DOI:
10.1039/D0QO00279H
(Review Article)
Org. Chem. Front., 2020,
7, 1527-1569
Transition metal-catalyzed coupling of heterocyclic alkenes via C–H functionalization: recent trends and applications
Received
9th March 2020
, Accepted 28th April 2020
First published on 29th April 2020
Abstract
Heterocyclic alkenes represent an important class of reactive feedstock and valuable synthons for the synthesis of biologically important heterocyclic scaffolds. Although functionalized heterocyclic alkenes and their derivatives can be accessed via metal-catalyzed cross-coupling reactions, yet the prefunctionalisation of starting materials makes the process a bit cumbersome. On the other hand, transition-metal-catalyzed C–H functionalization is one of the most persuasive and front-line research areas in modern synthetic organic chemistry for atom/step-economy, viability and high efficacy. This critical review highlights the recent advances in coupling of heterocyclic alkenes by transition-metal-catalyzed reactions with special emphasis on arylation, alkenylation, alkylation, hydroarylation, ring-opening addition and annulation/cyclization via the C–H functionalization strategy reported since 2015.
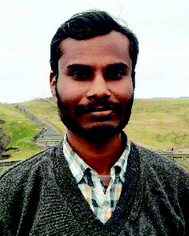 Sundaravel Vivek Kumar | Sundaravel Vivek Kumar was raised in Tamil Nadu, India. He received his M.Sc. degree from Madurai Kamaraj University in 2011. He earned his Ph.D. in chemistry under the joint direction of Professor S. Muthusubramanian and Professor S. Perumal at the same university in 2016. Subsequently, he worked as a research associate supervised by Dr R. Ranjith Kumar at Madurai Kamaraj University. He was then a SERB National Post-Doctoral research fellow (2017–2019) in Professor T. Punniyamurthy's research group at the Department of Chemistry, IIT Guwahati, where he studied Rh-catalyzed C–H activation reactions. He is currently an Irish Research Council (IRC) Post-Doctoral Research Fellow in the laboratory of Professor Patrick Guiry at University College Dublin, Ireland, working on the design and development of chiral P,N-ligands for asymmetric catalysis. |
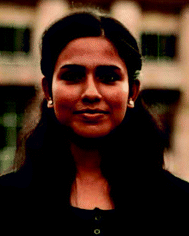 Sonbidya Banerjee | Sonbidya Banerjee was born in West Bengal, India. She received her B.Sc. at West Bengal State University. After obtaining her M.Sc. from Calcutta University, she joined the group of Professor T. Punniyamurthy as a PhD student at the Indian Institute of Technology Guwahati where she studies transition-metal-catalyzed C–H functionalization by chelation assistance. |
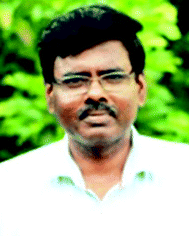 Tharmalingam Punniyamurthy | Tharmalingam Punniyamurthy received his Ph.D. from the Indian Institute of Technology Kanpur (Professor J. Iqbal, 1995). After postdoctoral research at North Dakota State University (Prof. M. P. Sibi, 1995–1996), Kyushu University (Professor T. Katsuki, 1997–1999) and Montpellier University II (Professor A. Vioux and Professor J. J. E. Moreau, 2000–2001), he joined the Indian Institute of Technology Guwahati and became HAG Professor in 2015. He is a visiting Professor at Oxford University (2007), Kyushu University (2013) and the Scripps Research Institute San Diego (2014). He is an elected fellow of The Indian Academy of Sciences (2015), The National Academy of Sciences (2018) and The Royal Society of Chemistry (2014). His research interests include the study of sustainable synthetic methodologies for organic transformations. |
1. Introduction
Heterocyclic alkenes are one of the most privileged structural scaffolds in synthetic, medicinal and materials sciences (Scheme 1).1,2 Consequently, the functionalization of heterocyclic alkenes is one of the exciting research areas and has gained considerable attention. Heterocyclic alkenes such as pyrones, pyridones, coumarins and isoquinolones are important key intermediates, which possess two or more feasible reactive C–H sites, thereby making the selective C–H functionalization very challenging. Similarly, the functionalization of unsymmetrical heterocyclic alkenes is also a formidable task owing to the two possible competitive reactive sites. Maleimides are also useful activated alkenes, which have been largely studied in diverse reactions such as conjugate addition, cycloaddition, cross-coupling and Diels–Alder reactions3 to give the succinimide architecture that are broadly wielded in natural and bioactive compounds.4 Heterobicyclic alkenes are another class of valuable strained ring systems that have an innate reactivity and possess a unique template to obtain natural products5 and complex heterocycles with two or multiple chiral centres, and a variety of reactions including ring-opening, cycloaddition and isomerization have been explored.6
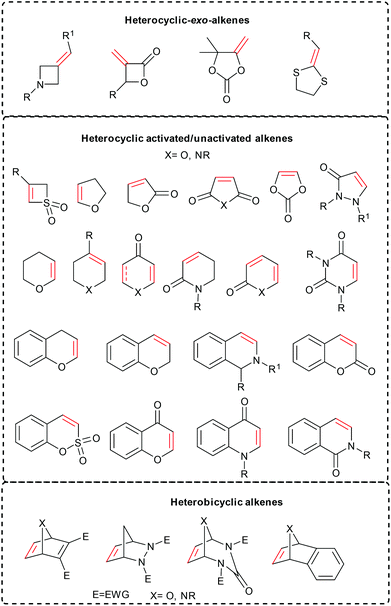 |
| Scheme 1 Some selected examples of heterocyclic alkenes. | |
Though metal-catalyzed cross-coupling reactions7 have been one of the most promising synthetic approaches for accessing the functionalized heterocyclic alkenes, their application is inevitably associated with shortcomings such as the requirement of a pre-functionalized substrate precursor.8 Transition-metal-catalyzed C–H functionalization has thus emerged as a corner-stone research topic in catalysis and has heralded a new synthetic era, with methodologies that afford a crisp route to obtain complex molecules in an atom- and step-economical fashion with broad substrate scope.9 Among these, the C–H activation reaction involves the cleavage of C–H bonds to form a reactive M–C intermediate that interacts with the coupling partner to afford the functionalized product. Importantly, the nature of M–C insertion depends on the substrate, solvent, additive, transition-metal and ligand. Mainly four types of mechanisms, σ-bond metathesis in early transition-metal complexes, oxidative addition in electron-rich late transition-metal complexes, electrophilic activation in electron-deficient late transition-metal complexes and Lewis-base assisted metalation, are involved.10
Recently, the Miura group reviewed the functionalization of pyridones,11 while McGlacken12a and Hong12b groups covered pyrone/pyridone and coumarin/chromene functionalization, respectively.12 Later, the coupling of maleimides was reviewed by Jeganmohan and co-workers.13 Much progress has been made in the coupling of heterocyclic alkenes via C–H activation. This review will deliberate transition-metal-catalyzed coupling of the heterocyclic alkenes including exo-heterocyclic alkenes via an organometallic C–H functionalization strategy and depict their development since 2015 to present, except the reactions involving radical intermediates. The focus will be on highlighting the feasibility in terms of catalytic competence, selectivity and mechanistic manifolds. The literature has been classified based on reaction types and further arranged by substrate complexity.
1.1. General coupling patterns of heterocyclic alkenes
Coupling of heterocyclic alkenes can be achieved specifically by C–H activation via two types of approaches (Scheme 2).
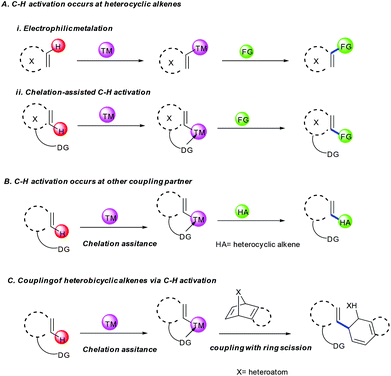 |
| Scheme 2 General coupling patterns of heterocyclic alkenes. | |
• C–H activation at heterocyclic alkenes (pyrones, pyridones, coumarins, chromenes and quinolones)
• C–H activation at another coupling partner (arenes or heteroarenes).
In the first case, C–H activation takes place via electrophilic metalation e.g. concerted metalation deprotonation (CMD) followed by coupling with the other partner or chelation assisted C–H activation and subsequent coupling with the other reacting partner (Scheme 2A(i) and (ii)). In the second case, the C–H activation occurs at another partner (arene/heteroarene) by chelation assistance that interacts with the heterocyclic alkene, leading to coupling via migratory insertion and reductive elimination or protonation of the M–C bond (Scheme 2B). While heterobicyclic alkenes have inherent reactivity owing to the ring strain, which can couple in various ways. In general, C–H activation takes place first at arenes, which interact with the heterobicyclic alkene and subsequently migratory insertion and ring scission induced by β-heteroatom elimination afford the final product (Scheme 2C). A comprehensive view of the mechanistic aspects of every type of the reaction is discussed in the appropriate places of the article.
2. Arylation
Arylation via C–H activation leads to an important C–C bond formation. Aryl halides/-boronic acids/-carboxylic acids have been generally utilized as the aryl source. The coupling of thietes with aryl bromides has been achieved by Pd-catalysis (Scheme 3).14 A series of substrates are compatible with functional group tolerance. The C–H activation most likely arises through a base-assisted internal substitution (BIES) rather than the CMD process. Some of the arylated thietes inhibit the tubulin polymerization.
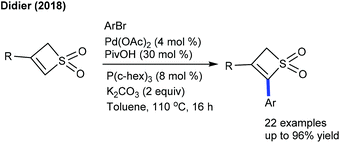 |
| Scheme 3 Arylation of thietes. | |
Recently, the Ferry group showed the arylation of anomeric C–H bonds of glycals with aryl iodides (Scheme 4).15 Irrespective of the electronic properties, aryl iodides are tolerated. Besides, D-galactal and D-lactal prove to be good coupling partners. Control studies exclude the possibility of usual carbopalladation and β-elimination. In 2016, Ru-catalyzed regioselective C–H arylation of cyclic enamides has been achieved (Scheme 5).16 The reaction takes place selectively at the sp2 α-C–H bond rather than the sp3 α-C–H bond that allows the access of a range of heteroaryl dehydropyrrolidines. Piperidine and azepenes furnish the desired products, while alkenyl/styrenyl boronic acids participate albeit giving less yield.
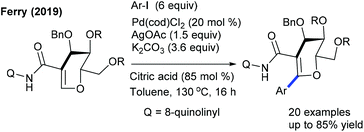 |
| Scheme 4 Arylation of glycals. | |
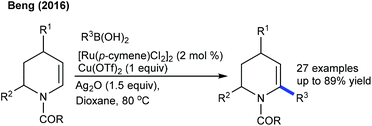 |
| Scheme 5 Arylation of cyclic enamides with boronic acids. | |
The Canterbury group presented a Pd-catalyzed β-selective arylation of α,β-unsaturated valerolactams (Scheme 6).17 Among the tested phosphine-based ligands, amphos provides the best results. The procedure is more sensitive towards the substitution on the aryl ring and N-substitution of the lactams. The C6-arylation of pyridones can be achieved with aryl trifluoroborates employing Rh-catalysis (Scheme 7).18 Aryl trifluoroborates with varied electronic properties afford a small library of desired products, while a steric effect notably affects the reaction.
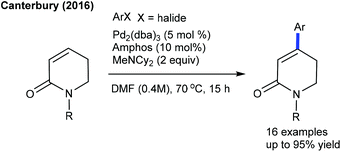 |
| Scheme 6 β-Arylation of α,β-unsaturated valerolactams. | |
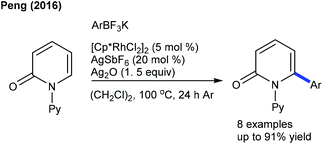 |
| Scheme 7 Arylation of pyridones using aryl trifluoroborates. | |
The synthesis of heteroarylated phenols can be achieved via the C6-arylation of pyridones using quinine diazides.19 This oxidant-free procedure is studied with diverse pyridine substrates. Recently, Waser and co-workers carried out C6-indolation of pyridone (Scheme 8).20 The combination of the additive AgSbF6 and Lewis acid Zn(OTf)2 plays a crucial role. Functional group tolerance and construction of valuable indole-tethered heterocycles are the important features; however the scope of the indole is rather limited. Pd-Catalyzed C5-arylated pyridones are obtained by the reaction of pyridones with aryl iodides (Scheme 9).21 Addition of AgNO3 confers the electrophilic nature of the Pd-catalyst; however, it aids in resuming the activity of the active catalyst. A wide range of electronically diverse substrates are compatible although the products are formed in moderate yields.
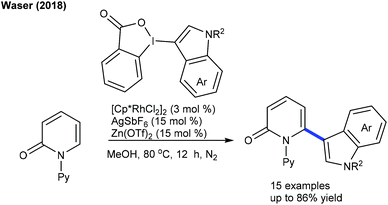 |
| Scheme 8 C6-Indolation of pyridones. | |
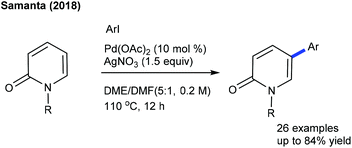 |
| Scheme 9 C5-Arylation of pyridones. | |
The arylation has been found to be successful utilizing an arene as an aryl source, which is attractive due to its atom economy (Scheme 10).22 The reaction of C4-monosubstituted chromenes provides C2-arylation products. In contrast, C4-disubstituted chromenes give benzopyrans. Furthermore, electron-deficient arenes yield C3-arylated products. A similar kind of ring contraction is observed with the coupling of coumarins using aryl bromides as the aryl source (Scheme 11).23 This reaction is compatible with a broad range of substrates.
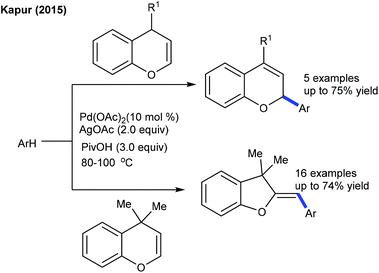 |
| Scheme 10 Synthesis of benzopyran via C–C bond migration. | |
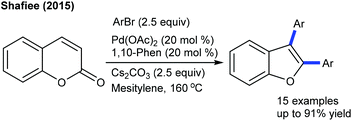 |
| Scheme 11 Domino C–H and C–C activation of coumarins. | |
The decarboxylative coupling of aryl carboxylic acids is effective at the C2 position of quinolone and isoquinolone at elevated temperature (Scheme 12).24 The Hong group successfully developed the catalyst controlled C4-arylation of isoquinolones using diaryliodonium salts as an aryl source.25 Ar2IBF4 is the best coupling partner, although ortho-substituted iodonium salts are less reactive. They further showed an inherent reactivity and site-selectivity in the C–H functionalization of enaminones and chromones (Scheme 13).26 According to Kapur's report (Scheme 10), they have utilized an excess of an arene as the aryl source. The following conclusions are observed:
• The site-selectivity differs on switching the mechanism between CMD and carbopalladation.
• The C–H activation of an enaminone and a chromone occurs via CMD more readily at the π-electron rich position and affords obvious selectivity at C-3.
• Arylation of the enaminone generally provides a C-3 arylated product due to the high activation barrier at C-2.
• Arylation of the chromone occurs favourably at C-2 via carbopalladation since a metal interacts more strongly at C-2 compared to C-3.
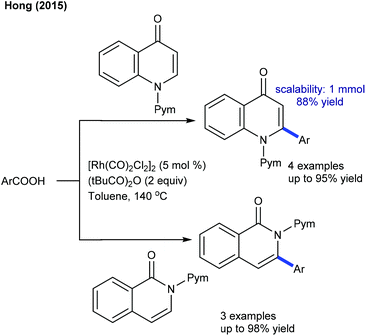 |
| Scheme 12 C2-Arylation of quinolones/isoquinolones. | |
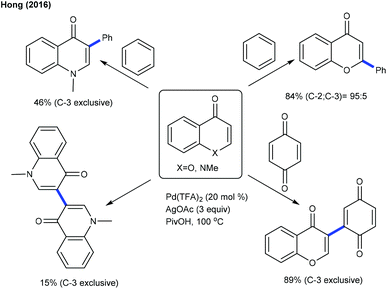 |
| Scheme 13 Functionalization of enaminones and chromones. | |
Later, the arylation of 2-pyridones and 1-isoquinolones is accomplished using Ru-catalysis (Scheme 14).27 The trio Ru/Cu/Ag catalytic system provides an effective synthetic tool to access the arylated products.
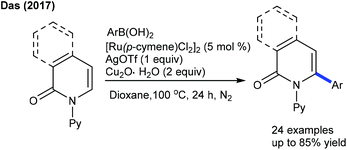 |
| Scheme 14 Arylation of 2-pyridones/1-isoquinolones with aryl boronic acids. | |
Efforts are made towards the functionalization of uracil. The dehydrogenative coupling with benzofurans is successful using PivOH at room temperature (Scheme 15).28 The scope of the procedure is somewhat limited, and the mechanism involves an electrophilic palladation, while the C6-arylation employing aryl halides and boronic acids is effective at 110 °C (Scheme 16).29 The combination of Pd(OAc)2, CuI, xantphos and DBU is essential. Aryl iodides/-bromides provide the best results, while ArB(OH)2/ArBF3K give inferior results. Xantphos accelerates the oxidative addition and reductive elimination steps. A similar strategy is used for the C3-arylation of pyrido[1,2-a]pyrimidin-4-ones using aryl bromide (Scheme 17).30 Ag-Salt plays a dual role as an oxidant and a halo-sequestering agent. A ligand is necessary for this coupling, and PCy3 shows promising results. A broad range of aryl bromides are coupled. Aryl iodides exhibit similar reactivities, whereas aryl chloride remains intact. Other coupling partners such as aryl triflate and -tosylate exhibit less reactivity.
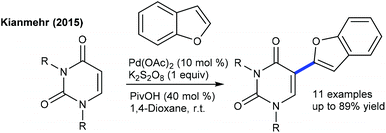 |
| Scheme 15 Dehydrogenative coupling of uracil with benzofurans. | |
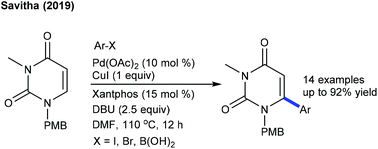 |
| Scheme 16 C6-Arylation of uracil with aryl halides/-boronic acids. | |
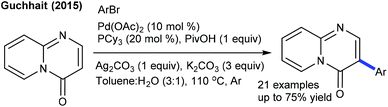 |
| Scheme 17 C3-Arylation of pyrido[1,2-a]pyrimidin-4-ones. | |
The functionalization of oxabicyclic alkenes is attractive. The Hiyama group showed an example of an alkynyloxy group as a hydrogen accepting director for the arylation of an oxabicyclic alkene (Scheme 18).31 This reaction at 100 °C provides a mixture of naphthylated and cyclized products in lower yields. However, at elevated temperature (140 °C), the cyclized product is formed in moderate yield. Cheng and co-workers examined the Catellani-type reaction of aryl iodides, oxanorbornadiene and hexamethyldisilane (Scheme 19).32 A heterocyclic alkene plays a twin role as an ortho C–H activator and an ethylene surrogate. In particular, estrone, amino acids and heterocyclic iodides prove to be competent substrates. Organogermananes/-stannanes also work well; however, the latter needs a K3PO4 additive instead of K2CO3 though it provides less yield. They extended the study to norbornenes under slightly modified conditions.33 Solvents play a leading role, and the generality is evaluated with diverse aryl iodides and norbornenes.
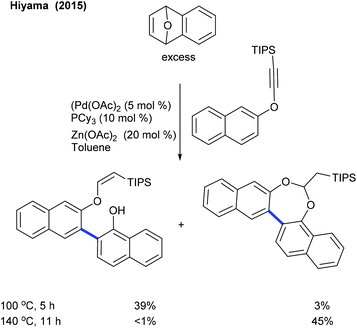 |
| Scheme 18 Functionalization with oxabenzonorbornadienes. | |
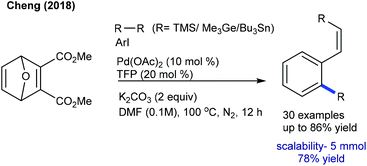 |
| Scheme 19 Synthesis of (Z)-β-substituted vinylsilanes by Catellani-type reaction. | |
Oxabicyclic alkenes are found to be valuable 2-naphthylation sources and have been considerably investigated. This reaction generally comprises a C–H activation, ring-opening, addition and hydration sequence. The Miura group disclosed the Rh-catalyzed coupling of oxabicyclic alkenes with aryl phosphines (Scheme 20).34 Phosphine oxide shows an excellent directing ability compared to phosphine sulfide, which may be due to the latter's thiophilic nature. This procedure provides good yields of products, and some derivatives give a mixture of aromatized and hydroarylated products.
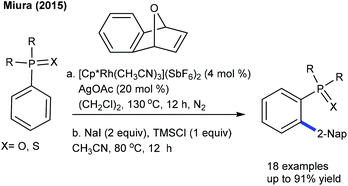 |
| Scheme 20 Coupling of heterobicyclic alkenes with aryl phosphines. | |
Li and co-workers described a Co-catalyzed C2-naphthylation of indoles (Scheme 21).35 A blend of AcOH and AgSbF6 effectively promotes the reaction (AgSbF6 acts as a Lewis acid). With slightly tuned conditions, sterically congested C-3 and C-7 substituted indoles can be functionalized. The mechanism involves the reversible C–H cleavage via the CMD pathway. This strategy is extended to the coupling of arenes with oxabenzonorbornadienes (Scheme 22),36 where N-pyrimidinylbenzimidazoles deliver oxa-bicycle core retained products. Under modified conditions, a series of electronically diverse 2-arylpyridines serve as efficient coupling partners, giving naphthylated products. This method is complementary to the Li procedure (Scheme 21); however, it requires higher reaction temperature. Recently, Ru-catalyzed C–H naphthylation of anilides with oxabenzonorbornadienes has been achieved (Scheme 23).37 Electron-rich anilides display a higher reactivity and the C–H activation occurs via a redox-neutral pathway.
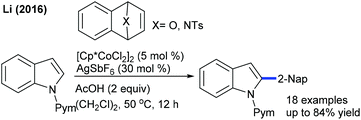 |
| Scheme 21 C2-Naphthylation of indoles. | |
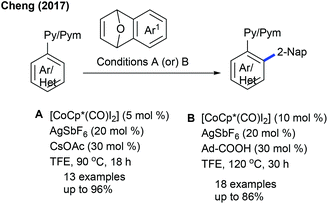 |
| Scheme 22 Addition of arenes with oxabenzonorbornadienes. | |
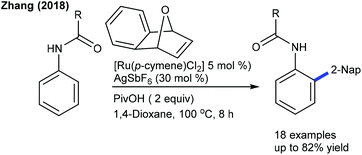 |
| Scheme 23 Naphthylation of anilides with oxabenzonorbornadienes. | |
More recently, Shi and co-workers reported an elegant route to obtain an axial chiral aldehyde via a Pd-catalyzed, atroposelective C–H naphthylation of rac-biaryl aldehydes with oxabenzonorbornadienes (Scheme 24).38L-tert-Leucine serves as a chiral transient directing group and stereocontrolling unit. A series of substituted biaryl and oxabenzonorbornadienes react efficiently with excellent ee (up to >99%). Yet the aryl part having F or 1,3-dioxole groups in oxabenzonorbornadienes are less reactive though without sacrificing the ee. Particularly, an undehydrated product is observed with electron-deficient oxabenzonorbornadiene. The outcome of the chiral aldehyde is further transformed into the active chiral catalyst, which is evaluated for the asymmetric reaction of (E)-chalcone with glycine-derived amides or peptides.
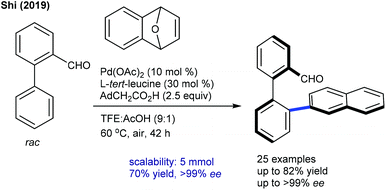 |
| Scheme 24 Naphthylation of rac-biaryl aldehydes. | |
3. Alkenylation
Alkenylation of arenes with cyclic alkenes is one of the puzzling tasks, because of the formation of a rigid bicyclic intermediate, and unavailability of syn β-hydrogen for elimination. This kind of reaction is mostly expected to give a Michael type of product. Nevertheless, the Hong group showed a single example of alkenylation utilizing maleimide under mild reaction conditions (Scheme 25).39
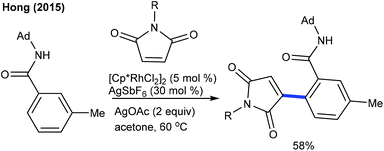 |
| Scheme 25 Coupling of benzamide with maleimides. | |
Later, the coupling of aryl ketones with maleimide has been successfully achieved.40 Ag2CO3 plays a vital role. Electronically diverse maleimides are tolerated, and electron-rich aryl ketones display better reactivity. This strategy has been extended to indoles using COCF3 chelation assistance.41 An additive and an oxidant play an indispensable role. Ag2CO3 gives the Heck-type product, while a AgOAc and ACOH combination leads to hydroarylation. The procedure can be utilized for the alkenylation of aryl acetamides.42para-Substituted aryl acetamides exhibit good reactivity, while ortho-/meta-substituted substrates are less effective. Similarly, the alkenylation of acetanilides can be accomplished (Scheme 26A).43 In addition, the carboxylate directed C–H activation/decarboxylative Heck-type coupling of benzoic acid is achieved (Scheme 26B).44 The selectivity depends on the reaction medium. THF enables alkenylation, whereas TFE produces the annulation product. Similarly, the C5-alkenylation of 2-amino-1,4-naphthoquinones can be accomplished (Scheme 26C).45 The product selectivity depends on the reaction conditions. For instance, alkenylation (45–74%) is observed under basic conditions, whereas acidic conditions give the alkylation product. Moreover, the coupling of acrylamides is effective via C–H alkylation and alkene migration (Scheme 27).46 Integration of a cationic rhodium catalyst with PivOH significantly promotes the reaction. The coupling of a range of acyl amides is shown, although secondary and tertiary substrates give a mixture of E- and Z-products. Weakly coordinating ketone directed Co-catalyzed C4-alkenylation of indole with active alkenes including maleimides is successful (Scheme 28).47 Modest yields of the desired products are obtained, and a significant influence of the electronic effect is noted. However, the scope of the reaction is rather limited.
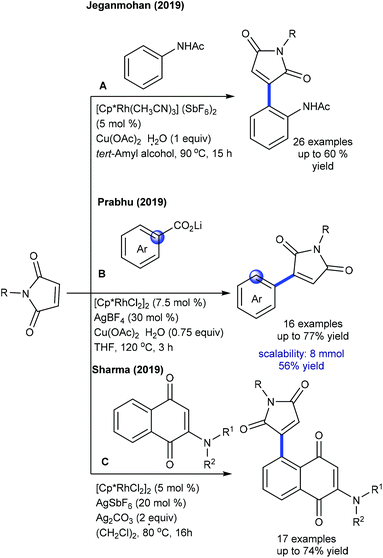 |
| Scheme 26 Alkenylation using maleimides. | |
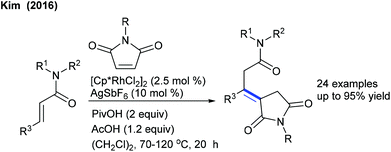 |
| Scheme 27 Cross-coupling of acrylamides with maleimides. | |
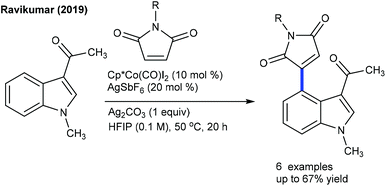 |
| Scheme 28 C4-Alkenylation of indoles with maleimides. | |
Alkenylation of other types of heterocyclic alkenes has been achieved by exploiting electron-deficient alkenes, styrenes and alkenoic acids where C–H activation takes place at the heterocyclic alkene part through an electrophilic metalation pathway. A Pd-catalyzed C–C coupling of dihydropyranones with acrylates is successful under mild conditions (Scheme 29).48 An electron-deficient alkene is competent in delivering the product, whereas styrene is less effective. However, pyrones readily undergo C3 C–C coupling with styrenes (Scheme 30).49 Electronically varied styrenes are tolerated. At slightly elevated temperature, pyridones also react. The Li group presented the Rh-catalyzed redox-neutral coupling of 4-acyl-1-sulfonyl triazoles with isoquinolones in the presence of PivOH (Scheme 31).50 However, this reaction suffers due to the E,Z-mixture of isomers and limited substrate scope.
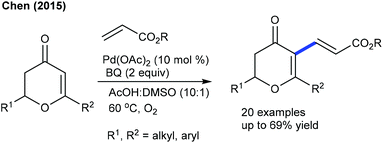 |
| Scheme 29 C3-Alkenylation of dihydropyranones. | |
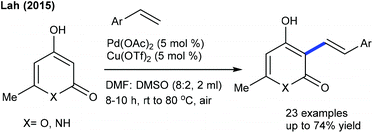 |
| Scheme 30 C3-Alkenylation of pyrones/pyridones with styrenes. | |
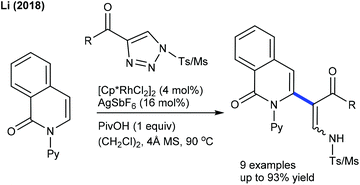 |
| Scheme 31 Coupling of isoquinolones with 4-acyl-1-sulfonyl triazoles. | |
Efforts are made towards achieving decarboxylative couplings. A Rh(I)-catalyzed reaction of quinolones and isoquinolones with aryl and alkyl alkenoic acids is accomplished (Scheme 32).24 Low catalyst loading and broad substrate scope are important features, although a high temperature (140 °C) is mandatory. A similar catalytic system is applied for the coupling of 2-/4-pyridones and isoquinolones with cinnamic acids (Scheme 33).51
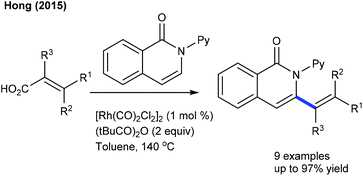 |
| Scheme 32 Decarbonylative C–H alkenylation of quinolones/isoquinolones. | |
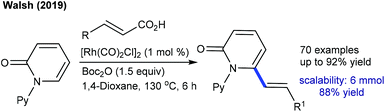 |
| Scheme 33 Decarbonylative C–H alkenylation of pyridones. | |
The coupling of acrylate with pyridones is studied for a solvent-controlled C6-alkenylation/alkylation at moderate temperature (Scheme 34).52 Reaction in DMF gives an alkenylated product and under modified conditions styrene also couples. Recently, the coupling of methylenecyclopropanes has been studied for both isoquinolones and pyridones (Scheme 35).53 A combination of [Cp*RhCl2]2 and AgSbF6 yields the best results. Reaction kinetics and deuterium scrambling experiments show an involvement of the reversible C–H cleavage that probably might be the rate determining step (KIE = 5.4).
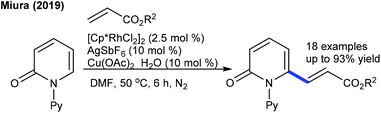 |
| Scheme 34 C6-Alkenylation of pyridones. | |
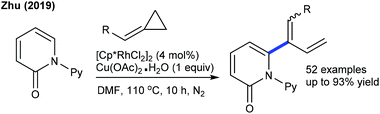 |
| Scheme 35 Coupling of isoquinolones/pyridones with methylenecyclopropanes. | |
The decarboxylative coupling of cinnamic acids with coumarins can be achieved at elevated temperature by Pd-catalysis (Scheme 36).54 The C3-alkenylation is effective with diverse functionalities. Electron-deficient cinnamic acid is less reactive compared to neutral and electron-rich substrates. Hong and co-workers developed the C–H alkenylation of sulfacoumarins with activated alkenes (Scheme 37).55 The procedure is compatible with a wide range of styrenes and utilizes O2 as the oxidant. Kapur and co-workers presented the assembly of C3-alkenated 1,2-dihydroisoquinolines (Scheme 38).56 The acetyl group serves as a removable directing group and a wide range of alkenes including electronically biased alkenes react providing moderate yields, while electron-rich alkenes fail.
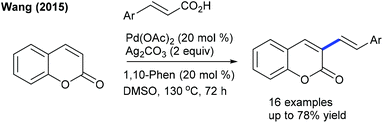 |
| Scheme 36 C3-Alkenylation of coumarins via decarboxylative cross-coupling. | |
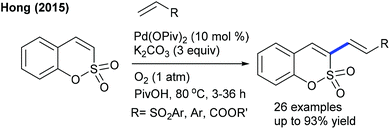 |
| Scheme 37 C3-Alkenylation of sulfacoumarins. | |
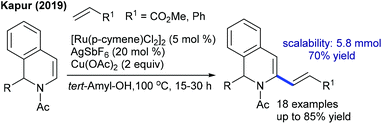 |
| Scheme 38 C3-Alkenylation of N-acetyl-1,2-dihydroisoquinolines. | |
The Huang group reported an environmentally benign alkenylation of uracil (Scheme 39).57 As mentioned above, this procedure also utilizes O2 as the oxidant along with a ligand, and mono-N-protected amino acids (MPAAs) Z-Phe-OH are found to be superior. Electronic/steric effects significantly influence the reaction. For instance, sterically hindered styrenes exhibit less reactivity. Substituted alkenes are viable albeit they give a mixture of isomers, whereas C-6 substituted uracil and free (NH) uracil fail to give the products.
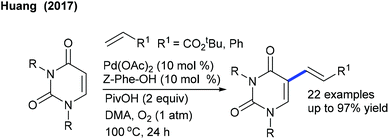 |
| Scheme 39 C3-Alkenylation of uracils. | |
4. Alkylation
Alkylation is a crucial transformation; especially heterocyclic alkenes have potential to afford heterocycle containing alkylated products through a sustainable pathway. In 2017, Ellman showed the coupling of benzamides with 3-(nitromethylene)azetidine with moderate yields (Scheme 40).58 Later, an interesting example of C–H furfurylation of α-acylketene dithioacetals with α-diazoketones has been reported (Scheme 41).59 A variety of functional groups are compatible; however, an increase of the ring-size of dithioacetal hampers the reactivity.
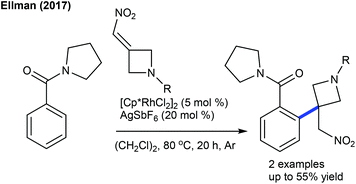 |
| Scheme 40 Alkylation of cyclic benzamides with 3-(nitromethylene)azetidine. | |
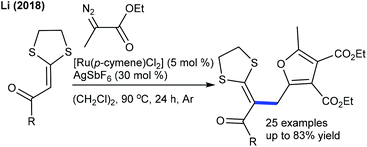 |
| Scheme 41 Furfurylation of α-acylketene dithioacetals with α-diazoketones. | |
Maleimides are not only an efficient source of alkenylation reactions, but have also been proved to be a competent alkyl resource. Rovis and co-workers presented three examples of syn-carboamination of alkenes including heterocyclic alkenes using a transient directing group (Scheme 42).60 The carboaminated products are obtained with electron-rich t-Bu-cyclopentadienyl cationic Rh-catalysis. The alkylation of the methyl group of 8-methylquinoline has been explored using maleimides (Scheme 43A).61 This reaction utilizes AdCO2H as an additive and a broad range of substrates are found to be successful. Later, the Sun group reported the same coupling by Cp*Co(III) catalysis (Scheme 43B).62 Diverse substrates with a range of functional groups are compatible; however, 8-ethylquinoline and unprotected maleimides are unsuccessful substrates. To overcome this issue, a slightly modified Co-based catalytic system has been introduced (Scheme 43C).63 Zhang and co-workers achieved coupling with enamides (Scheme 44).64 A wide range of enamides react; however, the cyclic enamide is an unsuccessful substrate.
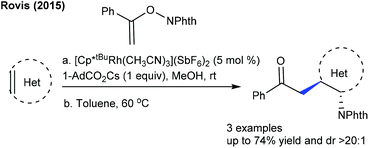 |
| Scheme 42
syn-Carboamination of heterocyclic alkenes. | |
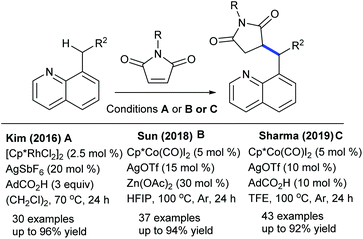 |
| Scheme 43 C(sp3)–H functionalization of 8-methylquinolins with maleimides. | |
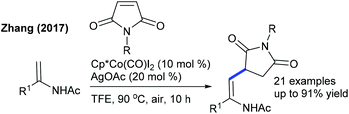 |
| Scheme 44 Coupling of enamides with maleimides. | |
The δ-C(sp3)–H alkylation of amino acids has been found to be successful by Pd-catalysis (Scheme 45).65 This procedure displays functional group tolerance and a series of amino acids are alkylated, though L-norvalines produce less yield owing to competing N,N-coordination. Dipeptides, tripeptides and tetrapeptides are viable, giving a mixture of diastereomers (up to 4
:
1). Recently, the Chatani group reported C–H alkylation of the ortho-methyl group of aryl amides (Scheme 46).66 Similar to Shi's procedure (Scheme 45), the preferential nature arises from the kinetically less favoured six-membered palladacycle in lieu of the kinetically favoured five-membered system, which is supported by DFT calculations. The mechanism involves the reversible C–H cleavage, migratory insertion of maleimide and protodemetalation.
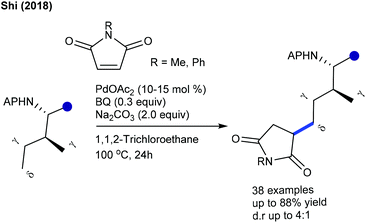 |
| Scheme 45 Alkylation of amino acids/peptides. | |
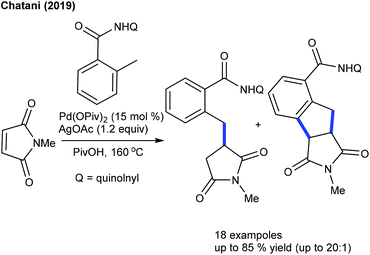 |
| Scheme 46 Alkylation of ortho-methylbenzamide with maleimides. | |
The Rh-(III) catalytic system is explored for the installation of an α-acyl alkyl group via C–H activation of arenes employing cyclic alkenyl carbonate under neat conditions (Scheme 47).67 Electron-deficient benzamides produce high yields, while the reaction of thiophene-2-carboxamide gives moderate yield. The products are converted to isocoumarin derivatives using AcOH.
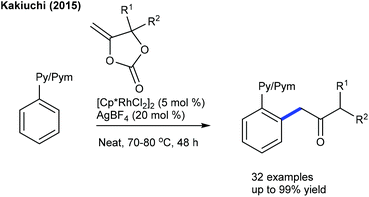 |
| Scheme 47 Coupling of arenes with cyclic alkenyl carbonates. | |
In 2016, the Peng group described the C6-alkylation of 2-pyridones with alkyl trifluoroborates (Scheme 48).18 Ester, alkenyl and amide group attached alkyl trifluoroborates are compatible. This strategy is extended to the coupling of diazo compounds (Scheme 49).68 A pyridine directing group produces superior results compared to pivaloyl, carbamoyl and methyl groups in N-protection. Low catalyst loading and mild reaction conditions are important features. Several electronically diverse pyridones, especially at the C3 position, give the best yield. The mechanism shows an involvement of the reversible electrophilic C–H activation. Furthermore, the coupling of acrylates is found to be successful (Scheme 50).52 Cu(OAc)2·H2O gives an acetate ligand to Rh and enhances the life time of the catalyst by reoxidizing the generated Rh(I) species. Good yields are obtained in polar and protic HFIP solvents. The reaction is compatible with a range of substituted pyridones and acrylates and the C–H activation step is reversible.
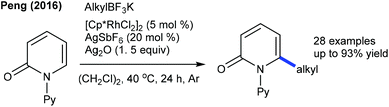 |
| Scheme 48 C6-Alkylation of pyridones. | |
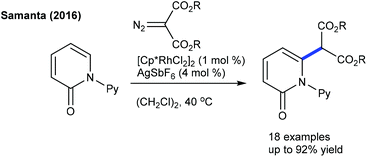 |
| Scheme 49 C6-Alkylation of pyridones using diazo compounds. | |
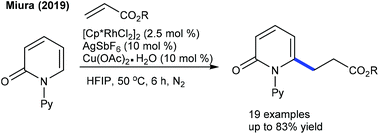 |
| Scheme 50 C6-Alkylation of pyridones with acrylates. | |
A rare example of C6-selective ring-contracting C–H alkylation of 2-pyridones is achieved (Scheme 51).69 This method is sensitive towards the steric and electronic features of pyridone. Apart from cyclooctadiene, norbornadiene, norbornene and styrene react, whereas 1,3-diene affords a mixture of annulated and addition products.
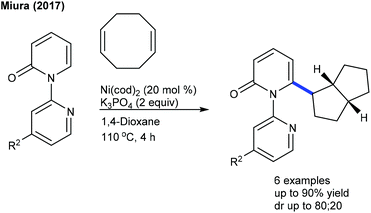 |
| Scheme 51 C6-Selective ring-contracting C–H alkylation of 2-pyridones. | |
5. C–H addition to C
C bonds
5.1. Hydroarylation
Hydroarylation of heterocyclic C
C bonds through C–H addition via chelation assistance has attracted much attention in recent years since it exemplifies a flexible atom-economical process. The Nishimura group reported a branch-selective hydroarylation of vinyl ethers, where the reaction of cyclic ethers occurs affording good yields (Scheme 52).70 The coupling of α,β-unsaturated γ-lactones with dihydrofuran is successful (Scheme 53).71 It is sensitive to the nature of the base and K2HPO4 or Na2CO3 affords the best results. ortho- and meta-substituted aryl amides give manoalkylation products and unsubstituted substrates produce dialkylation products, while α,β-unsaturated γ-lactones deliver diastereomeric mixtures.
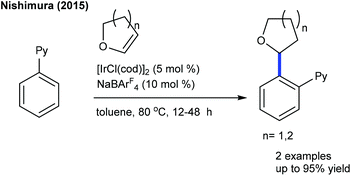 |
| Scheme 52 Hydroarylation of cyclic ethers. | |
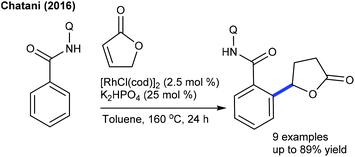 |
| Scheme 53 Alkylation with α,β-unsaturated γ-lactones. | |
Subsequently, an Ir-catalyzed enantioselective hydroarylation of alkenyl ethers is described through C–H activation and alkene isomerization (Scheme 54).72,73 They extended the reaction scope with differently functionalized aromatic ketones and 2H-chromenes. Ketones having electron-donating groups at ortho or para-positions exhibit the best ee. Furthermore, they designed a diastereoselective synthesis of α- and β-glycosyl arenes using an iridium/chiral BINAP catalytic system.74 Using (R)-BINAP provides a β-glycosyl arene, whereas the utilization of (S)-BINAP permits the generation of highly α-selective glycosyl arenes. A good to modest yield with excellent β-selectivity is obtained using (R)-BINAP with protected glycols, and (2-benzoimidazolinyl/2-benzoxazolyl) also acts as a good directing group. In contrast, acetophenone, acetophenone O-methyl oxime and N-(4-methoxyphenyl)-1-phenylethan-1-imine fail to react. Similarly, an S-BINAP based catalytic system gives the desired products with α-selectivity, while the selectivity drops when utilizing unprotected glycols. Pyrimidine, 2-benzoxazolyl and 2-benzothiazolyl are the workable directing groups, and the reaction of 1,3-di(2-pyridyl)-benzene/4,4′-di(pyridin-2-yl)-1,1′-biphenyl gives the dialkylated products in good yields.
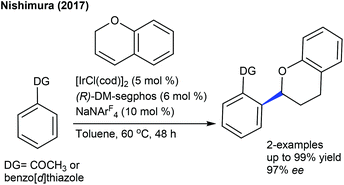 |
| Scheme 54 Hydroarylation of cyclic ethers. | |
Several studies are focused on the coupling of maleimides with aryl systems. The Prabhu group reported coupling with arylacetophenone (Scheme 55A).75 This protocol has some advantages such as the exclusive formation of the arylated product. This procedure is applicable to the coupling of N-benzoylindole,76 which provides 3-(indol-2-yl)succinimides in a regioselective manner. This transformation proceeds providing good yields but the substrate scope is narrow. This strategy is further extended to the coupling with aryl amides to produce 3-aryl succinimides;77 yet acetanilide gives less yield. Robust screening of the reaction conditions and directing ability of the various groups is covered. The C–C coupling with chromones has been successfully accomplished by Rh-catalysis (Scheme 55B).78,79 This reaction is effective in the presence of PivOH. However, a sterically hindered substrate fails to give the desired product, while an electron-deficient substrate displays poor reactivity.
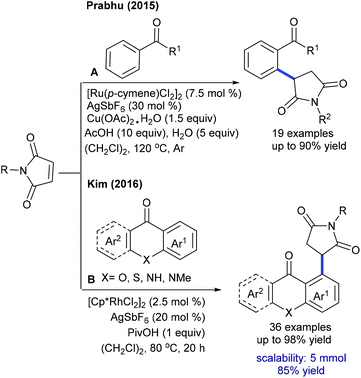 |
| Scheme 55 Coupling of arylacetophenone/chromenes with maleimides. | |
Ackermann and Prabhu groups demonstrated the Co-catalyzed coupling with arenes/indoles (Scheme 56A and B).80,81 An array of the substituents in the indole and maleimide are tolerated. Modified reaction conditions accommodate diverse aryl compounds.82 The hydroarylation of maleimides with indoles is successful in producing 2-indolylsuccinimides by Mn-catalysis (Scheme 56C).83 Among a series of Mn-sources screened, Mn2(CO)10 displays the best results. Several electronically diverse 2-pyridylindoles and maleimides are tolerated, but C-3 substituted indole is less reactive due to a steric effect. However, 2-pyridyl pyrrole and oxabicyclic alkenes efficiently react.
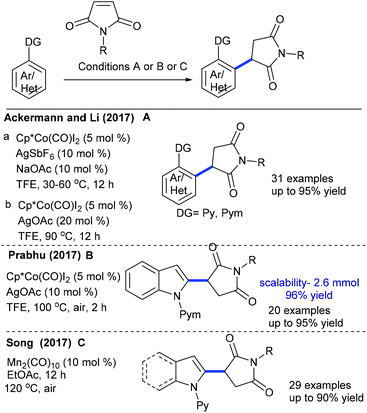 |
| Scheme 56 Hydroarylation of heteroarenes with maleimides by Co-catalysis. | |
Prabhu and co-workers reported carboxylic acids as traceless directing groups for the coupling of aryl nucleophiles.84 This procedure is highly sensitive towards the electronic nature of the aryl ring. Electron-rich aryl carboxylic acids react, while electron-deficient or moderately electron-rich substrates show inferior results. Even NH-free maleimide is amenable, whereas furan and pyrrole carboxylic acids are unsuccessful substrates. They also showed C-4 alkylation of indoles with maleimide (Scheme 57A).41 To attain finer results, 3 equiv. of AgOAc are essential. H/D exchange studies show 66% D incorporation at C4 and C2 positions, which reveals that C–H activation might be a reversible process. Later, Rh(I)-catalyzed coupling of aryl amides with maleimides has been achieved.85 The reaction is additive-free, and electronically diverse substrates are efficiently coupled. Furthermore, C7-coupling of indoline can be achieved.86 Electron-rich indoline gives the best yields, while electron-deficient substrates are less reactive. Besides, a diastereomeric mixture of products is observed when the substrates with multiple stereocenters are reacted. Subsequently, enone carbonyl directed ortho C–H bond coupling of arenes is demonstrated (Scheme 57B).87 A wide array of substituted chalcones react though a little influence of electronic effects is noted and the enone carbonyl group is essential to promote the C–H activation. The Cui group carried out ortho-alkylation of 2-aryl-1,2,3-triazole N-oxide (Scheme 57C).88 This method displays good efficiency using diverse substrates, while electron-deficient and sterically hindered substrates are less effective. In a related process, the Reddy group presented an ortho-C–H alkylation of N-sulfonylketimines (Scheme 57D).89 A broad range of ketimines and maleimides are compatible. However, electron-deficient N-sulfonylketimine, and n-butyl and Boc substituted maleimides are unsuccessful substrates.
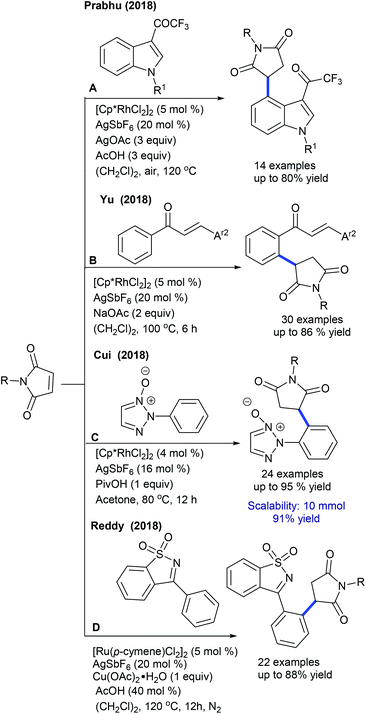 |
| Scheme 57 Coupling of maleimides with different aryl coupling partners. | |
Transient directing group assisted ortho-alkylation of aryl aldehydes is achieved (Scheme 58).90 The reaction proceeds selectively with high functional group tolerance. The reaction can be extended to aryl ketoximes by Co-catalysis (Scheme 59A).91 A series of oximes and maleimides are amenable; however, sterically demanding oxime is unfruitful. The mechanism shows an involvement of reversible fast C–H metalation. In addition, the coupling with aryl ketones is successful (Scheme 59B).92 High catalyst and additive loadings are required.
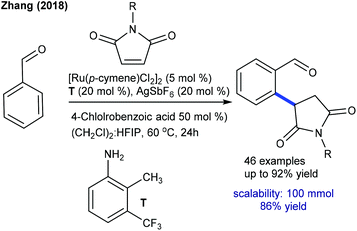 |
| Scheme 58
ortho-Alkylation of aryl aldehydes with maleimides with a transient directing group. | |
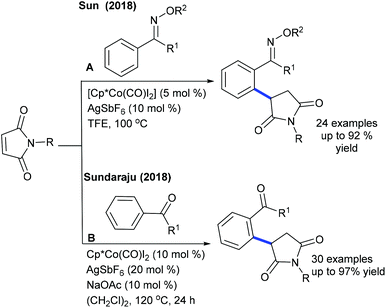 |
| Scheme 59 Hydroarylation of arenes with maleimides. | |
The C5-selective alkylation of 2-amino-1,4-naphthoquinones is found to be successful (Scheme 60A).45 This synthetic procedure is fairly similar to the previously reported methods. In addition, alkylation of cyclic amides is accomplished (Scheme 60B).93 Electronic and steric effects significantly affect the reaction. Maleimides having different substitution patterns couple successfully, while unsubstituted N–H maleimide is less reactive. An analogue approach is applied for the synthesis of succinimides via C-7 hydroarylation of indoline with maleimide (Scheme 60C).94 A pivaloyl directing group furnishes the best yield and addition of Zn(OTf)2 is valuable. Recently, the hydroarylation of diphenyl-1,2,4-thiadiazole with maleimide has been achieved (Scheme 60D).95 The use of an AcOH and Cu(OAc)2 mixture is important. This method shows broad substrate scope, although electron-poor diphenyl-1,2,4-thiadiazole is less reactive. Mechanistic studies involve the generation of a five-membered iridacycle with the reversible C–H cleavage (KIE = 2.0).
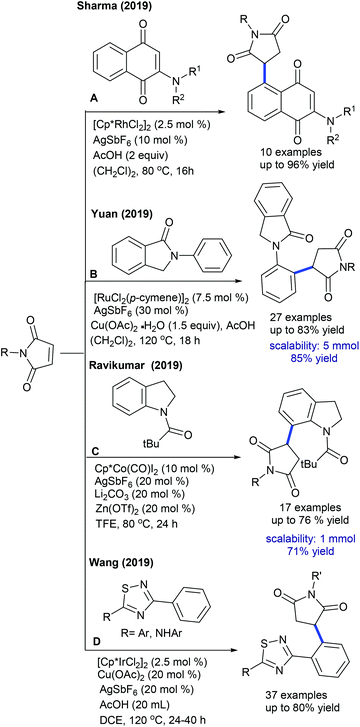 |
| Scheme 60 Coupling of maleimides with different aryl coupling partners. | |
Later, Baidya and Ackermann groups reported a Cu- and Ag-free decarboxylative coupling. The combination of [Ru(p-cymene)Cl2]2, Cy3PO and NaHCO3 is essential (Scheme 61A).96,97 Notably, electron-rich benzoic acids react faster, and the C–H activation occurs at a less hindered site when meta-substituted benzoic acids are utilized. The Ackermann group demonstrated the catalysis of [Ru(O2CMes)2(p-cymene)] with a broad range of aryl and alkyl alkenoic acids and maleimides (Scheme 61B).97 Kinetic isotopic experiments exhibit a negligible effect, indicating that C–H ruthenation is not a turn-over step. More recently, Shi and co-workers showed an environmentally benign protocol for the hydroarylation using benzoic acids in water (Scheme 62).98 Depending on the electronic properties of the benzoic acids, two kinds of addition products are formed viz. a conjugate or a decarboxylative conjugate.
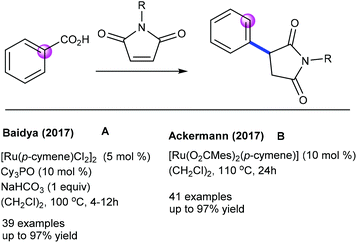 |
| Scheme 61 Alkylation of benzoic acids with maleimides. | |
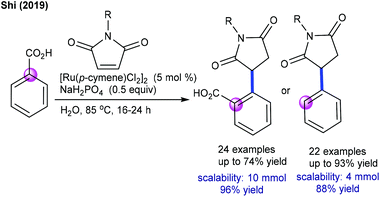 |
| Scheme 62 Alkylation of benzoic acids with maleimides in water. | |
Coumarin carboxylic acids are excellent coupling partners. The decarboxylative conjugate addition of coumarins with aryl pyridines is found to be successful (Scheme 63).99 The carboxylic acid group is essential, and a series of substrates react, while electron-deficient substrates show no reaction. In a subsequent study, they showed the stereoselective hydroacylation of bicyclic alkenes with 2-hydroxybenzaldehyde.100 Instead of [Ir(OH)(cod)]2, the use of IrCl(cod)2 or [Rh(OH)(cod)]2 shows no catalysis, while in the presence of KOH, the former gives comparable results, and the use of BINAP or (tBu)3P completely inhibits the reaction. Exo-products are observed; however, the scope of aldehydes is rather narrow. Later, they extended the hydroarylation of bicyclic alkenes to N-sulfonylbenzamides using a dippbz ligand.101
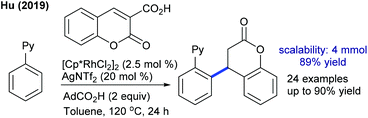 |
| Scheme 63 Synthesis of 4-arylchroman-2-ones. | |
The Miura group showed the coupling of oxa/azaheterobicyclic alkenes with aryl phosphoramides (Scheme 64).35 The electronic nature of the directing groups plays a critical role in achieving the selectivity. Bolm and co-workers disclosed a pioneering example for hydroarylation of heteroarenes with oxa/azabicyclic alkenes (Scheme 65).102 Dioxygen plays a decisive role in producing the alkylated product. Low catalyst loading (1 mol%) is required and a wide range of heteroarenes and bicyclic alkenes are viable.
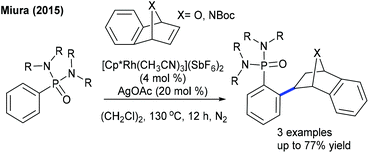 |
| Scheme 64 Functionalization of arylphosphoramides. | |
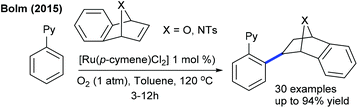 |
| Scheme 65 Hydroarylation of heteroarenes with oxa/azabenzonorbornadienes. | |
The hydroarylation of urea-derived bicyclic alkenes with heteroarenes is achieved (Scheme 66).103 The C–H activation takes place at the C-2 position of the heteroarene. Later, Chatani and co-workers reported an endo-selective hydroarylation of norbornene (Scheme 67).104 The reaction is quite efficient albeit high temperature is needed. The mechanism commences with the formation of Rh(I)X species a by the coordination of the substrate (Scheme 68). Electrophilic addition of the alkene provides complex b that undergoes a hydride shift to give the Rh–carbene complex c. Oxidative addition of the ortho-C–H bond to the Rh center furnishes the complex d, which can lead to the stereo-determining hydride migration to give e. Alternatively, d can convert to e via elimination and re-addition of HX, which cannot be excluded at this stage. Reductive elimination of e affords the product and regenerates the Rh(I) species.
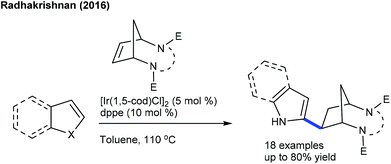 |
| Scheme 66 Hydroarylation of urea-derived bicyclic alkenes with heteroarenes. | |
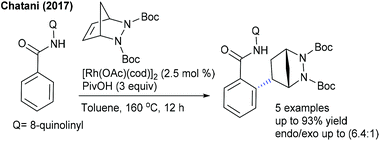 |
| Scheme 67
endo-Selective C–H hydroarylation of diazabicyclic alkenes. | |
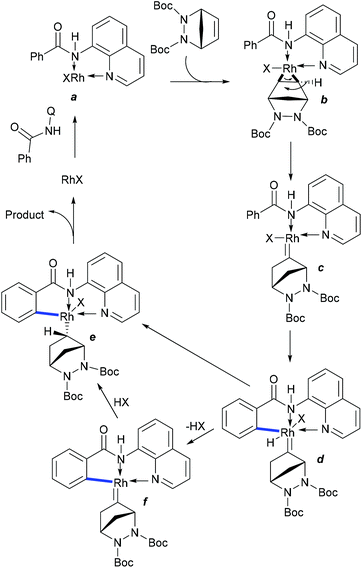 |
| Scheme 68 Mechanism of endo-selective C–H hydroarylation of diazabicyclic alkenes. | |
Later, the hydroarylation of arenes with heterobicyclic alkenes by Re-catalysis was found to be successful (Scheme 69).105 The reaction proceeds at high temperature (130 °C) in the presence of NaOAc. A wide range of arenes, heterobicyclic alkenes and heterocyclic azoles work effectively to generate the exo-products. Notably, 2-naphthyl pyridine gives a mixture of regioisomers.
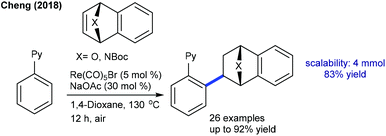 |
| Scheme 69 Reaction of arenes with oxabenzonorbornadienes. | |
Recently, the hydroarylation of oxa-/azabenzonorbornadienes with quinoline-N-oxides has been accomplished (Scheme 70).106 Electron-withdrawing group attached quinolone N-oxides are unsuccessful substrates. Furthermore, the chemo-divergent coupling of sulfoxonium ylides with oxa/azabicyclic alkenes is achieved (Scheme 71).107 The reaction gives a dialkylated bicycle retained product using PivOH. The electronic properties of aryl sulfoxonium ylides are compatible to give the dialkylated product, while ortho-/meta-substituted thienyl and naphthyl substrates give the monoalkylated products. Furthermore, six examples of ortho-C–H functionalization of phenols are achieved via the carbamination of heterobicyclic alkenes (Scheme 72).108 The ONHAc group acts as an oxidizing director, which permits the access to a diverse range of carbaminated products. Good yields are achieved using N-phenoxyamides with various bicyclic alkenes; however, the scope of the reaction is quite limited.
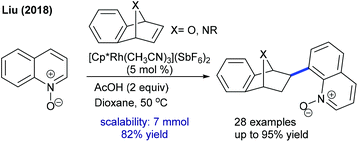 |
| Scheme 70 Hydroarylation of oxa-/azabenzonorbornadienes with quinoline-N-oxides. | |
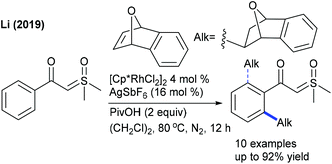 |
| Scheme 71 Dialkylation of sulfoxonium ylides with oxabicyclic alkenes. | |
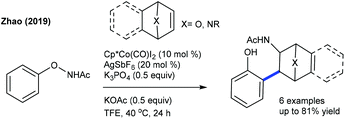 |
| Scheme 72 Carbamination of heterobicyclic alkenes. | |
5.2. Ring-opening and addition
These reactions focus on the use of heterobicyclic alkenes as the coupling partner due to the inherent reactivity of the strained systems. The reaction is initiated by C–H activation of arenes to form the M–C bond and the subsequent interaction of the strained rings leads to the ring-opening and addition products. In 2016, the Radhakrishnan group showed a Ru-catalyzed C-2 functionalization of indole with heterobicyclic alkenes (Scheme 73).103 Carboxamide serves as a directing group, which is cleaved at the end of the reaction. A substrate with a Ru catalyst can produce the ruthenacycle avia the C–H activation (Scheme 74). Cleavage of the C–N bond of the protecting group promotes the nucleophilic attack on the strained alkene to generate b. The C–N bond cleavage of the bicyclic alkene by the endo face via addition of acetate to the ruthenium species can generate c, which can undergo demetalation to afford the product and regenerate the Ru catalyst. Later, they have extended the strategy to the coupling of enamides using Rh-catalysis (Scheme 75).109 They further achieved the synthesis of cyclopentene functionalized aryl ketones with the transient directing group, where an imine acts as an autocleavable directing group.110 Typically, electron-rich aryl ketones produce the best results, while highly electron-deficient NO2 attached aryl ketones give inferior results.
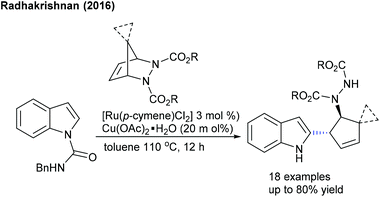 |
| Scheme 73 C2-Functionalization of indoles with heterobicyclic alkenes. | |
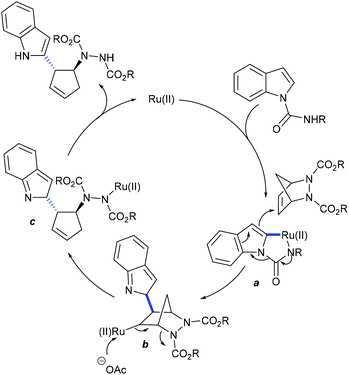 |
| Scheme 74 Mechanism of C2-functionalization indoles with heterobicyclic alkenes. | |
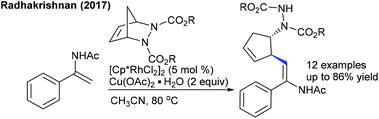 |
| Scheme 75 Desymmetrization of diazabicyclic alkenes with enamides. | |
The Glorius group disclosed a seminal example where [MnBr(CO)5] shows an excellent activity for the introduction of a cyclopentene unit in arenes with diazabicycles (Scheme 76).111 Good yields are observed under solvent-free conditions albeit the substrate scope is limited. This reaction involves a C–H activation and six-membered ring scission sequence.
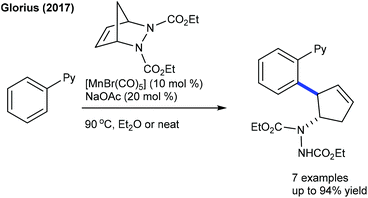 |
| Scheme 76 Ring-opening and addition of arenes with diazabicyclic alkenes. | |
Later, the Zhang group showed a similar type of vinylic C–H activation-desymmetrization of diazabicyclic alkenes with o-vinyl phenols (Scheme 77).112 The advantages of this protocol are low catalyst loading, moderate temperature, and short reaction time. This strategy is extended to the coupling of anilide with 7-azabicyclic alkene.37 A range of substrates are compatible, while ortho-substituted anilides are less reactive. Kinetic isotope studies (KIE = 2.3 (competitive) and 2.7 (parallel)) suggest that the reaction involves the CMD pathway and the C–H cleavage might be the rate-determining step.
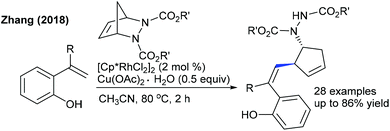 |
| Scheme 77 Vinylic C–H activation-desymmetrization of diazabicyclic alkenes. | |
Li and co-workers developed the coupling of indole with 7-azabenzonorbornadiene using a Cramer-type CpxRh(III) complex (Scheme 78).113 In search of additives, AgOAc/Ag2SO4 proves to be a good choice, which activates the alkene and facilitates the C–H activation. A variety of indoles and azabenzonorbornadienes tolerate the reaction. 7-Substituted indoles provide moderate ee, whereas 7-F indole affords high ee. The enantioselective model encompasses rapid rhodacycle formation (KIE = 1.3), alkene insertion and stereospecific β-nitrogen elimination. Subsequently, they came up with a seminal example of enantioselective [3 + 2]-annulation of benzamides with azabicyclic alkenes.114N-Alkyl substituents plays an indispensable role in the reactivity and selectivity and mainly N-cyclopentyl substituted amides provide a favourable result. The product yield and selectivity are significantly increased when the reaction is performed using a mixture of anisole and MTBE as a solvent.
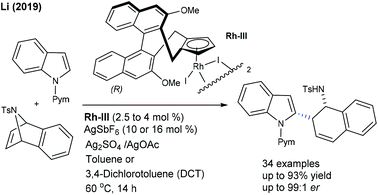 |
| Scheme 78 Coupling of indole with 7-azabenzonorbornadienes. | |
The Cramer group pioneered the enantioselective synthesis of cyclopentylamines employing a chiral CpxRh(III)-catalyzed C–H activation and ring-opening sequence (Scheme 79).115 On screening of the chiral Rh precatalysts having different alkene partners, and different benzoate sources, CpxRhI(cod) and bis(o-toluoyl) peroxide give the best results. The nature of alkenes and benzoate sources is largely influences in the reactivity and selectivity. The reaction tolerates a variety of oximes and diazabicycles, providing good ee. Subsequently, they evaluated a series of CpxRh(III) catalysts with differently substituted chiral backbones. MeO-biphep type catalysts show excellent reactivity.116
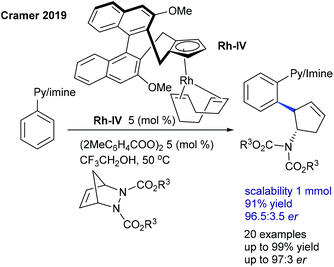 |
| Scheme 79 Ring-opening reaction of arenes with diazabicyclic alkenes. | |
Fan and co-workers reported the ring-opening C–H addition of azabenzonorbornadienes with N-sulfonyl ketimines (Scheme 80).117 Both the coupling partners react well, while a slightly lower yield is observed with the sterically congested substrates. Oxabenzonorbornadienes react albeit providing lower yield. They tried to achieve the asymmetric version employing chiral ligands such as (R)-BINAP, (R)-Difluorphos, (R,S)-PPF-PtBu2 and (R)-SIPHOS-Ph-Mor. This strategy has been extended to the coupling of aryl ketoximes (Scheme 81).118 The reaction is related to Fan's work, however, without the use of an additive. 2-Arylated hydronaphthylamines are formed, which can lead to 13,14-dehydrobenzophenanthridine using HCl. Mechanistic studies include the isolated rhodacycle and H/D labelling experiments.
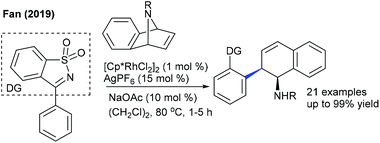 |
| Scheme 80 Coupling of 7-azabenzonorbornadiene with N-sulfonyl ketimines. | |
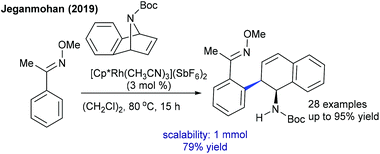 |
| Scheme 81 Coupling of azabenzonorbornadienes with aromatic ketoximes. | |
6. Annulation/cyclization
These reactions have been greatly explored. The tandem C–C and C–N coupling of methyleneoxetanones with N-phenoxyacetamides is pursued to produce chromene-3-carboxylic acids.119 The reaction is extended to solvent-controlled cyclization with benzamides. Compared to allylation, 2.5 mol% catalyst is sufficient to carry out the benzoazepine synthesis. β-H elimination is the key step for the product selectivity. These reaction conditions with a slight modification are studied for the coupling of N-aryl urea to furnish quinolones (Scheme 82).120 Product selectivity depends on the solvent choice. For instance, a cyclized product is observed in TFE. Furthermore, this strategy is successful for the [4 + 3]-annulation with 2-aryl-1H-imidazoles to give benzoazepines (Scheme 83).121 In 2-aryl-1H-imidazole, the aryl part with meta-OMe gives a mixture of regioisomers, and especially the C–H activation occurs in a more hindered site when the reaction of the meta-fluoro derivative is studied. Waldmann groups developed the synthesis of JasCp ligands for enantioselective C–H activation of benzamides with alkenes including dihydrofuran (Scheme 84).122
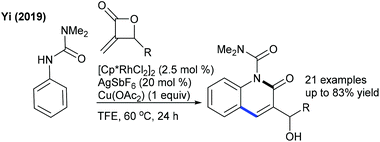 |
| Scheme 82 Reaction of N-aryl ureas with methyleneoxetanones. | |
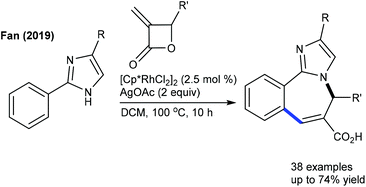 |
| Scheme 83 Annulation of 2-aryl-1H-imidazoles with methyleneoxetanones. | |
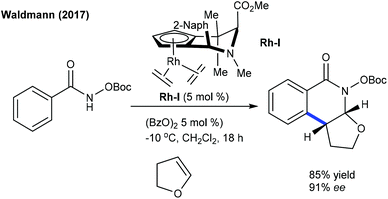 |
| Scheme 84 Coupling of benzamides with dihydrofuran. | |
Miura and co-workers presented the oxidative coupling of benzamides with maleimides (Scheme 85A).123 The use of Cy2NMe improves the chemoselectivity and accelerates the reaction. Furthermore, the annulation with 7-azaindole is successful via a double C–H activation strategy to provide spiro products in the presence of AcOH (Scheme 85B).124 The oxidant-free coupling with benzimidates is successful in affording spirosuccinimides (Scheme 85C).125 Excellent yields of the desired products are acquired with a series of substituted benzimidates and maleimides. Jeganmohan and co-workers focused on the synthesis of isoindolone spirosuccinimides (Scheme 85D).126 The reaction involves a Co(I)/Co(III) catalytic cycle with irreversible fast C–H activation.
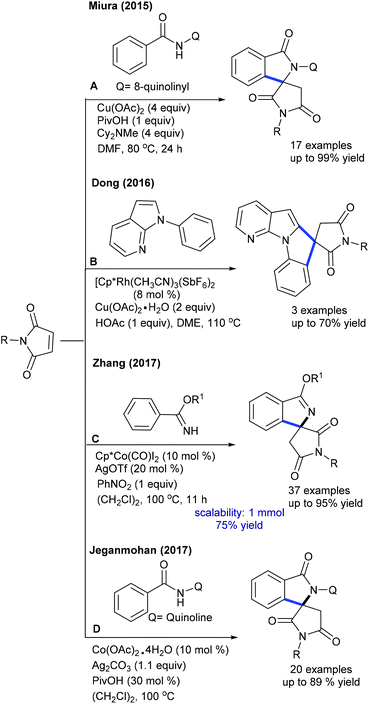 |
| Scheme 85 Oxidative coupling of benzamides/arenes with maleimides. | |
Later, the assembly of spirosuccinimides via directed C–H spirocyclization is achieved (Scheme 86A).127 Functional group tolerance is an important feature, whereas the steric effects influence the reactivity. The directing group is cleavable via reductive cleavage by SmI2. Recently, the use of oxidizing directing groups has attracted considerable attention because they obviate the utilization of stoichiometric external oxidants, and the C–H activation proceeds via the redox economy under mild reaction conditions.128 The use of N–O oxidizing group directed C–H activation and 1,1 cyclization has been demonstrated for the coupling with N-methoxy benzamides (Scheme 86B).129 Various functional groups tolerate the reaction, though a sterically encumbered substrate shows less reactivity. Interestingly, the reaction of 2-thienyl benzamide furnishes the alkenylated product instead of a cyclized one. A similar type of coupling is accomplished with sodium benzoate (Scheme 86C).44 This solvent controlled cationic Rh-based reaction is applied for a small library of substrates. Furthermore, the coupling with pyrrolidinediones is studied to produce polyheterocycles (Scheme 86D).130 This low catalyst loading method is applicable to diverse substrates to give the spiroproducts. The mechanism involves the reversible C–H cleavage (KIE = 2.33), migratory insertion of maleimide, E2-elimination and aza-Michael addition. Tandem reaction of maleimides with styrenes is accomplished using pseudo-Diels–Alder reaction (Scheme 87).131 Substrates with various substitution patterns are compatible.
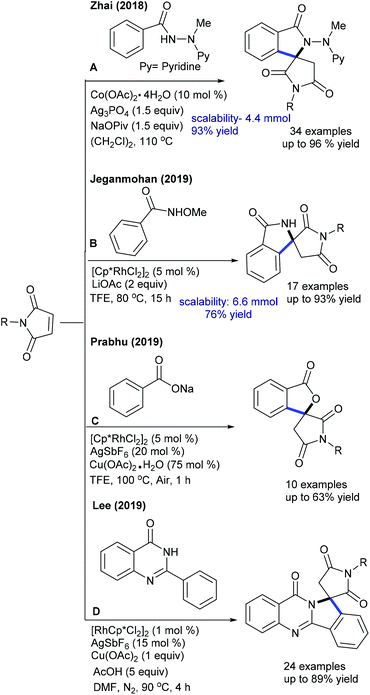 |
| Scheme 86 Synthesis of polyheterocycles bearing pyrrolidinediones. | |
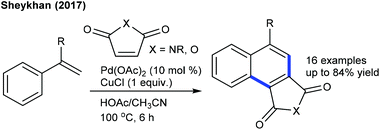 |
| Scheme 87 Coupling of styrenes with maleimides. | |
The coupling with N-sulfinyl ketoimine has been found to be effective.132 An in situ formed cationic Rh-catalyst affords superior results compared to a cationic Rh-complex, and the N-tert-butylsulfinyl group plays a critical role. In addition, the coupling with azobenzenes produces oxindoles via a Rh-catalyzed C–H activation and Zn-mediated reductive cyclization.133 These reaction conditions are extended to [3 + 2]-annulation with cyclic N-acyl ketimine to yield spiroisoindolinones,134 while the coupling with N-sulfonyl aldimines readily takes place to afford 1-aminoindanes (Scheme 88A).135 Pertinently, the N-sulfonyl part in aldimine is important, and on switching from the sulfonyl to aryl/alkyl/alkylsulfinyl group, the reaction fails. The Luo group has overcome the impediment of this procedure, as unactivated aromatic aldimines/ketimines are utilized for the synthesis of 1-aminoindanes.136 This neutral Rh/Cu-based catalytic system is suited for a wide range of cyclic/acyclic ketimines/aldimines. Particularly, the electron-rich cyclic ketimines provide the best yields. In addition, the Jeganmohan group showed the Ru-catalyzed cycloaddition of N-sulfonyl aryl aldimines with maleimides (Scheme 88B).137 The N-sulfonyl directing group and acetate ion sources are crucial for the success of this reaction. This strategy is extended to the construction of succinimide linked oxindoles.138 An excess of Cu(OAc)2·H2O is required and several N-aryl acrylamides react with a range of maleimides, while a mixture of regioisomers is obtained with meta-halogen substituted aryl acrylamides. This strategy is extended to an oxidative [3 + 2]-annulation of 2-acetyl-1-arylhydrazines to give pyrrolo[3,4-b]indole-1,3-diones (Scheme 88C).139 Hydrazine with an electron-withdrawing group displays less reactivity. Furthermore, the [4 + 2]-annulation of N-chlorobenzamides with maleimides is achieved (Scheme 88D).140 The use of the N–Cl group plays a foremost role, whereas other oxidizing directing groups such as N–OMe and N–OH are unreactive. However, the sterically congested benzamide is less reactive. Variations of alkyl protected maleimides are applicable to afford the products in good yields.
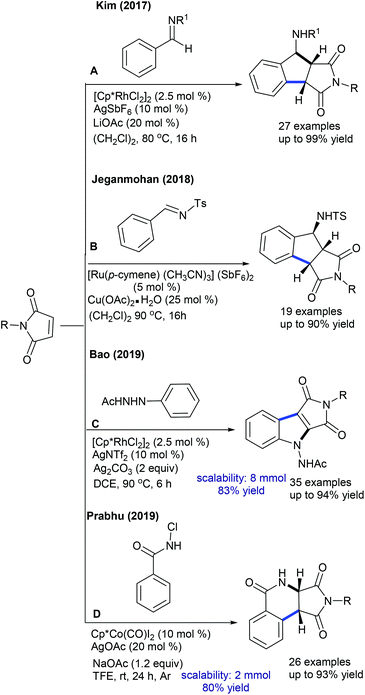 |
| Scheme 88 Synthesis of 1-aminoindanes/fused heterocycles. | |
The coupling of maleimides has been further extended to the construction of fused and spiro polyheterocycles (Scheme 89).141 AdCO2H serves as an additive to give fused heterocycles, while a spiro compound is formed using diisopropylethylamines. This switchable procedure is utilized to access fused or spiro polyheterocycles, though the basic conditions give a mixture of products. The mechanism involves Rh(III)/Rh(I) catalysis and kinetic isotope studies (KIE = 5.3) suggest that the C–H activation step might be the rate-limiting step. The Wang group described the arylation of enamides (Scheme 90).142 Addition of a Brønsted acid promotes the reaction and O2 serves as the oxidant. This protocol affords a small panel of fused N-heterocycles. A mixture of regioisomers is generated in 3-substituted substrates. Aporhoeadane alkaloids palmanine, lennoxamine and chilenamine can be synthesized in three or four steps.
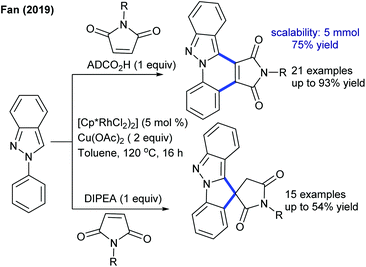 |
| Scheme 89 Oxidative annulation of 2-aryl indazoles with maleimides. | |
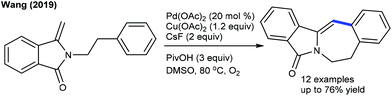 |
| Scheme 90 Direct intramolecular arylation of enamide by C–H activation. | |
Recently, the Miura group has found the coupling of vinylene carbonate with benzamides (Scheme 91).143 An external oxidant and a base are not required, as vinylene carbonate itself acts as an internal oxidant and acetylene surrogate. The tandem C–C coupling of α-benzoylketene dithioacetols with α-diazoketones is found to be successful in the presence of PivOH to give naphthalenones (Scheme 92).59 This double C–H activation shows good functional group tolerance but the reaction of α-diazomalonate gives an uncyclized product.
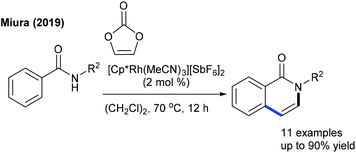 |
| Scheme 91 Annulative coupling of vinylene carbonate with benzamide. | |
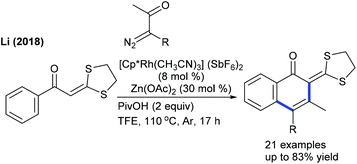 |
| Scheme 92 Coupling of α-benzoylketene dithioacetols with α-diazoketones. | |
Few studies are focused on the coupling of alkynes to lead to polycyclic scaffolds. Redox-neutral coupling with 4-phenyl-1,3-oxazol-2(3H)-ones is realized to give spiro[indene-1,4′-oxazolidinones] (Scheme 93).144 The reaction tolerates a broad range of substrates. An electron-rich alkyne couples efficiently, and even unsymmetrical and heterocyclic alkynes work albeit providing moderate yields. This strategy is extended to the oxidative annulation of antipyrine with alkynes via Ru catalysis (Scheme 94).145 A broad range of substrates react, while unsymmetrical alkynes give a mixture of regioisomers. Ru(p-cymene)Cl2 with Cu(OAc)2 can generate the active Ru(II) catalyst a, which can react with substrate via directed irreversible C–H to produce b (Scheme 95). Chelation of the alkyne and subsequent migratory insertion and cleavage of the N–N bond can produce the Ru(IV) complex c. Reductive elimination of Ru-metal and additional C–H activation of the substituted phenyl ring of the indole derivative can deliver the nine-membered Ru-complex d. Elimination of the ketene type fragment can give the ring contracted Ru-complex e. Another molecule of alkyne insertion can give f that can lead to reductive elimination to furnish the product and Ru species, which is readily oxidized by Cu(OAc)2 to fulfil the catalytic cycle.
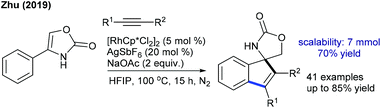 |
| Scheme 93 Coupling of 4-phenyl-1,3-oxazol-2(3H)-ones with alkynes. | |
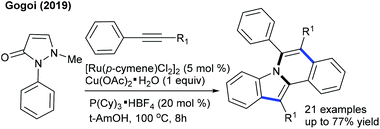 |
| Scheme 94 Oxidative annulation of antipyrine with alkynes. | |
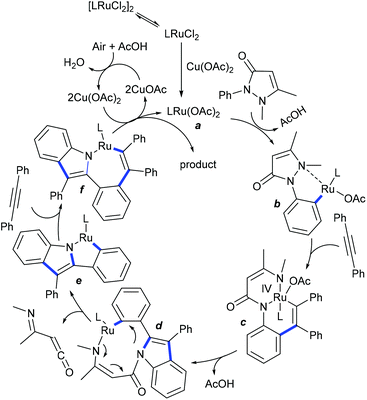 |
| Scheme 95 Mechanism of Ru-catalyzed oxidative annulation. | |
Rh-Catalyzed coupling of acrylates with heterocyclic alkenyl carboxylic acids can be achieved to give furan-2(5H)-ones (Scheme 96).146 High oxidant loading and longer reaction time are the notable issues. The reaction utilizes the carboxylic acid directed C–H activation, migratory insertion, β-hydride elimination and Michael addition processes. While many advances are made in the annulation of various coupling partners to diverse heterocycles,147 limited studies are focused on the synthesis of spirocyclic compounds. The Peneau group showed the synthesis of spiropiperidines (Scheme 97).148 The differently substituted aryl ring, linkers and ring-size of the N-heterocycles are tolerated to provide the target spirocycles, which can be converted to tetracyclic compounds by treatment with TFA. An electron-withdrawing protecting group on the nitrogen is necessary; however, a Cbz protected variant is an unsuccessful substrate.
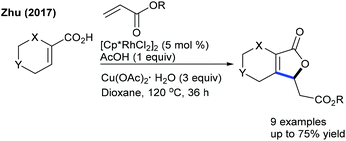 |
| Scheme 96 Reaction of heterocyclic alkene carboxylic acid with acrylates. | |
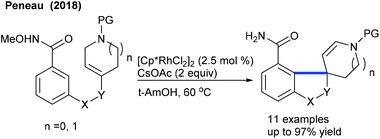 |
| Scheme 97 Synthesis of spiropiperidine derivatives. | |
The Stanley group showed two examples of enantioselective Rh-catalyzed hydroacylation and α-epimerization (Scheme 98).149 (R)-DTBM-Segphos is used as a chiral ligand and NaBARF (BARF = tetrakis(3,5-bis(trifluoromethyl)phenyl)-borate) acts as an additive, which allows the intramolecular coupling of 2-(3,6-dihydro-2H-pyran-4-yl)benzaldehydes to provide the products with up to 97% ee. In contrast, other heterocyclic alkenes such as nitrogen and sulfur analogues are unsuccessful substrates.
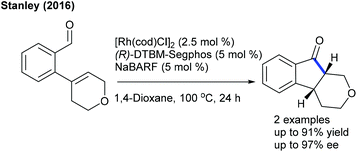 |
| Scheme 98 Hydroacylation/α-epimerization of 2-(3,6-dihydro-2H-pyran-4-yl)aryl aldehydes. | |
Few studies are focused on the use of Ni-catalysis. The Cramer group reported regio-divergent annulation of pyridones (Scheme 99).150 1,6-Annulated pyridones are obtained and the ligand controls the mode of cyclization. For instance, cyclooctadiene fosters exo-cyclization, and the bulky N-heterocyclic carbene ligand induces endo-cyclization. Substrates bearing 1,2-disubstitution across the double bond are coupled via both the modes. Furthermore, the enantioselective synthesis of 1,6-annulated 2-pyridones exploiting a chiral N-heterocyclic carbene L1 is successful. The reaction proceeds through endo-cyclization to give the product in moderate ee. In continuation, the screening of various chiral N-heterocyclic carbene ligands is performed. A sterically congested NHC-ligand provides the best selectivity.151 2-Pyridones and 4-pyridones cyclize to enantiopure products, while cis- or trans-alkenes react well, albeit with lower selectivity.
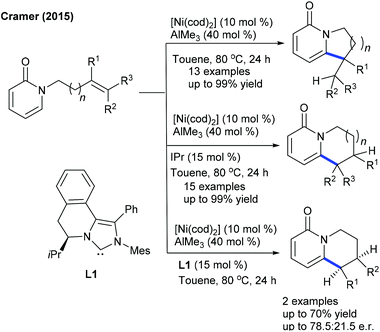 |
| Scheme 99 Regio-divergent annulation of pyridones. | |
The Katsina group showed the coupling of alkenes with 4-hydroxy-2-pyridones to give furo[3,2-c]-pyridones (Scheme 100).152 Product selectivity depends on the nature of the solvent and additives. The reaction using HCOOH as an additive in CH3CN provides the cyclized product along with a minor amount (>10%) of its regioisomer. A small panel of alkenes are studied, with 2-pentene and α-pinene affording uncyclized products. Electron-rich/unactivated alkenes are less reactive and the substrate scope is somewhat limited. A substrate with Pd(OAc)2 can provide avia C–H activation (Scheme 101). Migratory insertion of pyridone–palladium species b to the alkene can give c, which can lead to reductive elimination to yield d or the alkene and Pd(0) species that is oxidized by Cu(OAc)2 to complete the catalytic cycle.
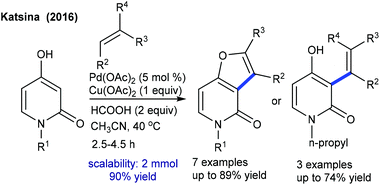 |
| Scheme 100 Synthesis of functionalized furo[3,2-c]-pyridones. | |
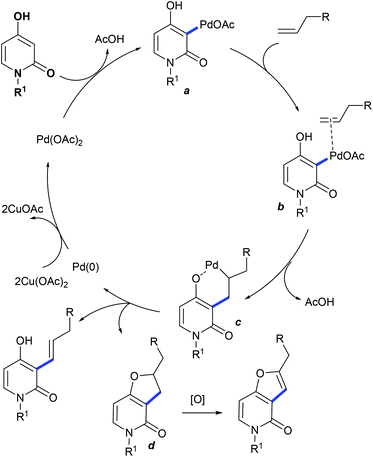 |
| Scheme 101 Mechanism for the synthesis of functionalized furo[3,2-c]-pyridones. | |
The regioselective arylation of 2-pyrones has been found to be successful.153 PivOH significantly improves the reaction yield. A series of substrates are studied; however, 6-benzyl and 6-(4-iodophenyl) derivatives are unsuccessful substrates and a C-3 substituted 4-fluorophenyl derivative produces a mixture of regioisomers, while 2-coumarin, 2-pyridone and 2-quinolone are competent, and the procedure is also compatible with the arylation of 3-halo-2-pyrones.154 PPh3 is suitable for 3-bromo-2-pyrones, while 3-chloro-2-pyrone produces the best results using the bulkier PCy3·HBF4. Ligand-free C5-arylation of pyrones and pyridones can be achieved (Scheme 102).155 Blocking at the C3-position with a chlorine atom is essential; however, the protocol suffers from limited substrate scope. The cyclization of 4-phenoxy coumarin can be realised via double C–H activation.156 This method obviates the use of activating groups (bromo and iodo groups), while it needs high temperature (140 °C). The synthesis of flemichapparin C is covered and the mechanism involves the reversible C–H activation (KIE = 1.08).
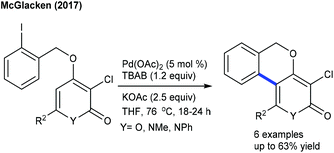 |
| Scheme 102 Intramolecular C5-arylation of pyrones/pyridones. | |
The Miura group reported the C6-borylation of pyridones (Scheme 103).157 This procedure is highly sensitive towards the electronic nature of the substrates. The products are useful compounds for further synthetic transformations albeit limited substrates scope is an issue of this process. Propargyl alcohols are coupled with 2H-[1,2′-bipyridin]-2-one (Scheme 104).158 The reactivity of both the coupling partners is significantly disturbed by the steric and electronic nature of the functional groups. This strategy is extended to the coupling of anthranils with pyridones to produce quinolone-fused polycycles.159 Internal alkynes can be coupled with pyridones/quinolones (Scheme 105).160,161 The reaction involves the well-established Rh(III)/Rh(I) catalytic cycle and the final π-conjugated heterocycles display a good sensing ability in the detection of nitroaromatics. In addition, the diheteroarylation of allenes is successful via the integration of C–H activation and a Smiles rearrangement (Scheme 106).162 These reaction conditions are extended to C–H prenylation of pyridine and isoquinolone with allenes employing NaOAc.163 However, these reactions are limited to heterocyclic alkenes.
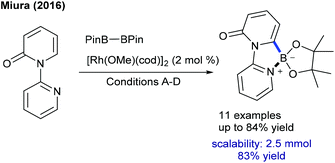 |
| Scheme 103 C6-Borylation of pyridones. | |
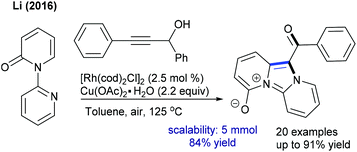 |
| Scheme 104 Alkenylation and concomitant intramolecular annulation. | |
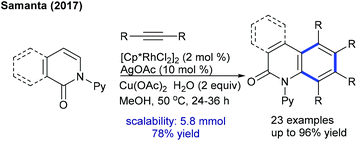 |
| Scheme 105 Oxidative coupling of pyridones with alkynes. | |
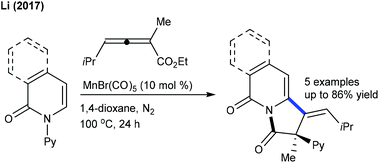 |
| Scheme 106 Diheteroarylation of allenes. | |
Recently, Ackermann and co-workers pioneered a pivotal example of the synthesis of structurally complex polycycles via a C–H activation, Diels–Alder reaction and alkyne annulation sequence (Scheme 107).164 A variety of pyridones and substituted propargylic carbonates participate with good yields. The proposed mechanism involves a reversible fast C–H cleavage (KIE = 1.2) via chelation assistance to afford a manganacycle a (Scheme 108). Alkyne insertion leads to the formation of b. Subsequent β-oxygen elimination provides c and regenerates the active catalyst. A BPh3-mediated Diels–Alder reaction with cyanide elimination via retro-Diels–Alder reaction affords the final product.
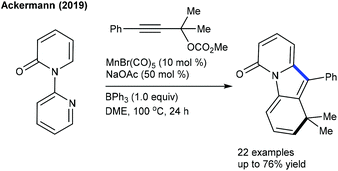 |
| Scheme 107 Synthesis of structurally complex polycycles. | |
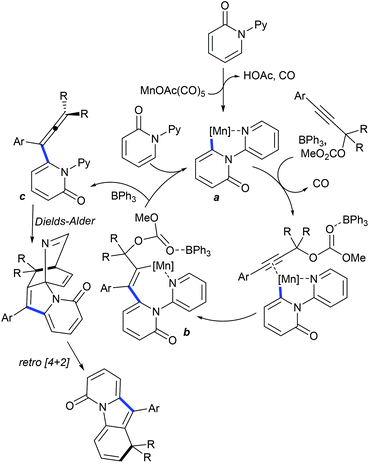 |
| Scheme 108 Catalytic cycle of Mn-catalyzed synthesis of complex polycycles. | |
The Wang group reported a [4 + 2]-cycloaddition of heterocycles including pyridones and isoquinolones with 1,3 dienes (Scheme 109).165 The mechanism involves Rh-catalyzed C–H activation to produce rhodacycle a (Scheme 110). Subsequent coordination and insertion of a 1,3-diene can form the σ-allyl metal species b, which presumably exists in equilibrium with the π-allylmetal species c, which may undergo intramolecular nucleophilic allylic substitution to deliver d that on deprotonation can give e. The latter can convert to the product via an oxidation process. This strategy has been extended to the coupling of pyridones with 1,3-enynes to give quaternary ammonium salts.166
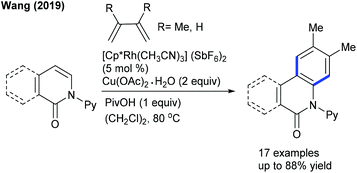 |
| Scheme 109 [4 + 2]-Cycloaddition of pyridones/isoquinolones with 1,3 dienes. | |
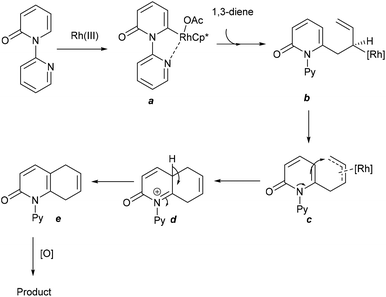 |
| Scheme 110 Reaction pathway of [4 + 2]-cycloaddition of pyridones/isoquinolones with 1,3-dienes. | |
The carbonylation of 2-phenol chromone can be pursued to produce frutinone (Scheme 111).167 Solvent plays the profound role, while Cu(OAc)2 acts as the oxidant. A series of substrates are tolerated, with some derivatives inhibiting the activity of the CYP1A2 enzyme. Activation of the C–H bond occurs through electrophilic palladation. Hu and co-workers focused on intramolecular C–C coupling of coumarins to produce indole[3,2-c]coumarins (Scheme 112).168,169 Method A utilizes air as the oxidant and excludes the use of an additional base though it needs higher catalyst loading (15 mol%), while method B uses AgOAc as the oxidant. The former is typically suitable for unsubstituted coumarin, while the later works well for the substituted coumarin. This method is extended to the synthesis of indolo[3,2-c]pyrones and indolo[3,2-c]quinolinones. Later, a couple of reports appeared on the synthesis of indole[3,2-c]coumarins that are closely related to the earlier methods.170 A similar strategy is applied to benzothiophene synthesis (Scheme 113).171ortho-/para-Functionalized thiocoumarin/pyrone react well, while, the reaction of meta-functionalized substrates is not examined.
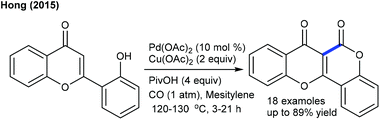 |
| Scheme 111 Carbonylation of 2-phenolchromone. | |
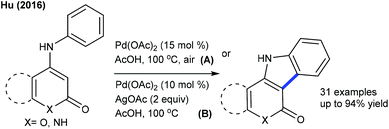 |
| Scheme 112 Synthesis of indole[3,2-c]coumarins. | |
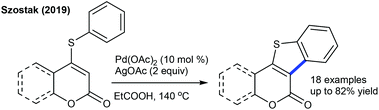 |
| Scheme 113 Synthesis of benzothiophenes. | |
Apart from intramolecular C–C couplings, tandem inter and followed by intramolecular C–C couplings has also been explored.172–174 Diaryliodinium salts couple with coumarin to yield 4,5-benzocoumarins (Scheme 114).173 The reaction tolerates a broad range of functional groups, although disubstituted coumarins are less reactive. Furthermore, the coupling of 4-hydroxycoumarin with an aryne (generated in situ) is successful (Scheme 115).174 Electron-deficient coumarins exhibit greater reactivity compared to electron-neutral/rich substrates. Mono-substituted arynes provide a mixture of regioisomers and the synthesis of flemichapparin C is covered. The proposed catalytic cycle begins with the Pd-salt a of 4-hydroxycoumarin (Scheme 116). Formation of the Pd(II) species b and the subsequent C-3 C–H activation occur to produce c, which undergoes carbopalladation of the aryne to afford d. Reductive elimination gives the product and Pd(0), which is reoxidized to Pd(II) by Cu(OAc)2 to resume the catalytic cycle.
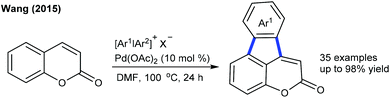 |
| Scheme 114 Vicinal double C–H activation of diaryliodinium salts with coumarins. | |
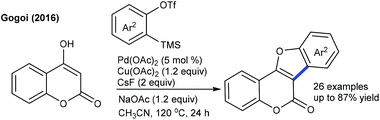 |
| Scheme 115 Oxidative annulation of 4-hydroxycoumarins with an aryne. | |
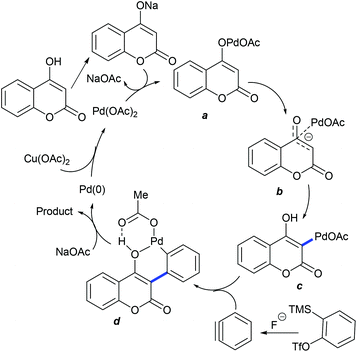 |
| Scheme 116 Mechanism of coumestan synthesis from 4-hydroxycoumarins and benzyne. | |
Swamy and co-workers reported carboxamide directed C–C and C–N couplings of 2H-chromenes with alkynes to produce benzopyrone-fused pyridones (Scheme 117).175 The yields are good, although the substrate scope is rather constrained with respect to 2H-chromene. Double C–H activation is achieved with an excess alkyne; however, high catalyst loading (8 mol%) and elevated temperature are essential. Later, the coupling of alkynes with coumarinyl ketoxime esters can be accomplished under redox-neutral conditions (Scheme 118).176 A set of functionalized coumarinyl ketoxime esters are converted into the target compounds, while unsymmetrical alkynes give a mixture of regioisomers. The scope of alkynes is limited since the alkyl substituted alkynes and Z-ketoximes display no reaction.
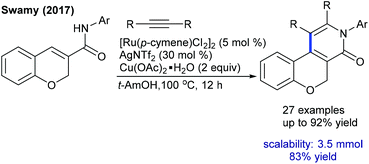 |
| Scheme 117 Assembly of benzopyrone-fused pyridones. | |
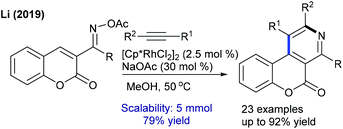 |
| Scheme 118 Annulation of coumarinyl ketoxime esters with alkynes. | |
Heterobicyclic alkenes can be coupled by different pathways to produce the annulated compounds. In 2016, the coupling of alkynol with oxa/azabicyclic alkenes has been achieved (Scheme 119).177 Using synergistic Rh(III) and Sc(II) catalysis, a broad range of substrates can be coupled. In the same year, the Co-catalyzed diastereoselective [3 + 2]-cycloaddition of bicyclic alkenes with aromatic amides was achieved (Scheme 120).178 A wide range of substituted aromatic amides and oxabicyclic alkenes are efficiently coupled. However, azabicyclic alkenes show inferior results. The Bolm group accomplished a similar annulation of N-methoxybenzamides with heterobicyclic alkenes (Scheme 121).179 Electron-rich oxabenzonorbornadienes give superior results. Similarly, the coupling of N-methoxyindazomides can be accomplished with heterobicyclic alkenes under Rh–Ag catalysis, which provides diverse indolo[3,2-c]heteroarenes.180
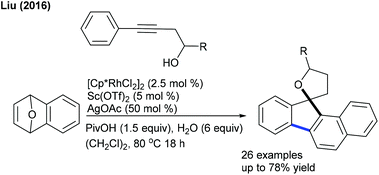 |
| Scheme 119 Synthesis of spirocyclic dihydrobenzo[a]fluorenefurans. | |
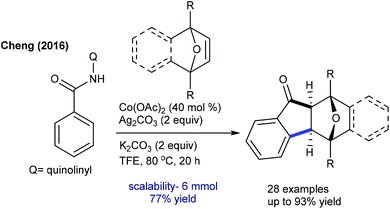 |
| Scheme 120 [3 + 2]-Cycloaddition of aromatic amides with bicyclic alkenes. | |
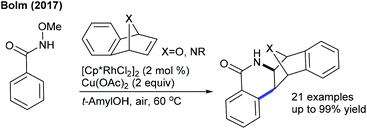 |
| Scheme 121 Annulation of N-methoxybenzamides with heterobicyclic alkenes. | |
The Glorius group came up with a rare example of the synthesis of polycycles using the combination of C–H activation and Wagner–Meerwein-type reaction181 of N-phenoxyacetamide with azabenzonorbornadiene (Scheme 122). NMR studies reveal that the reaction of a rhodacycle with an alkene at low temperature yields an alkene inserted Rh-complex and produces an unstable spirocyclopropane (detected by NMR), which on treatment with AcOH undergoes a rearrangement to give the product. The proposed catalytic cycle starts with the formation of rhodacycle avia irreversible fast C–H metallation (KIE = 1.0) (Scheme 123). Insertion of the alkene generates the seven-membered rhodacycle b. Protonation using AcOH furnishes c, which readily transforms to fvia an intramolecular nucleophilic attack and acid promoted rearrangement of d to deliver the product. The protonation of e releases the active Rh(III) catalyst to complete the catalytic cycle. Later, they accomplished the dearomatization of N-(naphthalen-1-yloxy)acetamides with alkenes.182 Oxabenzonorbornadienes are the amenable coupling partner. The naphthyl ring stabilizes the spirocyclopropane product. Furthermore, the synthesis of polycyclic hydrocarbon bearing cyclobutadienoid is achieved (Scheme 124).183 Among a set of ligands screened, JohnPhos gives exclusively the single product. Generally, electron-rich aryl bromides produce the higher yield, in contrast electron-deficient aryl bromides shows less effective.
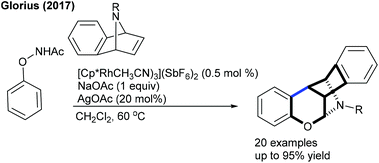 |
| Scheme 122 Synthesis of bridged polycycles via combination of C–H activation and Wagner–Meerwein-type rearrangement. | |
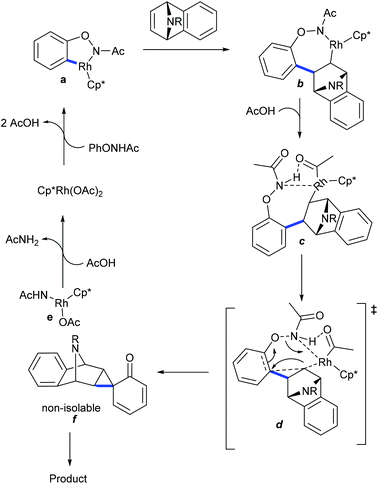 |
| Scheme 123 Mechanism of the combination of C–H activation and Wagner–Meerwein-type rearrangement. | |
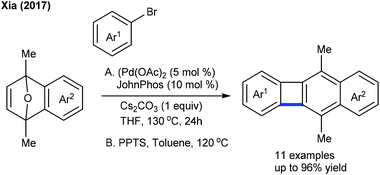 |
| Scheme 124 Synthesis of polycyclic conjugated hydrocarbons. | |
The Radhakrishnan group developed an annulation of enamides with diazabicyclic alkenes (Scheme 125).109 Electron-poor substrates react efficiently. In addition, Tsuji–Trost and benzylic C–H activation of alkyl 2-iodo-3-methylbenzoate works well to produce cyclopentannulated indanes.184 Furthermore, oxidative coupling of salicylaldehyde with bicyclic alkenes is achieved to give fused chromene (Scheme 126).185 Relatively a similar reaction is applied for the construction of isoquinolone-fused heterocycles.186 Diverse urea derived bicyclic alkenes and o-acetyl ketoximes are compatible. This reaction can be extended to benzamides using neutral Rh-catalysis to afford quinolones in good yields.
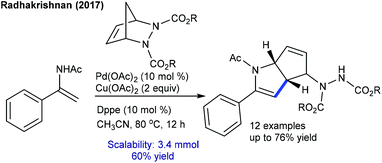 |
| Scheme 125 Oxidative annulation of enamides with diazabicyclic alkenes. | |
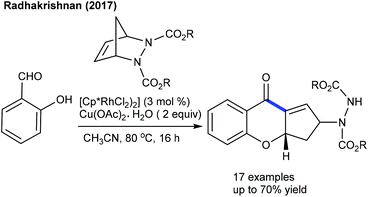 |
| Scheme 126 Oxidative coupling of salicylaldehydes with diazabicyclic alkenes. | |
Perekalin and co-workers showed the assembly of enantiopure dihydroisoquinolines by chiral-planar Rh(III)-catalysis (Scheme 127).187 The introduction of the Boc group is essential for the activation of phenylhydroxamic acid while Ag2CO3 acts as an iodide abstracting agent and CsOAc assists in the abstraction of the ortho-hydrogen atom of phenylhydroxamic acid. The origin of enantioselectivity is explained by a less-hindered side approach of the alkene. Furthermore, the synthesis of phosphorus heterocycles is successful using the [4 + 2]-cycloaddition of phosphinamide with oxabicyclic alkenes (Scheme 128).188 A broad range of heterocyclic phosphinamides and azabicyclic alkenes are tolerated. This method is extended to the coupling of N-methoxybenzamides with heterobicyclic alkenes.189 The N–OMe oxidizing group is essential, and compatible with a series of benzamides and alkenes. The synthesis and application of chiral CpxCo(III)-complexes for C–H activation of N-chlorobenzamides with alkenes including heterocyclic alkenes have been reported (Scheme 129).190
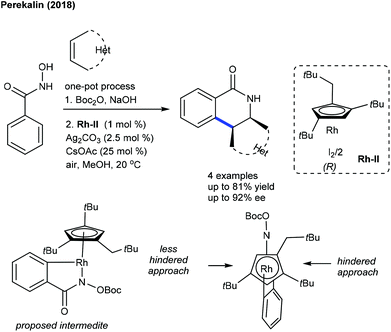 |
| Scheme 127 Synthesis of enantiopure dihydroisoquinolones. | |
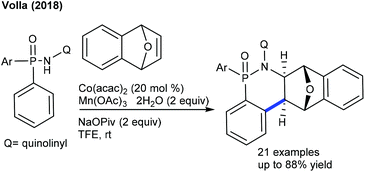 |
| Scheme 128 [4 + 2]-Cycloaddition of phosphinamides with heterobicyclic alkenes. | |
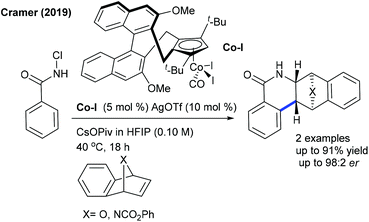 |
| Scheme 129 Annulation of N-chlorobenzamides with heterocyclic alkenes. | |
The Chatani group developed the synthesis of epoxybenzofluorenone through the coupling of benzamide with heterobicyclic alkenes (Scheme 130).191 8-Aminoquinoline chelation assistance is necessary, and the addition of excess AgOAc (6 equiv.) plays a valuable role in the removal of 8-aminoquinoline since it inhibits the catalytic activity of Ni(OTf)2. Mechanistic experiments suggest an involvement of the irreversible C–H bond cleavage (KIE = 2.8 (one-pot) and 1.8 (parallel)) that might be the rate-limiting step. Furthermore, the synthesis of benzo[b]fluorenones is accomplished via a Co-catalyzed [3 + 2]-annulation, ring-opening and dehydration sequence (Scheme 131).192 Diverse products are obtained utilizing O2; however, the requirement of a higher amount of Cs2CO3 (5 equiv.) and elevated temperature (140 °C) limits their potential applications. The irreversible C–H cleavage is confirmed by H/D exchange studies, and the mechanism is related to Cheng's report (Scheme 120) via Cs2CO3-mediated ring-opening and dehydration. In addition, [4 + 2]-annulation of sulfoxonium ylides with oxa/azabicyclic alkenes is achieved.106 Naphthalen-1(2H)-ones are formed in reasonable yields from diverse sulfoxonium ylides. The protocol is not limited to oxabicyclic alkenes alone, but azabicyclic alkenes having electronically varied groups are also coupled. The catalytic cycle involves oxygen directed irreversible C–H metalation (parallel KIE = 3.9).
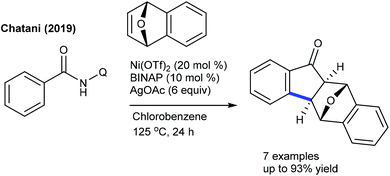 |
| Scheme 130 Reaction of benzamides with oxabenzonorbornadienes. | |
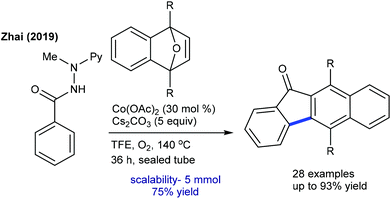 |
| Scheme 131 Synthesis of benzo[b]fluorenones. | |
Our group showed the coupling of aryl-2H-indazoles with 7-azabenzonorbornadienes (Scheme 132).193 This double C–H activation occurs at C-2′ and C-3′ instead of C-2′ and C-3 positions. A broad range of 7-azabenzonorbornadienes and aryl-2H-indazoles were evaluated, and steric and electronic effects were found to significantly affect the reaction. We extended this strategy to the dual C-7 and C-6 C–H functionalization of indolines with broad substrate scope and functional group tolerance.194 The synthetic and mechanistic aspects are explored. Recently, a rare example of three-component [2 + 3 + 1]-annulation of 3-iodochromones, α-bromoacetophenones and norbornenes has been found to be successful (Scheme 133).195 In search of ligands, electron-deficient ligands produced the best results and chromone-fused polycycles showed good diastereoselectivity.
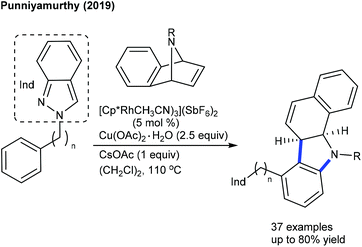 |
| Scheme 132 Coupling of aryl-2H-indazoles with 7-azabenzonorbornadiene. | |
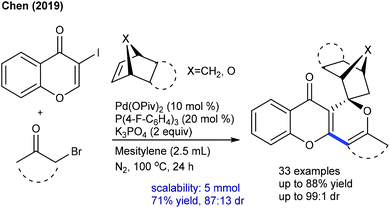 |
| Scheme 133 Synthesis of chromone-fused polycycles. | |
7. Other reactions
7.1. Alkynylation
Limited studies are focused on the alkynylation of heterocyclic alkenes. These reactions utilize a hypervalent iodine-alkyne reagent (TIPS-EBX) as an alkynyl source. The Hong group developed a Ru-catalyzed C-2 selective alkynylation of 4-quinolones to give a small library of alkynylated products (Scheme 134)196 and three examples are focused on the C3-alkynylation of isoquinolones by Rh-catalysis (Scheme 134).196 This strategy is extended to the C-6 alkynylation of pyridones.197 Good yields are observed using a wide array of substrates. Alkynylating reagents such as TBDS-EBX and tBu-EBX are effective, while TMS-EBX and Ph-EBX exhibit inferior results.
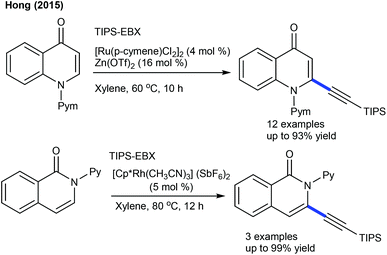 |
| Scheme 134 C3-Alkynylation of isoquinolones employing TIPS-EBX. | |
Au-catalysis was also explored for alkynylation. Li and co-workers described a C5-alkynylation of pyridones using TIPS-EPX as an alkynylating agent (Scheme 135).197 A series of N-alkyl pyridones react affording good yields, while N-aryl pyridines are not effective substrates due to less nucleophilicity. Later, C4-alkynylation of pyrazolones was found to be successful using TIPS-EBX (Scheme 136).198 The pyrazolones having different substituent patterns couple to afford good yields.
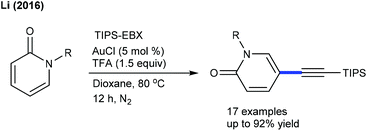 |
| Scheme 135 C5-Alkynylation of pyridones using TIPS-EPX. | |
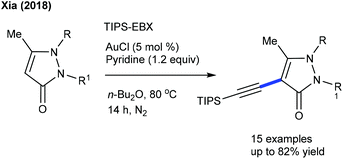 |
| Scheme 136 C4-Alkynylation of pyrazolones. | |
7.2. Allylation
Allylation is explored using strained methyleneoxetanone as the coupling partner. Li and co-workers showed the redox-neutral allylation of arenes (Scheme 137).199 This silver-free protocol is compatible with a wide range of substrates. The procedure is extended to 2-pyridone and isoquinolone. However, pyrimidyl-substituted benzimidazoles and carbazoles fail to give the desired products. Subsequently, Yi and co-workers described the allylation with benzamides (Scheme 138).200 The reaction in trifluoroethanol (TFE) gives the allylated products, whereas in 1,2-dichloroethane (DCE), benzoazepines are formed. This switchable nature of the selectivity is studied using DFT, which advocates energetically favourable β-O elimination in TFE. This methodology is extended to the synthesis of ortho-allylated N-aryl ureas.120 Changing the solvent from TFE (allylated product) to THF produces the annulated product.
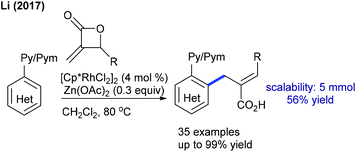 |
| Scheme 137 Redox-neutral coupling of methyleneoxetanones with arenes. | |
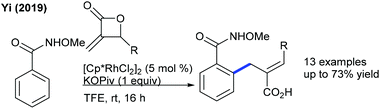 |
| Scheme 138 Allylation of benzamides with methyleneoxetanones. | |
In 2017, the C2-selective 3,3-difluoroallylation of pyridones was devised using 3-bromo-3,3-difluoropropenes by Mn-catalysis (Scheme 139).201 The “fluorine effect” strongly enhances the reaction efficiency. The proposed catalytic cycle involves the formation of manganacycle a through reversible C–H metalation (competitive KIE = 1.03 and parallel KIE = 1.06) (Scheme 140). Coordination with an alkene gives the π-alkenylmanganese complex b. Migratory insertion provides the seven-membered intermediate c, which leads to β-bromo elimination to give the product and regenerate the active catalyst.
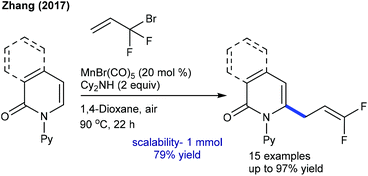 |
| Scheme 139 3,3-Difluoroallylation of pyridones. | |
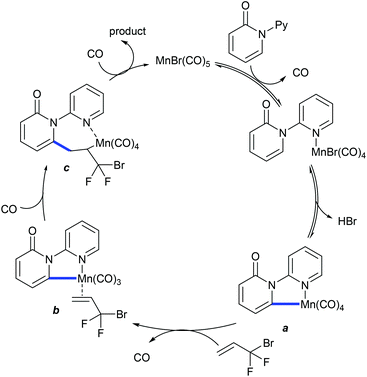 |
| Scheme 140 Catalytic cycle of Mn-catalyzed C2-selective 3,3-difluoroallylation. | |
7.3. Acylation
Few studies are available on acylation of heterocyclic alkenes. The acylation of pyrazolones is found to be successful using α-oxocarboxylic acids (Scheme 141A).202 Electronic and steric factors of the substituted pyrazolones and α-oxocarboxylic acids influence the reaction. For instance, electron-rich substrates act as good coupling partners. The catalytic system is extended to the use of aldehydes as an acylating agent for the synthesis of acyl pyrazolones (Scheme 141B).203 In comparison with Yotphan's process, 32 mol% of the metal salt is sufficient, which is applicable to access a variety of acylated pyrazolones. Nevertheless, alkyl and free NH pyrazolones are unsuccessful substrates.
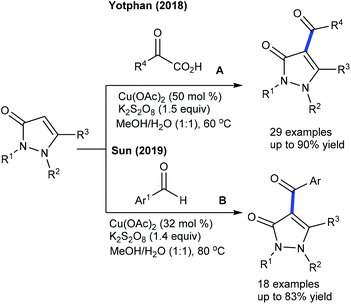 |
| Scheme 141 Synthesis of acyl pyrazolones using aldehydes. | |
7.4. Chalcogenation
Significant studies are focused on C–S and C–Se bond formation. The C6-selective C–S bond formation of pyridones with diaryl/dialkylsulfides is developed (Scheme 142).204 Regardless of the electronic and steric properties, the substrates are readily coupled. Furthermore, a Ru-catalyzed coupling of maleimides with diaryl diselenides and diaryl disulfides is achieved (Scheme 143).205 The reaction tolerates a series of maleimides with variable electronic features. However, the scope of diaryl diselenides is quite limited and elevated temperature is essential for diaryl disulfides that display poor reactivity. The procedure is extended to benzamides, which show moderate reactivity. Mechanistic studies corroborate the non-involvement of Kharasch type addition to the alkene. H/D-Exchange studies exclude β-hydride elimination, and probably involve the direct C–H functionalization.
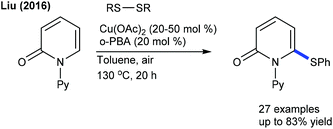 |
| Scheme 142 C6-Thiolation of pyridones. | |
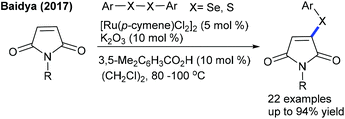 |
| Scheme 143 Functionalization of maleimides using heteroaryl electrophiles. | |
The Ackermann group designed the thiolation/selenylation of pyrazolones (Scheme 144).206 Triflate ions facilitate the base-assisted C–H metalation step. Good yields are obtained by coupling of pyrazolones with disulfides or diselenides; however, the scope of the reaction is somewhat limited. The Cu-catalyzed C–S/C–Se Bond formation of flavones with iodoarenes and KSCN/KSeCN is successful (Scheme 145).207 The coupling proceeds at high temperature (140 °C). Aryl iodides are the better coupling partners. Recently, C–H thiolation and thiocyanation of uracil derivatives have been accomplished.208 The combination of Cu(OTf)2 and K2S2O8 catalyzes the C–S/C–Se coupling. For thiocyanation, a catalyst along with a stoichiometric amount of I2 is required, and apart from NH4SCN and NaSCN, KSCN also shows a viable thiocyanating property. The electronic and steric aspects of disulfide and uracil strongly affect the outcome of the reaction.
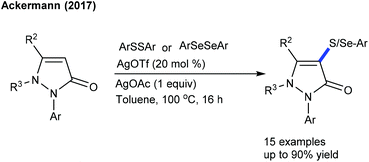 |
| Scheme 144 Thiolation/selenylation of pyrazolones. | |
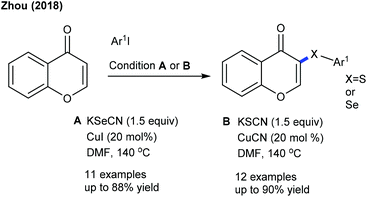 |
| Scheme 145 C-3 C–S/C–Se bond formation of flavones. | |
7.5. Amidation
The Cp*Co(III)-catalyzed C6-amidation of pyridones using oxazolones is accomplished (Scheme 146).209 A variety of pyridones and oxazolones are reacted. Isoquinolones and 4-pyridone prove to be viable substrates. Mechanistic studies suggest an involvement of electrophilic irreversible fast C–H cobaltation (KIE = 0.70 (one-pot) and 0.97 (parallel)). In addition, the amidation of isoquinolones and pyridones with azides is disclosed (Scheme 147).210 Sulfonyl, benzoyl and diphenyl phosphoryl azides are compatible, though the reaction of phenyl azide or benzyl azide is incompetent. Additionally, a diverse array of isoquinolones and pyridines have been proved to be good substrates.
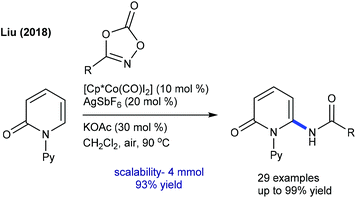 |
| Scheme 146 C6-Amidation of pyridones. | |
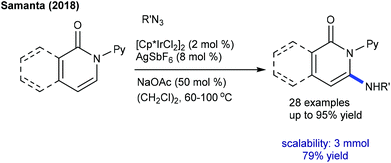 |
| Scheme 147 C3-Amidation of isoquinolones and pyridones with azides. | |
7.6. Miscellaneous reaction
The ethoxylation of cyclic α-aroylketene dithioacetal using Cu(OH)2 is shown in combination with PhI(OAc)2 and benzoquinone as the oxidants (Scheme 148).211 Two examples of C6-alkylation and C5-alkenylation of pyridones with acrylates have been reported (Scheme 149).67 In addition, the C6-acetoxylation of pyridones can be achieved using PIDA.212 However, the protocol has narrow substrate scope.
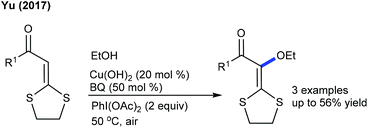 |
| Scheme 148 Ethoxylation of cyclic α-aroylketene dithioacetal. | |
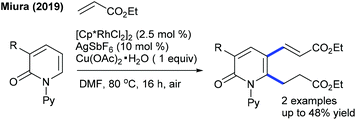 |
| Scheme 149 Alkenylation of pyridones with acrylate. | |
8. Conclusion and outlook
Transition-metal-catalyzed coupling of heterocyclic alkenes via C–H activation has become one of the important synthetic strategies enabling the introduction of an incredibly diverse array of heterocyclic units to produce diverse heterocyclic motifs. Considerable efforts have been made in terms of reactions such as arylation, alkenylation, alkylation, hydroarylation, ring-opening, annulation and cyclization. Noble metals, such as Pd, Rh and Ru, have been mostly used for such transformations. Pd-catalysts are the best for the coupling of pyrones, chromenes, coumarins and related systems with the C–H activation occurring mostly through electrophilic C–H metalation, while Rh-catalysts are efficient at the coupling of other types of heterocyclic alkenes including pyridones/isoquinolones, and the C–H activation takes place via chelation assistance. Metal-catalysts such as Co(III), Cu(II), Mn(I) and Ni(II) have been successfully applied but except Co(III), all others have limited studies on them. These transformations most often require stoichiometric amounts of the oxidants. Thus, utilization of less toxic and environmentally benign air or O2 is exceedingly anticipated and more attention should be paid. Furthermore, the C–H activation followed by multiple bond forming tandem reactions by Rh-catalysis shows the great tendency to assemble complex heterocyclic scaffolds. In addition, the coupling of heterocyclic alkenes via C–H activation of unactivated alkanes remains scarce until recently. Though promising findings have been reported, greater development of this field of research is still required. Furthermore, an enantioselective C–H activation route to coupling of heterocyclic alkenes is a challenging field and is in its primitive stage. Development of efficient catalysts and chiral ligands provides greater space for the synthetic community.
Conflicts of interest
There are no conflicts to declare.
Acknowledgements
We thank Dr. Tariq A. Shah of our group for his kind assistance in editing the manuscript.
Notes and references
-
(a) Y. S. Rao, Chemistry of butenolides, Chem. Rev., 1964, 64, 353 CrossRef CAS;
(b) A. A. Avetisyan and G. G. Tokmadzhyan, Chemistry of Δβ,γ-butenolides, Chem. Heterocycl. Compd., 1987, 23, 595 CrossRef;
(c) K. C. Nicolaou, J. A. Pfefferkorn, A. J. Roecker, G.-Q. Cao, S. Barluenga and H. J. Mitchell, Natural product-like combinatorial libraries based on privileged structures. 1. General principles and solid-phase synthesis of benzopyrans, J. Am. Chem. Soc., 2000, 122, 9939 CrossRef CAS;
(d) M. A. Musa, J. S. Cooperwood and M. O. F. Khan, A review of coumarin derivatives in pharmacotherapy of breast cancer, Curr. Med. Chem., 2008, 15, 2664 CrossRef CAS PubMed;
(e) F. G. Medin, J. G. Marrero, M. Macías-Alonso, M. C. González, I. Córdova-Guerrero, A. G. T. García and S. Osegueda-Robles, Coumarin heterocyclic derivatives: chemical synthesis and biological activity, Nat. Prod. Rep., 2015, 32, 1472 RSC;
(f) A. Nilsen, G. P. Miley, I. P. Forquer, M. W. Mather, K. Katneni, Y. Li, S. Pou, A. M. Pershing, A. M. Stickles, E. Ryan, J. X. Kelly, J. S. Doggett, K. L. White, D. J. Hinrichs, R. W. Winter, S. A. Charman, L. N. Zakharov, I. Bathurst, J. N. Burrows, A. B. Vaidya and M. K. Riscoe, Discovery, synthesis, and optimization of antimalarial 4(1H)-quinolone-3-diarylethers, J. Med. Chem., 2014, 57, 3818 CrossRef CAS PubMed;
(g) R. Çalışkan, O. Sari and M. Balci, Functionalization of oxabenzonorbornadiene: Manganese(III)-mediated oxidative addition of dimedone, J. Phys. Org. Chem., 2017, 30, 3720 CrossRef;
(h) J. M. J. M. Ravasco, H. Faustino, A. Trindade and P. M. P. Gois, Bioconjugation with maleimides: A useful tool for chemical biology, Chem. – Eur. J., 2019, 25, 43 CrossRef CAS PubMed;
(i) D. Cao, Z. Liu, P. Verwilst, S. Koo, P. Jangjili, J. S. Kim and W. Lin, Coumarin-based small-molecule fluorescent chemosensors, Chem. Rev., 2019, 119, 10403 CrossRef CAS PubMed;
(j) A. Ayad, Q. Al-Khdhairawi, G. A. Cordell, N. F. Thomas, N. Babu, S. Nagojappa and J.-F. F. Weber, Natural diterpene pyrones: chemistry and biology, Org. Biomol. Chem., 2019, 17, 8943 RSC.
-
(a) B. D. Krane and M. Shamma, The isoquinolone alkaloids, J. Nat. Prod., 1982, 45(4), 377 CrossRef CAS;
(b) J. L. McCormick, T. C. McKee, J. H. Cardellina II and M. R. Boyd, HIV inhibitory natural products. 26. quinoline alkaloids from Euodia roxburghiana, J. Nat. Prod., 1996, 59, 469 CrossRef CAS PubMed;
(c) P. Ramesh, N. S. Reddy and Y. Venkateswarlu, A new 1,2-dihydroisoquinoline from the sponge Petrosiasimilis, J. Nat. Prod., 1999, 62, 780 CrossRef CAS PubMed;
(d) J. P. Michael, Quinoline, quinazoline and acridone alkaloids, Nat. Prod. Rep., 2000, 17, 603 RSC;
(e) R. Pratap and V. J. Ram, Natural and synthetic chromenes, fused chromenes, and versatility of dihydrobenzo[h]chromenes in organic synthesis, Chem. Rev., 2014, 114, 10476 CrossRef CAS PubMed;
(f) M. T. T. Nguyen, T. H. Le, H. X. Nguyen, P. H. Dang, T. N. V. Do, M. Abe, R. Takagi and N. T. Nguyen, Artocarmins G-M, Prenylated 4-chromenones from the stems of Artocarpus rigida and their tyrosinase inhibitory activities, J. Nat. Prod., 2017, 80, 3172 CrossRef CAS PubMed;
(g) A. Stefanachi, F. Leonetti, L. Pisani, M. Catto and A. Carotti, Coumarin: A natural, privileged and versatile scaffold for bioactive compounds, Molecules, 2018, 23, 250 CrossRef PubMed.
-
(a) L. Li, W. Chen, W. Yang, Y. Pan, H. Liu, C.-H. Tan and Z. Jiang, Bicyclic guanidine-catalyzed asymmetric Michael additions of 3-benzyl-substituted oxindoles to N-maleimides, Chem. Commun., 2012, 48, 5124 RSC;
(b) Q. Zhao, X. Han, Y. Wei, M. Shi and Y. Lu, Asymmetric [3 + 2]-annulation of allenes with maleimides catalyzed by dipeptide-derived phosphines: facile creation of functionalized bicyclic cyclopentenes containing two tertiary stereogenic centers, Chem. Commun., 2012, 48, 970 RSC;
(c) L. H. Lima and J. (Steve) Zhou, A challenging Heck reaction of maleimides, Org. Chem. Front., 2015, 2, 775 RSC;
(d) S. M. Tan, A. C. Willis, M. N. Paddon-Row and M. S. Sherburn, Multicomponent diene-transmissive Diels–Alder sequences featuring aminodendralenes, Angew. Chem., Int. Ed., 2016, 55, 3081 CrossRef CAS PubMed;
(e) J. Li, S. Qiu, X. Ye, B. Zhu, H. Liu and Z. Jiang, Dipeptide-based chiral tertiary amine-catalyzed asymmetric conjugate addition reactions of 5H-thiazol/oxazol-4-ones, J. Org. Chem., 2016, 81, 11916 CrossRef CAS PubMed;
(f) J. Zhou, L. Bai, G. Liang, Y. Chen, Z. Gan, W. Wang, H. Zhou and Y. Yu, Organocatalytic asymmetric domino Michael/O-alkylation reaction for the construction of succinimide substituted 3(2H)-furanones catalyzed by quinine, RSC Adv., 2017, 7, 39885 RSC;
(g) E. Selva, L. M. Castelló, J. Mancebo-Aracil, V. Selva, C. Nájera, F. Foubelo and J. M. Sansano, Synthesis of pharmacophores containing a prolinate core using a multicomponent 1,3-dipolar cycloaddition of azomethine ylides, Tetrahedron, 2017, 73, 6840 CrossRef CAS.
-
(a) C. A. Miller and L. M. Long, Anticonvulsants. I. an investigation of N-R-α-R1-α-phenylsuccinimides, J. Am. Chem. Soc., 1951, 73, 4895 CrossRef CAS;
(b) A. M. Crider, T. M. Kolczynski and K. M. Yates, Synthesis and anticancer activity of nitrosourea derivatives of phensuximide, J. Med. Chem., 1980, 23, 324 CrossRef CAS PubMed;
(c) G. O. Rankin, K. Cressey-Veneziano, R.-T. Wang and P. I. Brown, Urinary tract effects of phensuximide in the Sprage-Dawley and Fischer 344 rat, J. Appl. Toxicol., 1986, 6, 349 CrossRef CAS PubMed;
(d) M. N. Deshpande, M. H. Cain, S. R. Patel, P. R. Singam, D. Brown, A. Gupta, J. Barkalow, G. Callen, K. Patel, R. Koops, M. Chorghade, H. Foote and R. Pariza, A scalable process for the novel antidepressant ABT-200, Org. Process Res. Dev., 1998, 2, 351 CrossRef CAS;
(e) F. A. Luzzio, D. Y. Duveau, E. R. Lepper and W. D. Figg, Synthesis of racemic cis-5-hydroxy-3-phthalimidoglutarimide. A metabolite of thalidomide isolated from human plasma, J. Org. Chem., 2005, 70, 10117 CrossRef CAS PubMed;
(f) A. Shoji, M. Kuwahara, H. Ozaki and H. Sawai, Modified DNA aptamer that binds the (R)-isomer of a thalidomide derivative with high enantioselectivity, J. Am. Chem. Soc., 2007, 129, 1456 CrossRef CAS PubMed;
(g) T. Ishiyama, K. Tokuda, T. Ishibashi, A. Ito, S. Toma and Y. Ohno, Lurasidone (SM-13496), a novel atypical antipsychotic drug, reverses MK-801-induced impairment of learning and memory in the rat passive-avoidance test, Eur. J. Pharmacol., 2007, 572, 160 CrossRef CAS PubMed;
(h) E. D. Deeks, Apremilast: A Review in psoriasis and psoriatic arthritis, Drugs, 2015, 75, 1393 CrossRef CAS PubMed;
(i) C. Bharkavi, S. V. Kumar, M. A. Ali, H. Osman, S. Muthusubramanian and S. Perumal, One-pot microwave assisted stereoselective synthesis of novel dihydro-2′H-spiro[indene-2,1′-pyrrolo-[3,4-c]pyrrole]-tetraones and evaluation of their antimycobacterial activity and inhibition of AChE, Bioorg. Med. Chem. Lett., 2017, 27, 3071 CrossRef CAS PubMed.
-
(a) C. Herdeis and C. Hartke, [4 + 2]-Cycloadducts of 5-benzyloxy-2-pyridone
with electron deficient dienophiles. Regio- and stereochemistry, Heterocycles, 1989, 29, 287 CrossRef CAS;
(b) R. P. Polniaszek and L. W. Dillard, Stereospecific total syntheses of decahydroquinoline alkaloids (+)-195A and (+)-2-epi-195A, J. Org. Chem., 1992, 57, 4103 CrossRef CAS;
(c) P. Chiu and M. Lautens, Using ring-opening reactions of oxabicyclic compounds as a strategy in organic synthesis, Top. Curr. Chem., 1997, 190, 1 CrossRef CAS;
(d) J. L. AceÇa, O. Arjona, M. L. Lejn and J. Plumet, Total synthesis of (+)-7-Deoxypancratistatin from furan, Org. Lett., 2000, 2, 3683 CrossRef PubMed;
(e) S. Madan and C.-H. Cheng, Nickel-catalyzed synthesis of benzocoumarins: Application to the total synthesis of arnottin I, J. Org. Chem., 2006, 71, 8312 CrossRef CAS PubMed;
(f) M. Odagi, K. Furukori, K. Takayama, K. Noguchi and K. Nagasawa, Total synthesis of rishirilide B by organocatalytic oxidative kinetic resolution: revision of absolute configuration of (+)-rishirilide B, Angew. Chem., Int. Ed., 2017, 56, 6609 CrossRef CAS PubMed.
-
(a) M. Lautens, K. Fagnou and S. Hiebert, Transition-metal-catalyzed enantioselective ring-opening reactions of oxabicyclic alkenes, Acc. Chem. Res., 2003, 36, 48 CrossRef CAS PubMed;
(b) D. K. Rayabarapu and C.-H. Cheng, New catalytic reactions of oxa- and azabicyclic alkenes, Acc. Chem. Res., 2007, 40, 971 CrossRef CAS PubMed;
(c) A. Menzek, L. Kelebekli, A. Altundas, E. Şahina and F. Polat, Cycloaddition reaction of 1,4-dihydronaphthalene 1,4-epoxide with cyclooctatetraene: Cope rearrangement in an adduct, Helv. Chim. Acta, 2008, 91, 2367 CrossRef CAS;
(d) M. Ballantine, M. L. Menard and W. Tam, Isomerization of 7-oxabenzonorbornadienes into naphthols catalyzed by [RuCl2(CO)3]2, J. Org. Chem., 2009, 74, 7570 CrossRef CAS PubMed;
(e) J. Zhu, G. C. Tsui and M. Lautens, Rhodium-catalyzed enantioselective nucleophilic fluorination: ring opening of oxabicyclic alkenes, Angew. Chem., Int. Ed., 2012, 51, 12353 CrossRef CAS PubMed;
(f) S. Li, Z. Lu, L. Meng and J. Wang, Iridium-catalyzed asymmetric addition of thiophenols to oxabenzonorbornadienes, Org. Lett., 2016, 18, 5276 CrossRef CAS PubMed;
(g) R. Boutin, S. Koh and W. Tam, Recent advances in transition-metal-catalyzed reactions of oxabenzonorborna-diene, Curr. Org. Synth., 2019, 16, 460 CrossRef CAS PubMed.
-
(a) N. Miyaura and A. Suzuki, Palladium-catalyzed cross-coupling reactions of organoboron compounds, Chem. Rev., 1995, 95, 2457 CrossRef CAS;
(b)
F. Diederich and P. J. Stang, Metal-Catalyzed Cross-Coupling Reactions, Wiley-VCH, Weinheim, Germany, 1998 CrossRef;
(c) I. P. Beletskaya and A. V. Cheprakov, The Heck reaction as a sharpening stone of palladium catalysis, Chem. Rev., 2000, 100, 3009 CrossRef CAS PubMed;
(d)
N. Miyaura, Cross-Coupling Reactions: A Practical Guide, Springer, Berlin, 2002 CrossRef;
(e) A. R. Muci and S. L. Buchwald, Practical palladium catalysts for C-N and C-O bond formation, Top. Curr. Chem., 2002, 219, 131 CrossRef CAS;
(f) J.-P. Corbet and G. Mignani, Selected patented cross-coupling reaction technologies, Chem. Rev., 2006, 106, 2651 CrossRef CAS PubMed;
(g)
M. Oestreich, The Mizoroki-Heck Reaction, John Wiley & Sons, 2009 CrossRef;
(h) R. Jana, T. P. Pathak and M. S. Sigman, Advances in transition metal (Pd, Ni, Fe)-catalyzed cross-coupling reactions using alkyl-organometallics as reaction partners, Chem. Rev., 2011, 111, 1417 CrossRef CAS PubMed.
-
(a) D. Alberico, M. E. Scott and M. Lautens, Aryl–aryl bond formation by transition-metal-catalyzed direct arylation, Chem. Rev., 2007, 107, 174 CrossRef CAS PubMed;
(b)
L. Ackermann, Modern Arylation Methods, Wiley-VCH, 2009 CrossRef;
(c) L. Ackermann, R. Vicente and A. R. Kapdi, Transition-metal-catalyzed direct arylation of (hetero)arenes by C-H bond cleavage, Angew. Chem., Int. Ed., 2009, 48, 9792 CrossRef CAS PubMed;
(d) D. Zhao, J. You and C. Hu, Recent progress in coupling of two heteroarenes, Chem. – Eur. J., 2011, 17, 5466 CrossRef CAS PubMed;
(e) J. Yamaguchi, A. D. Yamaguchi and K. Itami, C-H bond functionalization: emerging synthetic tools for natural products and pharmaceuticals, Angew. Chem., Int. Ed., 2012, 51, 8960 CrossRef CAS PubMed;
(f) R. Rossi, F. Bellina, M. Lessi and C. Manzini, Cross-coupling of heteroarenes by c-h functionalization: recent progress towards direct arylation and heteroarylation reactions involving heteroarenes containing one heteroatom, Adv. Synth. Catal., 2014, 356, 17 CrossRef CAS.
-
(a) G. Qiu and J. Wu, Transition metal-catalyzed direct remote C-H functionalization of alkyl groups via C(sp3)–H bond activation, Org. Chem. Front., 2015, 2, 169 RSC;
(b) W.-H. Rao and B.-F. Shi, Recent advances in copper-mediated chelation-assisted functionalization of unactivated C-H bonds, Org. Chem. Front., 2016, 3, 1028 RSC;
(c) W. Ma, P. Gandeepan, J. Lid and L. Ackermann, Recent advances in positional-selective alkenylations: removable guidance for twofold C-H activation, Org. Chem. Front., 2017, 4, 1435–1467 RSC;
(d) Q.-L. Yang, P. Fang and T.-S. Mei, Recent advances in organic electrochemical C-H functionalization, Chin. J. Chem., 2018, 36, 338 CrossRef CAS;
(e) Y. Liu, H. Yi and A. Lei, Oxidation-induced C-H functionalization: a formal way for C-H activation, Chin. J. Chem., 2018, 36, 692 CrossRef CAS;
(f) P. J. Borpatra, B. Deka, M. L. Deb and P. K. Baruah, Recent advances in intramolecular C-O/C-N/C-S bond formation via C-H functionalization, Org. Chem. Front., 2019, 6, 3445 RSC;
(g) R. Wang, Y. Luan and M. Ye, Transition metal-catalyzed allylic C(sp3)-H functionalization via η3-allylmetal intermediate, Chin. J. Chem., 2019, 37, 720 CrossRef CAS;
(h) Q. Zhang and B.-F. Shi, From reactivity and regioselectivity to stereoselectivity: an odyssey of designing PIP amine and related directing groups for C-H activation, Chin. J. Chem., 2019, 37, 720 CrossRef;
(i) Y.-H. Liu, Y.-N. Xia and B.-F. Shi, Ni-Catalyzed chelation-assisted direct functionalization of inert C-H bonds, Chin. J. Chem., 2020 DOI:10.1002/cjoc.201900468.
-
(a) V. Ritleng, C. Sirlin and M. Pfeffer, Ru-Rh-, and Pd-catalyzed C-C bond formation involving C–H activation and addition on unsaturated substrates: reactions and mechanistic aspects, Chem. Rev., 2002, 102, 1731 CrossRef CAS PubMed;
(b) G. Song, F. Wang and X. Li, C–C, C–O and C–N bond formation via rhodium(III)-catalyzed oxidative C–H activation, Chem. Soc. Rev., 2012, 41, 3651 RSC;
(c) Z. Chen, B. Wang, J. Zhang, W. Yu, Z. Liu and Y. Zhang, Transition metal-catalyzed C–H bond functionalizations by the use of diverse directing groups, Org. Chem. Front., 2015, 2, 1107 RSC;
(d) T. Gensch, M. N. Hopkinson, F. Glorius and J. Wencel-Delord, Mild metal-catalyzed C–H activation: examples and concepts, Chem. Soc. Rev., 2016, 45, 2900 RSC;
(e) Y. Park, Y. Kim and S. Chang, Transition metal-catalyzed C-H amination: scope, mechanism, and applications, Chem. Rev., 2017, 117, 9247 CrossRef CAS PubMed;
(f) Z. Dong, Z. Ren, S. J. Thompson, Y. Xu and G. Dong, Transition-metal-catalyzed C-H alkylation using alkenes, Chem. Rev., 2017, 117, 9333 CrossRef CAS PubMed;
(g) T. G. Saint-Denis, R.-Y. Zhu, G. Chen, Q.-F. Wu and J.-Q. Yu, Enantioselective C(sp3)-H bond activation by chiral transition metal catalysts, Science, 2018, 359, 759 CrossRef CAS PubMed;
(h) P. Gandeepan, T. Müller, D. Zell, G. Cera, S. Warratz and L. Ackermann, 3d Transition metals for C-H activation, Chem. Rev., 2019, 119, 2192 CrossRef CAS PubMed;
(i) S. Rej and N. Chatani, Rhodium-catalyzed C(sp2)- or C(sp3)–H bond functionalization assisted by removable
directing groups, Angew. Chem., Int. Ed., 2019, 58, 8304 CrossRef CAS PubMed.
- K. Hirano and M. Miura, A lesson for site-selective C–H functionalization on 2-pyridones: radical, organometallic, directing group and steric controls, Chem. Sci., 2018, 9, 22 RSC.
-
(a) A. M. Prendergast and G. P. McGlacken, Transition metal mediated C-H activation of 2-pyrones, 2-pyridones, 2-coumarins and 2-quinolones, Eur. J. Org. Chem., 2018, 6068 CrossRef CAS;
(b) D. Kang, K. Ahn and S. Hong, Site-selective C–H bond functionalization of chromones and coumarins, Asian J. Org. Chem., 2018, 7, 1136 CrossRef CAS.
- R. Manoharan and M. Jeganmohan, Alkylation, annulation, and alkenylation of organic molecules with maleimides by transition-metal-catalyzed C-H bond activation, Asian J. Org. Chem., 2019, 8, 1949 CrossRef CAS.
- M. Eisold, A. Müller-Deku, F. Reiners and D. Didier, Parallel approaches for the functionalization of thietes: α-metalation versus C–H activation, Org. Lett., 2018, 20, 4654 CrossRef CAS PubMed.
- M. Robichon, A. Bordessa, M. Malinowski, J. Uziel, N. Lubin-Germaina and A. Ferry, Access to C-aryl/alkenylglycosides by directed Pd-catalyzed C–H functionalisation of the anomeric position in glycal-type substrates, Chem. Commun., 2019, 55, 11806 RSC.
- T. K. Beng, S. Langevin, H. Braunstein and M. Khim, Regiocontrolled synthesis of (hetero)aryl and alkenyl dehydropyrrolidines, dehydropiperidines and azepenes by Ru-catalyzed, heteroatom-directed α-C–H activation/cross-coupling of cyclic enamides with boronic acids, Org. Biomol. Chem., 2016, 14, 830 RSC.
- D. P. Canterbury, K. D. Hesp and J. Polivkova, Palladium-catalyzed β-(hetero)arylation of α, β-unsaturated valerolactams, Org. Biomol. Chem., 2016, 14, 7731 RSC.
- P. Peng, J. Wang, H. Jiang and H. Liu, Rhodium(III)-catalyzed site-selective C–H alkylation and arylation of pyridones using organoboron reagents, Org. Lett., 2016, 18, 5376 CrossRef CAS PubMed.
- D. Das, P. Poddar, S. Maity and R. Samanta, Rhodium(III)-catalyzed C6-selective arylation of 2-pyridones and related heterocycles using quinone diazides: Syntheses of heteroarylated phenols, J. Org. Chem., 2017, 82, 3612 CrossRef CAS PubMed.
- E. Grenet, A. Das, P. Caramenti and J. Waser, Rhodium-catalyzed C-H functionalization of heteroarenes using indoleBX hypervalent iodine reagents, Beilstein J. Org. Chem., 2018, 14, 1208 CrossRef CAS PubMed.
- S. Maity, D. Das, S. Sarkar and R. Samanta, Direct Pd(II)-catalyzed site-selective C5-arylation of 2-pyridone using aryl iodides, Org. Lett., 2018, 20, 5167 CrossRef CAS PubMed.
- G. G. Pawar, V. K. Tiwari, H. S. Jena and M. Kapur, Heteroatom-guided, palladium-catalyzed, site-selective C-H arylation of 4H-chromenes: Diastereoselective assembly of the core structure of myristinin B through dual C-H functionalization, Chem. – Eur. J., 2015, 21, 9905 CrossRef CAS PubMed.
- M. Khoobi, F. Molaverdi, F. Jafarpour, M. Abbasnia, M. Kubicki and A. Shafiee, A one-pot domino C-H, C-C activation in coumarins: a fast track to 2,3-diaryl benzo[b]furans, Chem. Commun., 2015, 51, 11713 RSC.
- S. Kwon, D. Kang and S. Hong, Rh(I)-catalyzed site-selective decarbonylative alkenylation and arylation of quinolones under chelation assistance, Eur. J. Org. Chem., 2015, 3671 CrossRef CAS.
- S. Lee, S. Mah and S. Hong, Catalyst controlled divergent C4/C8 site-selective C-H arylation of isoquinolones, Org. Lett., 2015, 17, 3864 CrossRef CAS PubMed.
- H. Choi, M. Min, Q. Peng, D. Kang, R. S. Paton and S. Hong, Unraveling innate substrate control in site-selective palladium-catalyzed C-H heterocycle functionalization, Chem. Sci., 2016, 7, 3900 RSC.
- K. A. Kumar, P. Kannabonia and P. Das, Ruthenium-catalyzed site-selective C-H arylation of 2-pyridones and 1-isoquinolinones, Org. Biomol. Chem., 2017, 15, 5457 RSC.
- E. Kianmehr, M. Torabi, M. R. Khalkhali, N. Faghih and K. M. Khan, Palladium-catalyzed regioselective cross-dehydrogenative coupling of benzofurans with uracils at room temperature, Eur. J. Org. Chem., 2015, 2796 CrossRef CAS.
- B. Savitha, E. K. Reddy, C. S. A. Kumar, R. P. Karuvalam, M. S. A. Padusha, V. A. Bakulev, K. H. Narasimhamurthy, A. M. Sajith and M. N. Joy, A modified approach for the site-selective direct C-6 arylation of benzylated uracil, Tetrahedron Lett., 2019, 60, 151332 CrossRef CAS.
- S. K. Guchhait and G. Priyadarshani, Pd-Catalyzed Ag(I)-promoted C3-arylation of pyrido[1,2-a]pyrimidin-4-ones with bromo/iodo-arenes, J. Org. Chem., 2015, 80, 8482 CrossRef CAS PubMed.
- Y. Minami, T. Kodama and T. Hiyama, Dehydrogenative carbon–carbon bond formation using alkynyloxy moieties as hydrogen-accepting directing groups, Angew. Chem., Int. Ed., 2015, 54, 11813 CrossRef CAS PubMed.
- W. Lv, S. Wen, J. Yu and G. Cheng, Palladium-catalyzed ortho-silylation of aryl iodides with concomitant arylsilylation of oxanorbornadiene: Accessing functionalized (Z)-β-substituted vinylsilanes and their analogues, Org. Lett., 2018, 20, 4984 CrossRef CAS PubMed.
- W. Lv, J. Yu, B. Ge, S. Wen and G. Cheng, Palladium-catalyzed catellani-type bis-silylation and bis-germanylation of aryl iodides and norbornenes, J. Org. Chem., 2018, 83, 12683 CrossRef CAS PubMed.
- Y. Unoh, T. Satoh, K. Hirano and M. Miura, Rhodium(III)-catalyzed direct coupling of arylphosphine derivatives with heterobicyclic alkenes: a concise route to biarylphosphines and dibenzophosphole derivatives, ACS Catal., 2015, 5, 6634 CrossRef CAS.
- L. Kong, S. Yu, G. Tang, H. Wang, X. Zhou and X. Li, Cobalt(III)-catalyzed C–C coupling of arenes with 7-oxabenzonorbornadiene and 2-vinyloxirane via C–H activation, Org. Lett., 2016, 18, 3802 CrossRef CAS PubMed.
- K. Muralirajan, S. Prakash and C.-H. Cheng, Cobalt-catalyzed mild ring-opening addition of arenes C–H bond to 7-oxabicyclic alkenes, Adv. Synth. Catal., 2017, 359, 513 CrossRef CAS.
- Q. Yan, C. Xiong, S. Chu, Z. Liu and Y. Zhang, Ruthenium-catalyzed ring-opening addition of anilides to 7-azabenzonorbornadienes: a diastereoselective route to hydronaphthylamines, J. Org. Chem., 2018, 83, 5598 CrossRef CAS PubMed.
- G. Liao, H.-M. Chen, Y.-N. Xia, B. Li, Q.-J. Yao and B.-F. Shi, Synthesis of chiral aldehyde catalysts by Pd-catalyzed atroposelective C–H naphthylation, Angew. Chem., Int. Ed., 2019, 58, 11464 CrossRef CAS PubMed.
- Y. Moon, Y. Jeong, D. Kook and S. Hong, Rh(III)-catalyzed direct C–H/C–H cross-coupling of quinones with arenes assisted by a directing group: identification of carbazole quinones as GSKβ inhibitors, Org. Biomol. Chem., 2015, 13, 3918 RSC.
- K. R. Bettadapur, S. M. Sherikar, V. Lanke and K. R. Prabhu, Rh(III)-catalyzed C–H activation: Mizoroki–Heck-type reaction of maleimides, Asian J. Org. Chem., 2018, 7, 1338 CrossRef CAS.
- M. S. Sherikar, R. Kapanaiah and K. R. Prabhu, Rhodium(III)-catalyzed C–H activation at the C4-position of indole: switchable hydroarylation and oxidative Heck-type reactions of maleimides, Chem. Commun., 2018, 54, 11200 RSC.
- S. Jambu, R. Sivasakthikumaran and M. Jeganmohan, Aerobic oxidative alkenylation of weak O-coordinating arylacetamides with alkenes via a Rh(III)-catalyzed C–H activation, Org. Lett., 2019, 21, 1320 CrossRef CAS PubMed.
- M. Tamizmani, N. Gouranga and M. Jeganmohan, Rhodium(III)-catalyzed ortho-alkenylation of anilides with maleimides, ChemistrySelect, 2019, 4, 2976 CrossRef CAS.
- M. S. Sherikar and K. R. Prabhu, Weak coordinating carboxylate directed rhodium(III)-catalyzed C–H activation: switchable decarboxylative Heck-type and [4 + 1] annulation reactions with maleimides, Org. Lett., 2019, 21, 4525 CrossRef CAS PubMed.
- P. A. Yakkala, D. Giri, B. Chaudhary, P. Auti and S. Sharma, Regioselective C-H alkylation and alkenylation at the C5 position of 2-amino-1,4-naphthoquinones with maleimides under Rh(III) catalysis, Org. Chem. Front., 2019, 6, 2441 RSC.
- S. Sharma, S. H. Han, Y. Oh, N. K. Mishra, S. H. Lee, J. S. Oh and I. S. Kim, Cross-coupling of acrylamides and maleimides under rhodium catalysis: controlled olefin migration, Org. Lett., 2016, 18, 2568 CrossRef CAS PubMed.
- S. K. Banjare, T. Nanda and P. C. Ravikumar, Stereoselective hydroacylation of bicyclic alkenes with 2-hydroxybenzaldehydes catalyzed by hydroxoiridium/diene complexes, Org. Lett., 2019, 21, 8138 CrossRef CAS PubMed.
- S. Chen, X. Chang, Y. Tao, H. Chen and Y. Xia, Palladium(II)-catalyzed direct alkenylation of dihydropyranones, Org. Biomol. Chem., 2015, 13, 10675 RSC.
- H. U. Lah, F. Rasoola and S. K. Yousuf, Palladium catalyzed C(sp2)–C(sp2) bond formation. A highly regio- and chemoselective oxidative Heck C-3 alkenylation of pyrones and pyridones, RSC Adv., 2015, 5, 78958 RSC.
- M. Tian, B. Liu, J. Sun and X. Li, Rh(III)-catalyzed C–C coupling of diverse arenes and 4-acyl-1-sulfonyltriazoles via C–H activation, Org. Lett., 2018, 20, 4946 CrossRef CAS PubMed.
- H. Zhao, X. Xu, Z. Luo, L. Cao, B. Li, H. Li, L. Xu, Q. Fan and P. J. Walsh, Rhodium(I)-catalyzed C6-selective C-H alkenylation and polyenylation of 2-pyridones with alkenyl and conjugated polyenyl carboxylic acids, Chem. Sci., 2019, 10, 10089 RSC.
- S. Hazra, K. Hirano and M. Miura, Solvent-controlled rhodium-catalyzed C6-selective C-H alkenylation and alkylation of 2-pyridones with acrylates, Asian J. Org. Chem., 2019, 8, 1097 CrossRef CAS.
- Y.-Q. Zhu, Y.-X. Niu, L.-W. Hui, J.-L. He and K. Zhu, Reaction of isoquinolin-1(2H)-ones with methylenecyclopropanes via rhodium(III)-catalyzed C-H activation, Adv. Synth. Catal., 2019, 361, 2897 CrossRef CAS.
- X. Wang, S. Li, Y. Pan, H. Wang, Z. Chen and K. Huang, Regioselective palladium-catalyzed decarboxylative cross-coupling reaction of alkenyl acids with coumarins: synthesis of 3-styrylcoumarin compounds, J. Org. Chem., 2015, 80, 2407 CrossRef CAS PubMed.
- N. Kim, M. Min and S. Hong, Regioselective palladium(II)-catalyzed aerobic oxidative Heck-type C3 alkenylation of sulfocoumarins, Org. Chem. Front., 2015, 2, 1621 RSC.
- R. Das, N. P. Khot, A. S. Deshpande and M. Kapur, Ruthenium-catalyzed directed C(3)–H olefination of N-acetyl-1,2-dihydroisoquinolines: a method to achieve C3-olefinated isoquinolines, Synthesis, 2019, 51, 2515 CrossRef CAS.
- X. Zhang, L. Su, L. Qiu, Z. Fan, X. Zhang, S. Lin and Q. Huang, Palladium-catalyzed C–H olefination of uracils and caffeines using molecular oxygen as the sole oxidant, Org. Biomol. Chem., 2017, 15, 3499 RSC.
- T. J. Potter, D. N. Kamber, B. Q. Mercado and J. A. Ellman, Rh(III)-catalyzed aryl and alkenyl C–H bond addition to diverse nitroalkenes, ACS Catal., 2017, 7, 150 CrossRef CAS PubMed.
- M. Wang, L. Kong, Q. Wu and X. Li, Ruthenium- and Rhodium-catalyzed chemodivergent couplings of ketene dithioacetals and α-diazo ketones via C–H activation/functionalization, Org. Lett., 2018, 20, 4597 CrossRef CAS PubMed.
- T. Piou and T. Rovis, Rhodium-catalysed syn-carboamination of alkenes via a transient directing group, Nature, 2015, 527, 86 CrossRef CAS PubMed.
- S. Han, J. Park, S. Kim, S. H. Lee, S. Sharma, N. K. Mishra, Y. H. Jung and I. S. Kim, Rhodium(III)-catalyzed C(sp3)–H alkylation of 8-methylquinolines with maleimides, Org. Lett., 2016, 18, 4666 CrossRef CAS PubMed.
- X.-X. Chen, J.-T. Ren, J.-L. Xu, H. Xie, W. Sun, Y.-M. Li and M. Sun, Cobalt(III)-catalyzed 1,4-addition of C(sp3)-H bonds to maleimides, Synlett, 2018, 1601 CAS.
- R. Kumar, R. Kumar, D. Chandra and U. Sharma, Cp*CoIII-catalyzed alkylation of primary and secondary C(sp3)-H bonds of 8-alkylquinolines with maleimides, J. Org. Chem., 2019, 84, 1542 CrossRef CAS PubMed.
- W. Yu, W. Zhang, Y. Liu, Z. Liu and Y. Zhang, Cobalt(III)-catalyzed cross-coupling of enamides with allyl acetates/maleimides, Org. Chem. Front., 2017, 4, 77 RSC.
- B.-B. Zhan, Y. Li, J.-W. Xu, X.-L. Nie, J. Fan, L. Jin and B.-F. Shi, Site-selective δ-C(sp3)-H alkylation of amino acids and peptides with maleimides via a six-membered palladacycle, Angew. Chem., Int. Ed., 2018, 57, 5858 CrossRef CAS PubMed.
- Q. He, Y. Ano and N. Chatani, The Pd-catalyzed C-H alkylation of ortho-methyl-substituted aromatic amides with maleimide occurs preferentially at the ortho-methyl C–H bond over the ortho-C-H bond, Chem. Commun., 2019, 55, 9983 RSC.
- Y. Hara, S. Onodera, T. Kochi and F. Kakiuchi, Catalytic formation of α-aryl ketones by C-H functionalization with cyclic alkenyl carbonates and one-pot synthesis of isocoumarins, Org. Lett., 2015, 17, 4850 CrossRef CAS PubMed.
- D. Das, A. Biswas, U. Karmakar, S. Chand and R. Samanta, C6-selective direct alkylation of pyridones with diazo compounds under Rh(III)-catalyzed mild conditions, J. Org. Chem., 2016, 81, 842 CrossRef CAS PubMed.
- W. Miura, K. Hirano and M. Miura, Nickel-catalyzed directed C6-selective C-H alkylation of 2-pyridones with dienes and activated alkenes, J. Org. Chem., 2017, 82, 5337 CrossRef CAS PubMed.
- Y. Ebe and T. Nishimura, Iridium-catalyzed branch-selective hydroarylation of vinyl ethers via C-H bond activation, J. Am. Chem. Soc., 2015, 137, 5899 CrossRef CAS PubMed.
- K. Shibata and N. Chatani, Rhodium-catalyzed regioselective addition of the ortho C-H bond in aromatic amides to the C–C double bond in α,β-unsaturated γ-lactones and dihydrofurans, Chem. Sci., 2016, 7, 240 RSC.
- Y. Ebe, M. Onoda, T. Nishimura and H. Yorimitsu, Iridium-catalyzed regio- and enantioselective hydroarylation of alkenyl ethers by olefin isomerization, Angew. Chem., Int. Ed., 2017, 56, 5607 CrossRef CAS PubMed.
- K. Sakamoto and T. Nishimura, Iridium-catalyzed asymmetric hydroarylation of chromene derivatives with aromatic ketones: enantioselective synthesis of 2-arylchromanes, Adv. Synth. Catal., 2019, 361, 2124 CrossRef CAS.
- K. Sakamoto, M. Nagai, Y. Ebe, H. Yorimitsu and T. Nishimura, Iridium-catalyzed direct hydroarylation of glycals via C-H activation: ligand-controlled stereoselective synthesis of α- and β-C-glycosyl arenes, ACS Catal., 2019, 9, 1347 CrossRef CAS.
- K. R. Bettadapur, V. Lanke and K. R. Prabhu, Ru(II)-catalyzed C–H activation: ketone-directed novel 1,4-addition of ortho C–H Bond to maleimides, Org. Lett., 2015, 17, 4658 CrossRef PubMed.
- V. Lanke, K. R. Bettadapur and K. R. Prabhu, Site-selective addition of maleimide to indole at the C-2 position: Ru(II)-catalyzed C-H activation, Org. Lett., 2015, 17, 4662 CrossRef PubMed.
- P. Keshri, K. R. Bettadapur, V. Lanke and K. R. Prabhu, Ru(II)-catalyzed C–H activation: amide-directed 1,4-addition of the ortho C–H bond to maleimides, J. Org. Chem., 2016, 81, 6056 CrossRef CAS PubMed.
- S. Sharma, S. H. Han, H. Jo, S. Han, N. K. Mishra, M. Choi, T. Jeong, J. Park and I. S. Kim, Rhodium-catalyzed vinylic C-H functionalization of enol carbamates with maleimides, Eur. J. Org. Chem., 2016, 3611 CrossRef CAS.
- S. H. Han, S. Kim, U. De, N. K. Mishra, J. Park, S. Sharma, J. H. Kwak, S. Han, H. S. Kim and I. S. Kim, Synthesis of succinimide-containing chromones, naphthoquinones, and xanthones under Rh(III) catalysis: evaluation of anticancer activity, J. Org. Chem., 2016, 81, 12416 CrossRef CAS PubMed.
- Z. Zhang, S. Han, M. Tang, L. Ackermann and J. Li, C-H Alkylations of (hetero)arenes by maleimides and maleate esters
through Cobalt(III) catalysis, Org. Lett., 2017, 19, 3315 CrossRef CAS PubMed.
- N. Muniraj and K. R. Prabhu, Co(III)-catalyzed C–H activation: a site-selective conjugate addition of maleimide to indole at the C-2 position, ACS Omega, 2017, 2, 4470 CrossRef CAS PubMed.
- N. Muniraj and K. R. Prabhu, Cobalt(III)-catalyzed C-H activation: azo directed selective 1,4-addition of ortho C-H bond to maleimides, J. Org. Chem., 2017, 82, 6913 CrossRef CAS PubMed.
- S.-L. Liu, Y. Li, J.-R. Guo, G.-C. Yang, X.-H. Li, J.-F. Gong and M.-P. Song, An approach to 3-(indol-2-yl)succinimide derivatives by manganese-catalyzed C–H activation, Org. Lett., 2017, 19, 4042 CrossRef CAS PubMed.
- K. R. Bettadapur, V. Lanke and K. R. Prabhu, A deciduous directing group approach for the addition of aryl and vinyl nucleophiles to maleimides, Chem. Commun., 2017, 53, 6251 RSC.
- Q. He, T. Yamaguchi and N. Chatani, Rh(I)-catalyzed alkylation of ortho-C–H bonds in aromatic amides with maleimides, Org. Lett., 2017, 19, 4544 CrossRef CAS PubMed.
- C. Pan, Y. Wang, C. Wu and J.-T. Yu, Rhodium-catalyzed C7-alkylation of indolines with maleimides, Org. Biomol. Chem., 2018, 16, 693 RSC.
- J.-T. Yu, R. Chen, H. Jia and C. Pan, Rhodium-catalyzed site-selective ortho-C-H activation: enone carbonyl directed hydroarylation of maleimides, J. Org. Chem., 2018, 83, 12086 CrossRef CAS PubMed.
- J. Zhao, C. Pi, C. You, Y. Wang, X. Cui and Y. Wu, Rhodium(III)-catalyzed direct C-H alkylation of 2-aryl-1,2,3-triazole N-oxides with maleimides, Eur. J. Org. Chem., 2018, 6919 CrossRef CAS.
- K. N. Reddy, M. V. K. Rao, B. Sridhar and B. V. S. Reddy, Ru(II)-catalyzed hydroarylation of maleimides with cyclic N-sulfonylketimines through ortho-C-H bond activation, ChemistrySelect, 2018, 3, 5062 CrossRef CAS.
- F. Li, Y. Zhou, H. Yang, D. Liu, B. Sun and F.-L. Zhang, Assembly of diverse spirocyclic pyrrolidines via transient directing group enabled ortho-C(sp2)-H alkylation of benzaldehydes, Org. Lett., 2018, 20, 146 CrossRef CAS PubMed.
- X. Chen, J. Ren, H. Xie, W. Sun, M. Sun and B. Wu, Cobalt(III)-catalyzed 1,4-addition of C-H bonds of oximes to maleimides, Org. Chem. Front., 2018, 5, 184 RSC.
- R. Mandal, B. Emayavaramban and B. Sundararaju, Cp*Co(III)-catalyzed C-H alkylation with maleimides using weakly coordinating carbonyl directing groups, Org. Lett., 2018, 20, 2835 CrossRef CAS PubMed.
- Y.-C. Yuan, M. Goujon, C. Bruneau, T. Roisnel and R. G. Doria, Site-Selective ruthenium-catalyzed C-H bond arylations with boronic acids: exploiting isoindolinones as a weak directing group, J. Org. Chem., 2019, 84, 16183 CrossRef CAS PubMed.
- S. K. Banjare, R. Chebolu and P. C. Ravikumar, Cobalt catalyzed hydroarylation of Michael acceptors with indolines directed by a weakly coordinating functional group, Org. Lett., 2019, 21, 4049 CrossRef CAS.
- T. Tian, A.-S. Dong, D. Chen, X.-T. Cao and G. Wang, Regioselective C–C cross-coupling of 1,2,4-thiadiazoles with maleimides through iridium-catalyzed C-H activation, Org. Biomol. Chem., 2019, 17, 7664 RSC.
- A. Mandal, H. Sahoo, S. Dana and M. Baidya, Ruthenium(II)-catalyzed hydroarylation of maleimides using carboxylic acids as a traceless directing group, Org. Lett., 2017, 19, 4138 CrossRef CAS PubMed.
- N. Y. P. Kumar, T. Rogge, S. R. Yetra, A. Bechtoldt, E. Clot and L. Ackermann, Mild decarboxylative C–H alkylation: computational insights for solvent-robust Ruthenium(II) domino manifold, Chem. – Eur. J., 2017, 23, 17449 CrossRef CAS PubMed.
- F. Pu, Z.-W. Liu, L.-Y. Zhang, J. Fan and X.-Y. Shi, Switchable C-H alkylation of aromatic acids with maleimides in water: carboxyl as a diverse directing group, ChemCatChem, 2019, 11, 4116 CrossRef CAS.
- F. Han, S. Xun, L. Jia, Y. Zhang, L. Zou and X. Hu, Traceless-activation strategy for Rh-catalyzed Csp2-H arylation of coumarins, Org. Lett., 2019, 21, 5907 CrossRef CAS PubMed.
- M. Nagamoto and T. Nishimura, Stereoselective hydroacylation of bicyclic alkenes with 2-hydroxybenzaldehydes catalyzed by hydroxoiridium/diene complexes, Chem. Commun., 2015, 51, 13791 RSC.
- M. Nagamoto, J.-I. Fukuda, M. Hatano, H. Yorimitsu and T. Nishimura, Hydroxoiridium-catalyzed hydroarylation of alkynes and bicycloalkenes with N-sulfonylbenzamides, Org. Lett., 2017, 19, 5952 CrossRef CAS PubMed.
- H. Cheng, W. Dong, C. A. Dannenberg, S. Dong, Q. Guo and C. Bolm, Ruthenium-catalyzed hydroarylations of oxa- and azabicyclic alkenes, ACS Catal., 2015, 5, 2770 CrossRef CAS.
- P. S. Aparna, A. Vijayan, S. P. Raveendran, E. Suresh, R. L. Varma and K. V. Radhakrishnan, Ruthenium/Iridium-catalyzed C-2 activation of indoles with bicyclic olefins: an easy access to functionalized heterocyclic motifs, Synlett, 2017, 572 CAS.
- K. Shibata, S. Natsui, M. Tobisu, Y. Fukumoto and N. Chatani, An unusual endo-selective C-H hydroarylationof norbornene by the Rh(I)-catalyzed reaction of benzamides, Nat. Commun., 2017, 8, 1448 CrossRef PubMed.
- S. Prakash, Y.-C. Chang and C.-H. Cheng, Rhenium(I)-catalyzed ortho-C–H Addition to bicyclic alkenes, Chem. – Asian J., 2018, 13, 1664 CrossRef CAS PubMed.
- D.-Y. Li, Z.-L. Huang and P.-N. Liu, Heterobicyclic core retained hydroarylations through C-H activation: synthesis of epibatidine analogues, Org. Lett., 2018, 20, 2028 CrossRef CAS PubMed.
- P. Wang, Y. Xu, J. Sun and X. Li, Rhodium(III)-catalyzed chemo-divergent couplings of sulfoxonium ylides with oxa/azabicyclic olefins, Org. Lett., 2019, 21, 8459 CrossRef CAS PubMed.
- Y. Zhu, F. Chen, X. Zhao, D. Yan, W. Yong and J. Zhao, Cobalt(III)-catalyzed intermolecular carboamination of propiolates and bicyclic alkenes via non-annulative redox-neutral coupling, Org. Lett., 2019, 21, 5884 CrossRef CAS PubMed.
- P. V. Santhini, G. Nimisha, J. John, E. Suresh, R. L. Varma and K. V. Radhakrishnan, Pd-Catalyzed oxidative annulation of enamides with diazabicyclic olefins: rapid access to cyclopentene fused 2-pyrrolines, Chem. Commun., 2017, 53, 1848 RSC.
- P. V. Santhinia, G. Gopalana, A. S. Smrithya and K. V. Radhakrishnan, Rhodium(III)-catalyzed C-H activation/alkylation of diazabicyclic olefins with aryl ketones: facile synthesis of functionalized cyclopentenes, Synlett, 2018, 2023 Search PubMed.
- Q. Lu, F. J. R. Klauck and F. Glorius, Manganese-catalyzed allylation via sequential C-H and C-C/C-Het bond activation, Chem. Sci., 2017, 8, 3379 RSC.
- Y. Zhang, T. Shang, L. Li, Y. He, T. Wen, Y. Tu, S. Wang and D. Lin, Rh(III)-catalyzed C-H activation-desymmetrization of diazabicycles using enol as directing group: A straightforward approach to difunctionalized cyclopentenes, Tetrahedron Lett., 2018, 59, 1394 CrossRef CAS.
- X. Yang, G. Zheng and X. Li, Rhodium(III)-catalyzed enantioselective coupling of indoles and 7-azabenzonorbornadienes by C-H activation/desymmetrization, Angew. Chem., Int. Ed., 2019, 58, 322 CrossRef CAS PubMed.
- R. Mi, G. Zheng, Z. Qi and X. Li, Rhodium-catalyzed enantioselective oxidative [3 + 2]-annulation of arenes and azabicyclic olefins through twofold C-H activation, Angew. Chem., Int. Ed., 2019, 58, 17666 CrossRef CAS PubMed.
- S.-G. Wang and N. Cramer, An enantioselective CpxRh(III)-catalyzed C-H functionalization/ring-opening route to chiral cyclopentenylamines, Angew. Chem., Int. Ed., 2019, 58, 2514 CrossRef CAS PubMed.
- B. Duchemin, G. Smits and N. Cramer, RhI, IrIII and CoIII complexes with atropchiral biaryl cyclopentadienyl ligands: syntheses,
structures and catalytic activities, Organometallics, 2019, 38, 3939 CrossRef.
- X. Zhang, Y. Gao, J. Chen, R. Fan, G. Shi, Z. He and B. Fan, Rh(III)-catalyzed ring-opening addition of azabenzonorbornadienes with cyclic N-sulfonyl ketimines via C–H bond activation, Adv. Synth. Catal., 2019, 361, 4495 CrossRef CAS.
- V. Vinayagam, A. Mariappan, M. Jana and M. Jeganmohan, Rhodium(III)-catalyzed diastereoselective ring-opening of 7-azabenzonorbornadienes with aromatic ketoximes: synthesis of benzophenanthridine derivatives, J. Org. Chem., 2019, 84, 15590 CrossRef CAS PubMed.
- Z. Zhou, M. Bian, L. Zhao, H. Gao, J. Huang, X. Liu, X. Yu, X. Li and W. Yi, 2H-Chromene-3-carboxylic acid synthesis via solvent-controlled and Rhodium(III)-catalyzed redox-neutral C–H Activation/[3 + 3] annulation cascade, Org. Lett., 2018, 20, 3892 CrossRef CAS PubMed.
- G. Zhu, W. Shi, H. Gao, Z. Zhou, H. Song and W. Yi, Chemodivergent couplings of n-arylureas and methyleneoxetanones via Rh(III)-catalyzed and solvent-controlled C–H activation, Org. Lett., 2019, 21, 4143 CrossRef CAS PubMed.
- Y. Xu, L. Zhang, M. Liu, X. Zhang, X. Zhang and X. Fan, Synthesis of benzoazepine derivatives via Rh(III)-catalyzed inert C(sp2)–H functionalization and [4 + 3] annulation, Org. Biomol. Chem., 2019, 17, 8706 RSC.
- Z.-J. Jia, C. Merten, R. Gontla, C. G. Daniliuc, A. P. Antonchick and H. Waldmann, General enantioselective C-H activation with efficiently tunable cyclopentadienyl ligands, Angew. Chem., Int. Ed., 2017, 56, 2429 CrossRef CAS PubMed.
- W. Miura, K. Hirano and M. Miura, Copper-mediated oxidative coupling of benzamides with maleimides via directed C–H cleavage, Org. Lett., 2015, 17, 4034 CrossRef CAS PubMed.
- S.-S. Li, H. Lin, C.-F. Liu, Y.-Q. Xia, X.-M. Zhang and L. Dong, Rhodium-catalyzed tandem annulation reactions of 7-azaindoles with electron-deficient olefins via double C-H activation, Adv. Synth. Catal., 2016, 358, 1595 CrossRef CAS.
- N. Lv, Y. Liu, C. Xiong, Z. Liu and Y. Zhang, Cobalt-catalyzed oxidant-free spirocycle synthesis by liberation of hydrogen, Org. Lett., 2017, 19, 4640 CrossRef CAS PubMed.
- R. Manoharan and M. Jeganmohan, Cobalt-catalyzed oxidative cyclization of benzamides with maleimides: synthesis of isoindolone spirosuccinimides, Org. Lett., 2017, 19, 5884 CrossRef CAS PubMed.
- H. Zhao, X. Shao, T. Wang, S. Zhai, S. Qiu, C. Tao, H. Wanga and H. Zhai, A 2-(1-methylhydrazinyl)pyridine-directed C–H functionalization/spirocyclization cascade: facile access to spirosuccinimide derivatives, Chem. Commun., 2018, 54, 4927 RSC.
- F. W. Patureau and F. Glorius, Oxidizing directing groups enable efficient and innovative C-H activation reactions, Angew. Chem., Int. Ed., 2011, 50, 1977 CrossRef CAS PubMed.
- B. Ramesh, M. Tamizmani and M. Jeganmohan, Rhodium(III)-catalyzed redox-neutral 1,1-cyclization of N-methoxy benzamides with maleimides via C–H/N–H/N–O activation: detailed mechanistic investigation, J. Org. Chem., 2019, 84, 4058 CrossRef CAS PubMed.
- S. Devkota, H.-J. Lee, S. H. Kim and Y. R. Lee, Direct construction of diverse polyheterocycles bearing pyrrolidinediones via Rh(III)-catalyzed cascade C–H activation/spirocyclization, Adv. Synth. Catal., 2019, 361, 5587 CrossRef CAS.
- M. Sheykhan, M. Shafiee-Pour and M. Abbasnia, C–H activation under the guise of Diels–Alder reaction: annulation toward the synthesis of benzo[e]isoindole-1,3-diones, Org. Lett., 2017, 19, 1270 CrossRef CAS PubMed.
- Q. Wang, Y. Li, Z. Qi, F. Xie, Y. Lan and X. Li, Rhodium(III)-catalyzed annulation between N-sulfinyl ketoimines and activated olefins: C–H activation assisted by an oxidizing N–S bond, ACS Catal., 2016, 6, 1971 CrossRef CAS.
- S. H. Han, N. K. Mishra, H. Jo, Y. Oh, M. Jeon, S. Kim, W. J. Kim, J. S. Lee, H. S. Kim and I. S. Kim, One-pot synthesis of oxindoles through C-H alkylation and intramolecular cyclization of azobenzenes with internal olefins, Adv. Synth. Catal., 2017, 359, 2396 CrossRef CAS.
- S. Sharma, Y. Oh, N. K. Mishra, U. De, H. Jo, R. Sachan, H. S. Kim, Y. H. Jung and I. S. Kim, Rhodium-catalyzed [3 + 2]-annulation of cyclic N-acyl ketimines with activated olefins: anticancer activity of spiroisoindolinones, J. Org. Chem., 2017, 82, 3359 CrossRef CAS PubMed.
- S. H. Han, N. K. Mishra, M. Jeon, S. Kim, H. S. Kim, S.-Y. Jung, Y. H. Jung, J.-M. Ku and I. S. Kim, Rhodium(III)-catalyzed diastereoselective synthesis of 1-aminoindanes via C–H activation, Adv. Synth. Catal., 2017, 359, 3900 CrossRef CAS.
- C. Zhu, J. Luan, J. Fang, X. Zhao, X. Wu, Y. Li and Y. Luo, A Rhodium-catalyzed [3 + 2] annulation of general aromatic aldimines/ketimines and N-substituted maleimides, Org. Lett., 2018, 20, 5960 CrossRef CAS PubMed.
- M. Tamizmzni, B. Ramesh and M. Jeganmohan, Ruthenium(II)-catalyzed redox-free [3 + 2]-cycloaddition of N-sulfonyl aromatic aldimines with maleimides, J. Org. Chem., 2018, 83, 3746 CrossRef PubMed.
- R. Manoharan, R. Logeswaran and M. Jeganmohan, Ru(II)- or Rh(III)-catalyzed difunctionalization of alkenes by tandem cyclization of N-aryl acrylamides with alkenes, J. Org. Chem., 2019, 84, 14830 CrossRef CAS PubMed.
- H. Li, S. Zhang, X. Feng, X. Yu, Y. Yamamoto and M. Bao, Rhodium(III)-catalyzed oxidative [3 + 2] annulation of 2-acetyl-1-arylhydrazines with maleimides: synthesis of pyrrolo[3,4-b]indole-1,3-diones, Org. Lett., 2019, 21, 8563 CrossRef CAS PubMed.
- N. Muniraj and K. R. Prabhu, Cobalt(III)-catalyzed-[4 + 2] Annulation of N-chlorobenzamides with maleimides, Org. Lett., 2019, 21, 1068 CrossRef CAS PubMed.
- C. Guo, B. Li, H. Liu, X. Zhang, X. Zhang and X. Fan, Synthesis of fused or spiro polyheterocyclic compounds via the dehydrogenative annulation reactions of 2-arylindazoles with maleimides, Org. Lett., 2019, 21, 7189 CrossRef CAS PubMed.
- W. Zhu, S. Tong, J. Zhu and M.-X. Wang, Intramolecular arylation of tertiary enamides through Pd(OAc)2-c atalyzed dehydrogenative cross-coupling reaction: construction of fused N-heterocyclic scaffolds and synthesis of isoindolobenzazepine alkaloids, J. Org. Chem., 2019, 84, 2870 CrossRef CAS PubMed.
- K. Ghosh, Y. Nishii and M. Miura, Rhodium-catalyzed annulative coupling using vinylene carbonate as an oxidizing acetylene surrogate, ACS Catal., 2019, 9, 11455 CrossRef CAS.
- Z. Liu, W. Zhang, S. Guo and J. Zhu, Spiro[indene-1,4′-oxa-zolidinones] synthesis via Rh(III)-catalyzed coupling of 4-phenyl-1,3-oxazol-2(3H)-ones with alkynes: a redox-neutral approach, J. Org. Chem., 2019, 84, 11945 CrossRef CAS PubMed.
- S. Borthakur, B. Sarma and S. Gogoi, Ruthenium(II)-catalyzed oxidative double C–H activation and annulation reaction: synthesis of indolo[2,1-a]isoquinolines, Org. Lett., 2019, 21, 7878 CrossRef CAS PubMed.
- Y.-Q. Zhu, T.-F. Han, J.-L. He, M. Li, J.-X. Li and K. Zhu, Route to substituted furan-2(5H)-ones from cyclo-alkenecarboxylic acids and acrylates via C–H activation, J. Org. Chem., 2017, 82, 8598 CrossRef CAS PubMed.
-
(a) T. A. Davis, T. K. Hyster and T. Rovis, Rhodium(III)-catalyzed intramolecular hydroarylation, amidoarylation, and Heck-type reaction: three distinct pathways determined by an amide directing group, Angew. Chem., Int. Ed., 2013, 52, 14181 CrossRef CAS PubMed;
(b) N. Quiñones, A. Seoane, R. García-Fandiño, J. L. Mascareñas and M. Gulías, Rhodium(III)-catalyzed intramolecular annulations involving amide-directed C-H activations: synthetic scope and mechanistic studies, Chem. Sci., 2013, 4, 2874 RSC;
(c) Z. Shi, M. Boultadakis-Arapinis, D. C. Koester and F. Glorius, Rh(III)-Catalyzed intramolecular redox-neutral cyclization of alkenes via C-H activation, Chem. Commun., 2014, 50, 2650 RSC;
(d) B. Ye, P. A. Donets and N. Cramer, Chiral Cp-Rhodium(III)-catalyzed asymmetric hydroarylations of 1,1-disubstituted alkenes, Angew. Chem., Int. Ed., 2014, 53, 507 CrossRef CAS PubMed;
(e) S. Guo, P. Li, Z. Guan, L. Cai, S. Chen, A. Lin and H. Yao, Pd-catalyzed alkyne insertion/C-H activation/[4 + 2]-carboannulation of alkenes to the synthesis of polycyclics, Org. Lett., 2019, 21, 921 CrossRef CAS PubMed;
(f) T. J. Saiegh, H. Chédotal, C. Meyer and J. Cossy, Rhodium(III)-catalyzed C(sp2)–H functionalization of cyclobutenes. access to cyclobuta[c]pyridones and -pyridine, Org. Lett., 2019, 21, 8364 CrossRef CAS PubMed.
- A. Peneau, P. Retailleau, C. Guillou and L. Chabaud, Rhodium(III)-catalyzed synthesis of spiropiperidine derivatives via C-H activation, J. Org. Chem., 2018, 83, 2324 CrossRef CAS PubMed.
- K. F. Johnson, E. A. Schneider, B. P. Schumacher, A. Ellern, J. D. Scanlon and L. M. Stanley, Rhodium-catalyzed enantioselective intramolecular hydroacylation of trisubstituted alkenes, Chem. – Eur. J., 2016, 22, 15619 CrossRef CAS PubMed.
- P. A. Donets and N. Cramer, Ligand-controlled regiodivergent nickel-catalyzed annulation of pyridones, Angew. Chem., Int. Ed., 2015, 54, 633 CAS.
- J. Diesel, A. M. Finogenova and N. Cramer, Nickel-catalyzed enantioselective pyridone C-H functionalizations enabled by a bulky N-heterocyclic carbene ligand, J. Am. Chem. Soc., 2018, 140, 4489 CrossRef CAS PubMed.
- T. Katsina, E. E. Anagnostaki, F. Mitsa, V. Sarli and A. L. Zografos, Palladium-catalyzed direct alkenylation of 4-hydroxy-2-pyridones, RSC Adv., 2016, 6, 6978 RSC.
- L. M. Pardo, A. M. Prendergast, M.-T. Nolan, E. Ó. Muimhneacháin and G. P. McGlacken, Pd/pivalic acid mediated direct arylation of 2-pyrones and related heterocycles, Eur. J. Org. Chem., 2015, 3540 CrossRef CAS.
- M.-T. Nolan, L. M. Pardo, A. M. Prendergast and G. P. McGlacken, Intramolecular direct arylation of 3-halo-2-pyrones and 2-coumarins, J. Org. Chem., 2015, 80, 10904 CrossRef CAS PubMed.
- A. M. Prendergast, L. M. Pardo, I. J. S. Fairlamb and G. P. McGlacken, Access to some C5-cyclised 2-pyrones and 2-pyridones via direct arylation; retention of chloride as a synthetic handle, Eur. J. Org. Chem., 2017, 5119 CrossRef CAS.
- K. Mackey, L. M. Pardo, A. M. Prendergast, M.-T. Nolan, L. M. Bateman and G. P. McGlacken, Cyclization of 4-phenoxy-2-coumarins and 2-pyrones via a double C–H activation, Org. Lett., 2016, 18, 2540 CrossRef CAS PubMed.
- W. Miura, K. Hirano and M. Miura, Rhodium-catalyzed C6-selective C–H borylation of 2-pyridones, Org. Lett., 2016, 18, 3742 CrossRef CAS PubMed.
- T. Li, Z. Wang, K. Xu, W. Liu, X. Zhang, W. Mao, Y. Guo, X. Ge and F. Pan, Rhodium-catalyzed/copper-mediated tandem C(sp2)-H alkynylation and annulation: synthesis of 11-acylated imidazo[1,2-a: 3,4-a′]dipyridin-5-ium-4-olates from 2H-[1,2′bipyridin]-2-ones and propargyl alcohols, Org. Lett., 2016, 18, 1064 CrossRef CAS PubMed.
- S. Yu, Y. Li, X. Zhou, H. Wang, L. Kong and X. Li, Access to structurally diverse quinoline-fused heterocycles via rhodium(III)-catalyzed C-C/C-N coupling of bifunctional substrates, Org. Lett., 2016, 18, 2812 CrossRef CAS PubMed.
- X. Zhou, Y. Pan and X. Li, Catalyst-controlled regiodivergent alkyne insertion in the context of C-H activation and Diels-Alder reactions: synthesis of fused and bridged cycles, Angew. Chem., Int. Ed., 2017, 56, 8163 CrossRef CAS PubMed.
- A. Biswas, D. Giri, D. Das, A. De, S. K. Patra and R. Samanta, A Mild rhodium catalyzed direct synthesis of quinolones from pyridones: application in the detection of nitroaromatics, J. Org. Chem., 2017, 82, 10989 CrossRef CAS PubMed.
- S.-Y. Chen, X.-L. Han, J.-Q. Wu, Q. Li, Y. Chen and H. Wang, Manganese(I)-catalyzed regio- and stereoselective 1,2-diheteroarylation of allenes: combination of C-H activation and Smiles rearrangement, Angew. Chem., Int. Ed., 2017, 56, 9939 CrossRef CAS PubMed.
- S.-Y. Chen, Q. Li and H. Wang, Manganese(I)-catalyzed direct C–H allylation of arenes with allenes, J. Org. Chem., 2017, 82, 11173 CrossRef CAS PubMed.
- C. Zhu, R. Kuniyil and L. Ackermann, Manganese(I)-catalyzed C-H activation/Diels-Alder/retro-Diels-Alder domino alkyne annulation featuring transformable pyridines, Angew. Chem., Int. Ed., 2019, 58, 5338 CrossRef CAS PubMed.
- C.-J. Zhou, H. Gao, S.-L. Huang, S.-S. Zhang, J.-Q. Wu, B. Li, X. Jiang and H. Wang, Synthesis of benzofused N-heterocycles via Rh(III)-catalyzed direct benzannulation with 1,3-dienes, ACS Catal., 2019, 9, 556 CrossRef CAS.
- D. Bai, J. Xia, F. Song, X. Li, B. Liu, L. Liu, G. Zheng, X. Yang, J. Sun and X. Li, Rhodium(III)-catalyzed diverse [4 + 1]-annulation of arenes with 1,3-enynes via sp3/sp2 C–H activation and 1,4-rhodium migration, Chem. Sci., 2019, 10, 3987 RSC.
- Y. Shin, C. Yoo, Y. Moon, Y. Lee and S. Hong, Efficient synthesis of frutinone A and its derivatives through palladium-catalyzed C-H activation/carbonylation, Chem. – Asian J., 2015, 10, 878 CrossRef CAS PubMed.
- C. Cheng, W.-W. Chen, B. Liu and M.-H. Hu, Intramolecular cross-dehydrogenative coupling of 4-substituted coumarins: rapid and efficient access to coumestans and indole[3,2-c]coumarins, Org. Chem. Front., 2016, 3, 1111 RSC.
- C. Cheng, W.-W. Chen, B. Xu and M.-H. Hu, Access to indole-fused polyheterocycles via pd-catalyzed base-free intramolecular cross-dehydrogenative coupling, J. Org. Chem., 2016, 81, 11501 CrossRef CAS PubMed.
-
(a) T. Balalas, A. Abdul-Sada, D. J. Hadjipavlou-Litina and K. E. Litinas, Pd-catalyzed efficient synthesis of azacoumestans via intramolecular cross coupling of 4-(arylamino)coumarins in the presence of copper acetate under microwaves, Synthesis, 2017, 2575 CAS;
(b) A. Dey, M. A. Ali, S. Jana, S. Samanta and A. Hajra, Palladium-catalyzed synthesis of indole fused coumarins via cross-dehydrogenative coupling, Tetrahedron Lett., 2017, 58, 313 CrossRef CAS.
- J. Zhang, Y. Zhuang, Y. Ma, X. Yang and M. Szostak, Palladium-catalyzed synthesis of benzothiophenes via cross-dehydrogenative coupling of 4-arylthiocoumarins and pyrones, Adv. Synth. Catal., 2019, 361, 5709 CrossRef CAS.
- K. R. Reddy, P. Kannaboina and P. Das, Palladium-catalyzed chemoselective switch: synthesis of a new class of indenochromenes and pyrano[2,3-c]carbazoles, Asian J. Org. Chem., 2017, 6, 534 CrossRef CAS.
- X. Wu, Y. Yang, J. Han and L. Wang, Palladium catalyzed C–I and vicinal C–H dual activation of diaryliodonium salts for diarylation: synthesis of 4,5-benzocoumarins, Org. Lett., 2015, 17, 5654 CrossRef CAS PubMed.
- K. Neog, A. Borah and P. Gogoi, Palladium(II)-catalyzed C–H bond activation/C-C and C-O bond formation reaction cascade: direct synthesis of coumestans, J. Org. Chem., 2016, 81, 11971 CrossRef CAS PubMed.
- R. N. P. Tulichala, M. Shanker and K. C. K. Swamy, Ruthenium-catalyzed oxidative annulation and hydroarylation of chromene-3-carboxamides with alkynes via double C-H functionalization, J. Org. Chem., 2017, 82, 5068 CrossRef CAS PubMed.
- J.-S. Li, X.-Y. Xie, Q. Yang, P.-P. Yang, S. Jiang, Z.-W. Li, C.-H. Lu and W.-D. Liu, Synthesis of chromeno[3,4-c]pyridines by Rhodium(III)-catalyzed annulation of coumarinyl ketoxime esters and alkynes, Tetrahedron, 2019, 75, 4602 CrossRef CAS.
- D.
Y. Li, L. L. Jiang, S. Chen, Z. L. Huang, L. Dang, X. Y. Wu and P. N. Liu, Cascade reaction of alkynols and 7-oxabenzonorbornadienes involving transient hemiketal group directed C–H activation and synergistic RhIII/ScIII catalysis, Org. Lett., 2016, 18, 5134 CrossRef CAS PubMed.
- P. Gandeepan, P. Rajamalli and C.-H. Cheng, Diastereoselective [3 + 2]-annulation of aromatic/vinylic amides with bicyclic alkenes through cobalt-catalyzed C-H activation and intramolecular nucleophilic addition, Angew. Chem., Int. Ed., 2016, 55, 4308 CrossRef CAS PubMed.
- Y. Cheng, K. Parthasarathy and C. Bolm, Rhodium(III)-catalyzed annulation of N-methoxybenzamides with heterobicyclic alkenes by C-H functionalization: synthesis of benzo[b]phenanthridinones, Eur. J. Org. Chem., 2017, 1203 CrossRef CAS.
- P. Li, J. Wang, H. Li, W. Dong, Z. Peng and D. An, RhIII-catalyzed annulation of N-methoxyindozamides with heterobicyclic alkenes towards indolo[3,2-c]heteroarenes, Synth. Commun., 2018, 48, 1919 CrossRef CAS.
- X. Wang, A. Lerchen, T. Gensch, T. Knecht, C. G. Daniliuc and F. Glorius, Combination of Cp*RhIII-catalyzed C-H activation and a Wagner-Meerwein-type rearrangement, Angew. Chem., Int. Ed., 2017, 56, 1381 CrossRef CAS PubMed.
- X. Wang, Y. Li, T. Knecht, C. G. Daniliuc, K. N. Houk and F. Glorius, Unprecedented dearomatized spirocyclopropane in a sequential Rhodium(III)-catalyzed C-H activation and rearrangement reaction, Angew. Chem., Int. Ed., 2018, 57, 5520 CrossRef CAS PubMed.
- Z. Jin, Y. C. Teo, N. G. Zulaybar, M. D. Smith and Y. Xia, Streamlined synthesis of polycyclic conjugated hydrocarbons containing cyclobutadienoids via C-H activated annulation and aromatization, J. Am. Chem. Soc., 2017, 139, 1806 CrossRef CAS PubMed.
- P. V. Santhini, A. S. Smrithy, C. P. I. Jesin, S. Varughese, J. John and K. V. Radhakrishnan, Accessing highly functionalized cyclopentanoids via a cascade palladation approach: unprecedented benzylic C-H activation towards cyclopentenoindanes, Chem. Commun., 2018, 54, 2982 RSC.
- N. Vijayan, T. V. Baiju, E. Jijy, P. Prakash, M. Shimi, N. Joseph, P. M. Pihko, S. Varughese and K. V. Radhakrishnan, An easy access to fused chromanones via rhodium catalyzed oxidative coupling of salicylaldehydes with heterobicyclic olefins, Tetrahedron, 2016, 72, 4007 CrossRef.
- N. Vijayan, C. U. Jumaila and K. V. Radhakrishnan, Rhodium(III)-catalyzed C-H activation of O-acetyl ketoximes/N-methoxybenzamides toward the synthesis of isoquinoline/isoquinolone-fused bicycles, Asian J. Org. Chem., 2017, 6, 1561 CrossRef.
- E. A. Trifonova, N. M. Ankudinov, A. A. Mikhaylov, D. A. Chusov, Y. V. Nelyubina and D. S. Perekalin, A planar-chiral Rhodium(III) catalyst with a sterically demanding cyclopentadienyl ligand and its application in the enantioselective synthesis of dihydroisoquinolones, Angew. Chem., Int. Ed., 2018, 57, 7714 CrossRef CAS PubMed.
- R. Nallagonda, N. Thrimurtulu and C. M. R. Volla, Cobalt-catalyzed diastereoselective [4 + 2]-annulation of phosphinamides with heterobicyclic alkenes at room temperature, Adv. Synth. Catal., 2018, 360, 255 CrossRef CAS.
- A. Dey, A. Rathi and C. M. R. Volla, Cobalt(III)-catalyzed [4 + 2]-annulation of heterobicyclic alkenes by sp2 C–H activation, Asian J. Org. Chem., 2018, 7, 1362 CrossRef CAS.
- K. Ozols, Y.-S. Jang and N. Cramer, Chiral cyclopentadienyl Cobalt(III) complexes enable highly enantioselective 3d-metal-catalyzed C-H functionalizations, J. Am. Chem. Soc., 2019, 141, 5675 CrossRef CAS PubMed.
- A. Skhiri and N. Chatani, Nickel-catalyzed reaction of benzamides with bicyclic alkenes: cleavage of C–H and C–N bonds, Org. Lett., 2019, 21, 1774 CrossRef CAS PubMed.
- S. Qiu, S. Zhai, H. Wang, X. Chena and H. Zhai, One-pot synthesis of benzo[b]fluorenones via a cobalt-catalyzed MHP-directed [3 + 2]-annulation/ring-opening/dehydration sequence, Chem. Commun., 2019, 55, 4206 RSC.
- S. V. Kumar, S. Banerjee and T. Punniyamurthy, Rh-Catalyzed C-C/C-N bond formation via C–H activation: synthesis of 2H-indazol-2-yl-benzo[a]carbazoles, Org. Chem. Front., 2019, 6, 3885 RSC.
- S. Banerjee, S. V. Kumar and T. Punniyamurthy, Site-selective Rh-catalyzed C-7 and C-6 dual C-H functionalization of indolines: synthesis of functionalized pyrrolocarbazoles, J. Org. Chem., 2020, 85, 2793 CrossRef CAS PubMed.
- S.-Y. Yang, W.-Y. Han, C. He, B.-D. Cui, N.-W. Wan and Y.-Z. Chen, 2,2-Bifunctionalization of norbornene in palladium-catalyzed domino annulation, Org. Lett., 2019, 21, 8857 CrossRef CAS PubMed.
- D. Kang and S. Hong, Rh(III) and Ru(II)-catalyzed site-selective C–H alkynylation of quinolones, Org. Lett., 2015, 17, 1938 CrossRef CAS PubMed.
- Y. Li, F. Xie and X. Li, Formal gold- and rhodium-catalyzed regiodivergent C–H alkynylation of 2-pyridones, J. Org. Chem., 2016, 81, 715 CrossRef CAS PubMed.
- X. Wang, X. Li, Y. Zhang and L. Xia, Gold(I) and rhodium(III)-catalyzed formal regiodivergent C-H alkynylation of 1-arylpyrazolones, Org. Biomol. Chem., 2018, 16, 2860 RSC.
- J. Xia, L. Kong, X. Zhou, G. Zheng and X. Li, Access to substituted propenoic acids via Rh(III)-catalyzed C–H allylation of (hetero)arenes with methyleneoxetanones, Org. Lett., 2017, 19, 5972 CrossRef CAS PubMed.
- M. Bian, K. Ma, H. Mawjuda, X. Yu, X. Li, H. Gao, Z. Zhou and W. Yi, Rhodium(III)-catalyzed chemoselective C–H functionalization of benzamides with methyleneoxetanones controlled by the solvent, Org. Biomol. Chem., 2019, 17, 6114 RSC.
- J. Ni, H. Zhao and A. Zhang, Manganese(I)-catalyzed C-H 3,3-difluoroallylation of pyridones and indoles, Org. Lett., 2017, 19, 3159 CrossRef CAS PubMed.
- T. Kittikool, A. Thupyai, K. Phomphrai and S. Yotphan, Copper/persulfate-promoted oxidative decarboxylative C-H acylation of pyrazolones with α-oxocarboxylic acids: direct access to 4-acylpyrazolones under mild conditions, Adv. Synth. Catal., 2018, 360, 3345 CrossRef CAS.
- Y. Xiao, X. Wu, J. Teng, S. Sun, J.-T. Yu and J. Cheng, Copper-catalyzed acylation of pyrazolones with aldehydes to afford 4-acylpyrazolones, Org. Biomol. Chem., 2019, 17, 7552 RSC.
- P. Peng, J. Wang, C. Li, W. Zhu, H. Jiang and H. Liu, Cu(II)-catalyzed C6-selective C–H thiolation of 2-pyridones using air as the oxidant, RSC Adv., 2016, 6, 57441 RSC.
- S. Dana, A. Mandal, H. Sahoo and M. Baidya, Ru(II)-catalyzed C–H functionalization on maleimides with electrophiles: a demonstration of umpolung strategy, Org. Lett., 2017, 19, 1902 CrossRef CAS PubMed.
- W. Ma, H. Dong, D. Wang and L. Ackermann, Late-stage diversification of non-steroidal anti-inflammatory drugs by transition metal-catalyzed C-H alkenylations, thiolations and selenylations, Adv. Synth. Catal., 2017, 359, 966 CrossRef CAS.
- J. Zhu, B. Xu, J. Yu, Y. Ren, J. Wang, P. Xie, C. U. Pittman Jr. and A. Zhou, Copper-catalyzed generation of flavone selenide and thioether derivatives using KSeCN and KSCN via C–H functionalization, Org. Biomol. Chem., 2018, 16, 5999 RSC.
- M. Noikham and S. Yotphan, Copper-catalyzed regioselective direct C–H thiolation and thiocyanation of uracils, Eur. J. Org. Chem., 2019, 2759 CrossRef CAS.
- F. Gao, X. Han, C. Li, L. Liu, Z. Congae and H. Liu, Cobalt(III)-catalyzed site-selective C–H amidation of pyridones and isoquinolones, RSC Adv., 2018, 8, 32659 RSC.
- D. Das and R. Samanta, Iridium(III)-catalyzed regiocontrolled direct amidation of isoquinolones and pyridones, Adv. Synth. Catal., 2018, 360, 379 CrossRef CAS.
- Z. Liu, F. Huang, J. Lou, Q. Wang and Z. Yu, Copper-promoted direct C–H alkoxylation of S,S-functionalized internal olefins with alcohols, Org. Biomol. Chem., 2017, 15, 5535 RSC.
- S. Hazra, K. Hirano and M. Miura, Pyridine-directed Rh-catalyzed C6-selective C–H acetoxylation of 2-pyridones, Heterocycles, 2019, 101, 223 Search PubMed.
|
This journal is © the Partner Organisations 2020 |