DOI:
10.1039/D3RA05062A
(Review Article)
RSC Adv., 2023,
13, 30586-30605
Natural surfactant mediated bioremediation approaches for contaminated soil
Received
26th July 2023
, Accepted 7th September 2023
First published on 18th October 2023
Abstract
The treatment of environmental pollution by employing microorganisms is a promising technology, termed bioremediation, which has several advantages over the other established conventional remediation techniques. Consequently, there is an urgent inevitability to develop pragmatic techniques for bioremediation, accompanied by the potency of detoxifying soil environments completely. The bioremediation of contaminated soils has been shown to be an alternative that could be an economically viable way to restore polluted soil. The soil environments have long been extremely polluted by a number of contaminants, like agrochemicals, polyaromatic hydrocarbons, heavy metals, emerging pollutants, etc. In order to achieve a quick remediation overcoming several difficulties the utility of biosurfactants became an excellent advancement and that is why, nowadays, the biosurfactant mediated recovery of soil is a focus of interest to the researcher of the environmental science field specifically. This review provides an outline of the present scenario of soil bioremediation by employing a microbial biosurfactant. In addition to this, a brief account of the pollutants is highlighted along with how they contaminate the soil. Finally, we address the future outlook for bioremediation technologies that can be executed with a superior efficiency to restore a polluted area, even though its practical applicability has been cultivated tremendously over the few decades.
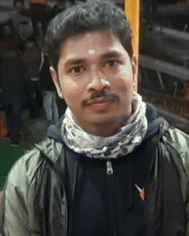 Pintu Sar | Dr Pintu Sar received his PhD degree from The University of Burdwan, India, in 2017. He has two years (2017–2019) of postdoctoral research experience at the Polymer Science Division, IISER-Kolkata. Over a decade of professional, research and teaching experience, he has contributed more than 26 articles in international journals of repute. His research is primarily focused on developing organic transformation in water with a fundamental understanding of the role of nanomicelle in controlling the reaction kinetics, including the remediation technologies. |
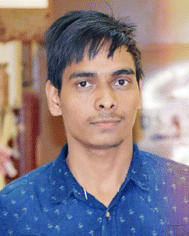 Sandip Kundu | Sandip Kundu achieved his MSc (Chemistry) from The University of Burdwan in 2019. He is currently working as an SRF under the supervision of Prof. Bidyut Saha in the Department of Chemistry at Burdwan University, WB, India. |
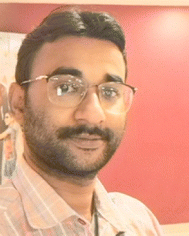 Aniruddha Ghosh | Dr Aniruddha Ghosh was awarded a doctoral degree from The University of Burdwan in 2016. He spent a couple of years as a National Postdoctoral Fellow in the “Heterogeneous Catalysis” division at the University of Kalyani. During his postdoctoral journey under a DST-SERB funded project he focused on catalytic organic synthesis by mesoporous nanomaterials in solvent free media. He primarily focuses on metal mediated organic transformations in micellar media. He has published 82 articles in reputed international journals. |
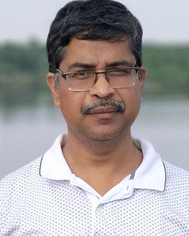 Bidyut Saha | Bidyut Saha is a Professor (Full) at the Department of Chemistry, The University of Burdwan, India. He obtained his PhD degree from Visva Bharati University, India, in 2007. He was a visiting scientist for the years 2009–2010 at the Department of Chemistry, UBC, Vancouver, Canada. His research team is working on the bioremediation of toxic metals, micellar catalysis, chemical kinetics, microemulsions, and metallogels. He has published 170 research/review articles in international journals together with 15 book chapters. He is now serving as Editor, Associate Editor and Editorial Board Member for various reputed journals. |
1. Introduction
Soil is a complex environmental component that presents as a mediator between groundwater and air; it is very difficult to regenerate. Basically, soil pollution is associated with the various pollutants exposed through human and other animal activities. The contaminating pollutants directly or indirectly penetrate into the soil environments through different channels. This leads to the concentration of the pollutants exceeding beyond the maximum soil capacity of accommodation as well as assimilation. Soil pollution readily depends on the contamination of both aspects, and includes several well-known factors, like the extensive use of pesticides and herbicides, incessant disposal of hazardous products, accidental oil spills, acid rain, and the discharge of intensely polluting agents from industries.1,2 The continuous growing impact of human activity and industrial development has severely influenced the environment through pollution with water insoluble organic hazardous chemicals. Since soil has a very complex structure (composed of organic matter, gases, minerals, liquids and micro-organisms), it is highly susceptible to be polluted by multifarious contaminants because of the binding ability with numerous chemical species. Nowadays industrial developments are increasing to enhance human society, the effect of which is responsible for creating an unhealthy environment. Several hazardous materials, including organic pollutants, heavy metals, solvents, plastics, nuclear hazards, and medical wastes are present in the soil with a high enough concentration to pollute the soil matrix.3–5 The existence of a number of hydrophobic organic hazardous components in the environment has caused serious threats, including mutagenic, carcinogenic and teratogenic effects, to mammals. Organic pollutants consist of a wide variety of organic xenobiotic chemicals, almost insoluble in the aquatic environment and that may impend in water, sediment or biota. The organic pollutants, especially gasoline, plastics, paints, pesticides, polycyclic aromatic hydrocarbons (PAHs), adhesives, polychlorinated biphenyls, benzene, ethylbenzene, toluene and many more, are actual hazards to the soil environment. Therefore, soil contamination is a serious environmental problem across the world, for which the agricultural output of land decreases, the health of human beings faces many serious threats, and underground water becomes polluted, which ultimately creates a negative impact on the ecosystem and biodiversity. Thus, it is necessitated to clean up contaminated soil to get our environment back into its native form. Several technologies have been developed to maintain the quality of soil,6,7 emphasizing the transformation and detoxification of pollutants. It is worth accounting for the disadvantages of physical and chemical remediation processes concerning high economic factors required by the treatment of a huge excess of hazardous waste leading to a direct detriment of the local ecosystem.8 The many advantages of bioremediation,9 such as the most economical, environmentally friendly and simplest approaches, over other existing technologies enable bioremediation for the permanent elimination of contaminants from polluted sites. Bioremediation is a smarter technology associated with the microbial species present in the environment involving the biological removal of pollutants from the contaminated soil matrix. The bioremediation process is not only concerned with the microorganisms but also deals with several metabolites which they fabricated, in particular surface-active agents. Hence, extensive studies have been reported on the remediation of contaminated soil by numerous research groups since its commencing.10–14 Bioremediation techniques essentially require suitable soil conditions for microbial activity and the accurate selection of the active microorganisms. The highly recalcitrant nature of some organic and inorganic pollutants makes some limitations to this promising technique. In this circumstance, biologically derived natural surfactants play a leading role to address the limitation of detoxifications using the bioremediation approach.15 Surface-active compounds are synthesized with the help of microorganisms, known as biosurfactants, and have the ability to assist the bioremediation process to be effective in the removal of pollutants from a soil matrix.16–20 The fact that biosurfactants can improve the solubilization of pollutants from contaminated soil and increase their solubility, which in turn enhances their bioavailability, is the cause of the growing interest in them.21 Decontamination of soil can be performed in situ (at the site where it is occurring – ‘soil flushing’) or ex situ (after the excavation of contaminated soil – ‘soil washing’), applying systematic arrangements. This includes stabilization, solidification, extraction of vapor as well as electrochemical techniques and biological or thermal treatment.22 It is best to first present a brief highlight of different classes of soil pollutants, possible sources of soil contamination, chemistry of biosurfactants, and microbial production of biosurfactants to illustrate the significant issues. Then the biosurfactant assisted bioremediation of soil (contaminated by hydrocarbons, heavy metals, and emerging pollutants) is demonstrated comprehensively together with numerous literature reports. Finally, a brief discussion on (a) the mechanistic action of biosurfactants on soil contaminants and (b) a comparison of biosurfactants with synthetic surfactants is essential in order to sketch out the significance of biosurfactants for soil bioremediation. At the end, a description about the limitations of biosurfactant assisted remediation along with concluding remarks make this review comprehensive.
2. Soil pollutants: possible route of contamination
Soils are the leading environmental sink for a variety of polluting agents, contamination of which increases the risk of ecological systems around the world. A wide diversity of inorganic and organic chemicals and pollutants may appear in soil in various identities, compositions and concentrations. Soils are polluted by the following primary chemicals and/or substances (Table 1).
Table 1 Numerous kinds of soil pollutants with their sources
Pollutants |
Sources/contamination path |
Ref. |
Naphthalene, phenanthrene, pyrene |
Fossil fuel combustion, traffic emissions, coke ovens, diesel and gasoline engines |
35 |
DDT |
Directly used as pesticides and agricultural pests and vectors |
36 |
HCB |
Air emissions, combustion products, municipal incinerators, volatilization and leaching from landfills |
37 |
PCBs |
Widely used as coolants and lubricants in transformers, also used in numerous electrical equipment |
38 |
α-HCH and β-HCH |
Leaching and volatilization, deposition of wastes from chemical industries |
38 |
Heavy metals (Pb, Cd, Cu, Hg, Sn, Zn) |
Disposal of wastes from industrial areas, leaded gasoline and paints, application of fertilizers and pesticides, sewage sludge, wastewater irrigation, spillage of petrochemicals, coal combustion residues |
39 |
Bisphenol A (BPA), phthalates, parabens, chlorpyrifos |
Plastics, plasticizers, pesticides |
40 |
2.1 Agrochemicals
Crop production is mainly governed by the presence of the required number of micronutrients: nitrogen, phosphorous and potassium (NPK). In order to meet the rising demand for food, synthetic fertilizers have been used extensively for a long time to maximize crop output. The excessive consumption of synthetic fertilizers changes the superiority and enrichment of the soil and, as a consequence, the soil becomes polluted. Together with the use of pesticides, including insecticides, herbicides, and fungicides, these are a matter of serious concern to the world because of their better interaction with the constituents of soil.23 A significant number of degraded products and residues build up in the soil environment as a result of the ongoing use of agrochemicals in agriculture, which may pose serious hazards to soil and food chain pollution.24 The types of agrochemicals are: insecticides (e.g., chlordimeform, diazinon, dimethoate, aldrin, chlordane, DDT, flumethrin, permethrin, etc.); acaricides (e.g., fenpyroximate, menthol, formic acid, tau-fluvalinate, thymol, etc.); herbicides (e.g., acetanilides, barban, alachlor, chlorbromuron, 2,4-D, 2,4,5-T, etc.); bactericides (e.g., copper hydrochloride, copper oxychloride, copper sulfate, different rice blast nets, dithane, polytrin, ridomil, and many more).
2.2 Polycyclic aromatic hydrocarbons (PAHs)
Due to the poisonous, mutagenic, and carcinogenic properties, organic pollutants coupled with petroleum, such as PAHs, are regarded as hazardous pollutants. The PAHs are very common organic pollutants, having two or more fused aromatic rings liable to pollute the soil, and tend to retain in the soil matrix for a range of time. The number of aromatic benzene rings determines the classification of PAHs; those with two or three rings are classified as low molecular weight (LMW) PAHs, including anthracene, naphthalene and phenanthrene, while those with four or more rings are classified as high molecular weight (HMW) PAHs, including chrysene, pyrene, benzo(a)pyrene, coronene, etc. Depending on their molecular weight, they have a variety of physicochemical and toxicological properties. Typically, both anthropogenic and natural activities result in the production of these.25
2.3 Persistent organic pollutants (POPs)
The POPs are organic compounds with a greater affinity towards the soil matrix, accounted by their bioaccumulation for a long period.26 The lipophilic and hydrophobic properties of POPs facilitate a strong interaction with cell membranes and consequently they can readily enter into the food chain of living organism, which leads to a significant risk for human health.27 Examples of POPs are: polychlorinated biphenyl (PCB), polychlorinated dibenzofurans (PCDFs), polybrominated diphenyl ethers, per- and polyfluoroalkylated substances (PFAS), short-chain chlorinated paraffins, etc.28
2.4 Toxic heavy metals
The atmospheric deposition of heavy metals from industrial areas, the disposal and treatment of waste, the use of commercial fertilizers, the use of sewage sludge, and other processes resulting from the deterioration of various materials are examples of heavy metal inputs into the environment.29,30 Many heavy metals are present in soils, and average quantities vary across the globe. Examples include Cu (20 mg kg−1), Cd (0.06 mg kg−1), Cr (20–200 mg kg−1), Pb (10–150 mg kg−1), Ni (40 mg kg−1), and Zn (10–300 mg kg−1). Nevertheless, the basic parent materials of pollution can lead to 10–1000 times higher concentrations of heavy metals in metal-rich soils. Hazardous metals in soils, such as As, Cd, Cr, Cu, Hg, Pb, and Zn, are thought to be the most contaminating metals there are, and their fundamental characteristics include non-degradability, persistence, bioaccumulation, and biomagnification in a food chain.31
2.5 Radioisotopes
Environmental radionuclides can be created artificially or naturally. According to estimates, on average, 79% of the radiation to which people are exposed comes from natural sources, 19% from medical use, and the remaining 2% is caused by radiation from nuclear power plants and weapons testing.32 The global effects of atmospheric nuclear weapons development and the functioning of nuclear facilities, however, have caused the majority of the public's worries about radiation from radionuclides. These two activities have contaminated substantial tracts of land with radionuclides and released a significant number of man-made radionuclides into the environment. Soils have been contaminated for much longer than anticipated by normal fission product radionuclides such 137Cs and 90Sr. A long time after the initial contamination, radioactive nuclei like 40K, 90Sr, 137Cs, 232Th, and 238U, as well as some radioactive wastes produced by nuclear reactors, are readily bio-available in the soil profile and are absorbed by plants, making them available for further dispersion within food chains.33
2.6 Emerging pollutants
Soils are affected by long-term exposure to the following class of chemicals which can promote microbial resistance and are ultimately involved in reducing the quality and excellency of agricultural soils. In addition to that, more than a few chemicals, attributed as endocrine disrupting chemicals (EDCs), are notorious for disturbing the function of endocrine systems, which can cause cancerous tumors, birth defects, and other developmental disorders, in association with adversely affecting human reproductive systems by reducing the sperm count.34 Examples are: bisphenol A (BPA), phthalates, tributyltin, methyl mercury, methylparaben, atrazine, etc.
3. Chemistry of biosurfactant
Synthetic surfactants are used for widespread applications, including detergency, personal care products, organic transformation, and medicinal purposes. Still, the toxicological and non-biodegradable characters of synthetic surfactants are creating considerable headaches. Surfactants from natural sources, known as biosurfactants, emerged to be an environmentally benign alternative to their synthetic community41,42 due to their high biodegradability, multi-functionality, low toxicity, and environmental compatibility.43
3.1 Biosurfactant: structure, properties and origin
Microbial surface-active chemicals are a class of structurally diverse molecules produced by different microorganisms. They are primarily categorized according to their chemical nature and microbial source. They are composed of an acid, peptide anions or cations, mono-, di-, or polysaccharides, which comprise their hydrophilic moiety, and fatty acids, which comprise their hydrophobic moiety. These structures have a wide range of features, such as the capacity to reduce the surface and interfacial tension of liquids and to create micelles and microemulsions between various phases. These substances can be generally classified into two classes: biosurfactants, which are low-molecular-weight (<1500 Da) substances, such as glycolipids, proteins, and lipopeptides,44 and bioemulsifiers, which are high-molecular-weight polymers of polysaccharides, lipoproteins, or lipopolysaccharide proteins (Fig. 1).
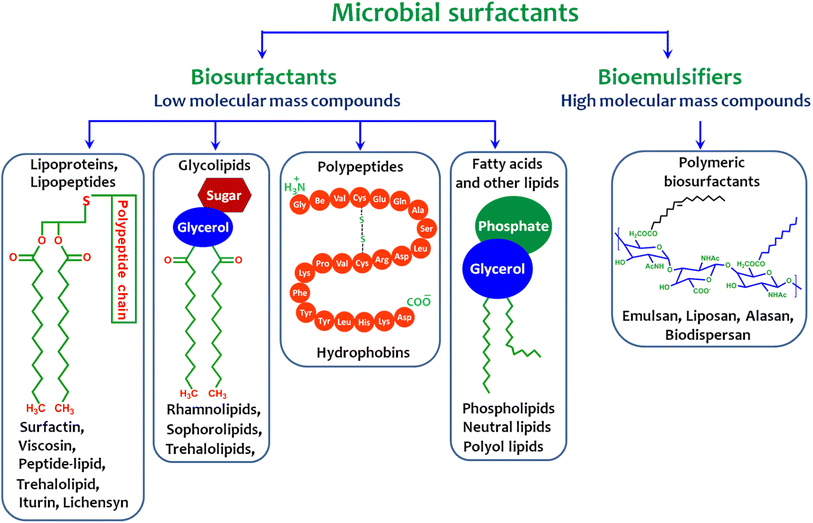 |
| Fig. 1 Classification of microbial surfactants along with their representative architectures. | |
Glycolipids are the microbial surfactants that have been investigated the most. Fig. 2 shows the proposed biosynthetic route for the production of glycolipids. Rhamnolipids, trehalolipids, sophorolipids, and mannosylerythritol lipids (MELs) are some of the most well-known substances among these.45 Since carbohydrates are the only available source of carbon for the synthesis of biopolymers, the formation of the hydrophilic moiety via a glycolytic pathway as well as the lipogenic pathway (lipid formation) is inhibited by microbial metabolism (shown in Fig. 2). A variety of enzymes break down a water-soluble substrate like glucose to generate intermediates like glucose 6-phosphate (G6P) in the glycolytic pathway. This G6P is one of the primary precursors of carbohydrates found in the hydrophilic zone of biopolymers. Glucose is initially transformed to pyruvate by glycolysis, then pyruvate is converted into acetyl-CoA, which further combines with oxaloacetate and results in the formation of malonyl-CoA, which is subsequently converted into a lipid.
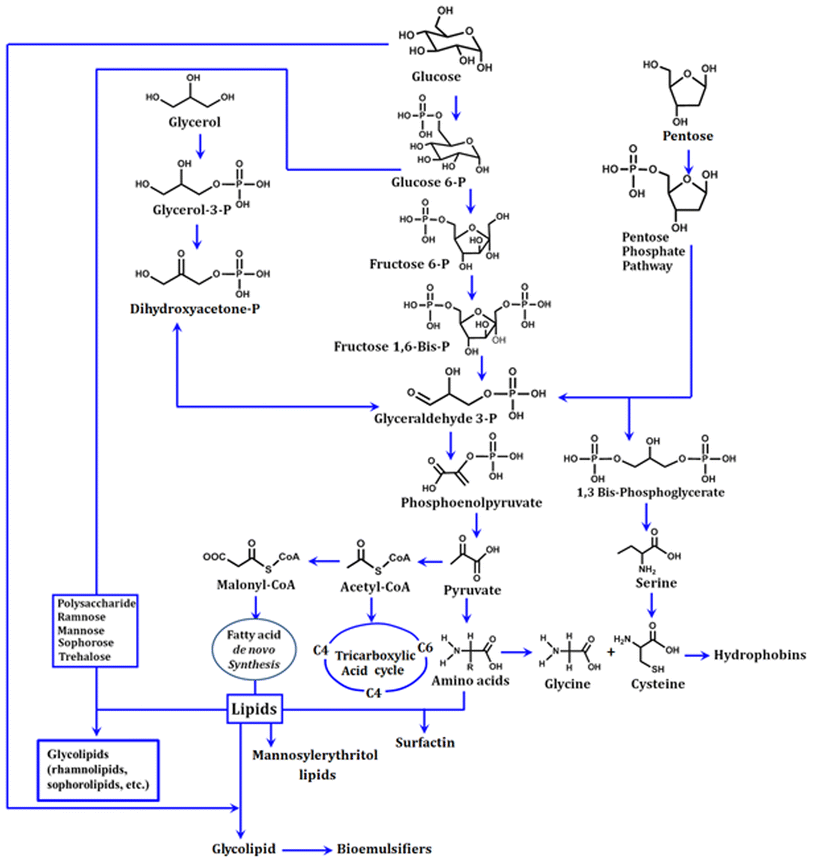 |
| Fig. 2 Biological synthetic routes for the synthesis of a variety of lipopeptides (i.e. surfactin), glycolipids (i.e. rhamnolipids, sophorolipids, etc.), bioemulsifiers and hydrophobins using carbohydrate substrates (redrawn and extended from ref. 50–52). | |
Microbial species present in the environment can be able to generate a variety of amphiphilic compounds with significant surface activities. Being a surfactant, biologically derived natural surfactants are also reported for their extensive applications in various fields.46 Biosurfactants, analogous to synthetic surfactants, form micelle like nano-aggregates within the concentration range of 1–200 mg L−1. However, a larger CMC value of biosurfactants has been accounted recently; for example, glycolipids and glycoproteins, produced by Acinetobacter baumannii47 and Rhizopus arrhizus,48 exhibit CMCs of 1200 and 1700 mg L−1, respectively. The rhamnolipids and surfactin are two well characterized natural surfactants that lower the surface tension of water from 73 to 30 mN m−1, as revealed for other biosurfactants.49
The applications of bio-derived surfactants are, though not completely, explored, but not limited to the industries of cosmetics, pharmaceuticals, agriculture, food, textile, painting, petroleum recovery and many others; the use of biosurfactants for remediation purposes is quite a lucrative and essential approach to remove pollutants from the environment through microbial degradation method.53 The contaminants, primarily organic pollutants, are usually highly recalcitrant and hardly removed by conventional remediation technologies. In addition to this, some toxic heavy metals can strongly interact with the soil matrix, which produces lots of complexities proportionally to eliminate the hazardous metals from the soil. The well-established bioremediation technique, assisted by the presence of biologically active surfactants, is a method through which the detoxification of polluted soil is executed at ease. The main problem arising in the course of a microbial bioremediation process is the availability of pollutants to the microorganism.
The insoluble nature of organic pollutants is susceptible to restricting their availability to the microorganisms. Biosurfactants are more effective in order to get a significant remediation of pollutants, since the bioavailability of contaminants, including hydrophobic compounds and heavy metals, are realistically increased in the presence of biosurfactants. Many surfactants of various types have already been studied for their potential use in accelerating the biodegradation of organic pollutants like PAHs.54 Before going into detail about the application part, it is necessary to characterize the structures of the biosurfactant (Fig. 3).
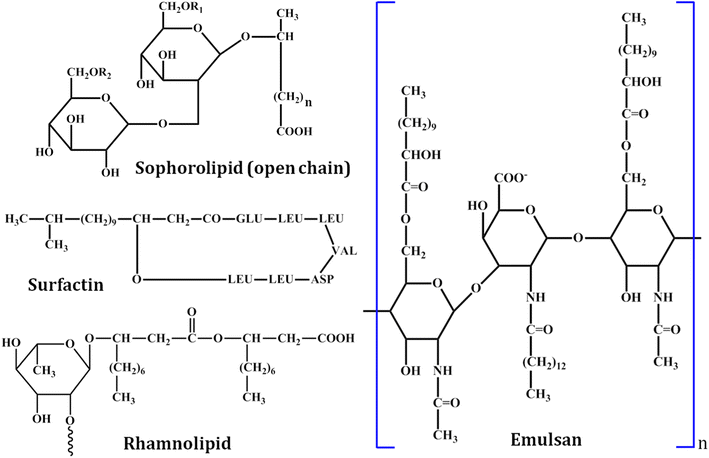 |
| Fig. 3 Chemical structures of selected biosurfactants: sophorolipid (open chain form), surfactin, rhamnolipid, and emulsan. | |
Based on their structural diversity, biosurfactants are classified into different categories, as mentioned earlier. Herein, inclusive information of the biosurfactants of various classes are summarized (Table 2) together with the microbial origins and related applications.
Table 2 Examples of the common biosurfactants, producing microbes and specific applications
Class |
Biosurfactant |
Microorganism |
Application |
Ref. |
Lipopeptides |
Surfactin |
Bacillus subtilis, Bacillus pumilus A |
Petroleum industry, environmental and agricultural applications |
55 and 56 |
Lichenysin |
Bacillus licheniformis |
Anti-adhesion activity |
57 and 58 |
Viscosin |
Pseudomonas fluorescens |
Antibacterial, antiviral, antitrypanosomal therapeutic |
59 |
Anikasin |
Pseudomonas fluorescens HKI0770 |
Used as amebicide, inhibit protozoan grazing |
60 |
Serrawettins |
Serratia marcescens |
Used in medical, pharmaceutical, agricultural and petroleum industries |
61 |
Glycolipids |
Rhamnolipids |
Pseudomonas aeruginosa, Pseudomonas putida |
Applied for environmental remediation |
62 |
Mannosylerythritol lipids |
Pseudozyma sp., Candida antartica, Ustilago maydis |
Repair of damaged hair, moisturization of dry skin, activation of fibroblast and papilla cells and protective effects in skin cells, and as an antioxidant |
63 |
Trehaloselipids |
Rhodococcus sp., Arthrobacter sp. |
Biomedical and industrial application in addition to bioremediation |
64 |
Sophorolipids |
Candida bombicola, Candida apicola, Candida batistae |
Amelioration of skin physiology, skin restructuring and repair |
65 |
Cellobiolipids |
Ustilago maydis |
Antifungal activity |
65 |
Phospholipids, neutral lipids, and fatty acids |
Phospholipid |
Thiobacillus thiooxidans |
Agents for respiratory failure |
66 |
Fatty acids |
Corynebacterium lepus |
Used as emulsifiers in food industries |
67 |
Neutral lipids |
N. erythropolis |
Partition the organic compound into a micellar core |
68 |
Polymeric |
Biodispersan |
A. calcoaceticus |
Prevent flocculation, fracturing of limestone, used in the paint industry |
69 |
Emulsan |
Acinetobacter calcoaceticus |
Hydrocarbon-in-water emulsions are stabilized |
70 |
Mannan protein emulsifiers |
Saccharomyces cerevisiae |
Stabilizes hydrocarbon in water emulsions |
71 |
Alasan |
Acinetobacter radioresistens |
Hydrocarbon-in-water emulsion stabilizer |
72 |
Siderophore |
Flavolipids |
Flavobacterium sp. |
Stable emulsifier used in remediation applications |
73 |
4. Biosurfactant assisted bioremediation of soil
Considering the severe risk factors for human health on account of soil pollution, there is an urgency to remove the intact pollutants from the soil media. The term “remediation” interprets elimination, deterioration or transformation of harmful contaminants into less detrimental chemicals. As revealed from the literature, the bioremediation approach is one of the best techniques74 established so far, continually employed from laboratory research to industry for exploring the biodegradation of contaminants. Soils are contaminated with a number of pollutants from hydrophobic organic compounds to emerging pollutants, including PAHs, POPs, toxic heavy metals, radionuclides, and so many other contaminants. In order to degrade or detoxify a wide variety of harmful compounds from the soil media using bioremediation techniques, the following factors are getting the major concern: (a) perfect assortment of microorganism, (b) maintaining favorable conditions (pH, aeration, temperature, etc.) for the remediation, (c) bioavailability of contaminants on spatial and temporal scales, and finally (d) the nature of the contaminants itself. In general, the biosurfactant influenced bioremediation method corresponds to in situ and ex situ pathways followed by the selection of biosurfactant addition (Fig. 4). The in situ methods involve the on-site generation of biosurfactant (soil flushing) while the ex situ methods require the introduction of externally synthesized biosurfactants into the contaminated site (soil washing).75 The introduction of externally synthesized biosurfactants is an existing approach which satisfactorily produces an excellent removal efficiency from laboratory to large industrial scales. Numerous biosurfactants, their employment in degrading or detoxifying distinct contaminants with a potential removal efficiency are described in the following sections.
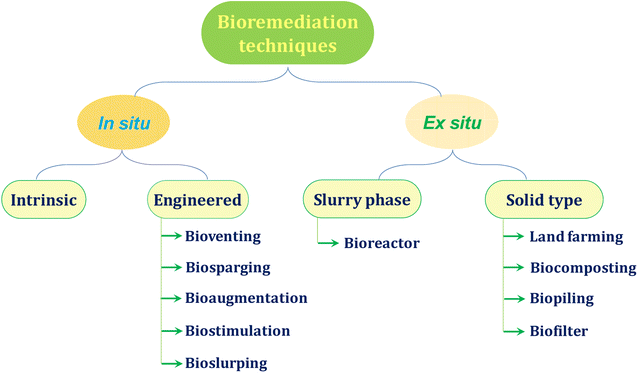 |
| Fig. 4 Bioremediation processes are mainly classified into two categories: in situ and ex situ. The in situ and ex situ processes have different pathways of bioremediation. | |
4.1 Biosurfactants used for the bioremediation of hydrocarbon contaminated soil
The lower solubility of hydrophobic PAH molecules accounts for their strong attachment with the soil matrix and hence it is tricky to remove them from soil media. A number of microorganisms generate natural surfactants which have a vibrant role in the biodegradation of contaminated PAHs and organic hydrophobic compounds from soil. Biologically derived surfactants significantly augment the rate of PAH biodegradation by increasing the bioavailability of hydrophobic substrates. Basically, hydrophobic organic compounds are not easily accessible, hence biologically produced surfactants improve the efficiency of desorption and accessibility of hydrophobic compounds so that organic pollutants become easily bio-accessible.76 Several researchers extensively investigated the potential activity of widely diverse microbial species exploited for PAH degradation. The biosurfactant rhamnolipid is a promising one in the degradation of hydrocarbons, as reported elsewhere (Table 3). Noticeably, bio-trickling saponin filters are involved in removing hexane.77 A pseudomonas strain-containing biofilm has also been shown to work as a (bio) filter for volatile organic chemicals, breaking down hexane and generating biosurfactants. Microorganisms that generate biosurfactants from hydrocarbons are vital in biofiltering because they can eliminate the pollution and prevent biomass collection.78 The degradation of a petroleum hydrocarbon from contaminated soil mainly depends on the useful step, the initial washing of soil by the biosurfactant.79 Soil washing, a practical approach in the removal of contaminants, proceeds through two mechanistic pathways: a soil roll-up mechanism and solubilization.80 It was observed that the biodegradation of PAHs increased as the concentration of the biosurfactant increased up to a threshold level. After this threshold level, the degradation efficiency of the biosurfactant reduces to some extent since a higher dose of biosurfactant interferes with the cellular membrane of the microbial species. Fig. 5 represents how the concentration of rhamnolipid influences the aqueous dispersion of hydrophobic organic compounds.81,82 Biosurfactants not only influence the solubility of organic contaminants but also control the surface hydrophobicity of the microbial cell. Again, the surface hydrophobicity of the cell drives cell adherence to hydrophobic organic compounds and cell-to-cell interactions.83
Table 3 The biosurfactant mediated remediation of organic contaminants from soil
Organic chemicals |
Microbial species and/or biosurfactant |
Removal efficiency (%) |
Ref. |
Phenanthrene (Phen) |
Pseudomonas stutzeri |
Phen: 86–95 |
83 |
Bacillus simplex |
Fluorine (F) |
Bacillus pumilus |
F: 65–86 |
Pyrene |
Pseudomonas aeruginosa SP4 |
84.6 |
84 |
Anthracene (A) |
Aeribacillus pallidus |
A: 92–96 |
85 |
Fluorene (F) |
Bacillus axarquiensis |
F: 83–86 |
Phenanthrene (Phen) |
Bacillus siamensis |
Phen: 16–54 |
Pyrene (P) |
Bacillus subtilis subsp. inaquosorum |
P: 51–71 |
Phenanthrene (Phen) |
Aeribacillus pallidus SL-1 |
Phen: 80 |
86 |
Pyrene (P) |
P: 50 |
n-Hexadecane (n-Hex) |
Pseudomonas aeruginosa san ai |
n-Hex: 80 |
87 |
n-Nonadecane (n-Dec) |
n-Dec: 98 |
Fluorene (F) |
F: 96 |
Phenanthrene (Phen) |
Phen: 50 |
Pyrene (P) |
P: 41 |
Pyrene |
Paenibacillus dendritiformis CN5 |
83.5 |
88 |
Gasoline |
Ludwigia octovalvis plantation and biosurfactant |
96.5 |
89 |
Total petroleum hydrocarbon (TPH) |
Maize plantation and rhamnolipid |
58 |
90 |
Motor oil |
Candida sphaerica-biosurfactant |
90 |
91 |
Petroleum hydrocarbon |
Bacillus subtilis A21-biosurfactant |
64.5 |
92 |
Diesel oil |
Staphylococcus epidermidis EVR4 |
84 |
93 |
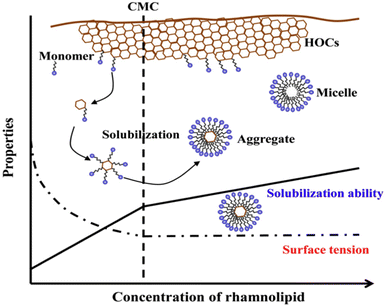 |
| Fig. 5 Schematic representation of rhamnolipid-enhanced aqueous dispersion of HOCs. Reprinted with permission from ref. 82 Copyright 2018 Elsevier. | |
In bioremediation processes of hydrophobic compounds from contaminated soil, the biosurfactants conduct three different modes of action based on their concentration and molecular weight.94 At lower concentrations (<CMC), the biosurfactant diminishes the surface and interfacial tension between soil–water and air–water systems. This reduction of interfacial force promotes the contact of biosurfactants with the soil-contaminant and thereby favors the mobilization of organic pollutants. When a sufficient amount of biosurfactant exists in the polluted soil matrix micellization occurs and those developed micelles can encapsulate the organic contaminant at the interior formed by the hydrophobic tail part of the biosurfactant molecules. Thus, the solubilization of hydrophobic organic compounds using the aggregated nanostructure of biosurfactants (above the CMC) is another mode of the bioremediation mechanism. These two mechanistic modes of the biosurfactant mediated remediation process are entirely based on the concentration of the employed biosurfactant having a low molecular weight (Fig. 6). When a biosurfactant of high molecular weight is used for soil bioremediation purposes, especially for the removal of hydrocarbon contaminated soil, then the emulsifying ability of the biosurfactant contributes the mechanistic role. A higher molecular weight biosurfactant encourages the biodegradation process following the solubilization and emulsification of organic hydrophobic contaminants.
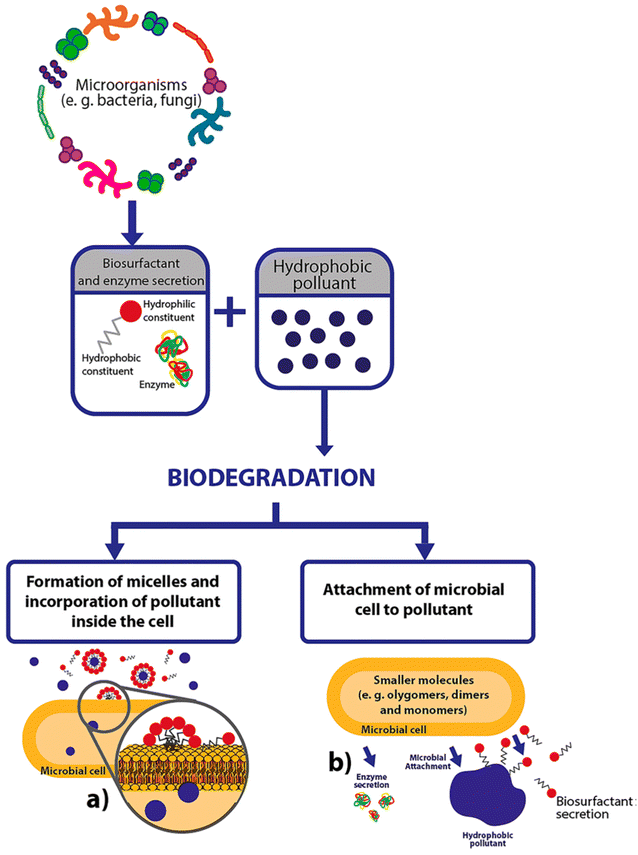 |
| Fig. 6 Probable mechanistic actions of biosurfactants in the microbial decay of hydrophobic pollutants from soil media: (a) generation of micelles and encapsulation of organic contaminants within the microbial cell and (b) microbial attachment of hydrophobic pollutants. Reprinted with permission from ref. 95. | |
In order to advance our knowledge of the mechanistic action of biosurfactants for the degradation of hydrophobic contaminants, additional research is actually needed to comprehend the actual interaction of biosurfactants with cells.96
4.2 Biosurfactants used for the bioremediation of heavy metals from soil
Metals can be readily accumulated in the soil media and thereby easily enter into the soil–food chain,97 which becomes a matter of concern to the entire environmentalist community and all other divisions of research. Soil microorganisms have a remarkable role on remediating these metallic toxins.98 Phytoremediation is a comparatively less destructive, environmentally friendly and cost-efficient remediation technique which allows soil clean-up over a large scale.99 In this context, plant growth promoting rhizobacteria, PGPR, assisting the remediation of toxic metal contaminated soil was reported to be an efficient bioremediation technique.100 In another recent study, the biomineralization technique was materialized when the microbial induced phosphate precipitation (MIPP) approach101 was shown to be feasible in the remediation of the potentially toxic ions. Providencia alcalifaciens strain 2EA was significantly active to remediate lead ions from lead contaminated soil through a biomineralization process for the inorganic phosphate.102 Pseudomonas putida and Leclercia adecarboxylata were proficient for the bioremediation of Pb2+ ions.103 Pseudomonas aeruginosa strain BS2 produced rhamnolipid biosurfactant successfully removed 92% of Cd and 88% of Pb from artificially contaminated soil.104 The greater potential of biosurfactant generated from the Candida tropicalis yeast was accounted for by the removal of Zn and Cu from polluted sand.105 The plant based green surfactant saponin in association with a microorganism generated rhamnolipid biosurfactant was introduced to treat cadmium contaminated soil; the maximum uptake of cadmium was 39.06 mg kg−1 of the rhamnolipid surfactant used in the phytoremediation pathway.106 The soil washing treatment is another approach for the bioremediation of contaminated soils containing more than one heavy metal. Better results were obtained when multiple biosurfactants, having affinities towards numerous heavy metals, were applied in a soil washing process. Such a kind of advancement in the bioremediation of more than one heavy metal from polluted soils was achieved with the employment of saponin, rhamnolipid and tannic acid.107 Rhamnolipid-sa1 alone was reported to be effective to reduce iron up to 60.34% from the contaminated soil.108 Another plant extracted biosurfactant, saponin, was believed to be efficient for removing heavy metals (Cu, Zn, Cr, Pb, Ni, Mn) using a sequential sludge washing method through the proposed mechanism in Fig. 7.109 Table 4 represents the impact of biosurfactants on the soil bioremediation of heavy metals with the corresponding removal efficiency. Biosurfactants can also stimulate heavy metals' mobilities along two separate paths—either through lowering the interfacial tension or by producing micelle like aggregates. On account of their amphiphilic nature, primarily at lower concentration, ZPC molecules adsorb at the interfaces of soil–water and metal–soil. This supports ameliorating soil wettability by minimizing the interfacial tension and strength of binding positively charged metal ions to soil materials. Subsequently, the heavy metal ions get complexed with a negatively charged biosurfactant, providing stabilization of the metal–biosurfactant conjugates. The strength of the metal–biosurfactant interaction is stronger than that of the metal–soil particle, leading to a more readily expulsion of the metal–biosurfactant complex from contaminated zones. Metal ions prefer to bind with oppositely charged particles or ions and exchange the equally charged ions (electrostatic interactions or ion exchange, eqn (1)).110 Ultimately, desorption of heavy metal ions takes place from the soil matrix and its complexation is noticed by the micelle like structure of the biosurfactant.111 A model mechanistic pathway is portrayed in Fig. 8, which represents the performance of a biosurfactant on heavy metal removal from contaminated soil.112 |
Soil–Mn+ + R–(COOH)m + H2O → R–O–Mn+–(COOH)m + 2H
| (1) |
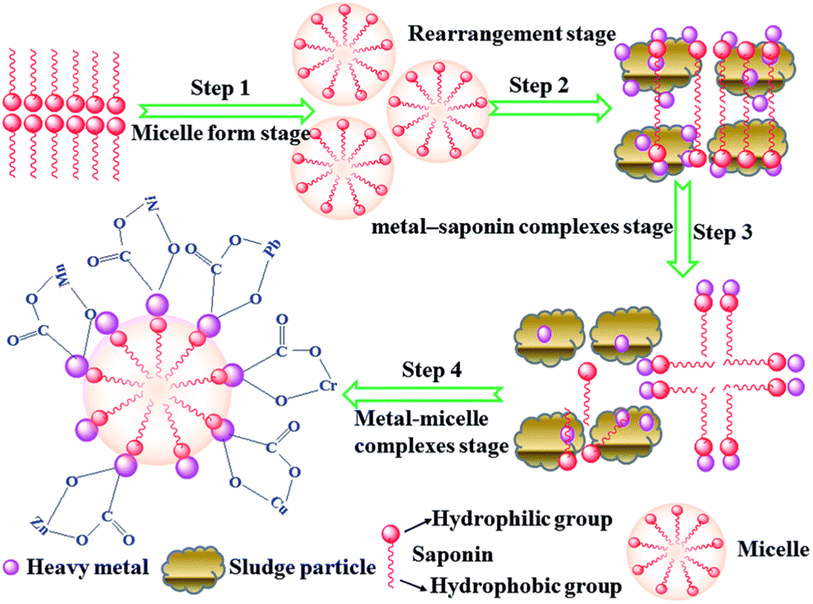 |
| Fig. 7 Mechanism of saponin assisted heavy metal removal from soil–sludge. Reprinted with permission from ref. 109 Copyright 2017 Royal Society of Chemistry. | |
Table 4 The biosurfactant enhances the remediation of heavy metals from contaminated soil
Heavy metals |
Bioremediator species and/or biosurfactant |
Removal efficiency (%) |
Ref. |
Cd |
Rhamnolipid and saponin |
ND |
113 |
Cr, Pb, Cd, Ni, Cu |
Di-rhamnolipid from Pseudomonas aeruginosa BS2 |
ND |
114 |
Fe |
Candida sphaerica |
95 |
115 |
Zn |
90 |
Pb |
79 |
Cu |
Rhamnolipid from Pseudomonas aeruginosa MTCC 2297 |
74 |
116 |
Cu |
Saponin |
60 |
109 |
Zn |
65 |
Cr |
67 |
Pb |
40 |
Ni |
57 |
Mn |
35 |
Ni |
Rhamnolipid and saponin |
87 |
117 |
Cr |
71 |
V |
70 |
Cd |
Rhamnolipid with Citric acid |
76.4 |
118 |
Pb |
28.1 |
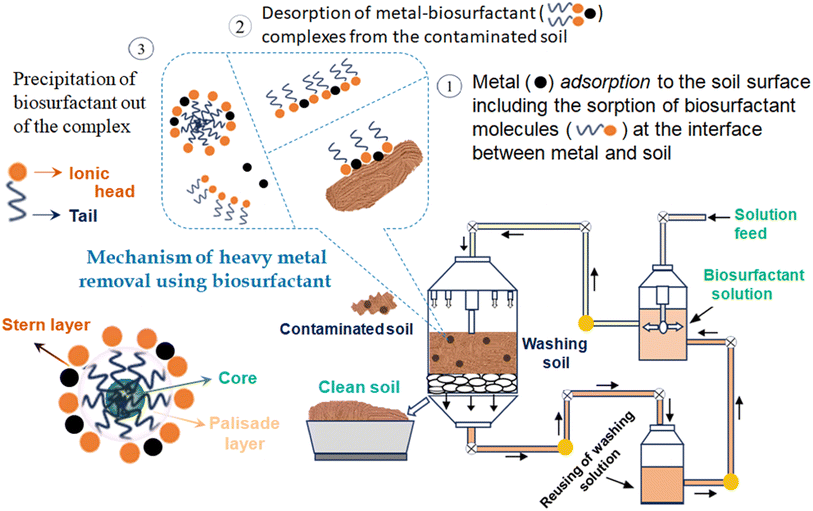 |
| Fig. 8 Technique for heavy metal removal from contaminated soil using biosurfactants and the obtained removal rates for Cu, Zn, Cr, and Cd of 90–100% in normal conditions. | |
It is indispensable to have much skill and experience about the origin, chemical structure and resulting properties of the biosurfactants to realize the exact mechanisms of biosurfactant activity in soil bioremediation. The mechanisms of bioremediation are connected with the complicated interactions between microorganisms, biosurfactant, pollutant and soil, as demonstrated in the following section.
4.3 Biosurfactants used for bioremediation of emerging pollutants from soil
Bioremediation is an emerging technology by which environmental restoration can be easily achieved following the detoxification of emerging pollutants blended with the soil matrix, as discussed in several reports.119–122 Biosurfactant plays a physiological role in increasing the bioavailability of soil bound emerging pollutants. The biosurfactant assisted removal of emerging organic contaminants occurs through two steps: mobilization and solubilization. Recently, the degradation of organophosphorus pesticides (e.g., Phox) from tainted soil using a plant growth promoting rhizobacteria, Bacillus amyloliquefaciens YP6, was successfully investigated.123,124 In another study, phenanthrene and imidacloprid were significantly removed by a pesticide degrading microbe, Bacillus thuringiensis isolated from marine sediment.125 Recent investigations regarding the role of biosurfactant on the bioremediation of emerging and predominating pollutants are encapsulated in Table 5. Recently, a Lysinibacillus sphaericus IITR51 strain generated rhamnolipid was reported to enhance the solubility of hydrophobic pesticides and thereby increase the bioavailability of organic chemicals.126
Table 5 Biosurfactant-promoted bioremediation of soils contaminated with emerging pollutants
Pollutants |
Microbial species and/or biosurfactant |
Removal efficiency (%) |
Ref. |
Triclosan |
Soil indigenous microbes and rhamnolipid |
94 |
127 |
DDT |
Arthrobacter globiformis and rhamnolipid |
64.3 |
128 |
Carbendazim |
Rhodococcus sp. D-1 and rhamnolipid |
97.3 |
129 |
Endosulfan |
Mixed bacterial culture |
99 |
130 |
α- and β-endosulfan |
Natural surfactant extracted from mesquite seed and guar gums |
65–94 (for α-) |
131 |
41–80 (for β-) |
Diethyl phthalate |
Pseudomonas sp. DNE-S1 produced biosurfactant |
97.8 |
132 |
Atrazine |
Bacillus velezensis MHNK1 produced surfactin |
100 |
133 |
Quinalphos |
Pseudomonas aeruginosa Q10 |
94 |
134 |
β-Cypermethrin |
Pseudomonas aeruginosa CH7 |
90 |
135 |
Hexachlorocyclohexane |
C. striatus plantation and Rhodococcus erythropolis ET54b |
33 |
136 |
Epoxiconazole and fludioxonil |
Pseudomonas sp., Ochrobactrum sp. and Comamonas sp. |
56 |
137 |
Cyprodinil |
Acinetobacter sp. |
78 |
138 |
Indeed, a biosurfactant molecule can enter the cellular phospholipid membrane and alter its fluidity and permeability.
Biosurfactants assist the adsorption of pollutants by amplifying the permeability of the bacterial cell membrane through:
• An adsorption process at the outer leaflet.
• Shifting to the inner membrane.
• Insertion between the phospholipid bilayer.
The membrane fluidity of a cell is also altered by biosurfactants when the proportion of saturated to unsaturated fatty acids in the lipid bilayer diminishes. The larger the quantity of unsaturated fatty acids in the membrane, the higher is its fluidity. A better fluidity signifies an effective transportation of hydrophobic organic compounds through the membranes of a microbial cell.
Finally, the function of biosurfactants in soil bioremediation processes is represented in Fig. 9.122
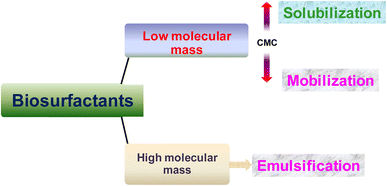 |
| Fig. 9 Functions of biosurfactants in the remediation of various soil pollutants. | |
5. Comparison of biosurfactants with synthetic surfactants for purposes of soil bioremediation
Solubilization utilizing surfactants at concentrations above their CMC values has been extensively researched in in situ soil washing.139 It is thought that nonionic surfactants, like triton, tween, and brij, which have a high hydrophobicity, are excellent for promoting the dissolution of hydrophobic organics in soil. However, it was demonstrated for nonionic surfactants that the desorption efficiency of petroleum hydrocarbons was not significantly impacted by the increase in surfactant concentration from 2× to 10× CMC.140 At surfactant concentrations above the CMC, the biodegradation of contaminants in soil systems has also been observed to be inhibited. Moreover, a lot of regularly used synthetic surfactants are poorly biodegradable and toxic, and their use may cause an accumulation of environmentally hazardous substances in soil.141 Biosurfactants made by microorganisms have recently discovered a new use in environmental clean-up procedures. In comparison to synthetic surfactants, biosurfactants have a number of distinct advantages, such as biocompatibility and biodegradability, multifunctional properties, a stable activity under extreme environmental conditions (such as high or low temperature and high pressure, pH and salinity), and can therefore be more successful in the remediation of contaminated soil.142–144 In earlier experimental studies, the effectiveness of synthetic and bio-surfactants in the soil bioremediation process was reported. For example:
(a) Rhamnolipid and surfactin, two biosurfactants, were compared to synthetic surfactants (Tween 80 and Triton X-100) for the removal of petroleum hydrocarbons from damaged soils. Rhamnolipids, surfactin, and synthetic surfactants like Tween 80 and Triton X-100 were all utilized at 0.2% mass each. Findings indicated that total petroleum hydrocarbons could be removed from polluted soil by 23%, 14%, 6%, and 4%, respectively. This demonstrated how much more effective biosurfactants are than synthetic surfactants.145
(b) SDS's capacity for removing substances was contrasted with that of biosurfactants, like saponin and rhamnolipid. The study revealed that SDS was more effective than rhamnolipid and saponin at removing crude oil from the soil. Different surfactants, however, have varying degrees of affinity for crude oil's constituents. For instance, saponin and SDS interact well with aromatic hydrocarbons while SDS is better at removing aliphatic hydrocarbons. SDS is more expensive and less biodegradable than biosurfactants but is more efficient.146
(c) In contrast to Triton X-100 (synthetic surfactant), which is non-biodegradable under anaerobic conditions and only partially biodegradable under aerobic conditions (soluble COD removal efficiency of 47.1% after 10 days at concentrations below 900 mg L−1), rhamnolipid biosurfactants are biodegradable under both aerobic and anaerobic conditions (soluble COD removal efficiency of 74% after 10 days and 47.2% after 6 days, respectively).147
Considering the benefit of microbial surfactants, including easy synthesis from renewable feed stocks, biodegradability, low toxicity, and higher foaming ability, over those of the synthetic surfactants suggests a high demand in a variety of industries. So biosurfactants appeared as the best candidate to replace the preexisting commercially available synthetic surfactants. However, there are various challenges in the synthesis, isolation, purification, characterization and use of them, especially at the commercial level. Extraction of green surfactants from plants and microbial species is a very costly and time-consuming process, since a mixture of numerous species are present together.148 Thus, a few critical limits of biosurfactants need to be revealed in context of soil bioremediation for the generation of healthy future.
6. Critical limits and future prospect of biosurfactants
Biosurfactant assisted technology is not very appropriate for the high scale remediation of organic contaminants; indeed, biosurfactants produce a synergistic effect with the native microbial community. Two major critical boundaries of biosurfactants are required to be resolved before establishing a practical application. Preliminary, the production and isolation of biosurfactants by maximum strains is reasonably small and needs to be highly elevated. Normally, the development conditions of bacteria are optimized. In this regard, it has been reported that Bacillus amyloliquefaciens A3 exhibits a satisfactory yield of biosurfactants. The mutant 1–24 of Bacillus amyloliquefaciens A3 supplied an important direction to the bioremediation of petroleum hydrocarbon-based contaminants from a practical standpoint. Bioremediation of in situ contaminated soil by Bacillus amyloliquefaciens A3 follows through ARTP mutagenesis.149
However, some methods, such as engineering bacterial growth and bacterial mutagenesis, are mostly applied to further progress. These bacteria are prepared for the bioremediation of petroleum-based hydrocarbons. The second-most issue that needs to be settled requires a special analysis which accounts for the evaluation of the potential efficiency by applying biosurfactants to decontaminate petroleum hydrocarbon contaminated soils.
7. Conclusion
Soil is the most fundamental but complex component that secures food safety and human health. It retains a key function in protecting the environment to safeguard and sustain the ecological balance. Soil health restoration is a great issue since soil pollution is a hidden danger below our feet. So, needless to say that now an environmentally friendly remediation technology is important for society. One of the recent symposiums (GSOP18), held in 2018 with a theme song “It is time to fight soil pollution: be the solution to soil pollution,” was addressed for a consciousness about the urgency of the conservation of soils. Bioremediation is comparatively a smarter tool to fight soil pollutants since it deals with the biological treatment of contaminants. In this approach, a number of microorganisms that produce biologically derived surfactants are significantly utilized to break down hazardous organic materials. Microbial species increase the rate of the biodegradation process through mobilization and solubilization of contaminants, resulting in a substantial detoxification of soil. Biosurfactant-enhanced soil washing is a well-recognized permanent treatment for soils contaminated with PAHs, heavy metals, and other organic compounds.
Soil bioremediation technologies can be executed more effectively by the following approach:
(a) Mixed culture media can be more adjuvant to soil polluted with multiple hazardous substances.
(b) Biosurfactants derived from plant together with microbial species can be more efficacious than employing them separately for the bioremediation of mixed contaminants.
(c) Integrated treatment can be another way of remediation and reclamation of land contaminated with hazardous materials.
(d) Requirement to advance biosurfactant based detoxification and biodegradation techniques through the exploitation of genetically engineered microbes for extremely contaminated sites.
The cost and availability of these compounds, however, pose the biggest challenge to the employment of biosurfactants in the bioremediation of soil. Therefore, more cost-effective, eco-friendly technologies are unquestionably necessary to address the present issue, since the contaminants' type and extent are always changing, creating new problems and raising a permanent risk to the entire ecosystem. On a practical level, there is a significant distinction between the laboratory setting and the actual remediation of oil-contaminated soil site restoration. In fact, it is exceedingly challenging for laboratory results of remediation to be manifested and implemented into the actual remediation field. Nevertheless, on account of the complexity issues of the reality provisos, the diversity in the type of different pollutants, the unequal distribution of contaminants and the variation of soil pollution extent, an individual remediation technique cannot indulge all types of contaminated land.
Author contributions
BS contributed to the conceptualization and supervision. PS contributed to the writing – original draft. AG and SK contributed to the writing – review and editing. All authors contributed to the article and approved the submitted version.
Conflicts of interest
The authors declare no competing financial interests.
Acknowledgements
The authors are thankful and do acknowledge their respective organizations for extending adequate resources and facility support.
References
- A. Zwolak, M. Sarzynska, E. Szpyrka and K. Stawarczyk, Sources of Soil Pollution by Heavy Metals and Their Accumulation in Vegetables: A Review, Water, Air, Soil Pollut., 2019, 230, 164 CrossRef.
- P. Baweja, S. Kumar and G. Kumar, Fertilizers and Pesticides: Their Impact on Soil Health and Environment, in Soil Health. Soil Biology, ed. B. Giri and A. Varma, Springer, Cham, 2020, vol. 59, pp. 265–285 Search PubMed.
- N. Rodríguez-Eugenio, M. McLaughlin and D. Pennock, Soil Pollution: A Hidden Reality, FAO, Rome, 2018 Search PubMed.
- S. Mishra, R. N. Bharagava, N. More, A. Yadav, S. Zainith, S. Mani and P. Chowdhary, Heavy metal contamination: an alarming threat to environment and human health, in Environmental Biotechnology: For Sustainable Future, ed. R. C. Sobti, N. K. Arora and R. Kothari, Springer Singapore, 2019, pp. 103–125 Search PubMed.
- N. M. Aljamali, A. M. Jawad, A. J. Alabbasy and L. A. M. Salih, A Literature Review on Types of Contamination (Biological, Chemical, Medical), IJGC, 2019, 5, 7–14 Search PubMed.
- E. Morillo and J. Villaverde, Advanced technologies for the remediation of pesticide-contaminated soils, Sci. Total Environ., 2017, 586, 576–597 CrossRef CAS PubMed.
- S. Guo, F. Li, P. Li, S. Wang, Q. Zhao, G. Li, B. Wu and P. Tai, Study on Remediation Technologies of Organic and Heavy Metal Contaminated Soils, in Twenty Years of Research and Development on Soil Pollution and Remediation in China, ed. Y. Luo and T.C. Tu, Springer, Singapore, 2018 Search PubMed.
- Y. Lv, J. Bao and L. Zhu, A comprehensive review of recent and perspective technologies and challenges for the remediation of oil-contaminated sites, Energy Rep., 2022, 8, 7976–7988 CrossRef.
- C. C. Azubuike, C. B. Chikere and G. C. Okpokwasili, Bioremediation techniques–classification based on site of application: principles, advantages, limitations and prospects, World J. Microbiol. Biotechnol., 2016, 32, 180 CrossRef PubMed.
- S. Rajendran, T. A. K. Priya, K. S. Khoo, T. K. A. Hoang, H.-S. Ng, H. S. H. Munawaroh, C. Karaman, Y. Orooji and P. L. Show, A critical review on various remediation approaches for heavy metal contaminants removal from contaminated soils, Chemosphere, 2022, 287, 132369 CrossRef CAS PubMed.
- S. Ye, G. Zeng, H. Wu, C. Zhang, J. Dai, J. Liang, J. Yu, X. Ren, H. Yi, M. Cheng and C. Zhang, Biological technologies for the remediation of co-contaminated soil, Crit. Rev. Biotechnol., 2017, 37, 1062–1076 CrossRef CAS PubMed.
- X. Mao, R. Jiang, W. Xiao and J. Yu, Use of surfactants for the remediation of contaminated soils: a review, J. Hazard. Mater., 2015, 285, 419–435 CrossRef CAS PubMed.
- C. M. Martínez-Escudero, I. Garrido, C. Ros, P. Flores, P. Hellín, F. Contreras and J. Fenoll, Remediation of pesticides in commercial farm soils by solarization and ozonation techniques, J. Environ. Manage., 2023, 329, 117062 CrossRef PubMed.
- J. Qu, R. Liu, X. Bi, Z. Li, K. Li, Q. Hu, X. Zhang, G. Zhang, S. Ma and Y. Zhang, Remediation of atrazine contaminated soil by microwave activated persulfate system: performance, mechanism and DFT calculation, J. Clean. Prod., 2023, 399, 136546 CrossRef CAS.
- A. R. Markande, D. Patel and S. Varjani, A review on biosurfactants: properties, applications and current developments, Bioresour. Technol., 2021, 330, 124963 CrossRef CAS PubMed.
- D. S. Pardhi, R. R. Panchal, V. H. Raval, R. G. Joshi, P. Poczai, W. H. Almalki and K. N. Rajput, Microbial surfactants: a journey from fundamentals to recent advances, Front. Microbiol., 2022, 13, 982603 CrossRef PubMed.
- S. Mishra, Z. Lin, S. Pang, Y. Zhang, P. Bhatt and S. Chen, Biosurfactant is a powerful tool for the bioremediation of heavy metals from contaminated soils, J. Hazard. Mater., 2021, 418, 126253 CrossRef CAS PubMed.
- V. S. Nagtode, C. Cardoza, H. K. A. Yasin, S. N. Mali, S. M. Tambe, P. Roy, K. Singh, A. Goel, P. D. Amin, B. R. Thorat, J. N. Cruz and A. P. Pratap, Green Surfactants (Biosurfactants): A Petroleum-Free Substitute for Sustainability-Comparison, Applications, Market, and Future Prospects, ACS Omega, 2023, 8, 11674–11699 CrossRef CAS PubMed.
- S. O. Badmus, H. K. Amusa, T. A. Oyehan and T. A. Saleh, Environmental risks and toxicity of surfactants: overview of analysis, assessment, and remediation techniques, Environ. Sci. Pollut. Res., 2021, 28, 62085–62104 CrossRef CAS PubMed.
- T. A. Saleh, Surface Science of Adsorbents and Nanoadsorbents: Properties and Applications in Environmental Remediation, 2022, ISBN: 9780128498767 Search PubMed.
- C. E. Drakontis and S. Amin, Biosurfactants: formulations, properties, and applications, Curr. Opin. Colloid Interface Sci., 2020, 48, 77–90 CrossRef CAS.
- N. T. Khan, N. Jameel and M. J. Khan, A brief overview of contaminated soil remediation methods, Indian J. Biotechnol., 2018, 14, 168–171 Search PubMed.
- S. Sun, V. Sidhu, Y. Rong and Y. Zheng, Pesticide Pollution in Agricultural Soils and Sustainable Remediation Methods: A Review, Curr. Pollut. Rep., 2018, 4, 240–250 CrossRef CAS.
- M. W. Aktar, D. Sengupta and A. Chowdhury, Impact of pesticides use in agriculture: their benefits and hazards, Arabian J. Chem., 2009, 2, 1–12 CrossRef.
- H. I. Eldos, N. Zouari, S. Saeed and M. A. Al-Ghouti, Recent advances in the treatment of PAHs in the environment: application of nanomaterial-based technologies, Arab. J. Chem., 2022, 15, 103918 CrossRef CAS.
- M. A. Ashraf, Persistent organic pollutants (POPs): a global issue, a global challenge, Environ. Sci. Pollut. Res., 2017, 24, 4223–4227 CrossRef PubMed.
- O. M. L. Alharbi, A. A. Basheer, R. A. Khattab and I. Ali, Health and environmental effects of persistent organic pollutants, J. Mol. Liq., 2018, 263, 442–453 CrossRef CAS.
- http://chm.pops.int/TheConvention/ThePOPs/ListingofPOPs/tabid/2509/Default.aspx.
- K. Skordas and A. Kelepertsis, Soil contamination by toxic metals in the cultivated region of Agia, Thessaly, Greece. Identification of sources of contamination, Environ. Geol., 2005, 48(4), 615–624 CrossRef CAS.
- G. Zhang, J. Bai, R. Xiao, Q. Zhao, J. Jia, B. Cui and X. Liu, Heavy metal fractions and ecological risk assessment in sediments from urban, rural and reclamation-affected rivers of the Pearl River Estuary, China, Chemosphere, 2017, 184, 278–288 CrossRef CAS PubMed.
- C. Li, K. Zhou, W. Qin, C. Tian, M. Qi, X. Yan and W. Han, A Review on Heavy Metals Contamination in Soil: Effects, Sources, and Remediation Techniques, Soil and Sediment Contamination, Int. J., 2019, 28, 380–394 CAS.
- M. I. Ojovan, W. E. Lee and S. N. Kalmykov, An introduction to nuclear waste immobilisation, Elsevier, 2019 Search PubMed.
- S. Dragović, Artificial neural network modeling in environmental radioactivity studies – A review, Sci. Total Environ., 2022, 847, 157526 CrossRef PubMed.
- T. Damstra, Potential Effects of Certain Persistent Organic Pollutants and Endocrine Disrupting Chemicals on the Health of Children, J. Toxicol., Clin. Toxicol., 2002, 40, 457–465 CrossRef CAS PubMed.
- M. J. Fabiańska, B. Kozielska, J. Konieczyński and A. Kowalski, Sources of organic pollution in particulate matter and soil of Silesian Agglomeration (Poland): evidence from geochemical markers, Environ. Geochem. Health, 2016, 38, 821–842 CrossRef PubMed.
- W. Xu, X. Wang and Z. Cai, Analytical chemistry of the persistent organic pollutants identified in the Stockholm Convention: A review, Anal. Chim. Acta, 2013, 790, 1–13 CrossRef CAS PubMed.
- J. L. Barber, A. J. Sweetman, D. van Wijk and K. C. Jones, Hexachlorobenzene in the global environment: emissions, levels, distribution, trends and processes, Sci. Total Environ., 2005, 349, 1–44 CrossRef CAS PubMed.
- J. Vijgen, P. C. Abhilash, Y. F. Li, R. Lal, M. Forter, J. Torres, N. Singh, M. Yunus, C. Tian, A. Schaffer and R. Weber, Hexachlorocyclohexane (HCH) as new Stockholm Convention POPs—a global perspective on the management of Lindane and its waste isomers, Environ. Sci. Pollut. Res., 2011, 18, 152–162 CrossRef CAS PubMed.
- B. J. Alloway, Heavy Metals in Soils: Trace Metals and Metalloids in Soils and their Bioavailability, Environmental Pollution, Springer, Netherlands, 3rd edn, 2013 Search PubMed.
- J. Jagne, D. White and F. Jefferson, Endocrine-Disrupting Chemicals: Adverse Effects of Bisphenol A and Parabens to Women's Health, Water, Air, Soil Pollut., 2016, 227, 182 CrossRef.
- S. Akbari, N. H. Abdurahman, R. M. Yunus, F. Fayaz and O. R. Alara, Biosurfactants—a new frontier for social and environmental safety: a mini review, Biotechnol. Res. Innov., 2018, 2, 81–90 CrossRef.
- R. Marchant and I. M. Banat, Microbial biosurfactants: challenges and opportunities for future exploitation, Trends Biotechnol., 2012, 30, 558–565 CrossRef CAS PubMed.
- R. Marchant and I. M. Banat, Biosurfactants: a sustainable replacement for chemical surfactants?, Biotechnol. Lett., 2012, 34, 1597–1605 CrossRef CAS PubMed.
- I. W. Hamley, Lipopeptides: from self-assembly to bioactivity, Chem. Commun., 2015, 51, 8574–8583 RSC.
- J. Yu, N. Grossiord, C. E. Koning and J. Loos, Controlling the dispersion of multi-wall carbon nanotubes in aqueous surfactant solution, Carbon, 2007, 45, 618–623 CrossRef CAS.
- D. K. F. Santos, R. D. Rufino, J. M. Luna, V. A. Santos and L. A. Sarubbo, Biosurfactants: Multifunctional Biomolecules of the 21st Century, Int. J. Mol. Sci., 2016, 17, 401 CrossRef PubMed.
- B. Gupta, S. Puri, I. S. Thakur and J. Kaur, Enhanced pyrene degradation by a biosurfactant producing Acinetobacter baumannii BJ5: growth kinetics, toxicity and substrate inhibition studies, Environ. Technol. Innovation, 2020, 19, 100804 CrossRef.
- M. A. Pele, D. R. Ribeaux, E. R. Vieira, A. F. Souza, M. A. C. Luna, D. M. Rodríguez, R. F. S. Andrade, C. Sales-Alviano, D. Sales-Alviano, E. Barreto-Bergter, A. L. C. M. A. Santiago and G. M. Campos-Takaki, Conversion of renewable substrates for biosurfactant production by Rhizopus arrhizus UCP 1607 and enhancing the removal of diesel oil from marine soil, Electron. J. Biotechnol., 2019, 38, 40–48 CrossRef CAS.
- M. M. Müller, J. H. Kügler, M. Henkel, M. Gerlitzki, B. Hörmann, M. Pöhnlein, C. Syldatk and R. Hausmann, Rhamnolipids—Next generation surfactants?, J. Biotechnol., 2012, 162, 366–380 CrossRef PubMed.
- L. Luft, T. C. Confortin, I. Todero, G. L. Zabot and M. A. Mazutti, An overview of fungal biopolymers: bioemulsifiers and biosurfactants compounds production, Crit. Rev. Biotechnol., 2020, 40, 1059–1080 CrossRef CAS PubMed.
- A. A. Jimoh, T. Y. Senbadejo, R. Adeleke and J. Lin, Development and Genetic Engineering of Hyper-Producing Microbial Strains for Improved Synthesis of Biosurfactants, Mol. Biotechnol., 2021, 63, 267–288 CrossRef CAS PubMed.
- L. Fernandes-Moutinho, F. Ramalho-Moura, R. Carvalho-Silvestre and A. S. Romão-Dumaresq, Microbial biosurfactants: a broad analysis of properties, applications, biosynthesis, and techno-economical assessment of rhamnolipid production, Biotechnol. Prog., 2021, 37, e3093 CrossRef PubMed.
- V. Rangarajan and M. Narayanan, Biosurfactants in Soil Bioremediation, in Advances in Soil Microbiology: Recent Trends and Future Prospects. Microorganisms for Sustainability, ed. T. Adhya, B. Lal, B. Mohapatra, D. Paul and S. Das, Springer, Singapore, 2018, vol. 3, pp. 193–204 Search PubMed.
- R. S. Makkar and K. J. Rockne, Comparison of synthetic surfactants and biosurfactants in enhancing biodegradation of polycyclic aromatic hydrocarbons, Environ. Toxicol. Chem., 2003, 22, 2280 CrossRef CAS PubMed.
- M. Geissler, K. Morabbi Heravi, M. Henkel and R. Hausmann, Lipopeptide biosurfactants from Bacillus species, in Biobased Surfactants: Synthesis, Properties, and Applications, ed. D. Hayes, D. K. Solaiman and R. D. Ashby, Elsevier Science, London, 2nd edn, 2019, pp. 205–240 Search PubMed.
- W.-C. Chen, R.-S. Juang and Y.-H. Wei, Applications of a lipopeptide biosurfactant, surfactin, produced by microorganisms, Biochem. Eng. J., 2015, 103, 158–169 CrossRef CAS.
- L. Fracchia, M. Cavallo, M. G. Martinotti and I. M. Banat, Biosurfactants and Bioemulsifiers Biomedical and Related Applications—Present Status and Future Potentials, Biomedical Science, Engineering and Technology, ed. D. N. Ghista, InTech., 2012, vol. 14, pp. 325–370, ISBN: 978-953-307-471-9 Search PubMed.
- J. Coronel-Leon, A. M. Marques, J. Bastida and A. Manresa, Optimizing the production of the biosurfactant lichenysin and its application in biofilm control, J. Appl. Microbiol., 2015, 120, 99–111 CrossRef PubMed.
- M. V. Laycock, P. D. Hildebrand, P. Thibault, J. A. Walter and J. L. C. Wright, Viscosin, a potent peptidolipid biosurfactant and phytopathogenic mediator produced by a pectolytic strain of Pseudomonas fluorescens, J. Agric. Food Chem., 1991, 39, 483–489 CrossRef CAS.
- S. Gotze, R. Herbst-Irmer, M. Klapper, H. Görls, K. R. A. Schneider, R. Barnett, T. Burks, U. Neu and P. Stallforth, Structure, Biosynthesis, and Biological Activity of the Cyclic Lipopeptide Anikasin, ACS Chem. Biol., 2017, 12, 2498–2502 CrossRef PubMed.
- T. Clements, T. Ndlovu, S. Khan and W. Khan, Biosurfactants produced by Serratia species: classification, biosynthesis, production and application, Appl. Microbiol. Biotechnol., 2019, 103, 589–602 CrossRef CAS PubMed.
- G. Liu, H. Zhong, X. Yang, Y. Liu, B. Shao and Z. Liu, Advances in applications of rhamnolipids biosurfactant in environmental remediation: a review, Biotechnol. Bioeng., 2018, 115, 796–814 CrossRef CAS PubMed.
- L. Fracchia, C. Ceresa, A. Franzetti, M. Cavallo, I. Gandolfi, J. Van Hamme, P. Gkorezis, R. Marchant and I. M. Banat, Industrial Applications of Biosurfactants. Biosurfactants: Production and Utilization-Processes, Technologies, and Economics, in Surfactant Science Series 159, ed. N. Kosaric and F. V. Sukan, CRC press, 2014, vol. 12, pp. 245–260 Search PubMed.
- A. Franzetti, I. Gandolfi, G. Bestetti, T. J. P. Smyth and I. M. Banat, Production and applications of trehalose lipid biosurfactants, Eur. J. Lipid Sci. Technol., 2010, 112, 617 CrossRef CAS.
- B. Teichmann, U. Linne, S. Hewald, M. A. Marahiel and M. Bolker, A biosynthetic gene cluster for a secreted cellobiose lipid with antifungal activity from Ustilago maydis, Mol. Microbiol., 2007, 66, 525–533 CrossRef CAS PubMed.
- S. Shekhar, A. Sundaramanickam and T. Balasubramanian, Biosurfactant Producing Microbes and their Potential Applications: A Review, Crit. Rev. Environ. Sci. Technol., 2014, 45, 1522–1554 CrossRef.
- D. G. Cooper, J. E. Zajic and C. Denis, Surface active properties of a biosurfactant fromCorynebacterium lepus, J. Am. Oil Chem. Soc., 1981, 58, 77–80 CrossRef CAS.
- A. J. Park, D. K. Cha and T. M. Holsen, Enhancing solubilization of sparingly soluble organic compounds by biosurfactants produced by Nocardia erythropolis, Water Environ. Res., 1998, 70, 351 CrossRef CAS.
- A. Elkeles, E. Rosenberg and E. Z. Ron, Production and Secretion of the Polysaccharide Biodispersan of Acinetobacter calcoaceticus A2 in Protein Secretion Mutants, Appl. Environ. Microbiol., 1994, 60, 4642–4645 CrossRef CAS PubMed.
- I. M. Banat, A. Franzetti, I. Gandolfi, G. Bestetti, M. G. Martinotti, L. Fracchia, T. J. Smyth and R. Marchant, Microbial biosurfactants production, applications and future potential, Appl. Microbiol. Biotechnol., 2010, 87, 427–444 CrossRef CAS PubMed.
- D. R. Cameron, D. G. Cooper and R. J. Neufeld, The mannoprotein of Saccharomyces cerevisiae is an effective bioemulsifier, Appl. Environ. Microbiol., 1988, 54, 1420–1425 CrossRef CAS PubMed.
- A. Toren, S. Navon-Venezia, E. Z. Ron and E. Rosenberg, Emulsifying Activities of Purified Alasan Proteins from Acinetobacter radioresistens KA53, Appl. Environ. Microbiol., 2001, 67, 1102–1106 CrossRef CAS PubMed.
- A. A. Bodour, C. Guerrero-Barajas, B. V. Jiorle, M. E. Malcomson, A. K. Paull, A. Somogyi, L. N. Trinh, R. B. Bates and R. M. Maier, tructure and Characterization of Flavolipids, a Novel Class of Biosurfactants Produced by Flavobacterium sp. Strain MTN11, Appl. Environ. Microbiol., 2004, 70, 114–120 CrossRef CAS PubMed.
- M. Megharaj, B. Ramakrishnan, K. Venkateswarlu, N. Sethunathan and R. Naidu, Bioremediation approaches for organic pollutants: a critical perspective, Environ. Int., 2011, 37, 1362–1375 CrossRef CAS PubMed.
- A. A. P. Selva Filho, A. Converti, R. C. F. S. da Silva and L. A. Sarubbo, Biosurfactants as Multifunctional Remediation Agents of Environmental Pollutants Generated by the Petroleum Industry, Energies, 2023, 16, 1209 CrossRef CAS.
- F. A. Bezza and E. M. Nkhalambayausi-Chirwa, Desorption kinetics of polycyclic aromatic hydrocarbons (PAHs) from contaminated soil and the effect of biosurfactant supplementation on the rapidly desorbing fractions, Biotechnol. Biotechnol. Equip., 2015, 29, 680–688 CrossRef CAS.
- Y. Tu, C. Yang, Y. Cheng, G. Zeng, L. Lu and L. Wang, Effect of saponins on nhexane removal in biotrickling filters, Bioresour. Technol., 2015, 175, 231–238 CrossRef CAS PubMed.
- S. He, Y. Ni, L. Lu, Q. Chai, T. Yu, Z. Shen and C. Yang, Simultaneous degradation of n-hexane and production of biosurfactants by Pseudomonas sp. strain NEE2 isolated from oil-contaminated soils, Chemosphere, 2020, 242, 125237 CrossRef CAS PubMed.
- I. O. Olasanmi and R. W. Thring, Evaluating rhamnolipid-enhanced washing as a first step in remediation of drill cuttings and petroleum-contaminated soils, J. Adv. Res., 2020, 21, 79–90 CrossRef CAS PubMed.
- X. Mao, J. Rui, X. Wei and J. Yu, Use of surfactants for the remediation of contaminated soils: a review, J. Hazard. Mater., 2015, 285, 419–435 CrossRef CAS PubMed.
- S. Gaur, A. Sahani, P. Chattopadhyay, S. Gupta and A. Jain, Remediation of Waste Engine Oil Contaminated Soil using Rhamnolipid based Detergent Formulation, Mater. Today: Proc., 2023, 77, 31–38 CrossRef CAS.
- Z. Zeng, Y. Liu, H. Zhong, R. Xiao, G. Zeng, Z. Liu, M. Cheng, C. Lai, C. Zhang, G. Liu and L. Qin, Mechanisms for rhamnolipids-mediated biodegradation of hydrophobic organic compounds, Sci. Total Environ., 2018, 634, 1–11 CrossRef CAS PubMed.
- S. Rabodonirina, R. Rasolomampianina, F. Krier, D. Drider, D. Merhaby, S. Net and B. Ouddane, Degradation of fluorene and phenanthrene in PAHs-contaminated soil using Pseudomonas and Bacillus strains isolated from oil spill sites, J. Environ. Manage., 2019, 232, 1–7 CrossRef CAS PubMed.
- S. Jorfi, A. Rezaee, G.-A. Mobeh-ali and N. A. Jaafarzadeh, Application of Biosurfactants Produced by Pseudomonas aeruginosa SP4 for Bioremediation of Soils Contaminated by Pyrene, Soil Sediment Contam., 2013, 22, 890–911 CrossRef.
- G. T. Mehetre, S. G. Dastager and M. S. Dharne, Biodegradation of mixed polycyclic aromatic hydrocarbons by pure and mixed cultures of biosurfactant producing thermophilic and thermo-tolerant bacteria, Sci. Total Environ., 2019, 679, 52–60 CrossRef CAS PubMed.
- W. Tao, J. Lin, W. Wang, H. Huang and S. Li, Biodegradation of aliphatic and polycyclic aromatic hydrocarbons by the thermophilic bioemulsifier-producing Aeribacillus pallidus strain SL-1, Ecotoxicol. Environ. Saf., 2020, 189, 109994 CrossRef CAS PubMed.
- A. Medic, M. Ljesevic, H. Inui, V. Beskoski, I. Kojic, K. Stojanovic and I. Karadzic, Efficient biodegradation of petroleum n-alkanes and polycyclic aromatic hydrocarbons by polyextremophilic Pseudomonas aeruginosa san ai with multidegradative capacity, RSC Adv., 2020, 10, 14060 RSC.
- F. A. Bezza and E. M. N. Chirwa, Pyrene biodegradation enhancement potential of lipopeptide biosurfactant produced by Paenibacillus dendritiformis CN5 strain, J. Hazard. Mater., 2017, 321, 218–227 CrossRef CAS PubMed.
- A. F. Almansoorya, H. A. Hasan, M. Idris, S. R. S. Abdullah and N. Anuar, Potential application of a biosurfactant in phytoremediation technology for treatment of gasoline-contaminated soil, Ecol. Eng., 2015, 84, 113–120 CrossRef.
- C. Liao, X. Liang, G. Lu, T. Thai, W. Xu and Z. Dang, Effect of surfactant amendment to PAHs-contaminated soil for phytoremediation by maize (Zea mays L.), Ecotoxicol. Environ. Saf., 2015, 112, 1–6 CrossRef CAS PubMed.
- J. Chaprao, I. N. S. Ferreira, P. F. Correa, R. D. Rufino, J. M. Luna, E. J. Silva and L. A. Sarubbo, Application of bacterial and yeast biosurfactants for enhanced removal and biodegradation of motor oil from contaminated sand, Electro. J. Biotechnol., 2015, 18, 471–479 CrossRef.
- A. K. Singh and S. S. Cameotra, Efficiency of lipopeptide biosurfactants in removal of petroleum hydrocarbons and heavy metals from contaminated soil, Environ. Sci. Pollut. Res., 2013, 20, 7367–7376 CrossRef CAS PubMed.
- J. Vaishnavi, S. Devanesan, M. S. AlSalhi, A. Rajasekar, A. Selvi, P. Srinivasan and M. Govarthanan, Biosurfactant mediated bioelectrokinetic remediation of diesel contaminated environment, Chemosphere, 2021, 264, 128377 CrossRef CAS PubMed.
- D. Mańko-Jurkowska, E. Ostrowska-Ligęza, A. Górska and R. Głowacka, The role of biosurfactants in soil remediation, Zeszyty Problemowe Postępów Nauk Rolniczych, 2019, 596, 33–43 CrossRef.
- C. Sánchez, A review of the role of biosurfactants in the biodegradation of hydrophobic organopollutants: production, mode of action, biosynthesis and applications, World J. Microbiol. Biotechnol., 2022, 38, 216 CrossRef PubMed.
- B. M. Karamchandani, A. A. Pawar, S. S. Pawar, S. Syed, N. S. Mone, S. G. Dalvi, P. K. S. M. Rahman, I. M. Banat and S. K. Satpute, Biosurfactants' multifarious functional potential for sustainable agricultural practices, Front. Bioeng. Biotechnol., 2022, 10, 1047279 CrossRef PubMed.
- L. J. Evans, Chemistry of metal retention by soils, Environ. Sci. Technol., 1989, 23(9), 1046–1056 CrossRef CAS.
- Y. S. Açıkel, Use of biosurfactants in the removal of heavy metal ions from soils, Springer, Netherlands, 2011, vol. 20, pp. 183–223 Search PubMed.
- S. Khalid, M. Shahid, N. K. Niazi, B. Murtaza, I. Bibi and C. Dumat, A comparison of technologies for remediation of heavy metal contaminated soils, J. Geochem. Explor., 2017, 182, 247–268 CrossRef CAS.
- J. K. Guo, H. Muhammad, T. Wei, X. H. Ren, H. L. Jia, S. Atif and L. Hua, Prospects and applications of plant growth promoting rhizobacteria to mitigate soil metal contamination: a review, Chemosphere, 2020, 246, 125823 CrossRef CAS PubMed.
- L. Jiang, X. Liu, H. Yin, Y. Liang, H. Liu, B. Miao, Q. Peng, D. Meng, S. Wang, J. Yang and Z. Guo, The utilization of biomineralization technique based on microbial induced phosphate precipitation in remediation of potentially toxic ions contaminated soil: a mini review, Ecotoxicol. Environ. Saf., 2020, 191, 110009 CrossRef CAS PubMed.
- M. M. Naik, D. Khanolkar and S. K. Dubey, Lead-resistant Providencia alcalifaciens strain 2EA bioprecipitates Pb+2 as lead phosphate, Lett. Appl. Microbiol., 2013, 56, 99–104 CrossRef CAS PubMed.
- Z. Teng, W. Shao, K. Zhang, Y. Huo and M. Li, Characterization of phosphate solubilizing bacteria isolated from heavy metal contaminated soils and their potential for lead immobilization, J. Environ. Manage., 2019, 231, 189–197 CrossRef CAS PubMed.
- A. A. Juwarkar, A. Nair, K. V. Dubey, S. K. Singh and S. Devotta, Biosurfactant technology for remediation of cadmium and lead contaminated soils, Chemosphere, 2007, 68, 1996–2002 CrossRef CAS PubMed.
- R. B. da Rocha Junior, H. M. Meira, D. G. Almeida, R. D. Rufino, J. M. Luna, V. A. Santos and L. A. Sarubbo, Application of a low-cost biosurfactant in heavy metal remediation processes, Biodegradation, 2019, 30, 215–233 CrossRef PubMed.
- P. Mekwichai, C. Tongcumpou, S. Kittipongvises and N. Tuntiwiwattanapun, Simultaneous biosurfactant-assisted remediation and corn cultivation on cadmium-contaminated soil, Ecotoxicol. Environ. Saf., 2020, 192, 110298 CrossRef CAS PubMed.
- Z. M. Gusiatin, M. Radziemska and A. Zochowska, Sequential soil washing with mixed biosurfactants is suitable for simultaneous removal of multi-metals from soils with different properties, pollution levels and ages, Environ. Earth Sci., 2019, 78, 529 CrossRef.
- T. A. Akintunde, O. P. Abioye, S. B. Oyeleke, B. E. Boboye and U. J. J. Ijah, Remediation of iron using rhamnolipid-surfactant produced by Pseudomonas aeruginosa, Res. J. Environ. Sci., 2015, 9, 169–177 CrossRef.
- J. Tang, J. He, T. Liu and X. Xin, Removal of heavy metals with sequential sludge washing techniques using saponin: optimization conditions, kinetics, removal effectiveness, binding intensity, mobility and mechanism, RSC Adv., 2017, 7, 33385 RSC.
- R. D. Rufino, G. I. B. Rodrigues, G. M. Campos-Takaki, L. A. Sarubbo and S. R. M. Ferreira, Application of a Yeast Biosurfactant in the Removal of Heavy Metals and Hydrophobic Contaminant in a Soil Used as Slurry Barrier, Appl. Environ. Soil Sci., 2011, 939648 Search PubMed.
- C. N. Mulligan, Biosurfactants for the remediation of metal contamination, in Handbook of Metal-Microbe Interactions and Bioremediation, ed. S. Das and H. R. Dash, CRC Press, Boca Raton, FL, USA, 2017 Search PubMed.
- S. Mishra, Z. Lin, S. Pang, Y. Zhang, P. Bhatt and S. Chen, Biosurfactant is a powerful tool for the bioremediation of heavy metals from contaminated soils, J. Hazard. Mater., 2021, 418, 126253 CrossRef CAS PubMed.
- Z. M. Gusiatin and M. Radziemska, Saponin versus rhamnolipids for remediation of Cd contaminated soils, Clean: Soil, Air, Water, 2018, 46, 1700071 Search PubMed.
- A. A. Juwarkar, K. V. Dubey, A. Nair and S. K. Singh, Bioremediation of multi-metal contaminated soil using biosurfactant — a novel approach, Indian J. Microbiol., 2008, 48, 142–146 CrossRef CAS PubMed.
- J. M. Luna, R. D. Rufino and L. A. Sarubbo, Biosurfactant from Candida sphaerica UCP0995 exhibiting heavy metal remediation properties, Process Saf. Environ. Prot., 2016, 102, 558–566 CrossRef CAS.
- N. M. Venkatesh and N. Vedaraman, Remediation of soil contaminated with copper using Rhamnolipids produced from Pseudomonas aeruginosa MTCC 2297 using waste frying rice bran oil, Ann. Microbiol., 2012, 62, 85–91 CrossRef CAS.
- A. D. Khiyavi, R. Hajimohammadi, H. Amani and H. Soltani, Synergistic Effect of Rhamnolipid and Saponin Biosurfactants on Removal of Heavy Metals from Oil Contaminated Soils, Tenside, Surfactants, Deterg., 2020, 57, 109–114 CrossRef CAS.
- J. Wan, D. Meng, T. Long, R. Ying, M. Ye, S. Zhang, Q. Li, Y. Zhou and Y. Lin, Simultaneous Removal of Lindane, Lead and Cadmium from Soils by Rhamnolipids Combined with Citric Acid, PLoS One, 2015, 10, e0129978 CrossRef PubMed.
- Z. Ahmad, M. Imran, S. Qadeer, S. Hussain, R. Kausar, L. Dawson and A. Khalid, Biosurfactants for sustainable soil management, in Advances in Agronomy, ed. D. Sparks, Academic Press, Massachusetts, MA, USA, 2018, vol. 150, pp. 81–130 Search PubMed.
- G. S. Chavez and R. M. Maier, Biosurfactants: a general overview, in Biosurfactants: From Genes to Applications, ed. G. Soberon-chavez, Springer-Verlag, Berlin, Germany, 2011, pp. 1–11 Search PubMed.
- C. N. Mulligan, A. Mudhoo and S. K. Sharma, Biosurfactants: Research Trends and Applications, CRC Press, Boca Raton, FL, 2014 Search PubMed.
- M. Pacwa-Płociniczak, G. A. Płaza, Z. Piotrowska-Seget and S. S. Cameotra, Environmental Applications of Biosurfactants: Recent Advances, Int. J. Mol. Sci., 2011, 12, 633–654 CrossRef PubMed.
- D. Meng, L.-X. Zhai, Q.-P. Tian, Z.-B. Guan, Y.-J. Cai and X.-R. Liao, Complete genome sequence of Bacillus amyloliquefaciens YP6, a plant growth rhizobacterium efficiently degrading a wide range of organophosphorus pesticides, J. Integr. Agric., 2019, 18, 2668–2672 CrossRef CAS.
- D. Meng, L. Zhang, J. Meng, Q. Tian, L. Zhai, Z. Hao, Z. Guan, Y. Cai and X. Liao, Evaluation of the Strain Bacillus amyloliquefaciens YP6 in Phox Degradation via Transcriptomic Data and Product Analysis, Molecules, 2019, 24, 3997 CrossRef CAS PubMed.
- L. Ferreira, E. Rosales, A. S. Danko, M. A. Sanroman and M. M. Pazos, Bacillus thuringiensis a promising bacterium for degrading emerging pollutants, Process Saf. Environ. Prot., 2016, 101, 19–26 CrossRef CAS.
- V. K. Gaur, A. Bajaj, R. K. Regar, M. Kamthan, R. R. Jha, J. K. Srivastava and N. Manickam, Rhamnolipid from a Lysinibacillus sphaericus strain IITR51 and its potential application for dissolution of hydrophobic pesticides, Bioresour. Technol., 2019, 272, 19–25 CrossRef CAS PubMed.
- Q. Guo, J. Yan, J. Wen, Y. Hu, Y. Chen and W. Wu, Rhamnolipid-enhanced aerobic biodegradation of triclosan (TCS) by indigenous microorganisms in water-sediment systems, Sci. Total Environ., 2016, 571, 1304–1311 CrossRef CAS PubMed.
- B. Wang, Q. Wang, W. Liu, X. Liu, J. Hou, Y. Teng, Y. Luo and P. Christie, Biosurfactant-producing microorganism Pseudomonas sp. SB assists the phytoremediation of DDT-contaminated soil by two grass species, Chemosphere, 2017, 182, 137–142 CrossRef CAS PubMed.
- N. Bai, S. Wang, R. Abuduaini, M. Zhang, X. Zhu and Y. Zhao, Rhamnolipid-aided biodegradation of carbendazim by Rhodococcus sp. D-1: characteristics, products, and phytotoxicity, Sci. Total Environ., 2017, 590–591, 343–351 CrossRef CAS PubMed.
- G. Odukkathil and N. Vasudevan, Enhanced biodegradation of endosulfan and its major metabolite endosulfate by a biosurfactant producing bacterium, J. Environ. Sci. Health, Part B, 2013, 48, 462–469 CrossRef CAS PubMed.
- I. Ortiz, M. A. Ávila-Chávez and L. G. Torres, Removal of α- and β-Endosulfan from Soils by Using Natural and Synthetic Surfactants, Asian J. Environ. Ecology, 2018, 6, 1–11 CrossRef.
- Y. Tao, H. Li, J. Gu, H. Shi, S. Han, Y. Jiao, G. Zhong, Q. Zhang, M. S. Akindolie, Y. Lin, Z. Chen and Y. Zhang, Metabolism of diethyl phthalate (DEP) and identification of degradation intermediates by Pseudomonas sp. DNE-S1, Ecotoxicol. Environ. Saf., 2019, 173, 411–418 CrossRef CAS PubMed.
- P. Jakinala, N. Lingampally, A. Kyama and B. Hameeda, Enhancement of atrazine biodegradation by marine isolate Bacillus velezensis MHNK1 in presence of surfactin lipopeptide, Ecotoxicol. Environ. Saf., 2019, 182, 109372 CrossRef CAS PubMed.
- A. M. Nair, S. Rebello, K. S. Rishad, A. K. Asok and M. S. Jisha, Biosurfactant Facilitated Biodegradation of Quinalphos at High Concentrations by Pseudomonas aeruginosa Q10, Soil and Sediment Contamination, Int. J., 2015, 24, 542–553 CAS.
- C. Zhang, S. Wang and Y. Yan, Isomerization and biodegradation of beta-cypermethrin by Pseudomonas aeruginosa CH7 with biosurfactant production, Bioresour. Technol., 2011, 102, 7139–7146 CrossRef CAS PubMed.
- C. Becerra-Castro, P. S. Kidd, B. Rodríguez-Garrido, C. Monterroso, P. Santos-Ucha and A. Prieto-Fernández, Phytoremediation of hexachlorocyclohexane (HCH)-contaminated soils using Cytisus striatus and bacterial inoculants in soils with distinct organic matter content, Environ. Pollut., 2013, 178, 202–210 CrossRef CAS PubMed.
- D. A. M. Alexandrino, A. P. Mucha, C. M. R. Almeida and M. F. Carvalho, Microbial degradation of two highly persistent fluorinated fungicides – epoxiconazole and fludioxonil, J. Hazard. Mater., 2020, 394, 122545 CrossRef CAS PubMed.
- X. Chen, S. He, X. Liu and J. Hu, Biobegradation and metabolic mechanism of cyprodinil by strain Acinetobacter sp. from a contaminated-agricultural soil in China, Ecotoxicol. Environ. Saf., 2018, 159, 190–197 CrossRef CAS PubMed.
- C. N. Mulligan, R. N. Yong and B. F. Gibbs, Surfactant-enhanced remediation of contaminated soil: a review, Eng. Geol., 2001, 60, 371–380 CrossRef.
- R. Doong and W. Lei, Solubilization and mineralization of polycyclic aromatic hydrocarbons by Pseudomonas putida in the presence of surfactant, J. Hazard. Mater., 2003, 96, 15–27 CrossRef CAS PubMed.
- D. S. Pardhi, R. R. Panchal, V. H. Raval, R. G. Joshi, P. Poczai, W. H. Almalki and K. N. Rajput, Microbial surfactants: a journey from fundamentals to recent advances, Front. Microbiol., 2022, 13, 982603 CrossRef PubMed.
- L. A. Sarubbo, M. G. C. Silva, I. J. B. Durval, K. G. O. Bezerra, B. G. Ribeiro, I. A. Silva, M. S. Twigg and I. M. Banat, Biosurfactants: production, properties, applications, trends, and general perspectives, Biochem. Eng. J., 2022, 181, 108377 CrossRef CAS.
- P. Johnson, A. Trybala, V. Starov and V. J. Pinfield, Effect of synthetic surfactants on the environment and the potential for substitution by biosurfactants, Adv. Colloid Interface Sci., 2021, 288, 102340 CrossRef CAS PubMed.
- R. Jahan, A. M. Bodratti, M. Tsianou and P. Alexandridis, Biosurfactants, natural alternatives to synthetic surfactants: physicochemical properties and applications, Adv. Colloid Interface Sci., 2020, 275, 102061 CrossRef CAS PubMed.
- C.-C. Lai, Y.-C. Huang, Y.-H. Wei and J.-S. Chang, Biosurfactant-enhanced removal of total petroleum hydrocarbons from contaminated soil, J. Hazard. Mater., 2009, 167, 609–614 CrossRef CAS PubMed.
- K. Urum, S. Grigson, T. Pekdemir and S. McMenamy, A comparison of the efficiency of different surfactants for removal of crude oil from contaminated soils, Chemosphere, 2006, 62, 1403–1410 CrossRef CAS PubMed.
- D. Mohan and C. U. Pittman Jr, Activated carbons and low cost adsorbents for remediation of tri- and hexavalent chromium from water, J. Hazard. Mater., 2006, 137, 762–811 CrossRef CAS PubMed.
- C. Verma, C. M. Hussain, M. A. Quraishi and A. Alfantazi, Green surfactants for corrosion control: design, performance and applications, Adv. Colloid Interface Sci., 2023, 311, 102822 CrossRef CAS PubMed.
- Y. Wang, S. Wu, H. Wang, Y. Dong, X. Li, S. Wang, H. Fan and X. Zhuang, Optimization of conditions for a surfactant-producing strain and application to petroleum hydrocarbon-contaminated soil bioremediation, Colloids Surf., B, 2022, 213, 112428 CrossRef CAS PubMed.
|
This journal is © The Royal Society of Chemistry 2023 |