DOI:
10.1039/C9QM00610A
(Research Article)
Mater. Chem. Front., 2020,
4, 532-536
A dual-mode orthogonally tunable fluorescent system covering the whole white light region†
Received
2nd October 2019
, Accepted 1st January 2020
First published on 2nd January 2020
Abstract
Using a supramolecular strategy to adjust the emission of organic small molecules has attracted considerable attention recently. Here, we design a fluorescent host–guest system consisting of two fluorophore components, perylene bis(diimide) (PDI) and coumarin, which could be adjusted in an orthogonal mode. This fluorescent host–guest system can be switched in multiple emissions, including white-light emission.
Developing white light emissive systems based on organic small molecules is crucial for organic light-emitting devices and their lighting applications.1,2 Many strategies have been developed to generate an organic white-light emissive system, one of which involves simultaneously mixing three types of fluorophores with primary RGB (red, green, and blue) colours in one system.3–5 By the multiple-component energy transfer process, white light emission would be obtained under single-wavelength excitation. However, this strategy requires a strictly fixed ratio and elaborate energy matching of the three fluorophores, which inevitably limits the library from expanding and practical applications. To overcome these limitations, some recent works presented a strategy to generate white-light emission by fluorescence-tunable molecular systems, meaning that the white light could be obtained by stimulus-induced modulation instead of tedious preparation by mixing in a fixed ratio,6,7 which facilitates the design and easier preparation. Such white-light emissive systems based on fluorescence-tunable molecular systems are also expected to be potentially developed into smart color-tunable light-emitting materials and devices.3,4,8,9
A fluorescent supramolecular system, especially an emissive host–guest system, is a rising emissive organic molecular system.10 Owing to the dynamic and stimulus-responsive advantages of supramolecular systems,11 some groups have developed white-light-emissive systems by tuning the emission of the multi-component supramolecular systems under external stimuli, such as macrocyclic host addition,6,12,13 excitation wavelength,6 and pH changes.12,14 These works exhibit the significance and potential of supramolecular fluorescent systems in the design and construction of tunable white light emissive materials. Therefore, it is a crucial task to enlarge the library of supramolecular white-light-emissive systems and routinize the control mode to precisely modulate the emission colours of the dynamic supramolecular systems.
In most previous cases, the emission of the two complementary colours, such as blue and yellow,15,16 could be modulated in a simultaneous but counter mode, thus resulting in a mixed-color emission including white light. However, in ideal organic light-emitting materials and devices, the two complementary colours should be tuned in an orthogonal mode, which means that the intensity of blue and yellow emission in an emission-mixed system can be tuned without interference by two discrete control units. This advanced control mode is crucial to generate pure white light emission due to its highly programmable and dual-mode-balanced ability compared with the single-stimulus-responsive fluorescent systems.2,9,15,17,18 However, it is a challenging task owing to the complexed dynamic nature of supramolecular fluorescent systems.
Herein, we report the design and fabrication of a novel dual-mode orthogonally tunable supramolecular fluorescent system. As shown in Fig. 1, two types of water-soluble fluorescent dyes, blue-light-emissive coumarin dye (1) and yellow-light-emissive perylene bis(diimide) (PDI) dye (2), are included in this system. These two dyes respectively respond to different stimulus types: the fluorescence intensity of 1 can be modulated by variable wavelengths of excitation lights; and that of 2 could be controlled by addition of macrocycle cucurbit[8]uril (CB[8]) owing to the enhanced emission enabled by host-inhibited aggregation.19 Interestingly, we find that the two fluorescence-tunable systems are orthogonally discrete, meaning that varying excitation wavelength (Mode I) only changes the fluorescence intensity of blue-emissive 1, and the addition/removal of host macrocycle CB[8] only affects the fluorescence intensity of yellow-light-emissive 2. Consequently, the mixture of the two emissive systems would result in an orthogonally tunable supramolecular fluorescent system, including white light emission in aqueous solution. The emission colour of this unique system can cover the whole region of white light by simple dual-mode modulation.
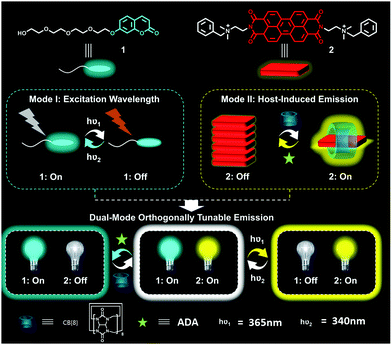 |
| Fig. 1 Schematic presentation of the orthogonally tunable fluorescent system. The hν1 and hν2 represent the excitation wavelengths to produce fluorescence of the dyes. | |
The synthesis procedure of the two fluorescent materials 1 and 2 can be found in the ESI.† All of the compound structures are confirmed by 1H and 13C NMR spectroscopies and high-resolution mass spectrometry (Fig. S1–S5, ESI†). The relationship between excitation wavelength and fluorescence of the two dyes is investigated as shown in Fig. 1. For 1, the fluorescence intensity at 400 nm decreases to less than 2% of the original intensity when the excitation wavelength varies from 340 nm to 370 nm (Fig. 2a and Fig. S6, ESI†), exhibiting a sensitive variation to the excitation wavelength. In contrast, compound 2 shows insensitive fluorescence response to the variation of excitation wavelength: the intensity only increases to 250% of the original state when the excitation wavelength varies from 340 nm to 370 nm (Fig. 2b and Fig. S7, ESI†). The remarkable differences of the fluorescence responses of the two dyes to the variation of excitation wavelengths are integrated as shown in Fig. 3c, indicating that varying excitation wavelengths (Mode I) bear response-specificity to the fluorescence intensity of 1 instead of 2, which meets our requirement in constructing the orthogonally controlled fluorescent system. Since the fluorescence intensity of 1 can be tuned obviously by changing the excitation wavelength, we focus on the adjustment of the other one (Mode II).
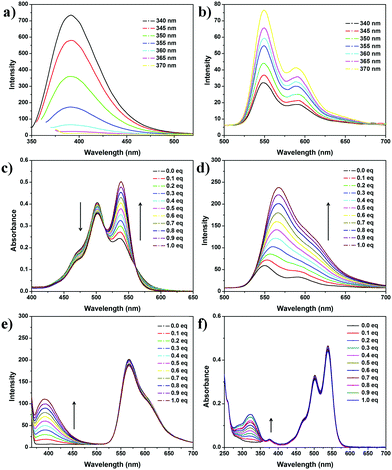 |
| Fig. 2 (a) Fluorescence emission spectra of 1 (10 μM) solution at different excitation wavelengths. (b) Fluorescence emission spectra of 2 (10 μM) solution at different excitation wavelengths. (c) UV-vis absorption and (d) fluorescence emission spectra (upon excitation at 355 nm) of 2 (10 μM) solution with increasing concentrations of CB[8]. (e) Fluorescence emission (upon excitation at 355 nm) and (f) UV-vis absorption spectra of 2 (10 μM) and CB[8] (10 μM) solution with increasing concentrations of 1. | |
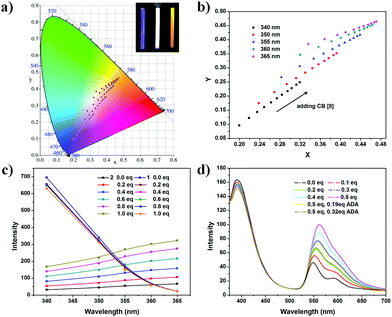 |
| Fig. 3 (a and b) The corresponding 1931 CIE coordinate diagram of 2 (10 μM) and 1 (10 μM) solution at increasing concentrations of CB[8] and different excitation wavelengths (inset: fluorescence photographs of the solution under specific conditions). (c) Plots of the intensity of 2 (10 μM) and 1 (10 μM) solutions with different equivalents of CB[8] at different excitation wavelengths. (d) Fluorescence emission spectra (upon excitation at 355 nm) of 2 (5 μM) and 1 (100 μM) solutions at increasing concentrations of CB[8] and addition of ADA. | |
To increase the fluorescence quantum yield of 2, the host-inhibited aggregation strategy is used. Macrocycle CB[8] can form a 1
:
1 host–guest complex with 2, inhibiting the π–π stacking aggregation of 2 and thus boosting the fluorescence intensity remarkably.19 The host–guest combination between 2 and CB[8] is studied by 1H NMR spectroscopy (Fig. S8, ESI†). The proton signal of 2 exhibits a broad peak owing to the aggregated state in D2O. After adding 1 molar equivalent CB[8], the PDI proton peaks shift downfield from 7.90 ppm to 7.97 ppm and showed remarkable sharpening, indicating the disassembly of 2 after addition of CB[8]. The benzyl proton peaks of 2 shift downfield obviously (Δ1.24 ppm), indicating that CB[8] binds the aromatic ring of PDI. After confirmation of the host–guest combination between CB[8] and 2, we then focus on the correlation between CB[8] concentration and the photophysical properties of the two dyes. Titration experiments are performed by adding CB[8] into the dye solutions. 2 exhibits a remarkable response to the addition of CB[8]. As shown in Fig. 2c, the absorption peak of 2 at 540 nm increases, while the absorption peak at 500 nm decreases slightly, which is attributed to the decreased H-aggregates induced by the inhibition effect of macrocycle CB[8]. No distinctive changes are observed after addition of 1 equivalent CB[8], suggesting the 1
:
1 type of host–guest system. Isothermal titration calorimetry also proves this conclusion (Fig. S9, ESI†). Significantly, the fluorescence peak of 2 increases remarkably with the addition of CB[8], which is also accompanied by a slight redshift of the peak wavelength from 550 nm to 570 nm (Fig. 2d).
Considering that there is still enough space in the cavity of the 2@CB[8] to bind another guest,19 we suspect that 1 can be bound into the cavity, which may cause change of the fluorescence.
First, we study whether 1 is responsive to the macrocycle CB[8]. According to the 1H NMR spectra (Fig. S10, ESI†), all the proton peaks of 1 show no obvious changes after addition of CB[8] to the solution of 1. In addition, 1 exhibits no response to the addition of CB[8] in optical absorption and fluorescence spectra (Fig. S11 and S12, ESI†), which is a reasonable result because of the lack of host–guest combination between neutral 1 and CB[8]. What is more, the result is also confirmed by fluorescence lifetime (Fig. S13, ESI†).
Then we try to find out whether 1 can be bound into the cavity of the 2·CB[8] complex. From 1H NMR spectra (Fig. S14, ESI†), the PDI proton and benzyl proton peaks of 2 have no remarkable shift when adding 1 to the solution of 2 and CB[8], suggesting that 1 does not bind in the cavity of 2@CB[8]. Further study of the UV/vis and fluorescence spectra confirms the proposed hypothesis (Fig. 2c and d). With the addition of a concentrated CB[8] solution to the 1 and 2 mixture, the absorption peak of 2 at 540 nm rises gradually and the absorption peak at 500 nm decreases slightly (Fig. S15, ESI†), almost the same as that of to the pure 1 solution (Fig. 2c). It is also confirmed by fluorescence spectra (Fig. S16, S17, ESI,† and Fig. 2d). To further confirm the orthogonality, a titration experiment is performed by adding 1 to the 1
:
1 molar ratio-mixed aqueous solution of 2 and CB[8] (Fig. 2e and f). The absorption and fluorescence spectra show no obvious change of the peak of 2@CB[8] when the coumarin concentration was increasing. Hence, the two dyes 1 and 2 exhibit different fluorescence responses to the addition of macrocycle CB[8], which is vital to construct the orthogonally switchable system.
Fluorescence emission of 1 can be easily tuned by changing the excitation wavelength (Fig. 2a). When the excitation wavelength changes from 340 nm to 370 nm, the fluorescence of 1 decreases to less than 2% of the original intensity, while that of 2 keeps basically unchanged (Fig. S18 and S19, ESI†). Comparing the maximum fluorescence emission peaks of 2 and 1 upon excitation at different wavelengths, it can be found that the maximum emission peaks of 2 change about 2 times upon excitation at 365 nm compared to that at 340 nm, while the peaks of 1 change about 30 times. Meanwhile, adding 0.2 or 1.0 equivalent of CB[8] solution, the same conclusion can be obtained (Fig. 3c and Fig. S19, ESI†), indicating that the fluorescence of 2@CB[8] acts in the same way as that of 2, when the excitation wavelength varies (Fig. S7, ESI†).
According to the above result, the blue-yellow color shift can be tuned by varying the excitation wavelength and the addition of macrocycle CB[8] (Fig. 3a). Fluorescence emission spectra of 2 (10 μM) and 1 (10 μM) solution upon excitation at different wavelengths show a tunable fluorescence from blue (CIE coordinates (0.20, 0.10)) to yellow (CIE coordinates (0.34,0.38)) (Fig. S18 and S20, ESI†). A white emission colour calculated to be (0.32, 0.35) could be met upon excitation at 360 nm. After adding CB[8] into the mixture, the fluorescence emission of 2 is greatly improved, showing a higher fluorescence quantum yield (yield of 2 = 11.1%, yield of 2@CB[8] = 47.4%, Fig. S21 and S22, ESI†). CIE coordinates of the colours change from (0.20,0.10) to (0.32, 0.25) (Fig. 3b).
After confirming that the supramolecular fluorescent system is dual-mode orthogonally tunable, we measured the fluorescence spectra of this system in an orthogonal fashion (addition of CB[8] solution and different excitation wavelengths). A very broad range of the color is found after all of the color coordinates are calculated. From the color diagram, it can be found that the CIE coordinates obtained from any one of the two modes are in a straight line within limits (Fig. 3a, b and Fig. S23, ESI†).
Varying excitation wavelength can be achieved easily, while the concentrations of 2@CB[8] can be controlled in a slight way (Fig. S24 and S25, ESI†). In addition, mode II is also a reversible switch. From ref. 19, we find that amantadine (ADA), a common guest, has a higher binding constant than that of PDI derivatives. The addition of amantadine can turn off the fluorescence emission of 2.
To get a more accurate white light and a higher system efficiency, different concentrations of the two dyes are tried. For instance, a solution of 2 (5 μM) and 1 (100 μM) can emit a white light (CIE coordinates (0.33, 0.32)) upon excitation at 355 nm, with 0.2 equivalent of CB[8] (1 μM) (Fig. 3d and Fig. S26, ESI†). The fluorescence emission changes from white to yellow after addition of more CB[8] solution (Fig. S27 and S28, ESI†). The addition of the stronger guest, amantadine, can turn off the yellow emission, leading to white emission again (Fig. 3d and Fig. S28, S29, ESI†). During the adjustment, the colours of the fluorescence emissions change from a cold white to a warm one.
Conclusions
In conclusion, we have developed a novel dual-mode orthogonally tunable supramolecular fluorescent system. In this fluorescent system, one dye exhibits a sensitive variation to the excitation wavelength, while the other one responds to the addition/removal of host CB[8]. This fluorescent system is orthogonal, easy to switch, and multi-colored. This simple strategy may lead to the appearance of more orthogonally controlled fluorescent systems and organic emitting devices.
Conflicts of interest
There are no conflicts to declare.
Acknowledgements
This work was supported by the National Natural Science Foundation of China (grants 21788102, 21790361, 21871084, 21672060, and 21421004), Shanghai Municipal Science and Technology Major Project (Grant No. 2018SHZDZX03), the Fundamental Research Funds for the Central Universities, the Programme of Introducing Talents of Discipline to Universities (grant B16017), Program of Shanghai Academic/Technology Research Leader (19XD1421100), and the Shanghai Science and Technology Committee (grant 17520750100). We thank the Research Center of Analysis and Test of East China University of Science and Technology for help with the material characterization.
References
-
(a) Q. Yang and J. Lehn, Bright White-Light Emission from a Single Organic Compound in the Solid State, Angew. Chem., Int. Ed., 2014, 53, 4572–4577 CrossRef CAS PubMed;
(b) X. Jin, C. Chen, C. Ren, L. Caia and J. Zhang, Bright white-light emission from a novel donor-acceptor organic molecule in the solid state via intermolecular charge transfer, Chem. Commun., 2014, 50, 15878–15881 RSC;
(c) D. K. Maiti, R. Bhattacharjee, A. Datta and A. Banerjee, Modulation of Fluorescence Resonance Energy Transfer Efficiency for White Light Emission from a Series of Stilbene-Perylene Based Donor-Acceptor Pair, J. Phys. Chem. C, 2013, 117, 23178–23189 CrossRef CAS;
(d) S. L. Sonawane and S. K. Asha, Blue, Green, and Orange-Red Emission from Polystyrene Microbeads for Solid-State White-Light and Multicolor Emission, J. Phys. Chem. B, 2014, 118, 9467–9475 CrossRef CAS PubMed;
(e) K. V. Rao, K. K. R. Datta, M. Eswaramoorthy and S. J. George, Highly Pure Solid-State White-Light Emission from Solution-Processable Soft-Hybrids, Adv. Mater., 2013, 25, 1713–1718 CrossRef CAS PubMed;
(f) W. Geng, Y. Liu, Y. Wang, Z. Xu, Z. Zheng, C. Yanga and D. Guo, A self-assembled white-light-emitting system in aqueous medium based on a macrocyclic amphiphile, Chem. Commun., 2017, 53, 392–395 RSC.
- W. Ding, J. Sun, G. Chen, L. Zhou, J. Wang, X. Gu, J. Wan, X. Pu, B. Tang and Z. L. Wang, Stretchable multi-luminescent fibers with AIEgens, J. Mater. Chem. C, 2019, 7, 10769–10776 RSC.
-
(a) C. Giansante, C. Schäfer, G. Raffy and A. D. Guerzo, Exploiting Direct and Cascade Energy Transfer for Color-Tunable and White-Light Emission in Three-Component Self-Assembled Nanofibers, J. Phys. Chem. C, 2012, 116, 21706–21716 CrossRef CAS;
(b) H. Peng, C. Sun, L. Niu, Y. Chen, L. Wu, C. Tung and Q. Yang, Supramolecular Polymeric Fluorescent Nanoparticles Based on Quadruple Hydrogen Bonds, Adv. Funct. Mater., 2016, 26, 5483–5489 CrossRef CAS;
(c) P. Pallavi, S. Bandyopadhyay, J. Louis, A. Deshmukh and A. Patra, A soluble conjugated porous organic polymer: efficient white light emission in solution, nanoparticles, gel and transparent thin film, Chem. Commun., 2017, 53, 1257–1260 RSC;
(d) Z. M. Hudson, D. J. Lunn, M. A. Winnik and I. Manners, Colour-tunable fluorescent multiblock micelles, Nat. Commun., 2014, 5, 3372 CrossRef PubMed;
(e) M. Zuo, W. Qian, T. Li, X. Hu, J. Jiang and L. Wang, Full-Color Tunable Fluorescent and Chemiluminescent Supramolecular Nanoparticles for Anti-counterfeiting Inks, ACS Appl. Mater. Interfaces, 2018, 10, 39214–39221 CrossRef CAS PubMed;
(f) H. Ding, J. Li, G. Xie, G. Lin, R. Chen, Z. Peng, C. Yang, B. Wang, J. Sun and C. Wang, An AIEgen-based 3D covalent organic framework for white light-emitting diodes, Nat. Commun., 2018, 9, 5234 CrossRef CAS PubMed;
(g) Z. Wang, Z. Wang, B. Lin, X. Hu, Y. Wei, C. Zhang, B. An, C. Wang and W. Lin, Warm-White-Light-Emitting Diode Based on a Dye-Loaded Metal-Organic Framework for Fast White-Light Communication, ACS Appl. Mater. Interfaces, 2017, 9, 35253–35259 CrossRef CAS PubMed;
(h) S. Guo, X. Yan, Z. Luo, M. Ding, H. Liu and X. Yang, Tunable WLED with UV chip based on all-inorganic perovskite quantum dots, Chem. Phys. Lett., 2019, 720, 124–127 CrossRef CAS.
- P. Chen, Q. Li, S. Grindy and N. Holten-Andersen, White-Light-Emitting Lanthanide Metallogels with Tunable Luminescence
and Reversible Stimuli-Responsive Properties, J. Am. Chem. Soc., 2015, 137, 11590–11593 CrossRef CAS PubMed.
- Z. Zhang, Y. He, L. Liu, X. Lü, X. Zhu, W. Wong, M. Panc and C. Suc, Pure white-light and colour-tuning of Eu3+-Gd3+-containing metallopolymer, Chem. Commun., 2016, 52, 3713–3716 RSC.
-
Q. Zhang, D. Li, X. Li, P. B. White, J. Mecinović, X. Ma, H. Ågren, R. J. M. Nolte and H. Tian, Multicolor Photoluminescence Including White-Light Emission by a Single Host–Guest Complex, J. Am. Chem. Soc., 2016, 138, 13541–13550 Search PubMed.
- Y. Lei, Q. Liao, H. Fu and J. Yao, Orange-Blue-Orange Triblock One-Dimensional Heterostructures of Organic Microrods for White-Light Emission, J. Am. Chem. Soc., 2010, 132, 1742–1743 CrossRef CAS PubMed.
- H. Wang, X. Ji, Z. Li, C. N. Zhu, X. Yang, T. Li, Z. Wu and F. Huang, Preparation of a white-light-emitting fluorescent supramolecular polymer gel with a single chromophore and use of the gel to fabricate a protected quick response code, Mater. Chem. Front., 2017, 1, 167–171 RSC.
- J. Li, H. Zhang, Y. Zhang, W. Zhou and Y. Liu, Room-Temperature Phosphorescence and Reversible White Light Switch Based on a Cyclodextrin Polypseudorotaxane Xerogel, Adv. Opt. Mater., 2019, 1900589 CrossRef CAS.
- X. Ni, S. Chen, Y. Yang and Z. Tao, Facile Cucurbit[8]uril-Based Supramolecular Approach To Fabricate Tunable Luminescent Materials in Aqueous Solution, J. Am. Chem. Soc., 2016, 138, 6177–6183 CrossRef CAS PubMed.
-
(a) Q. Zhang, D. Qu and H. Tian, Photo-Regulated Supramolecular Polymers: Shining Beyond Disassembly and Reassembly, Adv. Opt. Mater., 2019, 7, 1900033 CrossRef;
(b) Q. Zhang, S. Rao, T. Xie, X. Li, T. Xu, D. Li, D. Qu, Y. Long and H. Tian, Muscle-like Artificial Molecular Actuators for Nanoparticles, Chem, 2018, 4, 2670–2684 CrossRef CAS;
(c) Q. Zhang, Y. Deng, H. Luo, C. Shi, G. M. Geise, B. L. Feringa, He Tian and D. Qu, Assembling a Natural Small Molecule into a Supramolecular Network with High Structural Order and Dynamic Functions, J. Am. Chem. Soc., 2019, 141, 12804–12814 CrossRef CAS PubMed;
(d) Q. Zhang, C. Shi, D. Qu, Y. Long, B. L. Feringa and H. Tian, Exploring a naturally tailored small molecule for stretchable, self-healing, and adhesive supramolecular polymers, Sci. Adv., 2018, 4, eaat8192 CrossRef CAS PubMed;
(e) Q. Zhang, R. Xing, W. Wang, Y. Deng, D. Qu and H. Tian, Dynamic Adaptive Two-Dimensional Supramolecular Assemblies for On-Demand Filtration, iScience, 2019, 19, 14–24 CrossRef PubMed.
- L. Yang, F. Jia, J. Cui, S. Lu and W. Jiang, Light-Controlled Switching of a Non-photoresponsive Molecular Shuttle, Org. Lett., 2017, 19, 2945–2948 CrossRef CAS PubMed.
- H. Yang, Y. Liu, K. Liu, L. Yang, Z. Wang and X. Zhang, Rational Adjustment of Multicolor Emissions by Cucurbiturils-Based Host-Guest Chemistry and Photochemistry, Langmuir, 2013, 29, 12909–12914 CrossRef CAS PubMed.
- X. Zhang, S. Rehm, M. M. Safont-Sempere and F. Würthner, Vesicular perylene dye nanocapsules as supramolecular fluorescent pH sensor systems, Nat. Chem., 2009, 1, 623–629 CrossRef CAS PubMed.
- M. Zhang, S. Yin, J. Zhang, Z. Zhou, M. L. Saha, C. Lu and P. J. Stang, Metallacycle-cored supramolecular assemblies with tunable fluorescence including white-light emission, Proc. Natl.
Acad. Sci. U. S. A., 2017, 114, 3044–3049 CrossRef CAS PubMed.
-
(a) S. Shao, J. Ding, L. Wang, X. Jing and F. Wang, White Electroluminescence from All-Phosphorescent Single Polymers on a Fluorinated Poly(arylene ether phosphine oxide) Backbone Simultaneously Grafted with Blue and Yellow Phosphors, J. Am. Chem. Soc., 2012, 134, 20290–20293 CrossRef CAS PubMed;
(b) L. Zhu, C. Y. Ang, X. Li, K. T. Nguyen, S. Y. Tan, H. Ågren and Y. Zhao, Luminescent Color Conversion on Cyanostilbene-Functionalized Quantum Dots via In-situ Photo-Tuning, Adv. Mater., 2012, 24, 4020–4024 CrossRef CAS PubMed;
(c) S. Guo, Y. Song, Y. He, X. Hu and L. Wang, Highly Efficient Artificial Light-Harvesting Systems Constructed in Aqueous Solution Based on Supramolecular Self-Assembly, Angew. Chem., Int. Ed., 2018, 57, 3163–3167 CrossRef CAS PubMed;
(d) Q. Zhao, Y. Chen, S. Lia and Y. Liu, Tunable white-light emission by supramolecular self-sorting in highly swollen hydrogels, Chem. Commun., 2018, 54, 200–203 RSC.
-
(a) X. Yan, T. R. Cook, P. Wang, F. Huang and P. J. Stang, Highly emissive platinum(II) metallacages, Nat. Chem., 2015, 7, 342–348 CrossRef CAS PubMed;
(b) M. J. Bowers II, J. R. McBride and S. J. Rosenthal, White-Light Emission from Magic-Sized Cadmium Selenide Nanocrystals, J. Am. Chem. Soc., 2005, 127, 15378–15379 CrossRef PubMed;
(c) L. Zhu, M. T. Trinh, L. Yina and Z. Zhang, Sequential oligodiacetylene formation for progressive luminescent color conversion via co-micellar strategy, Chem. Sci., 2016, 7, 2058–2065 RSC;
(d) Y. Chen, P. Chen, H. Peng, Y. Zhao, H. Ding, L. Wu, C. Tunga and Q. Yang, Water-soluble, membrane-permeable organic fluorescent nanoparticles with large tunability in emission wavelengths and Stokes shifts, Chem. Commun., 2013, 49, 5877–5879 RSC;
(e) Z. Xie, C. Chen, S. Xu, J. Li, Y. Zhang, S. Liu, J. Xu and Z. Chi, White-Light Emission Strategy of a Single Organic Compound with Aggregation-Induced Emission and Delayed Fluorescence Properties, Angew. Chem., Int. Ed., 2015, 54, 7181–7184 CrossRef CAS PubMed;
(f) S. A. Schmid, R. Abbel, A. P. H. Schenning, E. W. Meijer, R. P. Sijbesma and L. M. Herz, Analyzing the Molecular Weight Distribution in Supramolecular Polymers, J. Am. Chem. Soc., 2009, 131, 17696–17704 CrossRef CAS PubMed;
(g) A. Ozawa, A. Shimizu, R. Nishiyabu and Y. Kubo, Thermo-responsive white-light emission based on tetraphenylethylene- and rhodamine B-containing boronate nanoparticles, Chem. Commun., 2015, 51, 118–121 RSC;
(h) P. Malakar, D. Modak and E. Prasad, Pure white light emission from organic molecules using solvent induced selective self-assembly, Chem. Commun., 2016, 52, 4309–4312 RSC.
- H. Wu and Y. Zhao, Colour-tunable ultra-long emission, Nat. Photonics, 2019, 13, 373–375 CrossRef CAS.
- F. Biedermann, E. Elmalem, I. Ghosh, W. M. Nau and O. A. Scherman, Strongly Fluorescent, Switchable Perylene Bis(diimide) Host–Guest Complexes with Cucurbit[8]uril In Water, Angew. Chem., Int. Ed., 2012, 51, 7739–7743 CrossRef CAS PubMed.
Footnote |
† Electronic supplementary information (ESI) available. See DOI: 10.1039/c9qm00610a |
|
This journal is © the Partner Organisations 2020 |
Click here to see how this site uses Cookies. View our privacy policy here.