DOI:
10.1039/C8SC05225E
(Edge Article)
Chem. Sci., 2019,
10, 3733-3737
Dithiane-directed Rh(III)-catalyzed amidation of unactivated C(sp3)–H bonds†
Received
23rd November 2018
, Accepted 18th February 2019
First published on 18th February 2019
Abstract
An oxidant-free Rh(III)-catalyzed direct amidation of alkyl dithianes via C(sp3)–H bond activation utilizing diverse and robust dioxazolone reagents is reported. The reaction hinges on use of a Cp*Rh(III) complex in combination with an essential amino-carboxylate additive to generate usefully protected 1,3-aminoaldehyde derivatives. The scalability of the reaction was demonstrated as was a series of downstream product functionalizations, including dithiane deprotection, anion alkylation and reductive desulfurization, highlighting the general applicability of this transformation in the synthesis of novel scaffolds and building blocks.
Dithianes are versatile building blocks in synthesis, acting as stable carbonyl and masked methylene surrogates as well as unique umpolung C1 acyl anion synthons as first pioneered by Corey and Seebach.1 Their synthetic accessibility combined with impressive and unique reactivity has led to widespread adoption of dithianes in complex target synthesis for this purpose.2 Alongside the very well-established lithium anion umpolung reactivity, discovery of new applications for this functional group has been the focus of much interest over the past decade3–6.
In parallel, transition metal catalyzed direct C–H functionalization reactions are a powerful strategy for step- and atom-economic synthesis,7 and Rh(III)-catalysis has emerged in recent years as a powerful tool in the activation of various C(sp2)–H bonds. This area has been extensively reviewed,8 with a wide range of oxygen and nitrogen containing directing groups being found to be suitable in chelation-assisted activation of such bonds. However the use of sulfur-containing groups has been much less developed;9 the high Lewis basicity of sulfur and its strong coordination to metal centres is generally problematic for catalysis due to potential formation of less reactive, thermodynamically stable cyclometallated species.
Extension of Rh(III)-catalysis to the much more challenging C(sp3)–H bond activation is an attractive, but difficult synthetic proposition which is still largely unrealized. Only a handful of examples exist for the activation of reactive C(sp3)–H bonds such as at acidic, allylic or benzylic positions.10 Regarding unactivated C(sp3)–H bonds, the high C–H bond strength, steric hinderance and low reactivity of the resulting Rh–C(alkyl) species are significant challenges which have only recently been reduced to practice by the seminal reports of You and Li.11 Both these reports have made a significant advancement to the field,12 but their use of strongly coordinating pyridyl and ketoxime functionality also highlights the need for identification of alternative directing groups which can be easily installed, cleaved, and enable synthetically useful downstream functionalization.
To this end, we decided to investigate dithiane functionality as a potential directing group for C–H activation of unreactive C(sp3)–H bonds.13 Dithianes have only previously been used once in catalytic C–H bond activation by Miura,14 enabling ortho C(sp2)–H alkenylation of benzylic dithianes with electron deficient olefins. Activation of alkyl substituted dithianes would therefore represent a powerful advancement, expanding the utility of this versatile functional group and enabling synthesis of sp3-rich scaffolds and building blocks bearing a useful umpolung acyl anion equivalent. Herein, we wish to report our findings (Scheme 1).
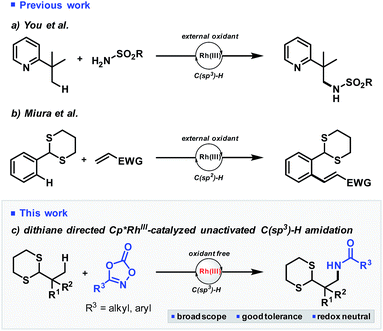 |
| Scheme 1 (a) You's seminal report on Rh(III)-catalyzed functionalization of unactivated C(sp3)–H bonds. (b) Miura's oxidative C(sp2)–H ortho alkenylation of benzylic dithianes. (c) This work. | |
We began our studies by assessing Cp*Rh(III) catalysis applied to pivaldehyde-derived dithiane 1a and dioxazolone 2a as model substrates. Pleasingly, treatment of 1a with catalytic [Cp*Rh(MeCN)3][(SbF6)2] and sodium benzoate in dichloroethane (DCE) at 80 °C led to formation of amidodithiane 3a in 16% yield (entry 1, Table 1). Remarkably, the reaction profile was extremely clean, with starting material conversion reflecting yield of product, and no bis-amidated was observed. Encouraged by this significant lead result establishing proof of concept, optimization studies were performed. Initial focus was placed on the solvent, and DCE proved optimal (entry 1), with toluene, dioxane and chloroform (entries 2–4) all completely suppressing reactivity. Next, a varied range of carboxylic acid salts15 – which were formed in situ by neutralisation of the acid with a slight excess of sodium tert-butoxide – were evaluated as additives to promote the reaction. Sterically bulky adamantyl and pivalic acid salts (entries 5 and 6), which are commonly used in C–H activation, provided only slightly improved yields of 23% and 18% respectively. Accordingly, we then turned towards amino acid salts as potential additives for the reaction (Table 1, entry 7 and Scheme 2). A range of differentially protected α-amino acid derivatives (4a–o) were screened, and interestingly Fmoc-protected sodium prolinate (4h) was found to be the most effective. Studies found 30 mol% of additive was optimal (entries 8–10) and use of sodium as the carboxylate counterion was critically important, with potassium, lithium and silver salts all displaying no reactivity whatsoever (entries 11–13). Temperature variations were also made, with the reaction yield decreasing when the temperature was both lowered to 70 °C (entry 14) or increased to 90 °C (entry 15). Finally, after extensive studies of all other reaction parameters, increasing the Rh catalyst loading from 10 to 15 mol% improved the yield to 71% (entry 16).
Table 1 Optimisation of reaction parameters

|
Entrya |
Solvent |
Additive [mol%] |
T [°C] |
Cat. loading [mol %] |
Yieldb [%] |
Reaction conditions: 1a (0.05 mmol), 2a (0.1 mmol), catalyst, additive, solvent (0.5 mL), temperature, 36 h.
Yield calculated by 1H NMR with methyl para-nitrobenzoate as internal standard; isolated yield of product in parentheses.
|
1 |
DCE |
PhCO2 Na (40) |
80 |
10 |
16 |
2 |
Toluene |
PhCO2 Na (40) |
80 |
10 |
0 |
3 |
Dioxane |
PhCO2 Na (40) |
80 |
10 |
Trace |
4 |
CHCI3 |
PhCO2 Na (40) |
80 |
10 |
Trace |
5 |
DCE |
AdCO2 Na (40) |
80 |
10 |
23 |
6 |
DCE |
PivCO2 Na (40) |
80 |
10 |
18 |
7 |
DCE |
Amino acid (40) |
80 |
10 |
Scheme 2
|
8 |
DCE |
Fmoc-Pro-ONa (40) |
80 |
10 |
55 |
9 |
DCE |
Fmoc-Pro-ONa (30) |
80 |
10 |
62 |
10 |
DCE |
Fmoc-Pro-ONa (20) |
80 |
10 |
57 |
11 |
DCE |
Fmoc-Pro-OK (30) |
80 |
10 |
0 |
12 |
DCE |
Fmoc-Pro-OLi (30) |
80 |
10 |
0 |
13 |
DCE |
Fmoc-Pro-OAg (30) |
80 |
10 |
0 |
14 |
DCE |
Fmoc-Pro-ONa (30) |
70 |
10 |
45 |
15 |
DCE |
Fmoc-Pro-ONa (30) |
90 |
10 |
47 |
16
|
DCE
|
Fmoc-Pro-ONa (30)
|
80
|
15
|
73(71)
|
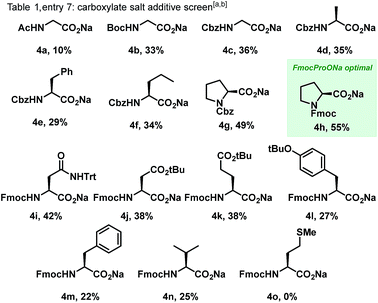 |
| Scheme 2 Evaluation of amino acid additives. aReaction conditions: 1a (0.05 mmol), 2a (0.1 mmol), [Cp*Rh(MeCN)3][(SbF6)2] (10 mol%), Fmoc-Pro-ONa (40 mol%), solvent (0.5 mL), temperature, 36 h. bYield calculated by 1H NMR with methyl para-nitrobenzoate as internal standard. | |
With optimal reaction conditions in hand, we explored the scope of the reaction, initially focusing on the dioxazolone amidating reagent. The reaction was found to be tolerant of a wide range of functionalized dioxazolones, with p-F, p-NO2 and m-OMe substituted phenylacetyl dioxazolones providing the amidated products in 74%, 40% and 67% yield respectively (Scheme 3, 3b–d). Use of aromatic substituted dioxazolones was also tolerated, although a slight decrease of yield to moderate levels was observed. Phenyl, p-tolyl and bis-meta-methoxy substituted dioxazolones afforded good yields of the corresponding products (3e–g). Furan-bearing dithiane 3h could also be synthesized in 46% yield. We postulate the reduced yield with aromatic dioxazolones was due to increased steric hinderance about the reaction centre, impeding reactivity. Despite this, a diverse range of acyclic alkyl substituted dioxazolones (2i–o) was shown to be successful, thus demonstrating applicability of this method towards the synthesis of sp3 rich scaffolds (3i–o). Finally, dioxazolones bearing medicinal chemistry-relevant small rings were also evaluated,16 with cyclopropyl (2n) and cyclobutyl (2o) bearing dithiane products (3n, 3o) isolated in 45% and 52% yield respectively.
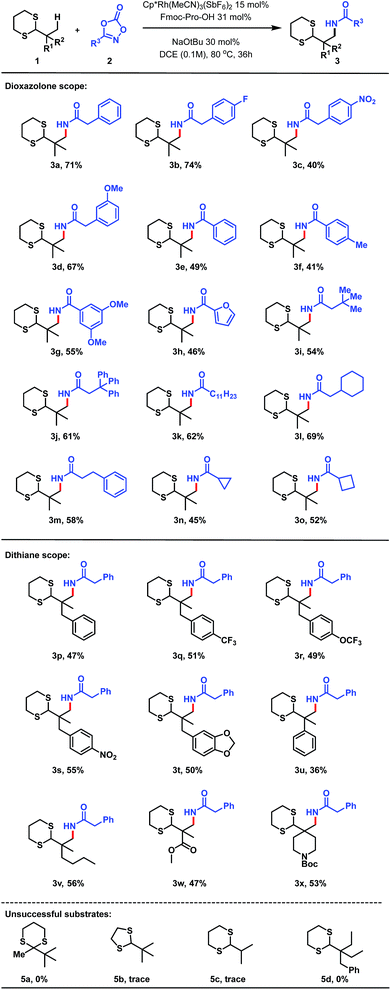 |
| Scheme 3 C(sp3)–H amidation of a range of dithianes 1 with diverse dioxazolones 2. | |
The scope with respect to the dithiane partner was also found to be broad. Pleasingly, phenyl bearing dithiane (1p) delivered the corresponding amidated product in 47% yield.17 Trifluoromethyl (1q) and trifluoromethoxy (1r) substitution on the aromatic ring was also successfully accommodated, giving amidated products (3q, 3r) in 51% and 49% yield respectively. The reaction was also tolerant of electron deficient para-nitro substituted dithiane (3s, 55%) as well as electron rich benzodioxole bearing dithiane (3t, 50%). Phenyl substitution directly at the quaternary centre delivered amidated product 3u, albeit in low yield. Extension of the alkyl chain to a four-carbon subunit (1v) displayed a 56% yield for the corresponding amidated product 3v. Pleasingly, methyl ester derived dithiane 1w could also be activated, delivering amidoester product 3w in 47% yield; demonstrating notable functional group tolerance for this methodology. Finally, N-Boc protected piperidine derived dithiane was utilized to afford 3x in 53% yield. Control reactions using modified substrates were also performed. Interestingly, ketone-derived dithiane 5a was completely unreactive to the standard reaction conditions, and dithiolane 5b delivered only trace amounts of amidated product, highlighting the significance of the 6-membered ring aldehyde-derived dithiane for this transformation. The lack of reactivity in the case of 5c demonstrates the requirement for an alpha-quaternary carbon. Ethyl-bearing dithiane 5d was found to be completely unreactive, showing the unique selectivity of this method for methyl C–H bonds.
To investigate the practicality and efficiency of this transformation, a 56-fold scale up to 2.8 mmol (500 mg of 1a) was performed using standard reaction conditions (Scheme 4a) and pleasingly 3a was afforded in good yield. To demonstrate synthetic utility and versatility of the dithiane moiety, product derivatization was explored. Deprotection of dithiane 3a to the corresponding aldehyde 6 was efficiently performed in 5 minutes with diacetoxyiodobenzene in a TFA/acetonitrile solvent mixture (Scheme 4b). Importantly, amido-dithiane 3e was demonstrated to be successful in the Corey-Seebach alkylation with allyl bromide, providing allylated derivative 7 in 61% (Scheme 4c). Finally, desulfurization with RANEY® nickel to reveal a masked quaternary methyl group was performed on dithiane 3x, affording 8 in 88% yield (Scheme 4d). This method provides an attractive method towards synthesis of such quaternary methyl bearing building blocks18 and structures such as 6–8 are attractive building blocks which could be of value within pharmaceutical drug discovery programmes.
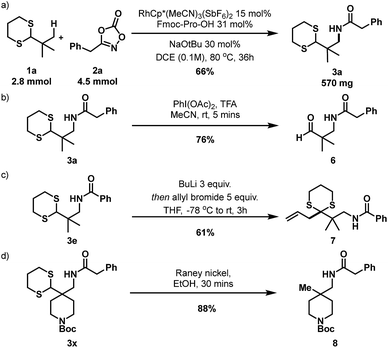 |
| Scheme 4 (a) 56-fold scale-up reaction. (b) Oxidative deprotection of dithiane. (c) Corey–Seebach alkylation with allyl bromide. (d) Reductive desulfurization. | |
Conclusions
We have discovered and developed a Cp*Rh(III) catalyzed C–H amidation of unactivated C(sp3)–H bonds utilizing the synthetically versatile dithiane as a directing group. The method is tolerant of a diverse range of aryl and alkyl dioxazolones as well as modifications on the dithiane partner. The work constitutes a rare example of Rh(III) catalyzed C(sp3)–H activation of unreactive C–H bonds, and first use of dithianes for such alkyl C–H bonds. Varied modifications of the products exploiting the versatility of the dithiane group were also performed, demonstrating the high synthetic value of this method for use within industry and academia.
Conflicts of interest
The authors declare no competing interests.
Acknowledgements
H. S. is grateful to the EPSRC Centre for Doctoral Training in Synthesis for Biology and Medicine (EP/L015838/1) for a studentship, generously supported by AstraZeneca, Diamond Light Source, Defence Science and Technology Laboratory, Evotec, GlaxoSmithKline, Janssen, Novartis, Pfizer, Syngenta, Takeda, UCB, and Vertex.
Notes and references
-
(a) E. J. Corey and D. Seebach, Angew. Chem., Int. Ed., 1965, 4, 1075 CrossRef CAS;
(b) E. J. Corey and D. Seebach, J. Org. Chem., 1975, 40, 231 CrossRef;
(c) D. Seebach, Angew. Chem., Int. Ed., 1979, 18, 239 CrossRef.
- For selected reviews for the use of dithianes in complex molecule synthesis see:
(a) M. Yus, C. Nájera and F. Foubelo, Tetrahedron, 2003, 59, 6147 CrossRef CAS;
(b) A. B. Smith III and C. M. Adams, Acc. Chem. Res., 2004, 37, 365 CrossRef PubMed;
(c) T. E. Burghardt, J. Sulfur Chem., 2005, 26, 411 CrossRef CAS.
-
(a) M. Z. Chen and G. C. Micalizio, J. Am. Chem. Soc., 2012, 134, 1352 CrossRef CAS PubMed;
(b) S. E. Denmark and L. R. Cullen, Org. Lett., 2014, 16, 70 CrossRef CAS PubMed;
(c) S. Kyasa and P. H. Dussault, Org. Lett., 2014, 16, 5235 CrossRef CAS PubMed;
(d) W. Du, L. Tian, J. Lai, X. Huo, X. Xie, X. She and S. Tang, Org. Lett., 2014, 16, 2470 CrossRef CAS PubMed;
(e) Y. Wang, Z. Zheng and L. Zhang, Angew. Chem., Int. Ed., 2014, 53, 9572 CrossRef CAS PubMed;
(f) A. Kondoh, M. Oishi, T. Takeda and M. Terada, Angew. Chem., Int. Ed., 2015, 54, 15836 CrossRef CAS PubMed;
(g) K. Yao, D. Liu, Q. Yuan, T. Imamoto, Y. Liu and W. Zhang, Org. Lett., 2016, 18, 6296 CrossRef CAS PubMed;
(h) Y. Liang, J. Lai, T. Liu and S. Tang, Org. Lett., 2016, 18, 5086 CrossRef CAS PubMed.
- S. A. Baker Dockrey, A. K. Makepeace and J. R. Schmink, Org. Lett., 2014, 16, 4730 CrossRef CAS PubMed.
- B. Yucel and P. J. Walsh, Adv. Synth. Catal., 2014, 356, 3659 CrossRef CAS PubMed.
-
(a) A. B. Smith III, S. M. Pitram, A. M. Boldi, M. J. Gaunt, C. Sfouggatakis and W. H. Moser, J. Am. Chem. Soc., 2003, 125, 14435 CrossRef PubMed;
(b) A. B. Smith III and M. Xiang, J. Am. Chem. Soc., 2006, 128, 66 CrossRef PubMed;
(c) B. Melillo and A. B. Smith III, Org. Lett., 2013, 15, 2282 CrossRef CAS PubMed;
(d) M. Z. Chen, O. Gutierrez and A. B. Smith III, Angew. Chem., Int. Ed., 2014, 53, 1279 CrossRef CAS PubMed.
- For selected reviews on the diverse field of transition metal catalysed C–H activation, see:
(a) K. Godula and D. Sames, Science, 2006, 312, 67 CrossRef CAS PubMed;
(b) J. Yang, Org. Biomol. Chem., 2015, 13, 1930 RSC;
(c) H. M. L. Davies and D. Morton, ACS Cent. Sci., 2017, 3, 936 CrossRef CAS PubMed;
(d) P. Gandeepan and L. Ackermann, Chem, 2018, 4, 199 CrossRef CAS;
(e) Y. Park, Y. Kim and S. Chang, Chem. Rev., 2017, 117, 9247 CrossRef CAS PubMed.
- Selected examples:
(a) S. Rakshit, C. Grohmann, T. Besset and F. Glorius, J. Am. Chem. Soc., 2011, 133, 2350 CrossRef CAS PubMed;
(b) Y. Li, B. J. Li, W. H. Wang, W. P. Huang, X. S. Zhang, K. Chen and Z.-J. Shi, Angew. Chem., Int. Ed., 2011, 50, 2115 (
Angew. Chem.
, 2011
, 123
, 2163
) CrossRef CAS PubMed;
(c) K. D. Hesp, R. G. Bergman and J. A. Ellman, J. Am. Chem. Soc., 2011, 133, 11430 CrossRef CAS PubMed;
(d) J. Jayakumar, K. Parthasarathy and C. H. Cheng, Angew.Chem. Int. Ed., 2012, 51, 197 (
Angew. Chem.
, 2012
, 124
, 201
) CrossRef CAS PubMed;
(e) C. Wang, H. Chen, Z. Wang, J. Chen and Y. Huang, Angew. Chem., Int. Ed., 2012, 51, 7242 (
Angew. Chem.
, 2012
, 124
, 7354
) CrossRef CAS PubMed;
(f) M. V. Pham, B. Ye and N. Cramer, Angew.Chem. Int. Ed., 2012, 51, 10610 (
Angew. Chem.
, 2012
, 124
, 10762
) CrossRef CAS PubMed;
(g) X. Tan, B. Liu, X. Li, B. Li, S. Xu, H. Song and B. Wang, J. Am. Chem. Soc., 2012, 134, 16163 CrossRef CAS PubMed;
(h) B. Liu, Y. Fan, Y. Gao, C. Sun, C. Xu and J. Zhu, J. Am. Chem. Soc., 2013, 135, 468 CrossRef CAS PubMed;
(i) G. Liu, Y. Shen, Z. Zhou and X. Lu, Angew. Chem., Int. Ed., 2013, 52, 6033 (
Angew. Chem.
, 2013
, 125
, 6149
) CrossRef CAS PubMed;
(j) Y. Lian, R. G. Bergman, L. D. Lavis and J. A. Ellman, J. Am. Chem. Soc., 2013, 135, 7122 CrossRef CAS PubMed;
(k) Z. Qi and X. Li, Angew. Chem., Int. Ed., 2013, 52, 8995 (
Angew. Chem.
, 2013
, 125
, 9165
) CrossRef CAS PubMed;
(l) D. Zhao, Z. Shi and F. Glorius, Angew. Chem., Int. Ed., 2013, 52, 12426 (
Angew. Chem.
, 2013
, 125
, 12652
) CrossRef CAS PubMed;
(m) Y. Chen, D. Wang, P. Duan, R. Ben, L. Dai, X. Shao, M. Hong, J. Zhao and Y. Huang, Nat. Commun., 2014, 5, 1 Search PubMed;
(n) Y. Fukui, P. Liu, Q. Liu, Z. –T. He, N. –Y. Wu, P. Tian and G. –Q. Lin, J. Am. Chem. Soc., 2014, 136, 15607 CrossRef CAS PubMed;
(o) D.-G. Yu, F. de Azambuja, T. Gensch, C. G. Daniliuc and F. Glorius, Angew. Chem., Int. Ed., 2014, 36, 9650 CrossRef PubMed;
(p) S. R. Neufeldt, G. Jiménez-Osés, J. R. Huckins, O. R. Thiel and K. N. Houk, J. Am. Chem. Soc., 2015, 137, 9843 CrossRef CAS PubMed;
(q) R. Dateer and S. Chang, J. Am. Chem. Soc., 2015, 15, 4908 CrossRef PubMed;
(r) J. Zheng, S.-B. Wang, C. Zheng and S.-L. You, Angew. Chem., Int. Ed., 2017, 16, 4540 CrossRef PubMed;
(s) X. Qi, Y. Li, R. Bai and Y. Lan, Acc. Chem. Res., 2017, 50, 2799 CrossRef CAS PubMed;
(t) S. Satake, T. Kurihara, K. Nishikawa, T. Mochizuki, M. Hatano, K. Ishihara, T. Yoshino and S. Matsunaga, Nature Catalysis, 2018, 1, 585 CrossRef;
(u) Z. Zhang, M. Tang, S. Han, L. Ackermann and J. Li, J. Org. Chem., 2017, 82, 664 CrossRef CAS PubMed;
(v) Y. Wu, Z. Chen, Y. Yang, W. Zhu and B. Zhou, J. Am. Chem. Soc., 2018, 140, 42 CrossRef CAS PubMed.
- For selected examples and reviews, see:
(a) R. Samanta and A. P. Antonchick, Angew. Chem., Int. Ed., 2011, 50, 5217 CrossRef CAS PubMed;
(b) M. Yu, Y. Xie, C. Xie and Y. Zhang, Org. Lett., 2012, 14, 2164 CrossRef CAS PubMed;
(c) T. Wesch, F. R. Leroux and F. Colobert, Adv. Synth. Catal., 2013, 355, 2139 CrossRef CAS;
(d) X.-S. Zhang, Q.-L. Zhu, Y.-F. Zhang, Y.-B. Li and Z.-J. Shi, Chem.–Eur. J., 2013, 19, 11898 CrossRef CAS PubMed;
(e) Y. Yokoyama, Y. Unoh, K. Hirano, T. Satoh and M. Miura, J. Org. Chem., 2014, 16, 7649 CrossRef PubMed;
(f) K. Nobushige, K. Hirano, T. Satoh and M. Miura, Org. Lett., 2014, 16, 1188 CrossRef CAS PubMed;
(g) B. Wang, C. Shen, J. Yao, H. Yin and Y. Zhang, Org. Lett., 2014, 16, 46 CrossRef CAS PubMed;
(h) C. K. Hazra, Q. Dherbassy, J. Welcel-Delord and F. Colobert, Angew. Chem., Int. Ed., 2014, 53, 13871 CrossRef CAS PubMed;
(i) P. Villuendas and E. P. Urriolabeitia, Org. Lett., 2015, 17, 3178 CrossRef CAS PubMed;
(j) J. E. Spangler, Y. Kobayashi, P. Verma, D. –H. Wang and J. –Q. Yu, J. Am. Chem. Soc., 2015, 137, 4534 CrossRef PubMed;
(k) P. Jain, P. Verma, G. Xia and J.-Q. Yu, Nat. Chem., 2017, 9, 140 CrossRef CAS PubMed;
(l) Z.-J. Cai, C.-X. Liu, Q. Gu and S.-L. You, Angew. Chem., Int. Ed., 2018, 57, 1296 CrossRef CAS PubMed;
(m) K.-X. Tang, C.-M. Wang, T.-H. Gao, L. Chen, L. Fan and L.-P. Sun, Adv. Synth. Catal., 2018, 361, 26–38 CrossRef;
(n) S. E. Mann, A. E. Aliev, G. J. Tizzard and T. D. Sheppard, Organometallics, 2011, 30, 1772 CrossRef CAS;
(o) S. R. Yetra, Z. Shen, H. Wang, L. Ackermann and Beilstein, J. Org. Chem., 2018, 14, 1546 CAS.
-
(a) X. Tan, B. Liu, X. Li, B. Li, S. Xu, H. Song and B. Wang, J. Am. Chem. Soc., 2012, 134, 16163 CrossRef CAS PubMed;
(b) S. Kawamorita, T. Miyazaki, T. Iwai, H. Ohmiya and M. Sawamura, J. Am. Chem. Soc., 2012, 134, 12924 CrossRef CAS PubMed;
(c) T. Cochet, V. Bellosta, D. Roche, J. –Y. Ortholand, A. Greiner and J. Cossy, Chem. Commun., 2012, 48, 10745 RSC;
(d) B. Liu, T. Zhou, B. Li, S. Xu, H. Song and B. Wang, Angew. Chem., Int. Ed., 2014, 53, 4191 (
Angew. Chem.
, 2014
, 126
, 4275
) CrossRef CAS PubMed;
(e) N. Wang, R. Li, L. Li, S. Xu, H. Song and B. Wang, J. Org. Chem., 2014, 79, 5379 CrossRef CAS PubMed;
(f) A. Archambeau and T. Rovis, Angew. Chem., Int. Ed., 2015, 54, 13337 CrossRef CAS PubMed;
(g) B. Zhou, Z. Chen, Y. Yang, W. AI, H. Tang, Y. Wu, W. Zhu and Y. Li, Angew. Chem., Int. Ed., 2015, 54, 12121 CrossRef CAS PubMed;
(h) W. Hou, Y. Yang, H. Feng, Y. Li and B. Zhou, Chem. Commun., 2016, 52, 9672 RSC;
(i) C. Yang, M. Zou, J. Liu, X. Wen, X. Sun, Y. Zhang and N. Jiao, Chem.–Eur. J., 2016, 22, 11165 CrossRef PubMed;
(j) B. Liu, P. Hu, X. Zhou, D. Bai, J. Chang and X. Li, Org. Lett., 2017, 19, 2086 CrossRef CAS PubMed.
-
(a) X. Huang, Y. Wang, J. Lan and J. You, Angew. Chem., Int. Ed., 2015, 54, 9404 CrossRef CAS PubMed;
(b) H. Wang, G. Tang and X. Li, Angew. Chem., Int. Ed., 2015, 127, 13241 CrossRef.
- For recent advances in Rh(III) catalyzed activation of unreactive C(sp3)–H bonds, see: C. Yuan, G. Tu and Y. Zhao, Org. Lett., 2017, 19, 356 CrossRef CAS PubMed.
- For previous work from our group on C–H functionalisation, see;
(a) P. W. Tan, A. M. Mak, M. B. Sullivan, D. J. Dixon and J. Seayad, Angew. Chem., Int. Ed., 2017, 56, 16550 CrossRef CAS PubMed;
(b) P. W. Tan, M. Haughey and D. J. Dixon, Chem. Commun., 2015, 51, 4406 RSC.
- Y. Unoh, K. Hirano, T. Satoh and M. Miura, Org. Lett., 2015, 17, 704 CrossRef CAS PubMed.
- For selected use of amino acid derived additives in C–H activation methodologies, see;
(a) G.-J. Cheng, Y.-F. Yang, P. Liu, P. Chen, T.-Yu. Sun, G. Li, X. Zhang, K. N. Houk, J.-Q. Yu and Y.-D. Wu, J. Am. Chem. Soc., 2014, 136, 894 CrossRef CAS PubMed;
(b) K. S. L. Chan, H.-Y. Fu and J.-Q. Yu, J. Am. Chem. Soc., 2015, 137, 2042 CrossRef CAS PubMed;
(c) Y. Zhu, X. Chen, C. Yuan, G. Li, J. Zhang and Y. Zhao, Nat. Chem., 2017, 8, 14904 CAS;
(d) J. J. Gair, B. E. Haines, A. S. Filatov, D. G. Musaev and J. C. Lewis, Chem. Sci., 2017, 8, 5746 RSC;
(e) K. Hong, H. Park and J.-Q. Yu, ACS Catal., 2017, 7, 6938 CrossRef CAS PubMed.
- For a comprehensive review on the use of rings in drug molecules, see: R. D. Taylor, M. MacCoss and A. D. G. Lawson, J. Med. Chem., 2014, 57, 5845 CrossRef CAS PubMed.
- Product 3p was formed as a racemate..
- For a review on the ‘magic methyl’ effect in drug discovery, see: H. Schönherr and T. Cernak, Angew. Chem., Int. Ed., 2013, 52, 12256 CrossRef PubMed.
Footnote |
† Electronic supplementary information (ESI) available: Experimental protocol and spectra data. See DOI: 10.1039/c8sc05225e |
|
This journal is © The Royal Society of Chemistry 2019 |
Click here to see how this site uses Cookies. View our privacy policy here.