DOI:
10.1039/D0RA05251E
(Paper)
RSC Adv., 2020,
10, 29171-29174
Direct access to multi-functionalized benzenes via [4 + 2] annulation of α-cyano-β-methylenones and α,β-unsaturated aldehydes†
Received
15th June 2020
, Accepted 28th July 2020
First published on 6th August 2020
Abstract
An efficient [4 + 2] benzannulation of α-cyano-β-methylenones and α,β-unsaturated aldehydes was achieved under metal-free reaction conditions selectively delivering a wide range of polyfunctional benzenes in high yields respectively (up to 94% yield).
Multi-substituted benzenes are privileged structural units ubiquitous in pharmaceuticals,1 natural products2 and advanced functional materials.3 Various excellent methodologies have been investigated for the construction of functionalized aromatics including nucleophilic or electrophilic substitution,4 transition metal-catalyzed coupling reactions5 and directed metalation.6 However, the widespread application of these strategies established thus far suffer from the limitations of functional groups introduced on the pre-existing benzene and regioselectivity issues. Among various synthetic methods, tandem benzannulation reactions arguably represent an attractive alternative to classical methods for rapid construction of polysubstituted benzenes in an atom-economical fashion.7 This protocol featuring an efficient transformation of acyclic building blocks into structurally valuable benzene skeletons. In this context, α-cyano-β-methylenones has been employed as substrates to format six-membered ring in tandem cyclization reactions due to the activation of the pronucleophile methyl group. In 2015, Tong and co-workers developed a phosphine-catalyzed addition/cycloaddition domino reactions of β′-acetoxy allenoate with 2-acyl-3-methyl-acrylonitriles to give 2-oxabicyclo[3.3.1]nonanes (Scheme 1a).8 Soon after that, the construction of benzonitrile derivatives and 1,3,5-trisubstituted benzenes via N-heterocyclic carbene catalysis has been reported by the groups of Wang and Ye independently (Scheme 1b).9 Then the synthesis of 1,3,5-trisubstituted benzenes by 1,8-diazabicyclo[5.4.0]undec-7-ene (DBU)-mediated annulation of α-cyano-β-methylenones and α,β-unsaturated carboxylic acids was also developed by Ye and co-workers (Scheme 1c).10 Shi et al. reported a base-promoted tandem cyclization reaction of α-cyano-β-methylenones and α,β-unsaturated enones, which have electron-withdrawing group (EWG), accessing to a wide range of benzonitriles in a different C–C bond formation process (Scheme 1d).11 As part of our ongoing interest in harnessing enones for developing new methodologies for the construction of functionalized benzenes, we have recently demonstrated NHC-catalyzed convenient benzonitrile assembly in the presence of oxidant.9a While the same reaction of enals and α-cyano-β-methylenones was conducted in the basic condition without NHC, a novel polyfunctionalized benzene product was obtained (Scheme 1e). The result inspired us to extend the synthetic potential of benzannulation strategy to access diverse benzonitriles, particularly from simpler, abundantly available starting materials.
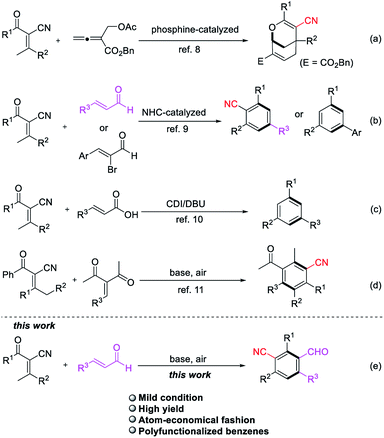 |
| Scheme 1 α-Cyano-β-methylenones in cycloaddition domino reactions. | |
At the outset, model reaction of 2-benzoyl-3-phenylbut-2-enenitrile 1a and cinnamaldehyde 2a was used to evaluate reaction parameters. Key results of condition optimization are summarized in Table 1. Several inorganic or organic bases were screened and NaOH turned out to be the most efficient for this transformation to give a novel product 3a. (33–78% yields, entries 1–9, Table 1). The structure of 3a was confirmed by X-ray crystallography as an unprecedented functionalized benzene.12 The configuration of products were assigned unambiguously by X-ray analysis of the product 3a. A quick solvent screening demonstrated that chloroform is the best choice to produce the benzannulation product 3a in a desirable yield (entries 10–13, Table 1. For additional details, see the ESI†). Reducing the loading of the cinnamaldehyde or NaOH to 1.2 equivalence led to dramatical loss of the yield (entries 14 &15, Table 1). And raising the reaction temperature to 50 °C resulted in lower yield (80% yield, entries 16, Table 1).
Table 1 Optimization of the reaction conditionsa
Finally, the standard reaction conditions for the base-promoted synthesis of the multi-functionalized benzene derivatives identified as follows: 1.5 equivalence of NaOH and CHCl3 as the solvent under an atmosphere of air for 12 hours at room temperature.
With the optimized reaction conditions in hand, we explored the scope of the reaction. A series of enones were examined, variation of the electronic nature of the aromatic ring (R1, including the substituted phenyl or thienyl) has little influence on the reaction efficiency (3b–f, 86–93% yields, Table 2). We subsequently examined the effect of R2 with different substitution patterns and electronic nature, β-arylenones with electron-rich and electron-deficient substituents were worked well to afford the functional benzonitriles in high yields (3g–r, 80–90% yields, Table 2). Enones with alkyl group on R2 position can also be used to afford their corresponding products (3s–v, 78–83% yields, Table 2) in good chemical yields.
Reaction conditions: 1a (0.1 mmol, 1.0 equiv.), 2a (0.15 mmol, 1.5 equiv.), NaOH (0.2 mmol, 2.0 equiv.), and CHCl3 (1 mL) for 12 h. |
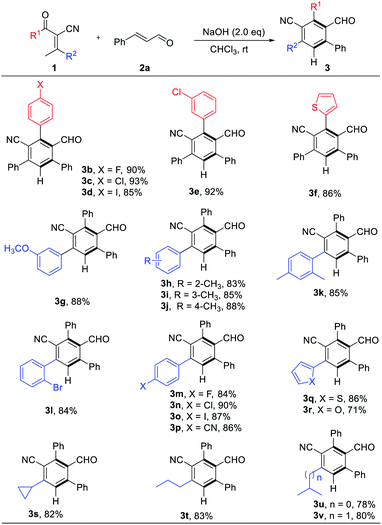 |
We next turned our attention to examine the scope of enals. Different substituents on the phenyl ring of cinnamaldehydes were tolerated even disregarding the position and properties, giving 4a–g in satisfying yields (82–92% yields, Table 3). With respect to heterocycles such as pyridine, furan, thiophene and naphthalenes were also compatible with the reaction conditions (4h–k, 82–88% yields, Table 3). Replacement of the β-phenyl substituent with an alkyl unit 4l & 4m had limited effect on reaction conversion, the corresponding products were obtained in good yields (82% & 80% yield, Table 3).
Reaction conditions: 1a (0.1 mmol, 1.0 equiv.), 2a (0.15 mmol, 1.5 equiv.), NaOH (0.2 mmol, 2.0 equiv.), and CHCl3 (1 mL) for 12 h. |
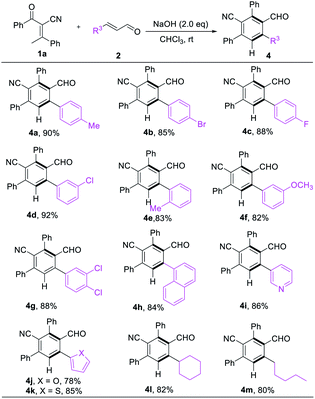 |
To highlight the practicality of this mild and efficient method, the reaction of 2-benzoyl-3-phenylbut-2-enenitrile 1a at 4.0 mmol scale proceed well under the standard conditions to generate the desired product in 88% yield (Scheme 2).
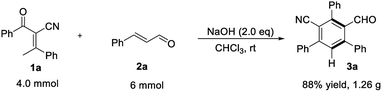 |
| Scheme 2 Gram-Scale Synthesis of 3a. | |
The formyl group could be easily reduced by using LiAlH4 in THF at reflux, leading to the formation of the benzyl alcohol product 5 in 95% yield while keeping the CN group intact. Suzuki coupling of 3o with phenylboronic acid furnished derivative 6 in 90% yield13 (Scheme 3).
 |
| Scheme 3 Synthetic transformation. | |
To gain insight into the role of air in this reaction, a control experiment was designed and investigated (Scheme 4). When the reaction of 1a and 2a was carried out under an argon atmosphere, the desired product 3a was obtained in 10% yield and product 7 could be isolated in 82% yield. The results indicate that oxygen is necessary for the oxidation process and played a key role in this reaction.
 |
| Scheme 4 Control experiment. | |
A postulated reaction course is illustrated in Scheme 5. Briefly, α-deprotonation of enone 1a in the presence of bases, subsequent 1,4-addition of deprotonated enone I to enal 2a generates intermediate II, which undergoes an intramolecular aldol reaction to yield the adduct 7.14 Lastly, dehydration of 7 followed by spontaneous oxidative aromatization affords the polysubstituted benzonitrile 3a.
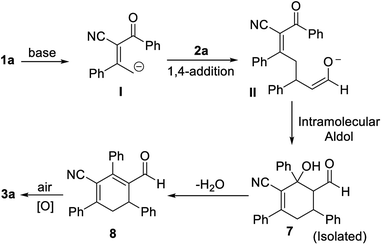 |
| Scheme 5 The proposed mechanism. | |
Conclusions
In summary, we have developed the example of catalyst-free [4 + 2] cycloaddition reaction of α-cyano-β-methylenones and α,β-unsaturated aldehydes. This protocol provides straightforward access to the corresponding highly functionalized benzenes in good to excellent yields under ambient conditions. Such studies are actively underway in our laboratory, and more results will be reported in due course.
Conflicts of interest
There are no conflicts to declare.
Acknowledgements
This work is financially supported by the National Natural Science Foundation of China (No. 21602179) and Science Foundation of Yangtze Normal University (No. 2017KYQD123, 2018QNRC17).
References
-
(a) K. L. Goa and A. J. Wagstaff, Drugs, 1996, 51, 820 CrossRef CAS;
(b) G. Ortar, Bioorg. Med. Chem. Lett., 2013, 23, 5614 CrossRef CAS;
(c) N. A. McGrath, M. Brichacek and J. T. Njardarson, J. Chem. Educ., 2010, 87, 1348 CrossRef CAS;
(d) M. Baumann, I. R. Baxendale, S. V. Ley and N. Nikbin, Beilstein J. Org. Chem., 2011, 7, 442 CrossRef CAS.
-
(a) A. Minatti and K. H. Dötz, J. Org. Chem., 2005, 70, 3045 CrossRef;
(b) X. Y. Mak, L. A. Crombie and R. L. Danheiser, J. Org. Chem., 2011, 76, 1852 CrossRef CAS;
(c) L. Fuhr, M. Rousseau, A. Plauth, F. C. Schroeder and S. Sauer, J. Nat. Prod., 2015, 78, 1160 CrossRef CAS;
(d) H. Li, Q. Chen, Z. Lu and A. Li, J. Am. Chem. Soc., 2016, 138, 15558 Search PubMed;
(e) P. Yang, M. Yao, J. Li, Y. Li and A. Li, Angew. Chem., Int. Ed., 2016, 55, 6964 CrossRef CAS;
(f) P. Finkbeiner, K. Murai, M. Röpke and R. Sarpong, J. Am. Chem. Soc., 2017, 139, 11349 CrossRef CAS;
(g) W. Chen, R. Guo, Z. Yang and J. Gong, J. Org. Chem., 2018, 83, 15524 CrossRef CAS.
- For selected reviews see:
(a) S. Serra, C. Fuganti and E. Brenna, Chem.–Eur. J., 2007, 13, 6782 CrossRef CAS;
(b) S. Kotha, S. Misra and S. Halder, Tetrahedron, 2008, 64, 10775 CrossRef CAS;
(c) L. Xiao, Z. Chen, B. Qu, J. Luo, S. Kong, Q. H. Gong and J. J. Kido, Adv. Mater., 2011, 23, 926 CrossRef CAS;
(d) O. Quinonero, C. Bressy and X. Bugaut, Angew. Chem., Int. Ed., 2014, 53, 10861 CrossRef CAS;
(e) T. N. Poudel, R. J. I. Tamargo, H. Cai and Y. R. Lee, Asian J. Org. Chem., 2018, 7, 985 CrossRef CAS.
- For selected reviews and examples, see:
(a) N. O. Calloway, Chem. Rev., 1935, 17, 327 CrossRef CAS;
(b) V. Snieckus, Beilstein J. Org. Chem., 2011, 7, 1215 CrossRef CAS;
(c) J. D. Kirkham, R. J. Butlin and J. P. Harrity, Angew. Chem., Int. Ed., 2012, 51, 6402 CrossRef CAS;
(d) S. Sivanathan, F. Körber and J. Scherkenbeck, Bioorg. Med. Chem., 2016, 24, 873 CrossRef CAS;
(e) R. Sunke, S. B. Nallapati, J. S. Kumar, K. S. Kumarb and M. Pal, Org. Biomol. Chem., 2017, 15, 4042 RSC.
-
(a) S. Saito and Y. Yamamoto, Chem. Rev., 2000, 100, 2901 CrossRef CAS;
(b) T. Kawasaki and Y. Yamamoto, J. Org. Chem., 2002, 67, 5138 CrossRef CAS;
(c) Y. Yamamoto, J. Ishii, H. Nishiyama and K. Itoh, J. Am. Chem. Soc., 2004, 126, 3712 CrossRef CAS;
(d) V. Gevorgyan, N. Tsuboya and Y. Yamamoto, J. Org. Chem., 2001, 66, 2743 CrossRef CAS;
(e) N. K. Swamy, L. K. Tatini, J. M. Babu, P. Annamalai and M. Pal, Chem. Commun., 2007, 1035 RSC;
(f) C. Xi, C. Chen, J. Lin and X. Hong, Org. Lett., 2005, 7, 347 CrossRef CAS;
(g) D. G. Yu, M. Yu, B. T. Guan, B. J. Li, Y. Zheng, Z. H. Wu and Z. J. Shi, Org. Lett., 2009, 11, 3374 CrossRef CAS;
(h) J. L. Gustafson, D. Lim, K. T. Barrett and S. J. Miller, Angew. Chem., Int. Ed., 2011, 50, 5125 CrossRef CAS;
(i) C. R. Reddy, U. Dilipkumar and M. D. Reddy, Org. Lett., 2014, 16, 3792 CrossRef;
(j) T. J. Colacot, New Trends in Cross-Coupling: Theory and Applications, Royal Society of Chemistry, Cambridge, 2015 Search PubMed;
(k) S. Zhou, B. W. Yan, S. X. Fan, J. S. Tian and T. P. Loh, Org. Lett., 2018, 20, 3975 CrossRef CAS.
-
(a) V. Snieckus, Chem. Rev., 1990, 90, 879 CrossRef CAS;
(b) W. A. L. Van Otterlo and C. B. De Koning, Chem. Rev., 2009, 109, 3743 CrossRef CAS;
(c) T. W. Lyons and M. S. Sanford, Chem. Rev., 2010, 110, 1147 CrossRef CAS;
(d) N. Kuhl, M. N. Hopkinson, J. Wencel-Delord and F. Glorius, Angew. Chem., Int. Ed., 2012, 51, 10236 CrossRef CAS;
(e) H. J. Reich, Chem. Rev., 2013, 113, 7130 CrossRef CAS.
- For selected reviews and examples, see:
(a) G. Domínguez and J. Pérez-Castells, Chem. Soc. Rev., 2011, 40, 3430 RSC;
(b) T. R. Hoye, B. Baire, D. Niu, P. H. Willoughby and B. P. Woods, Nature, 2012, 490, 208 CrossRef CAS;
(c) J. Feng and B. Liu, Tetrahedron Lett., 2015, 56, 1474 CrossRef CAS;
(d) T. N. Poudel, R. J. I. Tamargo, H. Cai and Y. R. Lee, Asian J. Org. Chem., 2018, 7, 985 CrossRef CAS;
(e) Z.-C. Shu, J.-B. Zhu, S. Liao, X.-L. Sun and Y. Tang, Tetrahedron, 2005, 61, 11449 CrossRef;
(f) P. Xie, Y. Huang and R. Chen, Chem.–Eur. J., 2012, 18, 7362 CrossRef CAS;
(g) A. Diallo, Y.-L. Zhao, H. Wang, S.-S. Li, C.-Q. Ren and Q. Liu, Org. Lett., 2012, 14, 5776 CrossRef CAS;
(h) L. Li, Y.-L. Zhao, H. Wang, Y.-J. Li, X. Xu and Q. Liu, Chem. Commun., 2014, 50, 6458 RSC;
(i) E. Gopi and I. N. N. Namboothiri, J. Org. Chem., 2014, 79, 7468 CrossRef CAS;
(j) T. N. Poudel and Y. R. Lee, Chem. Sci., 2015, 12, 7028 RSC;
(k) W. Song, S. A. Blaszczyk, J. Liu, S. Wang and W. Tang, Org. Biomol. Chem., 2017, 15, 7490 RSC;
(l) W. Tong, Q.-Y. Li, Y.-L. Xu, H.-S. Wang, Y.-Y. Chen and Y.-M. Pan, Adv. Synth. Catal., 2017, 359, 4025 CrossRef CAS;
(m) L. Satham and I. N. N. Namboothiri, J. Org. Chem., 2018, 83, 9471 CrossRef CAS;
(n) S. Li, X. X. Wu and S. Chen, Org. Biomol. Chem., 2019, 17, 789 RSC;
(o) C. Challa, J. Vellekkatt, J. Ravindran and R. S. Lankalapalli, Org. Biomol. Chem., 2014, 12, 8588 RSC;
(p) D. Yadav, S. K. Sharma and R. S. Menon, Org. Biomol. Chem., 2019, 17, 4073 RSC;
(q) Y. Xie, R. Huang, R. Li, C. Zhang, J. Fu, L. Zhao and J. Yuan, Chem. Commun., 2020, 56, 1948 RSC;
(r) F. Peng, Q. Zhao, W. Huang, S. J. Liu, Y. J. Zhong, Q. Mao, N. Zhang, G. He and B. Han, Green Chem., 2019, 21, 6179 RSC;
(s) Y. Ji, X. He, G. Li, Y. Ai, H. Li, C. Peng and B. Han, Org. Chem. Front., 2020, 7, 563 RSC.
- Y. Gu, P. Hu, C. Ni and X. Tong, J. Am. Chem. Soc., 2015, 137, 6400 CrossRef CAS.
-
(a) Q. Jia and J. Wang, Org. Lett., 2016, 18, 2212 CrossRef CAS;
(b) C.-L. Zhang, Z.-H. Gao, Z.-Q. Liang and S. Ye, Adv. Synth. Catal., 2016, 358, 2862 CrossRef CAS.
- C. L. Zhang, Z. F. Zhang, Z. H. Xia, Y. F. Han and S. Ye, J. Org. Chem., 2018, 83, 12507 CrossRef CAS.
- C.-Z. Zhu, Y. Wei and M. Shi, Adv. Synth. Catal., 2018, 360, 808 CrossRef CAS.
- CCDC-1957055 contains the supplementary crystallographic data of 3a for this paper.†.
-
(a) Z. Dost, S. Atilgan and E. U. Akkaya, Tetrahedron, 2006, 62, 8484 CrossRef CAS;
(b) A. Martin, C. Long, R. J. Forster and T. E. Keyes, Chem. Commun., 2012, 48, 5617 RSC.
- The adduct 7 was isolated from the reaction of 1a and 2a under standard conditions for 0.5 h and the structure was determined by NMR, HRMS, for details, see the ESI.†.
Footnote |
† Electronic supplementary information (ESI) available. CCDC 1957055. For ESI and crystallographic data in CIF or other electronic format see DOI: 10.1039/d0ra05251e |
|
This journal is © The Royal Society of Chemistry 2020 |
Click here to see how this site uses Cookies. View our privacy policy here.