DOI:
10.1039/D2SU00130F
(Paper)
RSC Sustain., 2023,
1, 584-591
Formulation of biobased soap gels from waste-derived feedstocks†
Received
12th December 2022
, Accepted 7th March 2023
First published on 8th March 2023
Abstract
Cooking fats and oils changes their material properties as they heat up and undergo hydrolysis, oxidation, and polymerization. Biobased soft materials including soap may be formulated using waste fats and oils. Gel formulations facilitate portioning and storage, do not spill, and their phase may be controlled using shear forces and heat. To evaluate waste lipids as replacements for edible or petroleum-derived soap feedstocks, cooked soybean oil and bacon fat are saponified and formulated into soaps and gels. To understand how cooked fats and oils and their sources impact soap solutions, viscometry and spectroscopy clarify how waste-derived products can be classified based on their material properties. Here, liquid soap mixtures made from waste lipids generate shear-thickening mixtures in contrast to those made from fresh oils which form shear-thinning mixtures. Gellants from renewable sources are screened for their compatibility with soap solutions and gel formulations are evaluated using infrared spectroscopy and melting points to evaluate the relationship between composition and function. A base soap gel formulation of saponified oil or fat, water, glycerol, and gelatin is described. Studying the design of biobased materials from waste resources allows future formulations to divert waste from drains and landfills to create functional biobased soft materials.
Sustainability spotlight
This work highlights strategies for upgrading household and commercial waste cooking oils and fats into biobased soap gels to prevent their improper disposal and mitigate their environmental impacts. It is important to create alternative strategies to waste oil valorization as current efforts to create biodiesel fuels contribute carbon to the atmosphere. This work describes formulations made of waste oils and fats with material properties comparable to those of available consumer products. It also evaluates the inclusion of waste feedstocks in a quantitative manner, similar to using the green metric of atom economy. This strategy focuses on diverting waste from drains and landfills to protect life on land and below water (U.N. S.D.G.s #14 and #15).
|
Introduction
Food waste is partially composed of fats and oils which pose a threat to municipal infrastructure due to their hydrophobicity and viscosity.1–3 Biodiesel production is one viable way to upgrade waste oils and fats and divert them from reaching drains and landfills.4–6 One disadvantage of a fuel product is that when combusted, it releases carbon dioxide, carbon monoxide, and particulate matter impacting the Earth's climate.7 A biomimetic application for upgraded waste oils and fats is to keep them as reservoirs of carbon in consumer products made of soft materials.8,9 This will help reduce the impacts of waste oils and fats on life on land and life below water, addressing the United Nations Sustainable Development Goals #14 and #15 respectively.3,7
Waste fats and oils contain carbon from renewable resources and are primarily composed of an array of triglycerides consisting of fatty acids on a glycerol backbone.3,8,9 The composition of these triglycerides varies based on the source of the oil or fat. Coconut oils possess more saturated medium-chain fatty acids, while soybean oils possess different unsaturated long-chain fatty acids.10 The composition of the oil or fat will impact the products derived from biobased feedstocks. Feedstocks may be selected based on how component triglycerides transform during cooking, saponification, and gelation.9,11
The composition and physical properties of fats and oils change after heating and cooking.12 While frying oil is often recycled, the saponification of cooked oils is a suitable process for making soaps from certain types of oils and fats.13,14 By comparing the makeup and properties of materials derived from common and commercially available fresh and cooked waste oils and fats we may better understand how waste resources can be used as feedstocks for commercial products.15–17
Infrared spectroscopy (IR) is used to evaluate the chemical makeup of soap mixtures while viscometry is used to evaluate the mixtures' responses to shear forces.18,19 To complement these methods, foamability, the capacity of a solution to form a metastable mixture with gas, is determined to help identify potential applications for different biobased soap mixtures in commercial and industrial settings such as dish soaps.20,21 Despite the utility of liquid soaps, their fluidity makes them prone to spilling and results in their being difficult to portion and store. To address these challenges liquids are thickened or solidified into gels.22,23
Gels are viscoelastic mixtures with properties of both liquids and solids.24–26 To form a gel, rheology modifiers or gellants can be added to liquid solutions. There are many different types of gellants, though abundant, natural, and renewable options include food-grade animal- and plant-derived gellants.27,28 Proteinaceous animal-derived gellants such as gelatin are derived from collagen, whereas many plant-based gellants are different natural and modified polysaccharides.24 Melting point ranges are used to characterize the thermal gel to solution transition.29 A melting point near body temperature gives end-users the ability to predictably control the phase of the gel.30 An ideal soap formulation might include a gel that is stable at room temperature, but one that liquefies with warm water, one's body temperature, or mild shear forces.
The environmental factor or E-factor is used to evaluate the material efficiency of a process or chemical transformation. It is determined by taking the ratio of the mass of the total final waste generated to the mass of the desired product.31 A low E-factor can indicate an efficient process. To evaluate the inclusion of waste-derived feedstocks in soaps and gels, a complementary metric, the percentage of waste incorporated in a product is used to compare waste-derived products. This is derived from a ratio of the mass of waste included in the product to the mass of the entire product (ESI eqn (1) and (2)).† Here, higher values up to 100% indicate that a product is primarily composed of waste-derived resources.
To evaluate the viability of waste lipids as feedstocks for biobased soaps and gels, several fresh and waste fats and oils are saponified and gelled to determine the impacts of lipid sources and cooking on the output products (Fig. 1). By understanding the types of materials that can be derived from biobased waste feedstocks we may divert waste from drains and landfills, store carbon in functional materials, and learn more about waste and waste-derived resources.32,33
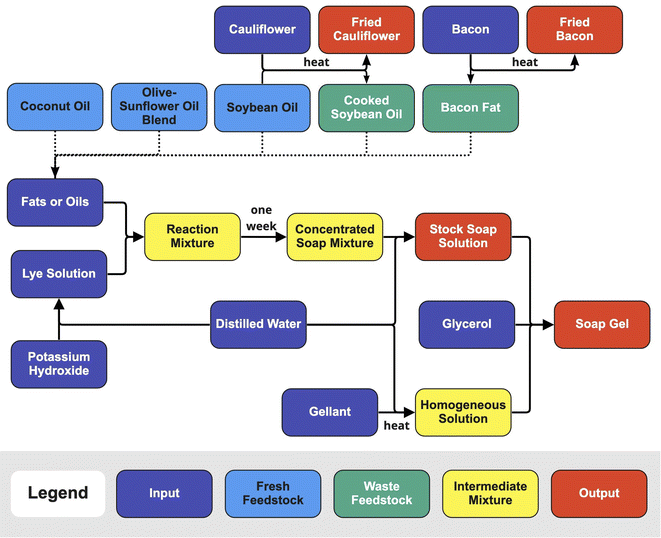 |
| Fig. 1 Process flow diagrams for generating waste lipid feedstocks saponification and gel formulation. | |
Experimental section
Materials used and methods for viscometry, infrared spectroscopy, and melting point range determinations are included in the ESI.†
Frying cauliflower and bacon
Soybean oil (1 L) is heated to 180 °C for 45 minutes and used to fry 500 grams of cauliflower. Separately, 500 grams of bacon are cooked at 180 °C for eight minutes. The oil and fat from each process are collected and filtered while liquid through a fine-mesh strainer.
Saponification of fresh and waste oils or fats
Oils or fats (200 g) were weighed into a 600 mL beaker. The relative amounts of potassium hydroxide (KOH) base for the coconut oil, blend oil (sunflower and olive oils), soybean oil and bacon fat were 250, 190, 190, and 200 mg KOH per gram of oil or fat respectively. 140 mg of sodium hydroxide (NaOH) per gram of blend oil was used.34 The KOH or NaOH base was slowly added in portions to 100 mL of water in a beaker while stirring. Caution was taken not to let the water overheat or evaporate upon the addition of the base. The clear basic solution is added dropwise to the stirred oil or fat. The reaction mixture was left for one week rotating in a sealed plastic bottle on a 764AVM U.S. Stoneware jar mill at 50% speed to promote mixing and allow for saponification to occur affording a foamy liquid (KOH) or solid (NaOH) concentrated soap.
Foamability of soap solutions
Foamability was evaluated using a Dual Range Pulse Matic 12 Osterizer by blending soap solutions and recording the volume of the resulting foam in liters. Soap solutions (100 mL) were blended with the ‘mix’ setting for two minutes. Blending was stopped and the foam volume (L) was recorded. The volume of the foam was recorded again after one week.
Stock soap and soap gel formulation
Concentrated liquid and solid soaps (125 g) were diluted with 210 g of water to afford stock soap solutions. Gellants were added based on reported concentrations (ESI Table I†).35 Renewable rheology modifiers and gellants screened included agars, carrageenan, gelatin, methylcellulose, pectin, sodium alginate-calcium chloride mixture, guar gum, and chia seeds. The mixtures were allowed to sit for 15 minutes to allow additives to bloom and then mixtures were stirred and heated to 50 °C. Glycerol (50 grams) was added and the mixture was stirred for five minutes before being removed from the heat and cast into vials and 100 mL molds. Formulations were left for 72 hours to set before samples were evaluated.
Results and discussion
Saponification of fresh and waste fats and oils
Both fresh and waste liquid oils and solid fats form liquid soap solutions upon saponification and dilution (Fig. 2A). Fresh oil-based soaps were more transparent and lighter in color than the source oil, whereas the waste oil-based soap was a darker yellow-orange in comparison (Fig. 2C). Soaps from lipid sources with shorter or more saturated fatty acid chains, including the coconut and bacon fat samples, form heterogeneous mixtures with a suspended solid fraction. When shaken, bacon fat soap becomes iridescent due to the mixing of clear liquid and opaque solid fractions.
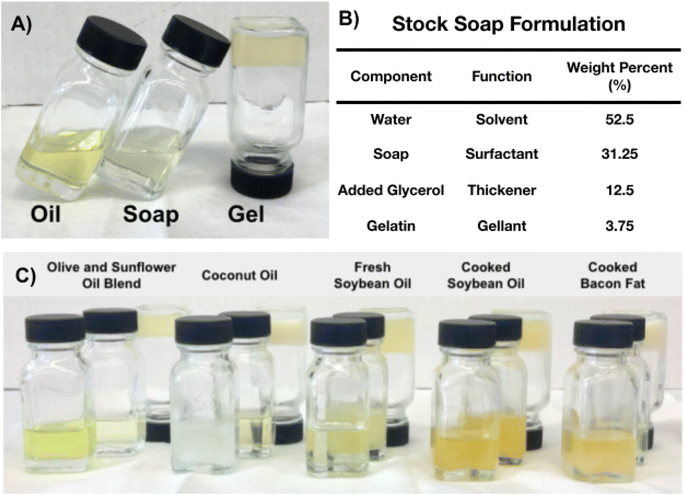 |
| Fig. 2 (A) Photograph of a sunflower and olive oil blend, blend oil soap, and a blend oil soap gel. (B) Composition and functions of soap gel components. (C) Oils, soaps, and gels from both fresh and waste fats and oils. | |
Foamability of soap solutions
All of the stock soap solutions mix with air and form stable foams. The fresh soybean oil soap foam is closest to the volume of foam from a commercial standard Dr Bronner's liquid soap (Table 1). The choice of base for saponification impacts the volume of foam. Soaps made from a blend of sunflower and olive oil treated with NaOH generated twice the foam as soaps made with KOH. Coconut oil soap with the largest foam volume has a foamability of approximately five times that of waste soybean oil with the lowest. Though waste soybean oil soap formed the lowest volume of foam, it was the only solution with foam remaining after one week.
Table 1 Foamability of fresh and waste oil-derived soaps compared to that of a commercial product
Sample |
Base |
Foam volume (L) |
Coconut oil soap |
KOH |
0.570 |
Fresh soybean oil soap |
KOH |
0.375 |
Waste soybean oil soap |
KOH |
0.115 |
Bacon fat soap |
KOH |
0.350 |
Blend oil soap |
KOH |
0.125 |
Blend oil soap |
NaOH |
0.250 |
Dr Bronner's |
KOH |
0.375 |
Viscometry of soap solutions
Rotational viscometry was used to evaluate the soap solutions' response to changes in shear forces. The soaps all demonstrated non-Newtonian behavior indicative of either shear-thinning or -thickening with an increase in the shear rate (ESI Fig. S1†). Soaps from the blend oil or fresh soybean oil demonstrated shear-thinning properties, where the viscosity decreased as the shear forces increased. The waste soybean oil soap and bacon fat soap demonstrated shear-thickening properties similar to the commercial soap, where the viscosity increased as shear forces increased. The coconut oil soap demonstrated multiple regimes of first shear-thinning properties at low shear rates and shear-thickening above 30 rpm. Soap solutions derived from oils that were liquid at room temperature had higher viscosities than those derived from coconut oil or bacon fat.
Tailoring the viscosity of waste-derived soap solutions
The material properties of bacon fat soaps were further analyzed using viscometry to understand how a single feedstock may be tailored to exhibit shear-thinning or thickening behavior. The viscosities of concentrated and dilute bacon fat-derived soap solutions were compared to that of the stock mixture composed of 53% water by weight (Fig. 2B and 3A). All concentrations of soap solution demonstrated shear-thickening properties at shear rates above 30 rpm. When increasing shear from 1.5 to 30 rpm more dilute soap solutions at lower viscosities demonstrated shear thickening, while high viscosity solutions demonstrated shear thinning. Stock soap solutions with increasing concentrations of glycerol increase in viscosity (Fig. 3B). When increasing shear rates concentrated glycerol-soap mixtures (10.5 and 14% glycerol) showed shear-thinning, and more dilute solutions (3.5 and 7.0%) demonstrated shear-thickening behavior. Additional glycerol modifies the viscosity of the liquid soap solution, but without a gellant, no stock solution formed a gel.
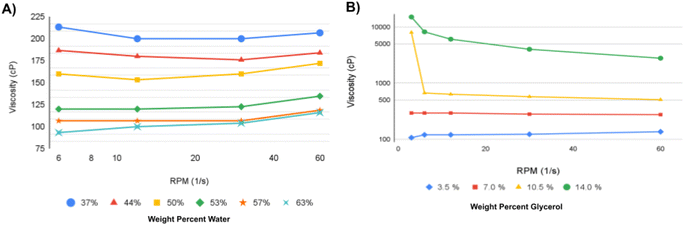 |
| Fig. 3 Graphs showing (A) the changing viscosity of bacon fat soap dilutions with water and (B) the non-Newtonian behaviors of soap-glycerol mixtures. Varying concentrations can help tailor material properties of the soaps. | |
Evaluating the compatibility of gellants and soap solutions
Different gellants from renewable resources were screened for their capability to gel stock soap solutions derived from waste soybean oil (ESI Table SI†). Heating, cooling, and then inverting soap-glycerol-gelatin formulations and viewing if the mixture flows can indicate whether a liquid mixture or gel has formed. Either carbohydrate- or protein-derived gellants were observed to form several types of mixtures. Homogeneous and separated solutions indicate that gelation did not occur. Weak or partial gels are solid-like coagulated mixtures that become fluid upon inversion. Of the samples tested with soap only gelatin and methylcellulose formed solid gels upon inversion. Methylcellulose gels were dispersions with visible droplets consisting of a gellant suspended in a thick viscous solution. The formulations subsequently described contain gelatin which formed uniform viscoelastic gels (Fig. 2C).
Formulation of soap solutions into gels
Adding both gelatin and glycerol to soap solutions gels each of the fresh and waste oil- and fat-derived soap solutions. The liquid nature of the soap solutions and the viscoelastic or more solid-like nature of the gel highlight the change in phase after gelation (Fig. 2A). Fig. 2B details the composition of formulated soap gels from the stock soap solutions. Compared to soap solutions, gels are translucent or opaque (Fig. 2C). The soaps derived from cooked oils retain a darker yellow-orange color in their formulated gels.
Comparing infrared profiles of feedstock oils, soaps, and gels
Oils and fat feedstocks show similar peaks in the infrared region from methylene and methyl C–H stretches in fatty acid chains between 2800 and 3200 cm−1 (Fig. 4A). Composed mostly of water and glycerol, soaps and gels show large O–H stretching bands between 2750 and 3750 cm−1 (Fig. 4B and C). Dr Bronner's liquid soap and the corresponding gel show peaks in the fingerprint region below 1500 cm−1 due to additional organics including aroma compounds. A strong carbonyl (C
O) stretch present at 1740 cm−1 in oil and fat samples broadens and shifts to lower wavenumbers upon esterification and metal complexation of component fatty acids.17 The carbonyl stretch shifts in all corresponding soap samples to 1650 cm−1 representing free fatty acids and 1540 cm−1 for the carbonyl group in the metal alkanoate surfactant.
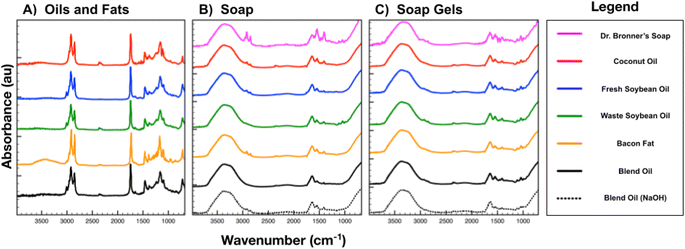 |
| Fig. 4 IR spectra of (A) fresh and waste oils and fats, (B) saponified mixtures of fresh and waste oils and fats, and soap and (C) soap gels from fresh and waste oils and fats, and commercial soap. Oils are saponified with potassium hydroxide (KOH) unless otherwise noted. | |
Comparing infrared profiles of fresh and waste soybean oil and soap gels
Infrared profiles of fresh and waste soybean oil and their soap gels indicate that varying the feedstock of the soap gel creates products with similar IR profiles (Fig. 5A and B). The C–H stretches at 2920 and 2850 cm−1 from the fresh and waste soybean oil are indicative of saturated long-chain fatty acids present in the triglycerides before and after cooking. The less intense methylene C–H stretch just above 3000 cm−1 indicates the presence of unsaturated lipids, which here do not appear to be greatly changed by the cooking or formulation process. Of note are minor free fatty acid peaks in both untreated fresh and waste soybean oil at 1640 cm−1 (Fig. 5C).
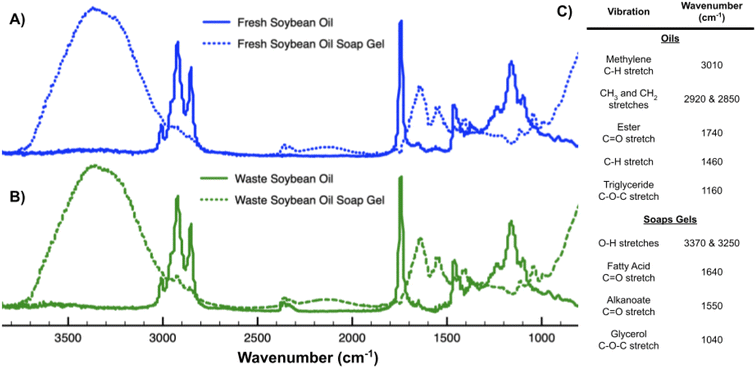 |
| Fig. 5 Comparison of infrared spectra of (A) fresh soybean oil and soap gels and (B) waste soybean oil and soap gels. (C) Table of notable peaks including the triglyceride carbonyl stretches present in oils and soap gels for the free fatty acids and the metal salts. | |
Melting points of soap gels
Melting point ranges are used to study the transition of formulations between the gel and solution states (ESI Table SII†). The full range of the melting points for the gelatin soap gels derived from fresh and waste oils and fats was from 28 °C to 37 °C, with all samples melting between ambient room temperatures and the human body temperature of 37 °C. The melting points of the coconut soap gel and the waste soybean oil soap gel were the closest to that of the gel formulated from the commercial soap. The coconut soap gel also had the lowest melting point, whereas the bacon fat soap gel had the highest melting point. All gels formed are thermoreversible and gelled again after cooling.
Comparing amounts of waste-derived feedstocks in soaps and gels
The percentage of waste-derived components in soaps and gels reflects a reduced dependence on fresh edible oils and fats needed for soap formulations. The percent of waste included in the soaps ranges from zero for fresh oil-derived samples to 59% for the waste-derived soybean oil and bacon fat stock soap formulations (ESI Table SIII†). The gels consisting of the soap, added water, and gellants have either zero percent included waste for fresh feedstocks or 31% waste included in the bacon fat and cooked soybean oil formulations. The percentage of waste included in products is related to the gellant-specific formulation and the source-specific saponification value, which is the amount of base required to react with component triglycerides.34
Discussion
The source of oils and fats and their triglycerides directly influences the composition and the material properties of derived soap solutions. Shorter saturated fatty acid chains in coconut oil or bacon fat serve to solidify these lipid sources at room temperature and form iridescent binary-phase soap solutions. Waste soybean oil-derived soaps can be used to create long-lasting low-foaming soaps.36 Low-foaming can indicate a surfactant mixture's high detergency and help reduce the amount of water used in washing.37 Species generated during the heating and cooking process contribute to these properties in soap formulations.
Soap solutions from fresh and waste feedstocks show shear-thinning and shear-thickening properties, respectively. As the fatty acids in triglycerides oxidize and polymerize during cooking they create species with higher molecular weights. Colloidal mixtures have been demonstrated to show multiple flow regimes in response to changes in shear forces.38,39 Soaps from bacon fat may prove to be a valuable feedstock when tailoring gelled commercial products with desired textural and stimuli-responsive behavior. Glycerol may be used to further control the behavior of the liquid phase comprising formulated gels.
Spectroscopic analysis indicates that liquid soap solutions derived from waste fats and oils are similar in composition to commercial liquid soap. The redshift of the feedstock's carbonyl stretch after saponification to lower wavenumbers indicates the transformation of the triglyceride's conjugated fatty acids into free fatty acids and organometallic surfactants. As the choice of feedstock for liquid soap impacts the solution's response to shear forces, soaps from both fresh and waste oils and fats may be paired in blends to create soaps with tailored properties.
Gelatin is a suitable gellant for solidifying oil- and fat-derived soap-glycerol mixtures. A plant-based gellant, methylcellulose, may prove to be a valuable vegan alternative for the gelation of soap mixtures.40,41 Here, waste-derived soap gels melt above room temperature but below body temperature. Of the many different desirable material properties for soap gels, a programmable transition between liquid and solid phases can give end-users control over the portioning, application, and storage of gelled soaps.
While a high percentage of waste-derived feedstocks does not guarantee a formulation to be sustainable, the minimization of fresh or edible feedstocks and the development of efficient chemical transformations correlate with the green chemistry principles of waste prevention and atom economy. Using waste-derived feedstocks is one strategy to mitigate the environmental impacts of consumer product manufacturing, and this mirrors current efforts to include high percentages of recycled content in consumer products.
Conclusion
Common fresh and cooked waste oils and fats were saponified and formulated into thermoreversible gels with glycerol and gelatin. Spectroscopy and viscometry demonstrate that soaps from fresh or cooked oils have similar compositional profiles yet different responses to shear forces. Infrared spectroscopy confirms the conversion of triglycerides into free fatty acids, glycerol, and metal alkanoates. Fresh saponified oils demonstrated non-Newtonian shear-thinning behavior, while waste-derived feedstocks demonstrated shear-thickening behavior. While fresh coconut oil soap had the highest foamability and waste soybean oil had the lowest, the latter was the only soap that formed a stable foam lasting for more than one week. Soaps from fresh and waste oils and fats were formulated into gels that melt below body temperature. Cooked oils and fats can be diverted from streams of food waste to be used as feedstocks to synthesize soap solutions, but their composition and material properties need further study along with a toxicological evaluation before incorporation into commercial products. Biobased soap gels may prove a meaningful way to reduce the amount of edible and petroleum-based feedstocks in consumer products and help store carbon in materials without sacrificing the desirable properties of commercial soap products.
Author contributions
MS designed the soap gel formulation. MS, AG, CM, MY, and JD collected the data. JS designed the analysis. AG, MY, and JS performed the analysis, and JS and AG wrote the manuscript.
Conflicts of interest
There are no conflicts of interest to declare.
Acknowledgements
The authors would like to acknowledge Manhattan College's Department of Chemistry and Biochemistry and Environmental Science Program for their support. Funding was provided by the School of Science, the Jasper Summer Scholars Research Program, and the Faculty Development Program. Dr Jonathan Patete provided training on the infrared spectrometer, and Dr Yelda Hangun-Balkir provided oils for saponification. Brock Sindt cooked and collected the soybean oil and bacon fat used for experiments.
References
- Z. Dou and J. D. Toth, Global Primary Data on Consumer Food Waste: Rate and Characteristics – A Review, Resour., Conserv. Recycl., 2021, 168, 105332, DOI:10.1016/j.resconrec.2020.105332
.
- J. Esteban and M. Ladero, Food Waste as a Source of Value-added Chemicals and Materials: A Biorefinery Perspective, Int. J. Food Sci. Technol., 2018, 53(5), 1095–1108 CrossRef CAS
.
- W. H. Foo, W. Y. Chia, D. Y. Y. Tang, S. S. N. Koay, S. S. Lim and K. W. Chew, The Conundrum of Waste Cooking Oil: Transforming Hazard into Energy, J. Hazard. Mater., 2021, 417, 126129 CrossRef CAS PubMed
.
- R. Stout, Biodiesel from Used Oil, J. Chem. Educ., 2007, 84(11), 1765–1771, DOI:10.1021/ed084p1765
.
- R. Alcantara, J. Amores, L. Canoira, E. Fidalgo, M. J. Franco and A. Navarro, Catalytic Production of Biodiesel from Soy-Bean Oil, Used Frying Oil and Tallow, Biomass Bioenergy, 2000, 18(6), 515–527, DOI:10.1016/S0961-9534(00)00014-3
.
- G. Santori, G. Di Nicola, M. Moglie and F. Polonara, A Review Analyzing the Industrial Biodiesel Production Practice Starting from Vegetable Oil Refining, Appl. Energy, 2012, 92, 109–132, DOI:10.1016/j.apenergy.2011.10.031
.
- S. Živković and M. Veljković, Environmental Impacts the of Production and Use of Biodiesel, Environ. Sci. Pollut. Res., 2018, 25(1), 191–199 CrossRef PubMed
.
-
R. J. Weselake, Storage Lipids, in Plant Lipids, Blackwell, 2020, pp. 162–225 Search PubMed
.
- G. John, S. Nagarajan, P. K. Vemula, J. R. Silverman and C. K. S. Pillai, Natural Monomers: A Mine for Functional and Sustainable Materials – Occurrence, Chemical Modification and Polymerization, Prog. Polym. Sci., 2019, 92, 158–209 CrossRef CAS
.
- R. Ravotti, J. Worlitschek, C. R. Pulham and A. Stamatiou, Triglycerides as Novel Phase-Change Materials: A Review and Assessment of Their Thermal Properties, Molecules, 2020, 25(23), 5572 CrossRef CAS PubMed
.
- A. Mathew and K. Anand, Comparison of Engine Characteristics with Biodiesels Produced from Fresh and Waste Cooking Oils, Biofuels, 2021, 12(6), 663–671 CrossRef CAS
.
- V. Plata, D. Ferreira-Beltrán and P. Gauthier-Maradei, Effect of Cooking Conditions on Selected Properties of Biodiesel Produced from Palm-Based Waste Cooking Oils, Energies, 2022, 15(3), 908 CrossRef CAS
.
- B. Antonić, D. Dordević, S. Jančíková, B. Tremlova and I. Kushkevych, Physicochemical Characterization of Home-Made Soap from Waste-Used Frying Oils, Processes, 2020, 8(10), 1219 CrossRef
.
- T. Maotsela, G. Danha and E. Muzenda, Utilization of Waste Cooking Oil and Tallow for Production of Toilet “Bath” Soap, Procedia Manuf., 2019, 35, 541–545 CrossRef
.
-
J. A. Ferreira, S. Agnihotri and M. J. Taherzadeh, Waste Biorefinery, in Sustainable resource recovery and zero waste approaches, Elsevier, 2019, pp. 35–52 Search PubMed
.
- J. Cárdenas, A. Orjuela, D. L. Sánchez, P. C. Narváez, B. Katryniok and J. Clark, Pre-Treatment of Used Cooking Oils for the Production of Green Chemicals: A Review, J. Clean. Prod., 2021, 289, 125129, DOI:10.1016/j.jclepro.2020.125129
.
- M. Berrios, M. A. Martín, A. F. Chica and A. Martín, Purification of Biodiesel from Used Cooking Oils, Appl. Energy, 2011, 88(11), 3625–3631, DOI:10.1016/j.apenergy.2011.04.060
.
-
ASTM D2357-11, Standard for Qualitative Classification of Surfactants by Infrared Absorption, in, Annual Book of ASTM Standards, ASTM International, West Conshohocken, PA, 2021, vol. 15.04 Search PubMed
.
- R. A. Meiklejohn, R. J. Meyer, S. M. Aronovic, H. A. Schuette and V. W. Meloche, Characterization of Long-Chain Fatty Acids by Infrared Spectroscopy, Anal. Chem., 1957, 29(3), 329–334, DOI:10.1021/ac60123a002
.
- A. Kuntom and H. Kifli, Properties of Soaps Derived from Distilled Palm Stearin and Palm Kernel Fatty Acids, J. Surfactants Deterg., 1998, 1(3), 329–334 CrossRef CAS
.
- A. O. Ameh, J. A. Muhammad and H. G. Audu, Synthesis and Characterization of Antiseptic Soap from Neem Oil and Shea Butter Oil, African J. Biotechnol., 2013, 12(29), 4656–4662 Search PubMed
.
- L. S. Mackenzie, H. Tyrrell, R. Thomas, A. S. Matharu, J. H. Clark and G. A. Hurst, Valorization of Waste Orange Peel to Produce Shear-Thinning Gels, J. Chem. Educ., 2019, 96(12), 3025–3029, DOI:10.1021/acs.jchemed.8b01009
.
- M. A. Delgado, C. Valencia, M. C. Sánchez, J. M. Franco and C. Gallegos, Influence of Soap Concentration and Oil Viscosity on the Rheology and Microstructure of Lubricating Greases, Ind. Eng. Chem. Res., 2006, 45(6), 1902–1910 CrossRef CAS
.
- L. Z. Rogovina, V. G. Vasil'ev and E. E. Braudo, Definition of the Concept of Polymer Gel, Polym. Sci., Ser. C, 2008, 50(1), 85–92 CrossRef
.
- A. Fameau and A. G. Marangoni, Back to the Future: Fatty Acids, the Green Genie to Design Smart Soft Materials, J. Am. Oil Chem. Soc., 2022, 99(7), 543–558 CrossRef CAS
.
- D. K. Smith, Applications of Supramolecular Gels, Mol. Gels Struct. Dyn., 2018, 300–371 CAS
.
-
N. A. Morrison, R. C. Clark, Y. L. Chen, T. Talashek and G. Sworn, Gelatin Alternatives for the Food Industry, in Physical chemistry and industrial application of gellan gum, Springer, 1999, pp. 127–131 Search PubMed
.
- F. Zha, J. Rao and B. Chen, Plant-Based Food Hydrogels: Constitutive Characteristics, Formation, and Modulation, Curr. Opin. Colloid Interface Sci., 2021, 56, 101505 CrossRef CAS
.
- J. Bello, H. C. A. Riese and J. R. Vinograd, Mechanism of Gelation of Gelatin. Influence of Certain Electrolytes on the Melting Points of Gels of Gelatin and Chemically Modified Gelatins, J. Phys. Chem., 1956, 60(9), 1299–1306 CrossRef CAS
.
- Y. Uraki, T. Imura, T. Kishimoto and M. Ubukata, Body Temperature-Responsive Gels Derived from Hydroxypropylcellulose Bearing Lignin, Carbohydr. Polym., 2004, 58(2), 123–130 CrossRef CAS
.
- J. R. Silverman and R. Hudson, Evaluating Feedstocks, Processes, and Products in the Teaching Laboratory: A Framework for Students To Use Metrics to Design Greener Chemistry Experiments, J. Chem. Educ., 2020, 97(2), 390–401, DOI:10.1021/acs.jchemed.9b00292
.
- M. Atasoy, I. Owusu-Agyeman, E. Plaza and Z. Cetecioglu, Bio-Based Volatile Fatty Acid Production and Recovery from Waste Streams: Current Status and Future Challenges, Bioresour. Technol., 2018, 268, 773–786 CrossRef CAS PubMed
.
- C. Rodriguez Correa and A. Kruse, Biobased Functional Carbon Materials: Production, Characterization, and Applications—A Review, Materials, 2018, 11(9), 1568 CrossRef PubMed
.
- S. T. Mabrouk, Making Useable, Quality Opaque or Transparent Soap, J. Chem. Educ., 2005, 82(10), 1534, DOI:10.1021/ed082p1534
.
-
M. Lersch, Texture – A hydrocolloid recipe collection (v.3.0), https://khymos.org/recipe-collection/, accessed Jun 21, 2022 Search PubMed
.
- N. R. Lee, M. Cortes-Clerget, A. B. Wood, D. J. Lippincott, H. Pang, F. A. Moghadam, F. Gallou and B. H. Lipshutz, Coolade. A Low-Foaming Surfactant for Organic Synthesis in Water, ChemSusChem, 2019, 12(13), 3159–3165, DOI:10.1002/cssc.201900369
.
- Y. Hirata, M. Ryu, Y. Oda, K. Igarashi, A. Nagatsuka, T. Furuta and M. Sugiura, Novel Characteristics of Sophorolipids, Yeast Glycolipid Biosurfactants, as Biodegradable Low-Foaming Surfactants, J. Biosci. Bioeng., 2009, 108(2), 142–146, DOI:10.1016/j.jbiosc.2009.03.012
.
- D. A. Sessoms, I. Bischofberger, L. Cipelletti and V. Trappe, Multiple Dynamic Regimes in Concentrated Microgel Systems, Philos. Trans. R. Soc., A, 2009, 367, 5013–5032 CrossRef CAS PubMed
.
- T. Kawasaki, A. Ikeda and L. Berthier, Thinning or Thickening? Multiple Rheological Regimes in Dense Suspensions of Soft Particles, EPL, 2014, 107(2), 28009 CrossRef
.
- L. Li, Thermal Gelation of Methylcellulose in Water: Scaling and Thermoreversibility, Macromolecules, 2002, 35(15), 5990–5998, DOI:10.1021/ma0201781
.
- K. Kobayashi, C. Huang and T. P. Lodge, Thermoreversible Gelation of Aqueous Methylcellulose Solutions, Macromolecules, 1999, 32(21), 7070–7077, DOI:10.1021/ma990242n
.
Footnotes |
† Electronic supplementary information (ESI) available. See DOI: https://doi.org/10.1039/d2su00130f |
‡ Present address: Department of Science and Mathematics, The Fashion Institute of Technology, 227 West 27th Street, #B831 New York, NY, 10001, USA. |
|
This journal is © The Royal Society of Chemistry 2023 |
Click here to see how this site uses Cookies. View our privacy policy here.