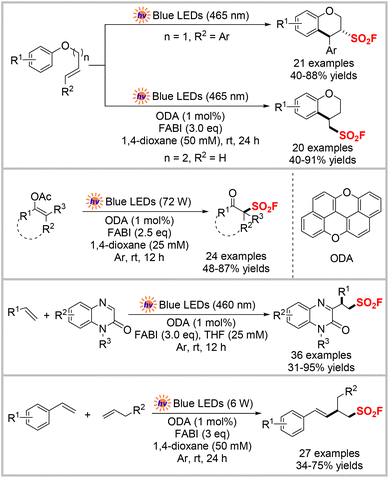 |
| Scheme 19 Photocatalytic fluorosulfonylation of different alkenes with FABI as radical precursors. | |
3. Electrochemical synthesis of sulfonyl fluorides
In the second section, we showed that photochemical strategies have become alternatives to conventional methods to access sulfonyl fluorides. These reactions occur under milder conditions. In the last five years, electrochemistry has emerged as another choice for sulfonyl fluoride synthesis, and in this section, we will summarize relevant literature on using this strategy to synthesize sulfonyl fluorides.
3.1 Direct electrochemical oxidation of thiols
Thiols and disulfide substrates can be used to directly synthesize sulfonyl fluorides without involving a sulfonyl chloride intermediate. However, this method requires the excess use of the expensive Selectfluor as an oxidant and fluorine reagent.58 In 2019, the Noël group described the first example of an electrochemical strategy to synthesize sulfonyl fluorides from thiols and disulfides. The approach employed widely-available thiols and disulfides as reagents and KF as an inexpensive and safe fluoride source under benign electrochemical conditions. This approach avoided the dependence on stoichiometric amounts of oxidants. The reaction featured a broad substrate scope, providing various aryl, heteroaryl, benzyl, and alkyl sulfonyl fluorides in moderate to high yields (Scheme 20).59 Based on their kinetics experiments and radical-trapping experiments, the authors proposed the electrochemical transformation mechanism shown in Scheme 20. First, the anodic oxidation of a thiol affords the corresponding disulfide, which is further oxidized and then reacts with nucleophilic fluoride to produce a sulfenyl fluoride. Subsequently, two successive oxidation processes generate the target sulfonyl fluoride product via sulfinyl fluoride as an inseparable intermediate. Later, the same group developed a flow chemistry protocol for the electrochemical oxidative coupling of thiols and KF.60 This approach requires only short reaction times (normally 5 min) and is scalable up to 10 mmol. This flow technology can be applied for the follow-up SuFEx reaction, which represents the first example of such a SuFEx flow reaction.
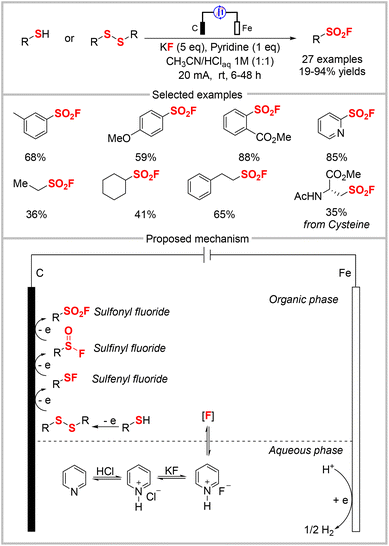 |
| Scheme 20 Electrochemical oxidative coupling of thiols and potassium fluoride. | |
3.2 FSO2Cl as a radical precursor
Sulfonyl fluoride radicals can be generated from FSO2Cl via photocatalysis, as well as electrochemical methods. In 2021, our group disclosed the electrochemical oxo-fluorosulfonylation of alkynes with FSO2Cl to synthesize β-keto sulfonyl fluorides (Scheme 21).61 This electrochemical fluorosulfonylation reaction displayed a broad substrate scope with good functional group tolerance and high chemoselectivity, under mild reaction conditions with air as the oxidant. It provided a wide range of β-keto sulfonyl fluorides in good to high yields. Interestingly, this protocol was applied to synthesize α-chloro β-keto sulfonyl fluorides using similar conditions as those for β-keto sulfonyl fluorides by simply changing the solvent from diethyl ether to tetrahydrofuran (THF). β-Keto sulfonyl fluorides are versatile building blocks that can participate in diverse reactions, including condensation, benzyne insertion, α-functionalization, and the SuFEx reaction. Preliminary studies showed that some β-keto sulfonyl fluoride derivatives display good antibacterial activities against Bursaphelenchus xylophilus and Colletotrichum gloeosporioides. Based on our control experiments, the mechanism for this diverse fluorosulfonylation was proposed, as depicted in Scheme 21. First, the reaction is initiated via single-electron transfer to FSO2Cl to produce ˙SO2F, which then adds to an alkyne to give a vinyl radical. This radical is then trapped by O2 to generate a peroxy radical, which is converted to an oxygen-centered radical via recombination and the Russell mechanism. Subsequently, the oxygen-centered radical is reduced at the cathode, followed by protonation to deliver β-keto sulfonyl fluoride. In THF, α-chloro β-keto sulfonyl fluoride is obtained in the presence of in situ-generated MgCl2 as a Lewis acid and FSO2Cl as an electrophilic chlorination reagent.
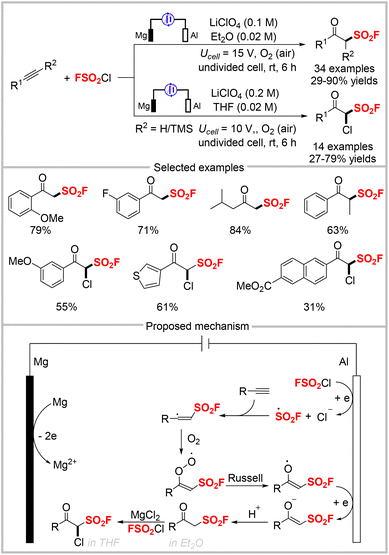 |
| Scheme 21 Electrochemical oxo-fluorosulfonylation of alkynes with FSO2Cl. | |
Although we have developed the first one-step electrochemical method for synthesizing β-keto sulfonyl fluorides from alkynes by using FSO2Cl as the radical precursor, the use of a sacrificial magnesium anode is unavoidable. Therefore, we developed an alternative electrochemical approach to obtain β-keto sulfonyl fluorides from readily-available vinyl triflates by using the inexpensive graphite felt as both the cathode and anode in 2021 (Scheme 22).62 This electrochemical fluorosulfonylation was tolerant to substrates bearing various functional groups, including halides, nitriles, methyl groups, and ketones. Notably, the substrate containing two vinyl triflates was fully fluorosulfonylated with FSO2Cl to afford the corresponding product in moderate yields. Additionally, a gram-scale reaction could be easily accomplished with improved efficiency compared with the 0.2 mmol-scale reaction, and diverse derivatizations of the product were also accomplished. The proposed mechanism for this fluorosulfonylation approach is shown at the bottom of Scheme 22. First, the cathodic reduction of FSO2Cl forms the sulfonyl fluoride radical, which upon radical addition to vinyl triflate gives an adduct radical species. β-Fragmentation of the resultant radical species forms the final product. The resulting ˙SO2CF3 radical releases SO2 to produce a ˙CF3 radical, which captures a hydrogen atom from diethyl ether to produce HCF3, as confirmed by 19F NMR analysis. The chloride anion was oxidized to a chloride radical at the anode.
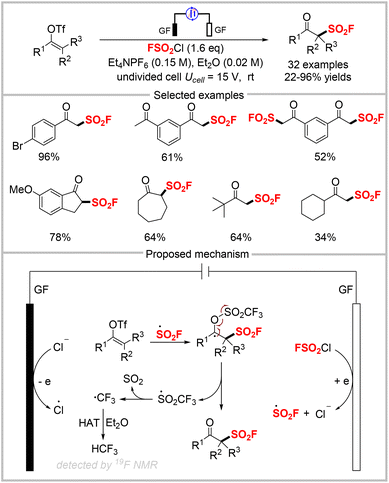 |
| Scheme 22 Electrochemical approach towards β-keto sulfonyl fluorides from vinyl triflates. | |
3.3 Anodic oxidation and fluorination strategy
In 2022, Cheng, Zhou, and co-workers reported the anodic oxidation of sulfinic salts to construct sulfonyl fluorides, wherein commercially available Et3N·HF served as the fluoride source (Scheme 23).63 In this electrochemical transformation, a series of sulfinic salts was smoothly converted to the desired aryl, aliphatic, and alkenyl sulfonyl fluorides without requiring an external oxidant. Two possible pathways were proposed by the authors, as outlined in Scheme 23. Initially, sodium sulfinate is oxidized at the anode to give the sulfonyl radical. Meanwhile, the bromide anion undergoes single-electron oxidation to afford a bromo radical. Radical–radical coupling of these two radicals generates a sulfonyl bromide intermediate, as confirmed by isolation. The subsequent halogen exchange bromide by fluoride produces the final product (path A). Alternatively, sodium sulfinate can also successively lose two electrons to generate a sulfonyl cation, which then undergoes nucleophilic addition with the bromide anion and halogen exchange to produce the product (path B). The nucleophilic attack of the sulfonyl cation by the fluoride anion directly affords the product (path C). Based on this, the authors developed a one-pot three-component reaction via a Pd-catalyzed cross-coupling between an aryl iodide and sodium sulfite, followed by an electrochemical transformation to sulfonyl fluoride.
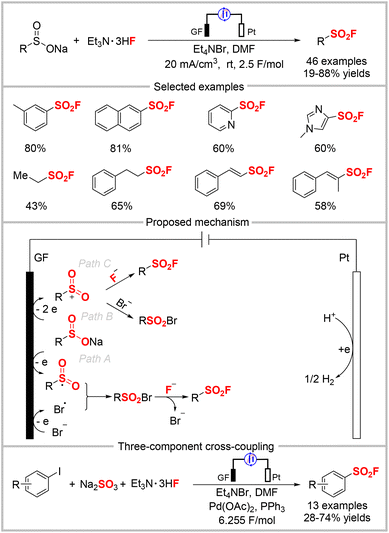 |
| Scheme 23 Anodic oxidation of sulfinic salts with Et3N·HF as the fluoride source. | |
Oh, Lee, and collaborators reported the synthesis of sulfonyl fluorides by reacting sulfonyl hydrazides and Et3N·HF under electrochemical conditions (Scheme 24).64 In contrast to the conventional method that uses the expensive Selectfluor as the fluoride reagent and elevated reaction temperatures (60 °C),65 this protocol utilizes Et3N·HF as a cheap fluoride source. The method was used to synthesize various aryl sulfonyl fluorides in good yields under mild conditions. However, some alkyl sulfonyl hydrazides were incompatible with this system, and no product was formed. Cyclic voltammetry (CV) studies indicated that nBu4NI acted both as the electrolyte and redox catalyst. Combined with the control experiments, a plausible mechanism was proposed by the authors, which is shown in Scheme 24. First, the iodide anion is oxidized to I2via I3− at the anode. Iodine is then transformed into an iodine radical via homolysis and then oxidizes the sulfonyl hydrazide to the sulfonyl hydrazine radical (path A). The sulfonyl hydrazine radical can also be generated by direct oxidization at the anode (path B). The resultant sulfonyl hydrazine radical was then oxidized by the iodine radical followed by the release of N2 to afford a sulfonyl radical, which can be oxidized either by the iodine radical or at the anode to produce the sulfonyl cation (path C or D). The cation is finally trapped by the fluoride anion to afford the target product.
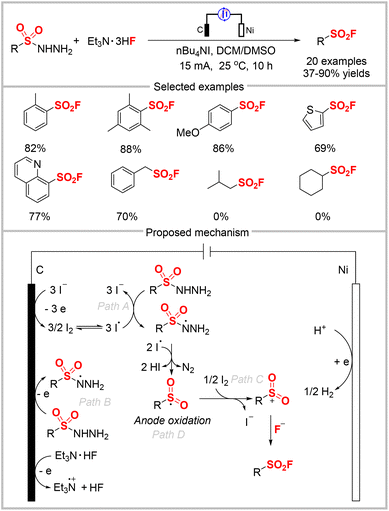 |
| Scheme 24 Anodic oxidation of sulfonyl hydrazides with Et3N·HF as the fluoride source. | |
3.4 SO2 insertion and fluorination strategy
In 2022, the Baran group developed a novel strategy involving nickel electrocatalytic sulfinylation followed by fluorination to construct sulfonyl fluorides using a one-pot, two-step procedure (Scheme 25).66 In most cases, this mild and economical approach provided higher yields of aryl sulfonyl fluorides than the established Pd-based catalysis approach.6,13d A SO2 stock solution was used as the sulfur source to avoid the poor solubility of other SO2 surrogates. In addition, aryl bromides carrying an electron-withdrawing group were compatible with the reaction, wherein NFSI was used as the fluoride source. The reaction could also be scaled-up to 45 mmol using a recycling flow system to afford the desired sulfonyl fluoride in 50% isolated yield, which was the same as the 0.3 mmol-scale reaction. The CV study showed a reversible reduction of SO2 at about −0.78 V in the presence of DIPEA, indicating that the amine coordination influenced its redox behavior. The addition of DIPEA was crucial to the reaction, as a significantly lower yield (<5%) was observed when it was absent. The proposed mechanism is presented in Scheme 25, in which SO2 is preferentially reduced to give the long-lived SO2˙− at the cathode. Then, the reduction of an in situ-generated NiII complex affords NiI, which undergoes oxidation–addition with an aryl iodide to produce the Ni(III) intermediate. The substitution of iodide and the reduction of the metal center by SO2˙− formed a SO2-coordinated aryl NiII complex. The subsequent SO2 insertion provides Ni-sulfinate, which is finally substituted by a halide to yield the aryl sulfinate product, which regenerates the catalyst. A control experiment indicated that NMPI acts as a reductive mediator to reduce the NiII complex.
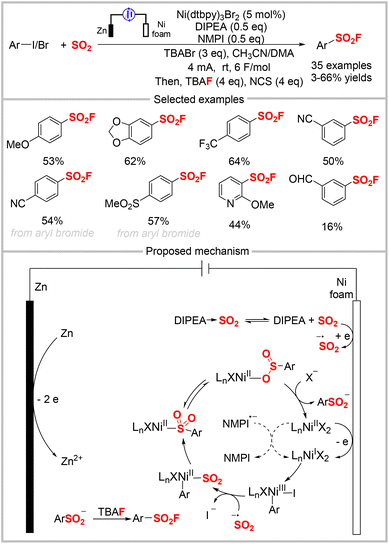 |
| Scheme 25 Nickel electrocatalytic sulfinylation and fluorination. | |
Kong, Cao, and co-workers reported the electrochemical oxidation of thianthrenium salts in combination with DABSO as the SO2 source and KHF2 as the F source to assemble sulfonyl fluorides (Scheme 26).67 Under their optimal conditions, aryl and alkyl thianthrenium salts were converted into the corresponding aryl and alkyl radicals, which was different from previous reports that mainly used thianthrenium salts as aryl radical precursors. This more practical approach does not require exogenous oxidants or catalysts. The authors proposed a mechanism for this fluorosulfonylation reaction, as outlined in Scheme 26. First, the single electron reduction of the thianthrenium salt affords a carbon-centered radical, which is captured by SO2 to produce a sulfonyl radical. The sulfonyl radical may react via two possible pathways. In the first, it combines with DABCO˙+ to afford a tethered intermediate that undergoes nucleophilic substitution to provide the final product. In the other, it is oxidized at the anode to produce a sulfonyl cation, followed by nucleophilic attack to give the product.
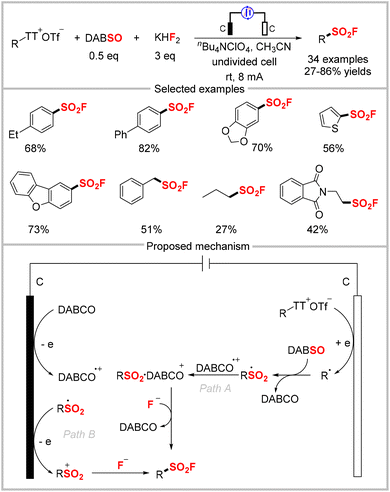 |
| Scheme 26 Electrochemical oxidation of thianthrenium salts. | |
4. Conclusions
As summarized above, several photocatalysis strategies have provided powerful alternatives for the synthesis of sulfonyl fluorides, but when using ESF as the radical acceptor, the products are limited to aliphatic ethyl-tethered ones. Radical fluorosulfonylation has been realized by using the solid-state imidazolium sulfonyl fluoride salt (FABI) as the radial precursor instead of gaseous FSO2Cl. However, these radical fluorosulfonylation reactions are still restricted to alkenes and alkynes and produce aliphatic and alkenyl sulfonyl fluorides. The synthesis of aromatic sulfonyl fluorides via radical fluorosulfonylation has not yet been reported, and the formation of imidazole as a by-product reduces the reaction efficiency. In contrast to photocatalysis, the electrosynthesis of sulfonyl fluorides is still in its early stages, with only seven reported examples under these conditions. It is expected that more approaches will be developed using this green and efficient electrochemical technology. Furthermore, the utilization of photoelectrochemistry,68 which integrates electrochemistry and molecular photocatalysis to enable transformations under mild electrode potentials without the need for exogenous chemical oxidants, remains unexplored in this field. While a flow system for the electrosynthesis of sulfonyl fluorides has been reported,59,60 increasing demands are expected for both photo- and electrosynthesis examples in flow chemistry. Although there are many examples of alkyl and alkenyl sulfonyl fluorides, there are no photo- or electrochemical methods for producing alkynyl sulfonyl fluorides. It is currently not possible to synthesize sulfonyl fluorides via radical–radical coupling using any of these reactions. There is only one known example of the synthesis of sulfonyl fluorides via direct C–H functionalization under photocatalytic conditions.69 More examples are desirable as this is more efficient than using pre-functionalized compounds as starting materials. It would also dramatically expand the reaction scope of sulfonyl fluorides. Until now, no chiral SO2F compounds have been constructed, despite reported approaches towards enantiopure compounds with a SO2F moiety.70 We expect that all of the above-mentioned directions will continue to expand in the coming years.
Conflicts of interest
There are no conflicts to declare.
Acknowledgements
We gratefully acknowledge the National Natural Science Foundation of China (22201133 and 32171724) and the Natural Science Foundation of Jiangsu Province (BK20210607) for their financial support of this work.
References
- W. Steinkopf, über Aromatische Sulfofluoride, J. Prakt. Chem., 1927, 117, 1–82 CrossRef CAS.
- J. Dong, L. Krasnova, M. G. Finn and K. B. Sharpless, Sulfur(VI) Fluoride Exchange (SuFEx): Another Good Reaction for Click Chemistry, Angew. Chem., Int. Ed., 2014, 53, 9430–9448 CrossRef CAS.
-
(a) P. K. Chinthakindi and P. I. Arvidsson, Sulfonyl fluorides (SFs): more than click reagents?, Eur. J. Org. Chem., 2018, 3648–3666 CrossRef CAS;
(b) M. Wei, D. Liang, X. Cao, W. Luo, G. Ma, Z. Liu and L. Li, A Broad-Spectrum Catalytic Amidation of Sulfonyl Fluorides and Fluorosulfates, Angew. Chem., Int. Ed., 2021, 60, 7397–7404 CrossRef CAS;
(c) C. J. Smedley, J. A. Homer, T. L. Gialelis, A. S. Barrow, R. A. Koelln and J. E. Moses, Accelerated SuFEx Click Chemistry For Modular Synthesis, Angew. Chem., Int. Ed., 2022, 61, e202112375 CrossRef CAS.
-
(a) A. V. Bogolubsky, Y. S. Moroz, P. K. Mykhailiuk, S. E. Pipko, A. I. Konovets, I. V. Sadkova and A. Tolmachev, Sulfonyl fluorides as alternative to sulfonyl chlorides in parallel synthesis of aliphatic sulfonamides, ACS Comb. Sci., 2014, 16, 192–197 CrossRef CAS;
(b) P. Mukherjee, C. P. Woroch, L. Cleary, M. Rusznak, R. W. Franzese, M. R. Reese, J. W. Tucker, J. M. Humphrey, S. M. Etuk, S. C. Kwan, C. W. am Ende and N. D. Ball, Sulfonamide Synthesis via Calcium Triflimide Activation of Sulfonyl Fluorides, Org. Lett., 2018, 20, 3943–3947 CrossRef CAS.
-
(a) L. L. Frye, E. L. Sullivan, K. P. Cusack and J. M. Funaro, Sulfonylation of organometallic reagents with arenesulfonyl fluorides: a simple one-step synthesis of sulfones, J. Org. Chem., 1992, 57, 697–701 CrossRef CAS;
(b) S. T. Shreiber and G. A. Molander, Alkyl (Het)Arylsulfones from SuFEx Reagents via Photochemical S–F Bond Activation, Org. Lett., 2023, 25, 2084–2087 CrossRef CAS.
- A. L. Tribby, I. Rodríguez, S. Shariffudin and N. D. Ball, Pd-Catalyzed Conversion of Aryl Iodides to Sulfonyl Fluorides Using SO2 Surrogate DABSO and Selectfluor, J. Org. Chem., 2017, 82, 2294–2299 CrossRef CAS.
-
(a) J. A. H. Inkster, K. Liu, S. Ait-Mohand, P. Schaffer, B. Guérin, T. J. Ruth and T. Storr, Sulfonyl Fluoride-Based Prosthetic Compounds as Potential 18F Labelling Agents, Chem. – Eur. J., 2012, 18, 11079–11087 CrossRef CAS;
(b) L. Matesic, N. A. Wyatt, B. H. Fraser, M. P. Roberts, T. Q. Pham and I. Greguric, Ascertaining the Suitability of Aryl Sulfonyl Fluorides for [18F]Radiochemistry Applications: A Systematic Investigation using Microfluidics, J. Org. Chem., 2013, 78, 11262–11270 CrossRef CAS;
(c) Q. Zheng, H. Xu, H. Wang, W.-G. H. Du, N. Wang, H. Xiong, Y. Gu, L. Noodleman, K. B. Sharpless, G. Yang and P. Wu, Sulfur [18F]Fluoride Exchange Click Chemistry Enabled Ultrafast Late-Stage Radiosynthesis, J. Am. Chem. Soc., 2021, 143, 3753–3763 CrossRef CAS;
(d) W. Zhang, X. Deng, F.-X. Zhang, J.-H. Lin, J.-C. Xiao and S. H. Liang, Synthesis and 18F Labeling of Alkenyl Sulfonyl Fluorides via an Unconventional Elimination Pathway, Org. Lett., 2022, 24, 4992–4997 CrossRef CAS.
- For selected reviews, see:
(a) A. S. Barrow, C. J. Smedley, Q. Zheng, S. Li, J. Dong and J. E. Moses, The Growing Applications of SuFEx Click Chemistry, Chem. Soc. Rev., 2019, 48, 4731–4758 RSC;
(b) S. N. Carneiro, S. R. Khasnavis, J. Lee, T. W. Butler, J. D. Majmudar, C. W. am Ende and N. D. Ball, Sulfur(VI) fluorides as tools in biomolecular and medicinal chemistry, Org. Biomol. Chem., 2023, 21, 1356–1372 RSC.
-
(a) A. Narayanan and L. H. Jones, Sulfonyl Fluorides as Privileged Warheads in Chemical Biology, Chem. Sci., 2015, 6, 2650–2659 RSC;
(b) O. O. Fadeyi, L. R. Hoth, C. Choi, X. Feng, A. Gopalsamy, E. C. Hett, R. E. Kyne, R. P. Robinson and L. H. Jones, Covalent Enzyme Inhibition through Fluorosulfate Modification of a Noncatalytic Serine Residue, ACS Chem. Biol., 2017, 12, 2015–2020 CrossRef CAS;
(c) L. H. Jones, Emerging Utility of Fluorosulfate Chemical Probes, ACS Med. Chem. Lett., 2018, 9, 584–586 CrossRef CAS;
(d) P. Martin-Gago and C. A. Olsen, Arylfluorosulfate-Based Electrophiles for Covalent Protein Labeling: A New Addition to the Arsenal, Angew. Chem., Int. Ed., 2019, 58, 957–966 CrossRef CAS;
(e) Q. Zheng, J. L. Woehl, S. Kitamura, D. Santos-Martins, C. J. Smedley, G. Li, S. Forli, J. E. Moses, D. W. Wolan and K. B, Sharpless, SuFEx-enabled, agnostic discovery of covalentinhibitors of human neutrophil elastase, Proc. Natl. Acad. Sci. U. S. A., 2019, 116, 18808–18814 CrossRef CAS;
(f) L. H. Jones and J. W. Kelly, Structure-based design and analysis of SuFEx chemical probes, RSC Med. Chem., 2020, 11, 10–17 RSC;
(g) A. Aatkar, A. Vuorinen, O. E. Longfield, K. Gilbert, R. Peltier-Heap, C. D. Wagner, F. Zappacosta, K. Rittinger, C.-w. Chung, D. House, N. C. O. Tomkinson and J. T. Bush, Efficient Ligand Discovery Using Sulfur(VI) Fluoride Reactive Fragments, ACS Chem. Biol., 2023, 18, 1926–1937 CrossRef CAS;
(h) S. Li, N. Wang, B. Yu, W. Su and L. Wang, Genetically encoded chemical crosslinking of carbohydrate, Nat. Chem., 2023, 15, 33–42 CrossRef CAS;
(i) Y. Chen, G. B. Craven, R. A. Kamber, A. Cuesta, S. Zhersh, Y. S. Moroz, M. C. Bassik and J. Taunton, Direct mapping of ligandable tyrosines and lysines in cells with chiral sulfonyl fluoride probes, Nat. Chem., 2023, 15, 1616–1625 CrossRef CAS.
-
(a) D. E. Mortenson, G. J. Brighty, L. Plate, G. Bare, W. Chen, S. Li, H. Wang, B. F. Cravatt, S. Forli, E. T. Powers, K. B. Sharpless, I. A. Wilson and J. W. Kelly, “Inverse Drug Discovery” strategy to identify proteins that are targeted by latent electrophiles as exemplified by aryl fluorosulfates, J. Am. Chem. Soc., 2018, 140, 200–210 CrossRef CAS;
(b) Z. Liu, J. Li, S. Li, G. Li, K. B. Sharpless and P. Wu, SuFEx click chemistry enabled late-stage drug functionalization, J. Am. Chem. Soc., 2018, 140, 2919–2925 CrossRef CAS;
(c) G. J. Brighty, R. C. Botham, S. Li, L. Nelson, D. E. Mortenson, G. Li, C. Morisseau, H. Wang, K. B. Sharpless and J. K. Kelly, Using sulfuramidimidoyl fluorides that undergo sulfur(VI) fluoride exchange for inverse drug discovery, Nat. Chem., 2020, 12, 906–913 CrossRef CAS;
(d) S. E. Dalton and S. Campos, Covalent Small Molecules as Enabling Platforms for Drug Discovery, ChemBioChem, 2020, 21, 1080–1100 CrossRef CAS.
-
(a) B. Gao, L. Zhang, Q. Zheng, F. Zhou, L. M. Klivansky, J. Lu, Y. Liu, J. Dong, P. Wu and K. B. Sharpless, Bifluoride-Catalysed Sulfur(VI) Fluoride Exchange Reaction for the Synthesis of Polysulfates and Polysulfonates, Nat. Chem., 2017, 9, 1083–1088 CrossRef CAS;
(b) C. Yang, J. P. Flynn and J. Niu, Facile Synthesis of Sequence Regulated Synthetic Polymers Using Orthogonal SuFEx and CuAAC Click Reactions, Angew. Chem., Int. Ed., 2018, 57, 16194–16199 CrossRef CAS;
(c) K. Durie, J. Yatvin, M. Kovaliov, G. H. Crane, J. Horn, S. Averick and J. Locklin, SuFEx Postpolymerization Modification Kinetics and Reactivity in Polymer Brushes, Macromolecules, 2018, 51, 297–305 CrossRef CAS;
(d) R. W. Kulow, J. W. Wu, C. Kim and Q. Michaudel, Synthesis of unsymmetrical sulfamides and polysulfamides via SuFEx click chemistry, Chem. Sci., 2020, 11, 7807–7812 RSC;
(e) H. Wan, S. Zhou, P. Gu, F. Zhou, D. Lyu, Q. Xu, A. Wang, H. Shi, Q. Xu and J. Lu, Polym. Chem., 2020, 11, 1033–1042 RSC;
(f) S. Li, G. Li, B. Gao, S. P. Pujari, X. Chen, H. Kim, F. Zhou, L. M. Klivansky, Y. Liu, H. Driss, D.-D. Liang, J. Lu, P. Wu, H. Zuilhof, J. Moses and K. B. Sharpless, SuFExable polymers with helical structures derived from thionyl tetrafluoride, Nat. Chem., 2021, 13, 858–867 CrossRef CAS;
(g) D.-D. Liang, S. P. Pujari, M. Subramaniam, M. Besten and H. Zuilhof, Configurationally Chiral SuFEx-Based Polymers, Angew. Chem., Int. Ed., 2022, 61, e202116158 CrossRef CAS.
-
(a) W. Davies and J. H. Dick, CCLXXXVI.—Aromatic sulphonyl fluorides. A convenient method of preparation, J. Chem. Soc., 1931, 2104–2109 RSC;
(b) T. A. Bianchi and L. A. Cate, Phase transfer catalysis. Preparation of aliphatic and aromatic sulfonyl fluorides, J. Org. Chem., 1977, 42, 2031–2032 CrossRef CAS.
-
(a) Y. Jiang, N. S. Alharbi, B. Sun and H.-L. Qin, Facile one-pot synthesis of sulfonyl fluorides from sulfonates or sulfonic acids, RSC Adv., 2019, 9, 13863–13867 RSC;
(b) B. J. Thomson, S. R. Khasnavis, E. C. Grigorian, R. Krishnan, T. D. Yassa, K. Lee, G. M. Sammis and N. D. Ball, Facile synthesis of sulfonyl fluorides from sulfonic acids, Chem. Commun., 2023, 59, 555–558 RSC;
(c) M. Pérez-Palau and J. Cornella, Synthesis of Sulfonyl Fluorides from Sulfonamides, Eur. J. Org. Chem., 2020, 2497–2500 CrossRef;
(d) A. T. Davies, J. M. Curto, S. W. Bagley and M. C. Willis, One-Pot Palladium-Catalyzed Synthesis of Sulfonyl Fluorides from Aryl Bromides, Chem. Sci., 2017, 8, 1233–1237 RSC.
-
(a) C. K. Prier, D. A. Rankic and D. W. C. MacMillan, Visible Light Photoredox Catalysis with Transition Metal Complexes: Applications in Organic Synthesis, Chem. Rev., 2013, 113, 5322–5363 CrossRef CAS;
(b) D. Staveness, I. Bosque and C. R. J. Stephenson, Free Radical Chemistry Enabled by Visible Light-Induced Electron Transfer, Acc. Chem. Res., 2016, 49, 2295–2306 CrossRef CAS;
(c) I. Ghosh, L. Marzo, A. Das, R. Shaikh and B. König, Visible Light Mediated Photoredox Catalytic Arylation Reactions, Acc. Chem. Res., 2016, 49, 1566–1577 CrossRef CAS;
(d) M. N. Hopkinson, A. Tlahuext-Aca and F. Glorius, Merging Visible Light Photoredox and Gold Catalysis, Acc. Chem. Res., 2016, 49, 2261–2272 CrossRef CAS;
(e) M. H. Shaw, J. Twilton and D. W. C. MacMillan, Photoredox Catalysis in Organic Chemistry, J. Org. Chem., 2016, 81, 6898–6926 CrossRef CAS;
(f) X. Lang, J. Zhao and X. Chen, Cooperative Photoredox Catalysis, Chem. Soc. Rev., 2016, 45, 3026–3038 RSC;
(g) N. L. Reed and T. P. Yoon, Oxidase Reactions in Photoredox Catalysis, Chem. Soc. Rev., 2021, 50, 2954–2967 RSC;
(h) M. S. Galliher, B. J. Roldan and C. R. J. Stephenson, Evolution towards Green Radical Generation in Total Synthesis, Chem. Soc. Rev., 2021, 50, 10044–10057 RSC.
-
(a) R. Francke and R. D. Little, Redox Catalysis in Organic Electrosynthesis: Basic Principles and Recent Developments, Chem. Soc. Rev., 2014, 43, 2492–2521 RSC;
(b) Y. Jiang, K. Xu and C. Zeng, Use of Electrochemistry in the Synthesis of Heterocyclic Structures, Chem. Rev., 2018, 118, 4485–4540 CrossRef CAS;
(c) K.-J. Jiao, Y.-K. Xing, Q.-L. Yang, H. Qiu and T.-S. Mei, Site-Selective C–H Functionalization Via Synergistic Use of Electrochemistry and Transition Metal Catalysis, Acc. Chem. Res., 2020, 53, 300–310 CrossRef CAS;
(d) J. Liu, L. Lu, D. Wood and S. Lin, New Redox Strategies in Organic Synthesis by Means of Electrochemistry and Photochemistry, ACS Cent. Sci., 2020, 6, 1317–1340 CrossRef CAS;
(e) Y. Yuan, J. Yang and A. Lei, Recent Advances in Electrochemical Oxidative Cross-Coupling with Hydrogen Evolution Involving Radicals, Chem. Soc. Rev., 2021, 50, 10058–10086 RSC;
(f) C. Ma, P. Fang, Z.-R. Liu, S.-S. Xu, K. Xu, X. Cheng, A. Lei, H.-C. Xu, C. Zeng and T.-S. Mei, Recent Advances in Organic Electrosynthesis Employing Transition Metal Complexes as Electrocatalysts, Sci. Bull., 2021, 66, 2412–2429 CrossRef CAS;
(g) L. F. T. Novaes, J. Liu, Y. Shen, L. Lu, J. M. Meinhardt and S. Lin, Electrocatalysis as an enabling technology for organic synthesis, Chem. Soc. Rev., 2021, 50, 7941–8002 RSC.
-
(a) T. Zhong, Z. Chen, J. Yi, G. Lu and J. Weng, Recent progress in the synthesis of sulfonyl fluorides for SuFEx click chemistry, Chin. Chem. Lett., 2021, 32, 2736–2750 CrossRef CAS;
(b) C. Lee, A. J. Cook, J. E. Elisabeth, N. C. Friede, G. M. Sammis and N. D. Ball, The Emerging Applications of Sulfur(VI) Fluorides in Catalysis, ACS Catal., 2021, 11, 6578–6589 CrossRef CAS;
(c) A. Chelagha, D. Louvel, A. Taponard, R. Berthelon and A. Tlili, Synthetic, Routes to Arylsulfonyl Fluorides, Catalysts, 2021, 11, 830 CrossRef CAS;
(d) F.-S. He, Y. Li and J. Wu, Fluorosulfonyl radicals: new horizons for the synthesis of sulfonyl fluorides, Org. Chem. Front., 2022, 9, 5299–5305 RSC;
(e) T. S.-B. Lou and M. C. Willis, Sulfonyl fluorides as targets and substrates in the development of new synthetic methods, Nat. Rev. Chem., 2022, 6, 146–162 CrossRef CAS;
(f) S. Barata-Vallejo, D. E. Yerien and A. Postigo, Synthetic strategies for fluorosulfonylated compounds: application to click chemistry reactions, Catal. Sci. Technol., 2023, 13, 2597–2617 RSC;
(g) D. Zeng, W.-P. Deng and X. Jiang, Advances in the construction of diverse SuFEx linkers, Natl. Sci. Rev., 2023, 10 DOI:10.1093/nsr/nwad123.
-
(a) J. J. Krutak, R. D. Burpitt, W. H. Moore and J. A. Hyatt, Chemistry of ethenesulfonyl fluoride. Fluorosulfonylethylation of organic compounds, J. Org. Chem., 1979, 44, 3847–3858 CrossRef CAS;
(b) Q. Chen, P. Mayer and H. Mayr, Ethenesulfonyl Fluoride: The Most Perfect Michael Acceptor Ever Found?, Angew. Chem., Int. Ed., 2016, 55, 12664–12667 CrossRef CAS;
(c) Y.-P. Meng, S.-M. Wang, W.-Y. Fang, Z.-Z. Xie, J. Leng, H. Alsulami and H.-L. Qin, Ethenesulfonyl Fluoride (ESF) and Its Derivatives in SuFEx Click Chemistry and More, Synthesis, 2020, 52, 673–687 CrossRef CAS;
(d) H.-L. Qin, Q. Zheng, G. A. L. Bare, P. Wu and K. B. Sharpless, A Heck–Matsuda Process for the Synthesis of β-Arylethenesulfonyl Fluorides: Selectively Addressable Bis-electrophiles for SuFEx Click Chemistry, Angew. Chem., Int. Ed., 2016, 55, 14155–14158 CrossRef CAS;
(e) P. K. Chinthakindi, K. B. Govender, A. S. Kumar, H. G. Kruger, T. Govender, T. Naicker and P. I. Arvidsson, A Synthesis of “Dual Warhead” β-Aryl Ethenesulfonyl Fluorides and One-Pot Reaction to β-Sultams, Org. Lett., 2017, 19, 480–483 CrossRef CAS;
(f) G.-F. Zha, Q. Zheng, J. Leng, P. Wu, H.-L. Qin and K. B. Sharpless, Palladium-Catalyzed Fluorosulfonylvinylation of Organic Iodides, Angew. Chem., Int. Ed., 2017, 56, 4849–4852 CrossRef CAS;
(g) S.-M. Wang, C. Li, J. Leng, S. N. A. Bukhari and H.-L. Qin, Rhodium(III)-catalyzed Oxidative Coupling of N-Methoxybenzamides and Ethenesulfonyl fluoride: a C–H Bond Activation Strategy for the Preparation of 2-Aryl ethenesulfonyl fluorides and Sulfonyl fluoride Substituted γ-Lactams, Org. Chem. Front., 2018, 5, 1411–1415 RSC;
(h) H. Jangra, Q. Chen, E. Fuks, I. Zenz, P. Mayer, A. R. Ofial, H. Zipse and H. Mayr, Nucleophilicity and Electrophilicity Parameters for Predicting Absolute Rate Constants of Highly Asynchronous 1,3-Dipolar Cycloadditions of Aryldiazomethanes, J. Am. Chem. Soc., 2018, 140, 16758–16772 CrossRef CAS.
- Y. A. Skalenko, T. V. Druzhenko, A. V. Denisenko, M. V. Samoilenko, O. P. Dacenko, S. A. Trofymchuk, O. O. Grygorenko, A. A. Tolmachev and P. K. Mykhailiuk, [2 + 2]-Photocycloaddition of N-Benzylmaleimide to Alkenes As an Approach to Functional 3-Azabicyclo[3.2.0]heptanes, J. Org. Chem., 2018, 83, 6275–6289 CrossRef CAS.
- J. Liu, S.-M. Wang and H.-L. Qin, Light-induced [2 + 2] cycloadditions for the construction of cyclobutane-fused pyridinyl sulfonyl fluorides, Org. Biomol. Chem., 2020, 18, 4019–4023 RSC.
- R. Xu, T. Xu, M. Yang, T. Cao and S. Liao, A rapid access to aliphatic sulfonyl fluorides, Nat. Commun., 2019, 10, 3752 CrossRef.
- X. Zhang, W.-Y. Fang, R. Lekkala, W. Tang and H.-L. Qin, An Easy, General and Practical Method for the Construction of Alkyl Sulfonyl Fluorides, Adv. Synth. Catal., 2020, 362, 3358–3363 CrossRef CAS.
- J. Cui, S. Ke, J. Zhao, S. Wu, W. Luo, S. Xu, X. Su and Y. Li, Photocatalytic access to aromatic keto sulfonyl fluorides from vinyl fluorosulfates, Org. Chem. Front., 2022, 9, 3540–3545 RSC.
- J. E. Erchinger, R. Hoogesteger, R. Laskar, S. Dutta, C. Hümpel, D. Rana, C. G. Daniliuc and F. Glorius, EnT-Mediated N−S Bond Homolysis of a Bifunctional Reagent Leading to Aliphatic Sulfonyl Fluorides, J. Am. Chem. Soc., 2023, 145, 2364–2374 CrossRef CAS.
-
(a) T. S. Lou, S. W. Bagley and M. C. Willis, Cyclic Alkenylsulfonyl Fluorides: Palladium-Catalyzed Synthesis and Functionalization of Compact Multifunctional Reagents, Angew. Chem., Int. Ed., 2019, 58, 18859–18863 CrossRef CAS;
(b) M. Magre and J. Cornella, Redox-Neutral Organometallic Elementary Steps at Bismuth: Catalytic Synthesis of Aryl Sulfonyl Fluorides, J. Am. Chem. Soc., 2021, 143, 21497–21502 CrossRef CAS.
-
(a) Y. Liu, H. Wu, Y. Guo, J.-C. Xiao, Q.-Y. Chen and C. Liu, Trifluoromethylfluorosulfonylation of Unactivated Alkenes Using Readily Available Ag(O2CCF2SO2F) and N-Fluorobenzenesulfonimide, Angew. Chem., Int. Ed., 2017, 56, 15432–15435 CrossRef CAS;
(b) Q. Lin, Y. Liu, Z. Xiao, L. Zheng, X. Zhou, Y. Guo, Q.-Y. Chen, C. Zheng and C. Liu, Intermolecular Oxidative Radical Fluoroalkylfluorosulfonylation of Unactivated Alkenes with (Fluoroalkyl)trimethylsilane, Silver Fluoride, Sulfur Dioxide and N-fluorobenzenesulfonimide, Org. Chem. Front., 2019, 6, 447–450 RSC;
(c) Y. Liu, D. Yu, Y. Guo, J.-C. Xiao, Q.-Y. Chen and C. Liu, Arenesulfonyl Fluoride Synthesis via Copper Catalyzed Fluorosulfonylation of Arenediazonium Salts, Org. Lett., 2020, 22, 2281–2286 CrossRef CAS;
(d) T. Zhong, M.-K. Pang, Z.-D. Chen, B. Zhang, J. Weng and G. Lu, Copper-Free Sandmeyer-Type Reaction for the Synthesis of Sulfonyl Fluorides, Org. Lett., 2020, 22, 3072–3078 CrossRef CAS;
(e) S. Liu, Y. Huang, X.-H. Xu and F.-L. Qing, Fluorosulfonylation of Arenediazonium Tetrafluoroborates with Na2S2O5 and N-fluorobenzenesulfonimide, J. Fluorine Chem., 2020, 240, 109653 CrossRef CAS;
(f) Z. Ma, Y. Liu, X. Ma, X. Hu, Y. Guo, Q.-Y. Chen and C. Liu, Aliphatic sulfonyl fluoride synthesis via reductive decarboxylative fluorosulfonylation of aliphatic carboxylic acid NHPI esters, Org. Chem. Front., 2022, 9, 1115–1120 RSC.
- Z.-D. Chen, X. Zhou, J.-T. Yi, H.-J. Diao, Q.-L. Chen, G. Lu and J. Weng, Catalytic Decarboxylative Fluorosulfonylation Enabled by Energy Transfer-Mediated Photocatalysis, Org. Lett., 2022, 24, 2474–2478 CrossRef CAS.
- H. Zhang, S. Li, H.-L. Zheng, G. Zhu, S. Liao and X. Nie, Photocatalytic fluorosulfonylation of aliphatic carboxylic acid NHPI esters, Org. Chem. Front., 2022, 9, 4854–4860 RSC.
- T. Xu, T. Cao, M. Yang, R. Xu, X. Nie and S. Liao, Decarboxylative Thiolation of Redox-Active Esters to Thioesters by Merging Photoredox and Copper Catalysis, Org. Lett., 2020, 22, 3692–3696 CrossRef CAS.
- V. T. Nguyen, G. C. Haug, V. D. Nguyen, N. T. H. Vuong, G. B. Karki, H. D. Arman and O. V. Larionov, Functional Group Divergence and the Structural Basis of Acridine Photocatalysis Revealed by Direct Decarboxysulfonylation, Chem. Sci., 2022, 13, 4170–4179 RSC.
- J.-T. Yi, X. Zhou, Q.-L. Chen, Z.-D. Chen, G. Lu and J. Weng, Copper-Catalyzed Direct Decarboxylative Fluorosulfonylation of Aliphatic Carboxylic Acids, Chem. Commun., 2022, 58, 9409–9412 RSC.
- C. Ou, Y. Cai, Y. Ma, H. Zhang, X. Ma and C. Liu, Aliphatic Sulfonyl Fluoride Synthesis via Decarboxylative Fluorosulfonylation of Hypervalent Iodine(III) Carboxylates, Org. Lett., 2023, 25, 6751–6756 CrossRef CAS.
- C. A. Vincent, M. I. Chiriac, L. Troian-Gautier and U. K. Tambar, Photocatalytic Sulfonyl Fluorination of Alkyl Organoboron Substrates, ACS Catal., 2023, 13, 3668–3675 CrossRef CAS.
-
(a) A. J. Brouwer, T. Ceylan, A. M. Jonker, T. van der Linden and R. M. J. Liskamp, Synthesis and biological evaluation of novel irreversible serine protease inhibitors using amino acid based sulfonyl fluorides as an electrophilic trap, Bioorg. Med. Chem., 2011, 19, 2397–2406 CrossRef CAS;
(b) S. Guardiola, R. Prades, L. Mendieta, A. J. Brouwer, J. Streefkerk, L. Nevola, T. Tarragó, R. M. J. Liskamp and E. Giralt, Targeted Covalent Inhibition of Prolyl Oligopeptidase (POP): Discovery of Sulfonylfluoride Peptidomimetics, Cell Chem. Biol., 2018, 25, 1031–1037 CrossRef CAS;
(c) R. Artschwager, D. J. Ward, S. Gannon, A. J. Brouwer, H. van de Langemheen, H. Kowalski and R. M. J. Liskamp, Potent and Highly Selective Inhibitors of the Proteasome Trypsin-like Site by Incorporation of Basic Side Chain Containing Amino Acid Derived Sulfonyl Fluorides, J. Med. Chem., 2018, 61, 5395–5411 CrossRef CAS.
- T. Zhong, J.-T. Yi, Z.-D. Chen, Q.-C. Zhuang, Y.-Z. Li, G. Lu and J. Weng, Photoredox-catalyzed aminofluorosulfonylation of unactivated olefins, Chem. Sci., 2021, 12, 9359–9365 RSC.
- J. A. Andrews, L. R. E. Pantaine, C. F. Palmer, D. L. Poole and M. C. Willis, Sulfinates from Amines: A Radical Approach to Alkyl Sulfonyl Derivatives via Donor−Acceptor Activation of Pyridinium Salts, Org. Lett., 2021, 23, 8488–8493 CrossRef CAS.
- D. Louvel, A. Chelagha, J. Rouillon, P.-A. Payard, L. Khrouz, C. Monnereau and A. Tlili, Metal-Free Visible-Light Synthesis of Arylsulfonyl Fluorides: Scope and Mechanism, Chem. – Eur. J., 2021, 27, 8704–8708 CrossRef CAS.
- C. A. Vincent, A. Ripak, L. Troian-Gautier and U. K. Tambar, Photocatalytic conversion of aryl diazonium salts to sulfonyl fluorides, Tetrahedron, 2023, 139, 133364 CrossRef CAS.
- T. T. Bui, V. H. Tran and H.-K. Kim, Visible-Light-Mediated Synthesis of Sulfonyl Fluorides from Arylazo Sulfones, Adv. Synth. Catal., 2022, 364, 341–347 CrossRef CAS.
- L. Shan, Z. Ma, C. Ou, Y. Cai, Y. Ma, Y. Guo, X. Ma and C. Liu, Sulfonyl fluoride synthesis via visible-light-mediated fluorosulfonylation of thianthrenium salts, Synth. Commun., 2023, 53, 1784–1798 CrossRef CAS.
- Z.-M. Yan, L. Qi, H.-J. Du, Z.-Q. Zhao, J.-L. Liu, Y.-C. Dong, W. Li and L.-J. Wang, Photocatalytic C−C Bond Cleavage and Fluorosulfonylation of Strained Cycloalkanols for Carbonyl-Containing Aliphatic Sulfonyl Fluorides, Org. Lett., 2023, 25, 7051–7056 CrossRef CAS.
- K. L. Wray and E. V. Feldman, Shock tube study of the decomposition kinetics of SO2F2, J. Chem. Phys., 1971, 54, 3445–3449 CrossRef CAS.
- G. A. Takacs, Heats of formation and bond dissociation energies of some simple sulfur- and halogen-containing molecules, J. Chem. Eng. Data, 1978, 23, 174–175 CrossRef CAS.
- T. Guo, G. Meng, X. Zhan, Q. Yang, T. Ma, L. Xu, K. B. Sharpless and J. Dong, A New Portal to SuFEx Click Chemistry: A Stable Fluorosulfuryl Imidazolium Salt Emerging as an “F−SO2+” Donor of Unprecedented Reactivity, Selectivity, and Scope, Angew. Chem., Int. Ed., 2018, 57, 2605–2610 CrossRef CAS.
- H. Zhou, P. Mukherjee, R. Liu, E. Evrard, D. Wang, J. M. Humphrey, T. W. Butler, L. R. Hoth, J. B. Sperry, S. K. Sakata, C. J. Helal and C. W. am Ende, Introduction of a Crystalline, Shelf-Stable Reagent for the Synthesis of Sulfur(VI) Fluorides, Org. Lett., 2018, 20, 812–815 CrossRef CAS.
-
(a) C. Lee, N. D. Ball and G. M. Sammis, One-pot fluorosulfurylation of Grignard reagents using sulfuryl fluoride, Chem. Commun., 2019, 55, 14753–14756 RSC;
(b) J. W. Wu, R. W. Kulow, M. J. Redding, A. J. Fine, S. M. Grayson and Q. Michaudel, Synthesis of Degradable Polysulfamides via Sulfur(VI) Fluoride Exchange Click Polymerization of AB-Type Monomers, ACS Polym. Au, 2023, 3, 259–266 CrossRef CAS.
-
(a) X. Nie, T. Xu, J. Song, A. Devaraj, B. Zhang, Y. Chen and S. Liao, Radical Fluorosulfonylation: Accessing Alkenyl Sulfonyl Fluorides from Alkenes, Angew. Chem., Int. Ed., 2021, 60, 3956–3960 CrossRef CAS;
(b) X. Nie and S. Liao, Radical Fluorosulfonylation: Accessing Alkenylsulfonyl Fluorides from Alkenes and Alkynes, Synlett, 2022, 401–408 CAS.
- X. Nie, T. Xu, Y. Hong, H. Zhang, C. Mao and S. Liao, Introducing A New Class of Sulfonyl Fluoride Hubs via Radical Chloro-Fluorosulfonylation of Alkynes, Angew. Chem., Int. Ed., 2021, 60, 22035–22042 CrossRef CAS.
- X. Zhao, D. Chen, S. Zhu, J. Luo, S. Liao, B. Zheng and S. Huang, Fluorosulfonylvinylation of Unactivated C(sp3)−H via Electron Donor−Acceptor Photoactivation, Org. Lett., 2023, 25, 3109–3113 CrossRef CAS.
- S. Shi, X. Zhao, D. Chen, J. Luo, S. Liao and S. Huang, C(sp3)–H fluorosulfonylvinylation/aza-Michael addition approach to FSO2-functionalized tetrahydropyridines, Org. Chem. Front., 2023, 10, 3805–3810 RSC.
-
(a) P. Wang, H. Zhang, X. Nie, T. Xu and S. Liao, Photoredox catalytic radical fluorosulfonylation of olefins enabled by a bench-stable redox-active fluorosulfonyl radical precursor, Nat. Commun., 2022, 13, 3370 CrossRef CAS;
(b) J. Li and J. Xiao, Photoredox Catalytic Radical Fluorosulfonylation of Olefins, Chin. J. Org. Chem., 2022, 42, 2593–2595 CrossRef.
- W. Zhang, Z. Zou, W. Zhao, S. Lu, Z. Wu, M. Huang, X. Wang, Y. Wang, Y. Liang, Y. Zhu, Y. Zheng and Y. Pan, Integrated redox-active reagents for photoinduced regio- and stereoselective fluorocarboborylation, Nat. Commun., 2020, 11, 2572 CrossRef CAS.
- W. Zhang, H. Li, X. Li, Z. Zou, M. Huang, J. Liu, X. Wang, S. Ni, Y. Pan and Y. Wang, A practical fluorosulfonylating platform via photocatalytic imidazolium-based SO2F radical reagent, Nat. Commun., 2022, 13, 3515 CrossRef CAS.
- P. Wang, H. Zhang, M. Zhao, S. Ji, L. Lin, N. Yang, X. Nie, J. Song and S. Liao, Radical Hydro-Fluorosulfonylation of Unactivated Alkenes and Alkynes, Angew. Chem., Int. Ed., 2022, 61, e202207684 CrossRef CAS.
- H. Zhang, N. Yang, J. Li, P. Wang, S. Li, L. Xie and S. Liao, Radical Fluorosulfonyl Arylation of Alkenes: Accessing FSO2-Functionalized Chromanes via Formal Endo and Exo Cyclization, Org. Lett., 2022, 24, 8170–8175 CrossRef CAS.
- P. Wang, S.-J. Li, H. Zhang, N. Yang and S. Liao, Photo-organocatalytic Synthesis of β-Keto Sulfonyl Fluorides via Radical Fluorosulfonylation of Vinyl Acetates, Synlett, 2023, 34, 471–476 CrossRef CAS.
- L. Lin, P. Wang, T. Dong, G. C. Tsui and S. Liao, Radical Fluorosulfonyl Heteroarylation of Unactivated Alkenes with Quinoxalin-2(1H)-ones and Related N-Heterocycles, Org. Lett., 2023, 25, 1088–1093 CrossRef CAS.
- N. Yang, C. Mao, H. Zhang, P. Wang, S. Li, L. Xie and S. Liao, FSO2 Radical-Initiated Tandem Addition Reaction of Two Different Olefins: A Facile Access to Multifunctional Aliphatic Sulfonyl Fluorides, Org. Lett., 2023, 25, 4478–4482 CrossRef CAS.
- M. Kirihara, S. Naito, Y. Ishizuka, H. Hanai and T. Noguchi, Oxidation of disulfides with Selectfluor™: concise syntheses of thiosulfonates and sulfonyl fluorides, Tetrahedron Lett., 2011, 52, 3086–3089 CrossRef CAS.
- G. Laudadio, A. de A. Bartolomeu, L. M. H. M. Verwijlen, Y. Cao, K. T. de Oliveira and T. Noël, Sulfonyl Fluoride Synthesis through Electrochemical Oxidative Coupling of
Thiols and Potassium Fluoride, J. Am. Chem. Soc., 2019, 141, 11832–11836 CrossRef CAS.
- Y. Cao, B. Adriaenssens, A. de A. Bartolomeu, G. Laudadio, K. T. de Oliveira and T. Noël, Accelerating sulfonyl fluoride synthesis through electrochemical oxidative coupling of thiols and potassium fluoride in flow, J. Flow Chem., 2020, 10, 191–197 CrossRef CAS.
- D. Chen, X. Nie, Q. Feng, Y. Zhang, Y. Wang, Q. Wang, L. Huang, S. Huang and S. Liao, Electrochemical OxoFluorosulfonylation of Alkynes under Air: Facile Access to β-Keto Sulfonyl Fluorides, Angew. Chem., Int. Ed., 2021, 60, 27271–27276 CrossRef CAS.
- Q. Feng, Y. Fu, Y. Zheng, S. Liao and S. Huang, Electrochemical Synthesis of β-Keto Sulfonyl Fluorides via Radical Fluorosulfonylation of Vinyl Triflates, Org. Lett., 2022, 24, 3702–3706 CrossRef CAS.
- L. Zhang, X. Cheng and Qi.-L. Zhou, Electrochemical Synthesis of Sulfonyl Fluorides with Triethylamine Hydrofluoride, Chin. J. Chem., 2022, 40, 1687–1692 CrossRef CAS.
- J. K. Park, J. Oh and S. Lee, Electrochemical synthesis of sulfonyl fluorides from sulfonyl hydrazides, Org. Chem. Front., 2022, 9, 3407–3413 RSC.
- L. Tang, Y. Yang, L. Wen, X. Yang and Z. Wang, Catalyst-free radical fluorination of sulfonyl hydrazides in water, Green Chem., 2016, 18, 1224–1228 RSC.
- T. S.-B. Lou, Y. Kawamata, T. Ewing, G. A. Correa-Otero, M. R. Collins and P. S. Baran, Scalable, Chemoselective Nickel Electrocatalytic Sulfinylation of Aryl Halides with SO2, Angew. Chem., Int. Ed., 2022, 61, e202208080 CrossRef CAS.
- X. Kong, Y. Chen, Q. Liu, W. Wang, S. Zhang, Q. Zhang, X. Chen, Y.-Q. Xu and Z.-Y. Cao, Selective Fluorosulfonylation of Thianthrenium Salts Enabled by Electrochemistry, Org. Lett., 2023, 25, 581–586 CrossRef CAS.
-
(a) L. Capaldo, L. L. Quadri and D. Ravelli, Merging Photocatalysis with Electrochemistry: The Dawn of a new Alliance in Organic Synthesis, Angew. Chem., Int. Ed., 2019, 58, 17508–17510 CrossRef CAS;
(b) J. P. Barham and B. Konig, Synthetic Photoelectrochemistry, Angew. Chem., Int. Ed., 2020, 59, 11732–11747 CrossRef CAS;
(c) L. Buglioni, F. Raymenants, A. Slattery, S. D. A. Zondag and T. Noël, Technological Innovations in Photochemistry for Organic Synthesis: Flow Chemistry, High-Throughput Experimentation, Scale-up, and Photoelectrochemistry, Chem. Rev., 2022, 122, 2752–2906 CrossRef CAS PubMed;
(d) H. Huang, K. A. Steiniger and T. H. Lambert, Electrophotocatalysis: Combining Light and Electricity to Catalyze Reactions, J. Am. Chem. Soc., 2022, 144, 12567–12583 CrossRef CAS;
(e) S. Wu, J. Kaur, T. A. Karl, X. Tian and J. P. Barham, Synthetic Molecular Photoelectrochemistry: New Frontiers in Synthetic Applications, Mechanistic Insights and Scalability, Angew. Chem., Int. Ed., 2022, 61, e202107811 CrossRef CAS.
- P. J. Sarver, N. B. Bissonnette and D. W. C. MacMillan, Decatungstate-Catalyzed C(sp3)−H Sulfinylation: Rapid Access to Diverse Organosulfur Functionality, J. Am. Chem. Soc., 2021, 143, 9737–9743 CrossRef CAS.
-
(a) B. Moku, W.-Y. Fang, J. Leng, E. A. B. Kantchev and H.-L. Qin, Rh(I)−Diene-Catalyzed Addition of (Hetero)Aryl Functionality to 1,3-Dienylsulfonyl Fluorides Achieving Exclusive Regioselectivity and High Enantioselectivity: Generality and Mechanism, ACS Catal., 2019, 9, 10477–10488 CrossRef CAS;
(b) J. Chen, B.-Q. Huang, Z.-Q. Wang, X.-J. Zhang and M. Yan, Asymmetric Conjugate Addition of Ethylene Sulfonyl Fluorides to 3-Amido-2-oxindoles: Synthesis of Chiral Spirocyclic Oxindole Sultams, Org. Lett., 2019, 21, 9742–9746 CrossRef CAS;
(c) B. Moku, W.-Y. Fang, J. Leng, L. Li, G.-F. Zha, K. P. Rakesh and H.-L. Qin, Rh-Catalyzed Highly Enantioselective Synthesis of Aliphatic Sulfonyl Fluorides, iScience, 2019, 21, 695–705 CrossRef CAS;
(d) J. Chen, D.-Y. Zhu, X.-J. Zhang and M. Yan, Highly Enantioselective Addition of N-2,2,2-Trifluoroethylisatin Ketimines to Ethylene Sulfonyl Fluoride, J. Org. Chem., 2021, 86, 3041–3048 CrossRef CAS.
|
This journal is © the Partner Organisations 2024 |
Click here to see how this site uses Cookies. View our privacy policy here.