DOI:
10.1039/C7QM00125H
(Research Article)
Mater. Chem. Front., 2017,
1, 1829-1835
Lighting up the interactions between bacteria and surfactants with aggregation-induced emission characteristics†
Received
17th March 2017
, Accepted 26th April 2017
First published on 27th April 2017
Abstract
We report a method for imaging and tracking the interactions between bacteria and surfactants by using two ionic surfactants with aggregation-induced emission (AIE) characteristics. The fluorescence of the cationic AIE-active surfactant, tetraphenylethene-dodecyltrimethylammonium bromide (TPE-DTAB), can be turned on in the presence of bacteria, while the anionic AIE-active surfactant, tetraphenylethene-sodium dodecyl sulfonate (TPE-SDS), hardly interacts with bacteria due to electrostatic repulsion. Moreover, TPE-DTAB is applied for imaging its interaction with bacteria. In the fluorescence images, TPE-DTAB with one positively-charged ammonium is firstly attracted to the anionic surfaces of bacteria through electrostatic attraction, and then its long alkyl chain could insert into the bacterial membrane. The disturbing effect of membrane permeability leads to the death of bacteria. Unexpectedly, TPE-DTAB containing a certain amount of TPE-SDS can attain a higher efficiency of killing bacteria. The negative charge of the bacterial membrane has decreased due to the neutralization of TPE-DTAB, making TPE-SDS easier to get close to and react with membrane proteins. The synergistic effect on membrane damage leads to the higher antibacterial efficiency and massive agglomerations of dead bacteria.
1. Introduction
Millions of bacteria live in the human body, and play a significant role in all aspects of human life from the body’s digestive system to healthy living (e.g., food processing, medical hygiene, and environmental protection).1 Although most bacteria are harmless or beneficial to humans, there are still some pathogenic bacteria that cause severe infections to the human body.2–4 Given their inseparable relationship with human health, research on the prevention and control of bacterial infections is attracting increasing attention year by year.5 And a lot of work has been done for developing new antibiotics, which are capable of killing or inhibiting bacterial growth in the treatment of bacterial infections.6,7 It is known that bacteria find their way in through the mouth and skin.8–10 Hence, is it possible that people could prevent harmful bacteria from entering their body before the treatment of bacterial infections using antibiotics? If the household detergents could eliminate bacteria effectively, then pre-control of bacterial hazards could be performed. Surfactants and amphiphilic compounds are the main components of household detergents.11,12 In the traditional view, nonionic and anionic surfactants are used as stabilizers or solubilizers for antibacterial agents,13,14 but cationic surfactants with quaternary ammonium could be the promising biocide candidates because of their close interactions with anionic bacterial surfaces.15–21 In the absence of antibacterial agents, however, the antibacterial effect of mixtures of two amphiphilic compounds is often better than that of the individual component.22,23 Moreover, the synergistic effect produced by mixing anionic and cationic surfactants has also been reported.24,25 To date, a variety of techniques, such as zeta potential analysis,26–28 scanning electron microscopy (SEM),29–31 electron paramagnetic resonance (EPR),32–34etc., have been used in studying the detailed interactions between cationic surfactants and anionic bacterial membranes. However, there is a lack of imaging methods for tracking their interaction processes.
Fluorescence microscopy provides the advantages of high-contrast, non-invasion, targeted labelling and direct observation in the solution state, and has become popular for process imaging and tracking.35–39 In principle, the interaction process of surfactants with bacteria could be seen if the surfactants can emit light under irradiation. With the discovery and development of aggregation-induced emission (AIE) luminogens, our group successively developed two kinds of AIE-active surfactants: anionic tetraphenylethene-sodium dodecyl sulfonate (TPE-SDS) and cationic tetraphenylethene-dodecyltrimethylammonium bromide (TPE-DTAB).40,41 They emit faint fluorescence in their good solvents without aggregates, but fluoresce strongly in the aggregated state with the restriction of intramolecular motion.42–47 These AIE-active surfactants have been successfully employed in the research on the visualization of the interactions between surfactants and polymers, proteins, and inorganic layered materials.41,48,49 Moreover, AIEgens-based imaging has been well developed in biological research.50–52
In this work, cationic TPE-DTAB, anionic TPE-SDS and TPE-DTAB/TPE-SDS systems were co-cultured with Gram-negative bacteria, E. coli, respectively. The fluorescence of cationic TPE-DTAB was turned on after mixing with E. coli while no fluorescence was observed for anionic TPE-SDS. Moreover, the interaction process of cationic TPE-DTAB with E. coli was imaged using a confocal laser scanning microscope (CLSM) (Scheme 1). At the beginning, no fluorescence was given by the non-aggregated TPE-DTAB and living E. coli. As time went on, cationic TPE-DTAB gradually bound with the anionic membrane of E. coli, and the cyan fluorescence could be observed as a result of the aggregation of TPE-DTAB on the bacterial membrane. Then, the hydrophobic chain of TPE-DTAB was intercalated into the lipid hydrophobic layer of E. coli, disrupting the bacterial membrane and causing the death of E. coli. The fluorescence emitted by TPE-DTAB and propidium iodide (PI) was observed simultaneously. Intriguingly, addition of a small amount of TPE-SDS into TPE-DTAB could improve the antibacterial efficiency from 78.6% to 99.4%. It could be due to the enhanced possibility of the interactions between anionic TPE-SDS and the bacterial membrane with decreased surface potential. Furthermore, larger aggregates of bacteria were also observed using the CLSM. This work not only provides a better understanding of the interaction process of surfactants with bacteria, but also helps broaden the horizon of detergent formulations for preventing bacterial hazards.
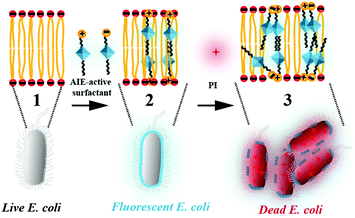 |
| Scheme 1 Schematic illustration of the interactions between the aggregation-induced emission (AIE)-active surfactant and Gram-negative E. coli. | |
2. Results and discussion
Compared with Gram-positive bacteria, Gram-negative bacteria have an outer membrane with barrier function, making them more difficult to be reacted with and killed.53E. coli, a typical example of Gram-negative bacteria, has been well-studied for years.54 To investigate the surfactant’s impact on bacterial behaviour, E. coli is selected to react with anionic TPE-SDS and cationic TPE-DTAB for the evaluation of bacterial viability, respectively. In these experiments, a H2O/dimethyl sulphoxide (DMSO) mixture (2
:
8 v/v) was utilized, in which DMSO can decrease the background fluorescence due to the excellent solubility of TPE-SDS and TPE-DTAB in DMSO. As shown in Fig. 1A, TPE-SDS is negatively charged with a long hydrophobic chain. After being incubated with E. coli for 0.5 h, the fluorescence intensity of TPE-SDS was still very weak and no obvious change was observed. It was mainly attributed to the electrostatic repulsion between anionic TPE-SDS and the negatively-charged membrane of E. coli, which was further confirmed by the photographs taken under a 365 nm ultraviolet lamp. This result demonstrated that the anionic surfactant was indeed hard to interact with bacteria. Apart from the opposite charges, TPE-DTAB has a structure similar to TPE-SDS (Fig. 1B). Under the same experimental conditions, the fluorescence intensity of TPE-DTAB intensely increased, indicating the interaction between TPE-DTAB and E. coli. Cationic TPE-DTAB could bind with the negatively-charged membrane of E. coli and aggregate on the membrane to induce strong fluorescence emission. The photographs taken under an ultraviolet lamp also showed the bright cyan emission of the mixtures of TPE-DTAB and E. coli. The fluorescence turn-on phenomenon of TPE-DTAB in the presence of bacteria not only provides evidence for the interaction between the cationic surfactant and bacteria but also enables a further visual investigation on the interaction process.
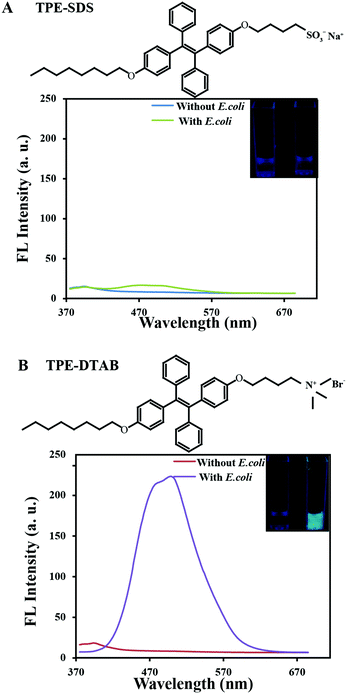 |
| Fig. 1 (A) Chemical structure of TPE-SDS and fluorescence spectra of H2O/DMSO (2 : 8, v/v) solution of TPE-SDS in the presence (green line) and absence (blue line) of E. coli. Inset: Photographs of H2O/DMSO (2 : 8, v/v) solution of TPE-SDS with/without 108 (colony forming units) CFU mL−1 of E. coli taken under 365 nm UV irradiation; (B) chemical structure of TPE-DTAB and the fluorescence spectra of H2O/DMSO (2 : 8, v/v) solution of TPE-DTAB in the presence (purple line) and absence (red line) of E. coli. Inset: Photographs of H2O/DMSO (2 : 8, v/v) solution of TPE-DTAB with/without 108 CFU mL−1 of E. coli taken under 365 nm UV irradiation. Excitation wavelength, 350 nm; PMT voltage, 400 V; slit, 5 nm. | |
In order to avoid E. coli damage from DMSO, all of the experiments on the antimicrobial action on E. coli were performed in DMSO–DMEM medium (2
:
98, v/v).55 Moreover, TPE-SDS or TPE-DTAB in DMSO–DMEM medium (2
:
98, v/v) has only a very weak fluorescence signal (Fig. S1, ESI†), and will not interfere with the imaging analysis of the interactions between bacteria and surfactants in the confocal microscope system. The interaction processes of anionic TPE-SDS and cationic TPE-DTAB with E. coli were visualized by the CLSM in the presence of PI. PI is a frequently-used fluorescence turn-on probe (red emission) to identify dead cells, which can bind with nucleic acid after entrance into the cells with a broken membrane.56Fig. 2A–C shows the fluorescence images of the mixtures of TPE-SDS and E. coli in the presence of PI at different times. Only a few red light-emitting dots appeared, indicating that TPE-SDS has a small impact on the membrane permeability of E. coli. In other words, most of the anionic TPE-SDS molecules cannot get close to the negatively-charged membrane of E. coli due to the electrostatic repulsion, resulting in the absence of turned-on cyan fluorescence and a poor bactericidal effect. This conclusion is consistent with that observed from the fluorescence spectra. Under the same experimental conditions, cationic TPE-DTAB was incubated with E. coli in the presence of PI (Fig. 2D–F). At the beginning, no fluorescence was observed from the non-aggregated TPE-DTAB and living E. coli (Fig. 2D). After being incubated for 10 min, glaring cyan fluorescence of TPE-DTAB was turned on as shown in Fig. 2E, suggesting that the cationic TPE-DTAB molecules bound with the membrane of E. coli. Since the red emission of PI did not appear, it could be deduced that the bacterial membrane integrity was not disrupted. Subsequently, both the cyan and red fluorescence were observed in Fig. 2F. This result demonstrated that the absorbed TPE-DTAB on the bacterial surfaces could further intercalate into the hydrophobic domain of the bacterial membrane (mainly through the hydrophobic effect) to disrupt the integrity of the bacterial membrane, leading to the loss of barrier function of the cell membrane and bacterial death. Thus, with the aid of the AIE-active surfactant, visual investigation of bacterial membrane interactions and antibacterial actions of the surfactants could be easily achieved.
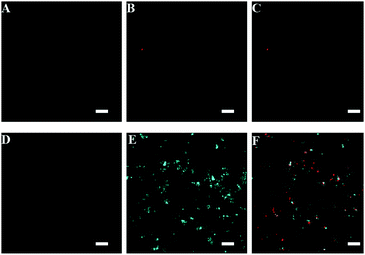 |
| Fig. 2 Fluorescence images of the mixtures of TPE-SDS and E. coli in the presence of PI at different times: (A) 0 min, (B) 10 min, and (C) 30 min; fluorescence images of the mixtures of TPE-DTAB and E. coli in the presence of PI at different times: (D) 0 min, (E) 10 min, and (F) 30 min. All of the fluorescence images were taken using a 405 nm laser. Scale bar: 10 μm. | |
To verify the bactericidal effect of TPE-SDS and TPE-DTAB, the images of Luria-Bertani (LB) agar plates were taken, and the colonies on each plate were counted for the quantification of the bactericidal effect on E. coli (Fig. 3). In the control group, the bacteria grew healthily without the addition of TPE-DTAB/TPE-SDS (Fig. 3A). With TPE-SDS alone, there is no obvious effect on bacteria viability (Fig. 3B). It has similar size and number of colonies to the control group, and only 3.8% of E. coli was killed (Fig. 3G). However, 78.6% of E. coli was killed on the plate after treatment with TPE-DTAB alone (Fig. 3F and G), suggesting the good killing effect of TPE-DTAB on E. coli. These results were entirely consistent with those of the fluorescence photographs. Surprisingly, 99.4% of E. coli was killed by using TPE-DTAB/TPE-SDS mixtures with a ratio of 5
:
1 (Fig. 3E and G). Its cytotoxicity to human cells was evaluated in a cell counting kit-8 (CCK-8) assay. As shown in Fig. S2 (ESI†), compared with the untreated cells, the cells treated with the TPE-DTAB/TPE-SDS mixture have lower cell activity (∼60%). The results fall in line with the previous findings that surfactants are toxic to human cells, since human cell membranes could be damaged by the surfactants in the same way as bacterial cell membranes.29 More bacterial viability assays were carried out to examine the antibacterial activity of TPE-DTAB/TPE-SDS mixtures with different ratios. A decreased amount of E. coli was observed after treatment with TPE-DTAB/TPE-SDS mixtures with a ratio of 1
:
9 (Fig. 3C), implying that just a small amount of TPE-DTAB could improve the killing efficiency of TPE-SDS apparently. Moreover, when the ratio of TPE-DTAB/TPE-SDS increased to 1.5
:
1 (Fig. 3D), the antibacterial activity (88.7%, Fig. 3G) was even higher than that of TPE-DTAB alone, further indicating the existence of the synergistic effect between TPE-DTAB and TPE-SDS for killing bacteria. All these results prove that anionic TPE-SDS alone cannot kill bacteria, but cationic TPE-DTAB together with anionic TPE-SDS can kill bacteria more efficiently.
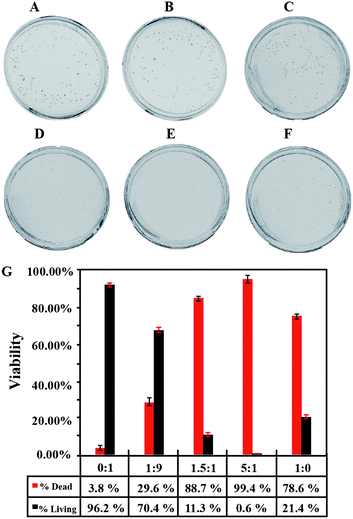 |
| Fig. 3 LB agar plates of E. coli (A) in the absence and presence of TPE-DTAB/TPE-SDS mixtures at different ratios: (B) 0 : 1, (C) 1 : 9, (D) 1.5 : 1, (E) 5 : 1, and (F) 1 : 0 for 0.5 h. (G) Viability of E. coli after 0.5 h exposure to TPE-DTAB/TPE-SDS mixtures with different ratios. | |
The excellent performance of TPE-DTAB/TPE-SDS mixtures for antibacterial activity encouraged us to further elucidate the detailed mechanism during the antibacterial process. Microscopic characterization studies via CLSM were carried out to gain insight into the interactions between TPE-DTAB/TPE-SDS mixtures and bacteria. Different ratios of TPE-DTAB/TPE-SDS mixtures and PI were incubated with E. coli for 30 min at 37 °C, and then directly visualized using the CLSM without any washing procedure. It was worth noting that with the help of DMSO, TPE-DTAB or TPE-SDS emitted more weakly in the solution than on the bacterial surfaces. As shown in Fig. 4A, in the presence of the TPE-DTAB/TPE-SDS complex with a ratio of 1
:
9, a few bacterial agglomerations appeared and emitted intense cyan light and weak red light. Both the stain effect and killing effect are relatively poor. With an increase in the synergistic effect of TPE-DTAB and TPE-SDS (Fig. 4B), some bigger agglomerations were observed with cyan and red fluorescence, implying relatively good stain and killing effects for bacteria. And when the ratio of the TPE-DTAB/TPE-SDS complex reached 5
:
1, there were massive agglomerations of bacteria with bright cyan and red emission in the confocal image (Fig. 4C). Taking advantage of the laser beam Z-scan technique, three-dimensional (3D) visualization of these luminescent agglomerations was also achieved. Fig. S3A–C (ESI†) shows the 3D images of bacterial agglomerations stained by the TPE-DTAB/TPE-SDS complex (cyan channel), PI (red channel) and the overlay image, respectively. However, as the ratio of TPE-DTAB/TPE-SDS continued to increase up to 1
:
0 (Fig. 4D), the size of agglomerations became smaller instead of larger. In combination with the results from LB agar plates, it can be concluded that there is a strong correlation between bacterial death and the size of bacterial agglomerations.57–59 Moreover, larger size of bacterial agglomerations might mean higher antibacterial efficiency.
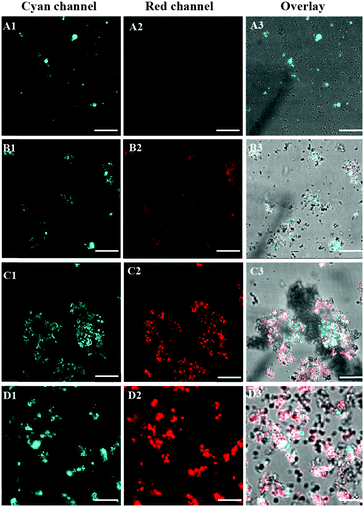 |
| Fig. 4 Fluorescence images of E. coli in the presence of PI and TPE-DTAB/TPE-SDS mixtures with different ratios: 1 : 9 (A1–A3), 1 : 1.5 (B1–B3), 5 : 1 (C1–C3) and 1 : 0 (D1–D3) for 0.5 h. Scale bar: 20 μm. Excitation wavelengths for TPE-DTAB/TPE-SDS mixtures and PI are 405 nm and 543 nm, respectively. | |
For a better understanding of the agglomeration mechanism, the ξ potential value change of the bacterial surface was also studied. It is known that when the absolute ξ potential value exceeds 30 mV, the colloidal system is stable without large agglomerations. The bacterial cell membrane is composed of lipids with a negative charge. And the adsorption of ionic surfactants on the cell membrane usually has a great impact on the ξ potential value of bacteria. Thus, we measured the ξ potential value of E. coli, TPE-DTAB/TPE-SDS complexes, and their mixtures, respectively (Fig. S4, ESI†). As expected, the ξ potential value of E. coli is negative (−42 mV). For the TPE-DTAB/TPE-SDS complexes, as the ratio of cationic TPE-DTAB and anionic TPE-SDS increased to above 1
:
1, the measured ξ potential value became positive. When E. coli was mixed with the negatively-charged TPE-DTAB/TPE-SDS complexes, the values of the ξ potential remained constant compared with that of E. coli alone. However, when the TPE-DTAB/TPE-SDS complexes had a positive charge, the ξ potential value of E. coli increased, which was higher than that of E. coli alone. Moreover, it was found that the sample composition with the lowest absolute ξ potential value just corresponded to the ratio of the highest killing efficiency to maximal agglomerations (5
:
1). The decreased negative charge of E. coli made the E. coli unstable. And it is easier for anionic TPE-SDS to get close to the E. coli surface and interact with the E. coli cell membrane through its hydrophobic part. The changes in the membrane morphology of E. coli cells were further investigated using SEM (Fig. S5, ESI†). In comparison with the untreated bacteria, the bacteria treated with TPE-DTAB/TPE-SDS (5
:
1) showed irregular shapes along with an extensively damaged membrane. This result confirmed the strong interaction of the TPE-DTAB/TPE-SDS mixture with the bacterial membrane. All of these findings can induce larger bacterial agglomerations and higher antibacterial efficiency.
3. Experimental section
Preparation of TPE-DTAB/TPE-SDS systems
TPE-SDS and TPE-DTAB were previously designed and synthesized by our group.40,41 The stock solutions of TPE-SDS (800 μM) and TPE-DTAB (800 μM) were prepared using DMSO as solvent. Then, the stock solution of TPE-SDS and TPE-DTAB was diluted and mixed to obtain different molar ratios of TPE-DTAB/TPE-SDS systems.
Characteristics of the effect of different TPE-DTAB/TPE-SDS systems on bacteria
The ξ potential values of TPE-DTAB/TPE-SDS mixtures with different ratios (0
:
1, 1
:
9, 1.5
:
1, 5
:
1, 1
:
0) before and after being incubated with E. coli were obtained using a Malvern Zetasizer 3000HS instrument. Untreated E. coli was also incubated under the same conditions as the control.
Fluorescence spectra were recorded using an F-7000 fluorescence spectrophotometer (Hitachi, Japan) with a slit of 5.0 nm and at a scanning rate of 1200 nm min−1.
3D fluorescence images were recorded on a confocal laser scanning microscope (Leica, TCS SP8).
Culture conditions for bacteria
LB broth consists of 10 g of tryptone, 5 g of yeast extract, and 10 g of NaCl in 1000 mL of sterile distilled water (pH 7.0). 40 μL of glycerol bacterial stocks were added to 4 mL of LB broth, and the bacteria were cultured at 37 °C on a shaker bed at 200 rpm for 10 h prior to the experiments.
Fluorescence assay
1 mL of bacterial suspension was centrifuged at 8000 r min−1 for 3 minutes, and the precipitate was mixed with 1 mL of TPE-DTAB/TPE-SDS mixtures. After vortex mixing, the mixtures of E. coli with different TPE-DTAB/TPE-SDS systems were incubated at 37 °C on a shaker bed at 200 rpm for 30 min. Untreated bacterial suspension (1 mL) served as the control. These samples were placed in confocal disks for fluorescence assay. All the images were obtained with an oil immersion 100× objective under a 405 nm laser.
Biocidal testing
The TPE-DTAB/TPE-SDS systems and E. coli mixtures were incubated for 0.5 h. 50 μL diluted sample solution was spread onto nutrient agar plates. The plates were incubated at 37 °C for 12 h, colonies were counted, and viabilities were calculated relative to the untreated control.
Scanning electron microscopy (SEM)
The untreated and treated E. coli were deposited onto a clean silicon wafer, respectively. After being dried, the E. coli cells were fixed in 1% glutaraldehyde PBS solution for 3 h and washed with 0.15% glutaraldehyde PBS solution. The E. coli cells were then dehydrated every 5 min sequentially with 10%, 30%, 50%, 70%, 90%, and 100% ethanol (twice). Finally, the prepared E. coli were dried, sputter coated, and observed using a Hitachi S-4700 SEM.
Cytotoxicity assay
HeLa cells were seeded into 96-well culture plates (104 cells per well) at 37 °C for 24 h. TPE-DTAB/TPE-SDS (5
:
1) was diluted with DMEM and added to 96-well culture plates, and then incubated at 37 °C for 3 h. 10 μL CCK-8 solution was added to each well. After being incubated for 1 h, the absorbance at 450 nm was recorded using a multimode plate reader in quadruplicate. The cell viability values were determined (four times) according to the following formula: cell viability (%) = A/A0 × 100%, where A and A0 are the average absorbances of the treated wells with samples and untreated wells, respectively.
4. Conclusions
In summary, the interaction process of TPE-DTAB and TPE-SDS with E. coli has been directly visualized owing to their AIE characteristics. The cationic ammonium group of TPE-DTAB can be attracted to the negatively charged surfaces of E. coli, and the cyan fluorescence of TPE-DTAB was turned on due to the restriction of intramolecular motions. Subsequently, the hydrophobic chains of TPE-DTAB can insert into the bacterial membrane, resulting in membrane damage and death of E. coli. Interestingly, although anionic TPE-SDS hardly interacts with bacteria due to electrostatic repulsion, its antibacterial activity can be opened up with the aid of TPE-DTAB. Compared with TPE-SDS alone, the TPE-DTAB/TPE-SDS systems are able to neutralize the negative charge of the bacterial membrane by TPE-DTAB, leading to further interaction of TPE-SDS with membrane proteins. The synergistic effect of TPE-DTAB and TPE-SDS with an optimal ratio of 5
:
1 leads to the higher antibacterial efficiency and massive agglomerations of dead bacteria. These imaging results are further verified by ξ potential analyses and the plate count method. Therefore, the imaging method may contribute to the fundamental research of the antibacterial mechanism of surfactants, and the development of new household detergents with higher antibacterial efficiency.
Acknowledgements
This work was supported by the National Basic Research Program of China (973 Program, 2014CB932103), the National Natural Science Foundation of China (21375006, 21656001, 21521005 and 21575010), the Innovation and Promotion Project of Beijing University of Chemical Technology, and the China Postdoctoral Science Foundation (2016M600031).
Notes and references
- D. Ivnitski, I. Abdel-Hamid, P. Atanasov and E. Wilkins, Biosens. Bioelectron., 1999, 14, 599–624 CrossRef CAS.
- M. E. Brecher and S. N. Hay, Clin. Microbiol. Rev., 2005, 18, 195–204 CrossRef PubMed.
- P. R. Shorten, A. B. Pleasants and T. K. Soboleva, Int. J. Food Microbiol., 2006, 108, 369–375 CAS.
-
T. E. Ford and R. R. Colwell, American Academy of Microbiology, DC, Washington, 1996 Search PubMed.
- L. Cegelski, G. R. Marshall, G. R. Eldridge and S. J. Hultgren, Nat. Rev. Microbiol., 2008, 6, 17–27 CrossRef CAS PubMed.
- K. Rasool, M. Helal, A. Ali, C. E. Ren, Y. Gogotsi and K. A. Mahmoud, ACS Nano, 2016, 10, 3674–3684 CrossRef CAS PubMed.
- Y. Y. Zhao, Y. Tian, Y. Cui, W. W. Liu, W. S. Ma and X. Y. Jiang, J. Am. Chem. Soc., 2010, 132, 12349–12356 CrossRef CAS PubMed.
- V. Nizet, T. Ohtake, X. Lauth, J. Trowbridge, J. Rudisill, R. A. Dorschner, V. Pestonjamasp, J. Piraino, K. Huttner and R. L. Gallo, Nature, 2001, 414, 454–457 CrossRef CAS PubMed.
- P. H. A. Lee, T. Ohtake, M. Zaiou, M. Murakami, J. A. Rudisill, K. H. Lin and R. L. Gallo, Proc. Natl. Acad. Sci. U. S. A., 2005, 102, 3750–3755 CrossRef CAS PubMed.
- V. Anesti, I. R. McDonald, M. Ramaswamy, W. G. Wade, D. P. Kelly and A. P. Wood, Environ. Microbiol., 2005, 7, 1227–1238 CrossRef CAS PubMed.
- F. Piérart, J. H. Wildhaber, I. Vrancken, S. G. Devadason and P. N. L. Souëf, Eur. Respir. J., 1999, 13, 673–678 CrossRef.
- T. Madsen, H. B. Boyd, D. Nylén, A. R. Pedersen, G. I. Petersen and F. Simonsen, Proj. Rep., 2001, 615, 221–461 Search PubMed.
- E. H. Hill, D. G. Evans and D. G. Whitten, Langmuir, 2013, 29, 9712–9720 CrossRef CAS PubMed.
- E. H. Hill, H. C. Pappas and D. G. Whitten, Langmuir, 2014, 30, 5052–5056 CrossRef CAS PubMed.
- M. Simões, M. O. Pereira and M. J. Vieira, Water Res., 2005, 39, 478–486 CrossRef PubMed.
- C. C. Zhou, F. G. Wang, H. Chen, M. Li, F. L. Qiao, Z. Liu, Y. B. Hou, C. X. Wu, Y. X. Fan, L. B. Liu, S. Wang and Y. L. Wang, ACS Appl. Mater. Interfaces, 2016, 8, 4242–4249 CAS.
- C. C. Zhou, D. Wang, M. W. Cao, Y. Chen, Z. Liu, C. X. Wu, W. H. Xu, S. Wangand and Y. L. Wang, ACS Appl. Mater. Interfaces, 2016, 8, 30811–30823 CAS.
- K. Nakata, T. Tsuchido and Y. Matsumura, J. Appl. Microbiol., 2011, 110, 568–579 CrossRef CAS PubMed.
- J. Haldar, P. Kondaiah and S. Bhattacharya, J. Med. Chem., 2005, 48, 3823–3831 CrossRef CAS PubMed.
- G. Viscardi, P. Quagliotto, C. Barolo, P. Savarino, E. Barni and E. Fisicaro, J. Org. Chem., 2000, 65, 8197–8203 CrossRef CAS PubMed.
- J. Hoque, P. Akkapeddi, V. Yarlagadda, D. S. S. M. Uppu, P. Kumar and J. Haldar, Langmuir, 2012, 28, 12225–12234 CrossRef CAS PubMed.
- P. Bruheim, H. Bredholt and K. Eimhjellen, Appl. Environ. Microbiol., 1999, 65, 1658–1661 CAS.
- C. F. Xing, Q. L. Xu, H. W. Tang, L. B. Liu and S. Wang, J. Am. Chem. Soc., 2009, 131, 13117–13124 CrossRef CAS PubMed.
-
R. M. Hill, Mixed Surfactant Systems, Marcel Dekker, New York, 1993 Search PubMed.
-
M. J. Rosen, Surfactants and Interfacial Phenomena, Wiley, New York, 1989 Search PubMed.
- K. A. Soni, A. K. Balasubramanian, A. Beskok and S. D. Pillai, Curr. Microbiol., 2008, 56, 93–97 CrossRef CAS PubMed.
- E. Kłodzińska, M. Szumski, E. Dziubakiewicz, K. Hrynkiewicz, E. Skwarek, W. Janusz and B. Buszewski, Electrophoresis, 2010, 31, 1590–1596 CrossRef PubMed.
- R. E. Martinez, O. S. Pokrovsky, J. Schott and E. H. Oelkers, J. Colloid Interface Sci., 2008, 323, 317–325 CrossRef CAS PubMed.
- S. S. Zhang, S. P. Ding, J. Yu, X. R. Chen, Q. F. Lei and W. J. Fang, Langmuir, 2015, 31, 12161–12169 CrossRef CAS PubMed.
- H. W. Gu, P. L. Ho, K. W. T. Tsang, L. Wang and B. Xu, J. Am. Chem. Soc., 2003, 125, 15702–15703 CrossRef CAS PubMed.
- I. Sondi and B. Salopek-Sondi, J. Colloid Interface Sci., 2004, 275, 177–182 CrossRef CAS PubMed.
- W. Nitschke, U. Feiler and A. W. Rutherford, Biochemistry, 1990, 29, 3834–3842 CrossRef CAS PubMed.
- R. E. Glover, R. R. Smith, M. V. Jones, S. K. Jackson and C. C. Rowlands, FEMS Microbiol. Lett., 1999, 177, 57–62 CrossRef CAS PubMed.
- J. B. Lynch, C. Juarez-Garciall, E. Munckll and L. Que, Jr, J. Biol. Chem., 1989, 264, 8091–8096 CAS.
- T. A. Klar, S. Jakobs, M. Dyba, A. Egner and S. W. Hell, Proc. Natl. Acad. Sci. U. S. A., 2000, 97, 8206–8210 CrossRef CAS.
- M. Martineau, T. Galli, G. Baux and J. Mothet, Glia, 2008, 56, 1271–1284 CrossRef PubMed.
- Z. K. Wang, S. J. Chen, J. W. Y. Lam, W. Qin, R. T. K. Kwok, N. Xie, Q. L. Hu and B. Z. Tang, J. Am. Chem. Soc., 2013, 135, 8238–8245 CrossRef CAS PubMed.
- X. Michalet, F. F. Pinaud, L. A. Bentolila, J. M. Tsay, S. Doose, J. J. Li, G. Sundaresan, A. M. Wu, S. S. Gambhir and S. Weiss, Science, 2005, 307, 538–544 CrossRef CAS PubMed.
- I. F. Sbalzarini and P. Koumoutsakos, J. Struct. Biol., 2005, 151, 182–195 CrossRef CAS PubMed.
- W. J. Guan, W. J. Zhou, C. Lu and B. Z. Tang, Angew. Chem., Int. Ed., 2015, 54, 15160–15164 CrossRef CAS PubMed.
- W. J. Guan, S. Wang, C. Lu and B. Z. Tang, Nat. Commun., 2016, 7, 11811–11818 CrossRef PubMed.
- Y. Q. Dong, J. W. Y. Lam and B. Z. Tang, J. Phys.
Chem. Lett., 2015, 6, 3429–3436 CAS.
- Z. J. Zhao, T. X. Chen, S. T. Jiang, Z. P. Liu, D. C. Fang and Y. Q. Dong, J. Mater. Chem. C, 2016, 4, 4800–4804 RSC.
- J. Yang, L. Li, Y. Yu, Z. C. Ren, Q. Peng, S. H. Ye, Q. Q. Li and Z. Li, Mater. Chem. Front., 2017, 1, 91–99 RSC.
- J. Yang, Z. C. Ren, Z. L. Xie, Y. J. Liu, C. Wang, Y. J. Xie, Q. Peng, B. Xu, W. J. Tian, F. Zhang, Z. G. Chi, Q. Q. Li and Z. Li, Angew. Chem., Int. Ed., 2017, 56, 880–884 CrossRef CAS PubMed.
- Z. Y. Wang, W. Bai, J. Q. Tong, Y. J. Wang, A. J. Qin, J. Z. Sun and B. Z. Tang, Chem. Commun., 2016, 52, 10365–10368 RSC.
- T. Hu, B. C. Yao, X. J. Chen, W. Z. Li, Z. G. Song, A. J. Qin, J. Z. Sun and B. Z. Tang, Chem. Commun., 2015, 51, 8849–8852 RSC.
- L. L. Jiao, L. J. Zhang, W. J. Guan and C. Lu, RSC Adv., 2016, 6, 88954–88958 RSC.
- Z. Li, W. J. Guan, C. Lu, X. R. Zhou, S. Z. Luo, Y. You and J. Ouyang, Chem. Commun., 2016, 52, 2807–2810 RSC.
- G. C. Yu, T. R. Cook, Y. Li, X. Z. Yan, D. Wu, L. Shao, J. Shen, G. P. Tang, F. H. Huang, X. Y. Chen and P. J. Stang, Proc. Natl. Acad. Sci. U. S. A., 2016, 113, 13720–13725 CrossRef CAS PubMed.
- G. C. Yu, G. P. Tang and F. H. Huang, J. Mater. Chem. C, 2014, 2, 6609–6617 RSC.
- G. C. Yu, D. Wu, Y. Li, Z. H. Zhang, L. Shao, J. Zhou, Q. L. Hu, G. P. Tang and F. H. Huang, Chem. Sci., 2016, 7, 3017–3024 RSC.
- S. J. Singer and G. L. Nicolson, Science, 1972, 175, 720–731 CAS.
- E. G. Zhao, Y. N. Hong, S. J. Chen, C. W. T. Leung, C. Y. K. Chan, R. T. K. Kwok, J. W. Y. Lam and B. Z. Tang, Adv. Healthcare Mater., 2014, 3, 88–96 CrossRef CAS PubMed.
- C. Friend, W. Scher, J. G. Holland and T. Sato, Proc. Natl. Acad. Sci. U. S. A., 1971, 68, 378–382 CrossRef CAS.
- E. G. Zhao, Y. L. Chen, H. Wang, S. J. Chen, J. W. Y. Lam, C. W. T. Leung, Y. N. Hong and B. Z. Tang, ACS Appl. Mater. Interfaces, 2015, 7, 7180–7188 CAS.
- P. L. Busch and W. Stumm, Environ. Sci. Technol., 1968, 2, 49–53 CrossRef CAS.
- M. B. Miller and B. L. Bassler, Annu. Rev. Microbiol., 2001, 55, 165–199 CrossRef CAS PubMed.
- H. X. Wu, A. Kuzmenko, S. J. Wan, L. Schaffer, A. Weiss, J. H. Fisher, K. S. Kim and F. X. McCormack, J. Clin. Invest., 2003, 111, 1589–1602 CrossRef CAS PubMed.
Footnote |
† Electronic supplementary information (ESI) available. See DOI: 10.1039/c7qm00125h |
|
This journal is © the Partner Organisations 2017 |
Click here to see how this site uses Cookies. View our privacy policy here.