DOI:
10.1039/C4RA07173E
(Paper)
RSC Adv., 2014,
4, 43477-43484
Journey on greener pathways: use of Euphorbia condylocarpa M. bieb as reductant and stabilizer for green synthesis of Au/Pd bimetallic nanoparticles as reusable catalysts in the Suzuki and Heck coupling reactions in water
Received
16th July 2014
, Accepted 1st September 2014
First published on 1st September 2014
Abstract
This work reports on the green synthesis of Au/Pd bimetallic nanoparticles by using Euphorbia condylocarpa M. bieb root extract and their application as separable catalysts for ligand-free Suzuki and Heck coupling reactions in water. The catalyst was characterized using powder XRD, SEM and EDS. This method has the advantages of high yields, simple methodology and easy work up. Catalytic efficiency remains unaltered even after several repeated cycles.
Introduction
The Suzuki and Heck coupling reaction provides a powerful and straightforward method for C–C bond formation, which has been widely applied to diverse areas such as natural product synthesis, pharmaceuticals, biologically active molecules and material science.1–3
Earlier reported methods for the Suzuki and Heck coupling reactions suffer from certain disadvantages such as the use of toxic, expensive and sensitive to air or moisture ligands and toxic organic solvents, the environmental pollution caused by utilization of homogeneous catalysts, low yields, long reaction times, tedious work-up, waste control and formation of side products.1–3
In recent years, organic reactions in water have received considerable attention. Water is cheap, non-toxic, non-combustible, non-explosive, and environmentally acceptable. The use of water instead of toxic organic solvents for organic processes is of interest.4
Among various palladium catalysts for the coupling reactions, homogeneous catalysts have been widely investigated, while less expensive heterogeneous catalysts received scanter attention.5 The problem with homogeneous catalysis is the difficulty to separate the catalyst from the reaction mixture and the impossibility to reuse it in consecutive reactions. Therefore, the need for ‘greener’ methods or chemical products that reduce or eliminate the use and generation of hazardous compounds is essential.
In recent years, metal nanoparticles have attracted a great attention as heterogeneous catalysts due to their interesting structure and high catalytic activities.6 The green synthesis techniques for the preparation of metal nanoparticles are generally synthetic routes that utilize relatively nontoxic solvents such as water, biological extracts, biological systems and microwave assisted synthesis.7 Green synthesis of NPs has several advantages over chemical synthesis, such as simplicity, cost effectiveness as well as compatibility for biomedical and pharmaceutical applications.
Few research studies are available about the green synthesis of Au/Pd bimetallic nanoparticles using plants.7d,e Thus, the potential of plants as biological materials for the synthesis of nanoparticles still should be explored.
The plants of the Euphorbiaceae contain acrid, milky, or colorless juice. Chemical data are available for several genera, especially Euphorbia, where more than 120 species have been investigated. The family Euphorbiaceae is rich in flavonoids, particularly flavones and flavonols, which have been identified from several genera. Furthermore, the previous studies in 1970 on Euphorbia condylocarpa demonstrated the presence of phytochemicals such as flavonoids, tetracyclic triterpenoids, and trifolin in different parts of the plant.8 The root of Euphorbia condylocarpa M. bieb has important applications in folk medicine for the treatment of the cancer, costiveness, and migraine and as an emollient. Recently our group investigated the optimized conditions for extraction of phenolics from the root of Euphorbia condylocarpa.8d
To date, there is no report on the green synthesis of Au/Pd bimetallic nanoparticles by utilizing the root extracts of Euphorbia condylocarpa M. bieb. In continuation of our recent works on the green chemistry and application of heterogeneous catalysts,9 we wish to report a new, rapid and green protocol for the preparation of water-dispersable Au/Pd bimetallic nanoparticles using Euphorbia condylocarpa M. bieb root extract (reducing and stabilizing agent) (Fig. 1) and its application as stable and heterogeneous catalysts in Suzuki and Heck coupling reactions in water (Scheme 1). The green synthesis of nanoparticles using Euphorbia condylocarpa M. bieb root extract provides a simple, cost effective and environment friendly route for the synthesis of nanomaterials without use of toxic organic solvents and hazardous and dangerous materials. Also, in this method there is no need to use high pressure, energy and temperature. Achievement of such rapid time scales for synthesis of metallic nanoparticles contributes to an increase in the efficiency of synthetic procedures using environmentally benign natural resources as an alternative to chemical synthesis protocols. More importantly, the synthesized Au/Pd bimetallic nanoparticles presented excellent catalytic activity, and could be easily separated from the reaction mixture and reused many times with no loss of activity, indicating a potential application in industry.
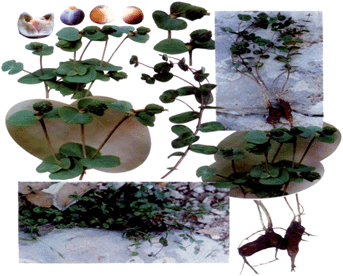 |
| Fig. 1 Image of Euphorbia condylocarpa M. bieb. | |
 |
| Scheme 1 Suzuki and Heck coupling reaction of different aryl iodines. | |
Result and discussion
The content of phenolics in the plant extracts at different times demonstrated a range of 2850 to 3650 GAE/dried weight in which the extract produced after 20 min boiling showed the maximum concentration of phenolics. Furthermore, the antioxidant potential of the E. condylocarpa aqueous extracts using FRAP (Ferric Reduction of Antioxidant Power) method demonstrated their reducing ability and made them suitable source for synthesis of metallic nanoparticles. Also, the extract obtained at the time 20 min showed the maximum antioxidant ability. Also, the phenolic content inside the extract after interaction and formation of nanoparticles was mainly decreased for interaction with salt solutions. Therefore, we can find that the content of phenolics inside the plant has a direct relation to the reducing ability and production of nanoparticles using this optimum plant aqueous extract (Scheme 2). The suggested mechanism for this biosynthesis is based on the reducing power of the plant metabolites and macroscopic change in color of the extract. The shift in the color from yellow to dark brown (as shown in Fig. 2) and also continuous Ultra violet signals at different times in constant wavelength are sign of a bio interaction and changing the properties of chromophores inside the molecules following the electronic transitions.
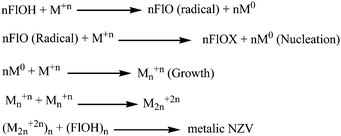 |
| Scheme 2 Reducing ability of antioxidants phenolics to produce metallic NPs where FIOH and NZV are flavoured and nano zero valent, respectively. | |
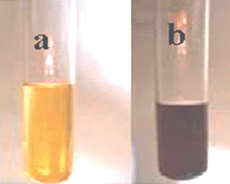 |
| Fig. 2 Photograph of Euphorbia condylocarpa M. bieb root extract (a) and green synthesized Au/Pd NPs produced after bio reaction (b). | |
In this paper, metal ions were reduced to nano zero valent (NZV) metallic particles using flavonoid (FlOH) contents of the plant as green (without a hazardous impact on the environment) chemicals (reducing agents) and an alternative to the commonly used hydride reducing agent, sodium borohydride. The use of the optimum plant extract for synthesis of nanoparticles represents some advantageous over other biological processes because it can be suitably scaled up for large-scale nanoparticles synthesis. In this study, Euphorbia condylocarpa M. bieb root extract has been used as a reducing and stabilizing agent for the synthesis of Au/Pd bimetallic nanoparticles. During the synthesis of Au/Pd bimetallic nanoparticles, we did not use any surfactants or synthetic polymer as synthetic stabilizing agents.
Fig. 3 shows the UV-vis spectra of Euphorbia condylocarpa M. bieb root extract. The UV spectrum with bonds at λnmmax 387 (bond 1) is due to the transition localized within the B ring of cinnamoyl system; whereas the peak depicted at 283 (bond 2) is consistent with absorbance of ring A of benzoyl system (Scheme 3). They are related to the π → π transitions and these absorbent bonds demonstrated the presence of flavon nuclei.
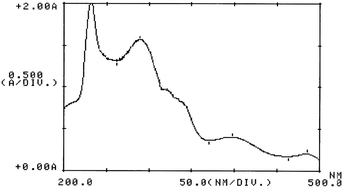 |
| Fig. 3 UV-vis spectrum of Euphorbia condylocarpa M. bieb root extract. | |
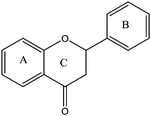 |
| Scheme 3 | |
The UV-vis spectra of Au/Pd bimetallic nanoparticles synthesis using the aqueous extract of Euphorbia condylocarpa at different times have been shown in Fig. 4. The surface plasmon resonance peak is centered at 542 nm and developed with increasing the time ranging 5 min to 45 min in which the appearance of the maxima in 45 min is demonstrating the end of the reaction.
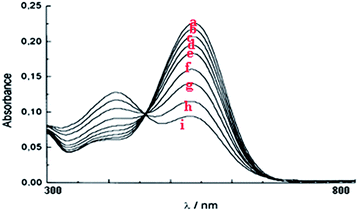 |
| Fig. 4 Synthesis of Au/Pd NPs using the aqueous extract of Euphorbia condylocarpa at different times. | |
The FT-IR analysis was carried out to identify the possible biomolecules responsible for the reduction of Au/Pd nanoparticles and capping of the bioreduced Au and Pd nanoparticles synthesized by the root extract (Fig. 5). The FT-IR spectrum depicted some peaks at 3436 to 3000, 1643, 1561, 1413 and 1277 cm−1 which represent free OH in molecule and OH group forming hydrogen bonds, carbonyl group (C
O), stretching C
C aromatic ring and C–OH stretching vibrations, respectively. These peaks suggested the presence of flavonoid and phenolics acids in the Euphorbia condylocarpa M. bieb root extract. The presence of flavonoid and phenolics acids in the extract could be responsible for the reduction of metal ions and formation of the corresponding metal nanoparticles. Flavonoid and phenolics acids could be adsorbed on the surface of metal nanoparticles, possibly by interaction through π-electrons interaction in the absence of other strong ligating agents.
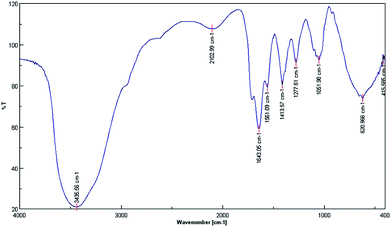 |
| Fig. 5 FT-IR spectrum of Euphorbia condylocarpa M. bieb root extract. | |
Au/Pd bimetallic nanoparticles were characterized using the powder XRD, SEM and EDS. Phase investigation of the crystallized product was performed by XRD and the powder diffraction pattern of Au/Pd bimetallic nanoparticles is presented in Fig. 6. The presence of gold and palladium was confirmed with powder XRD measurements.
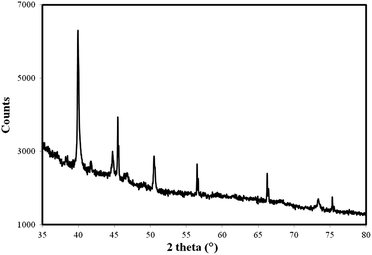 |
| Fig. 6 XRD powder pattern of Au/Pd bimetallic nanoparticles. | |
The morphology of the Au/Pd NPs was investigated by scanning electron microscopy (SEM) image displayed in Fig. 7. It is observed that the Pd particles are nanosized. Hence, Pd NPs have very narrow diameter distributions. Moreover, Au NPs appear over the surface of Pd NPs with average sizes of less than 80 nm.
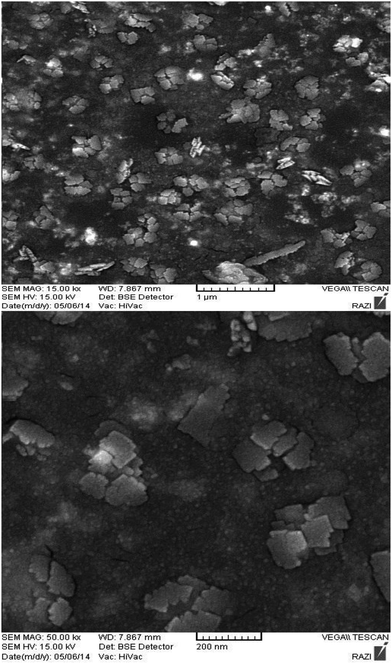 |
| Fig. 7 SEM images of Au/Pd bimetallic nanoparticles. | |
The elemental composition of the catalyst was also analyzed by the Energy Dispersive X-ray Spectroscopy (EDS) spectrum. It further confirmed that the Au/Pd bimetallic nanoparticles were composed of palladium, gold and oxygen (Fig. 8). EDS results show that Au, Pd and oxygen concentrations are about 2.94%, 10.89% and 86.17%, respectively. The excess oxygen is due to physical absorption of oxygen from environment during sample preparation.
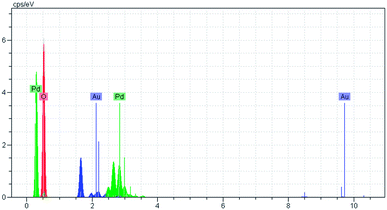 |
| Fig. 8 EDS spectrum of Au/Pd bimetallic nanoparticles. | |
Evaluation of the catalytic activity of Au/Pd bimetallic nanoparticles through the Suzuki coupling reaction
The catalytic behaviour of the Au/Pd bimetallic nanoparticles was studied for the Suzuki coupling reaction.
Initially, we employed iodobenzene and phenylboronic acid as model substrates for the development of optimized conditions. Several bases such as Cs2CO3, K2CO3, Et3N, Na2CO3 and NaOAc were examined. The results indicated that base had a demonstrative effect on the yield of product. Among the tested bases, K2CO3 was found to be superior for the highest yield of product (Table 1, entry 1). Control experiments show that there is no reaction in the absence of catalyst (Table 1, entry 6). However, addition of the catalyst to the mixture has rapidly increased the formation of product in high yields. A decrease in the catalyst loading from 0.2 to 0.1 mol% afforded the product in lower yield (Table 1, entry 8). No significant improvement on the yield was observed using higher amounts of the catalyst (Table 1, entry 7) and 0.2 mol% of the catalyst was found to be optimum. The best result was obtained with 1.0 mmol of aryl iodide, 1.1 mmol of phenylboronic acid, 0.4 mol% of catalyst, 2.0 mmol of K2CO3 and 20 mL of H2O at 80 °C, which gave the product in an excellent yield.
Table 1 Optimization of reaction conditions in Suzuki coupling reaction of iodobenzene with phenylboronic acida
Entry |
Au/Pd NPs (mol%) |
Base |
Yieldb (%) |
Reaction condition: 1.0 equiv. of aryl halide, 1.1 equiv. of phenylboronic acid, 0.2 mol% of catalyst and 2.0 equiv. of base, 80 °C, 24 h. Isolated yield. |
1 |
0.2 |
K2CO3 |
90 |
2 |
0.2 |
Cs2CO3 |
85 |
3 |
0.2 |
Na2CO3 |
76 |
4 |
0.2 |
NaOAc |
58 |
5 |
0.2 |
NEt3 |
37 |
6 |
0.0 |
K2CO3 |
0 |
7 |
0.4 |
K2CO3 |
91 |
8 |
0.1 |
K2CO3 |
69 |
Upon optimization of reaction conditions, the scope of the protocol was subsequently extended to a range of substituted aryl iodides and phenylboronic acids as substrates (Table 2). The electron-neutral, electron-rich and electron-poor aryl iodides reacted with phenylboronic acid very well to generate the corresponding coupling products in good to excellent yields under the standard reaction conditions.
Table 2 Suzuki coupling reaction of different aryl iodides with phenylboronic acidsa
Evaluation of the catalytic activity of Au/Pd bimetallic nanoparticles through the Heck coupling reaction
Due to the importance of Heck coupling reaction in synthetic organic chemistry for the manufacture of C–C bond, we next turned our attention to applying Au/Pd bimetallic nanoparticles to the Heck coupling reaction of organohalides with substituted styrenes. The desired products are obtained in good yields.
We initially selected iodobenzene and styrene as a model reaction. As expected, no target product could be detected in the absence of catalyst. Among the various bases (Cs2CO3, K2CO3, Et3N, Na2CO3 and NaOAc) tested in the presence of Au/Pd bimetallic nanoparticles in H2O, K2CO3 led to significant conversion. The best result was obtained with iodobenzene (1.0 mmol), styrene (1.5 mmol), Au/Pd bimetallic nanoparticles (0.2 mol%), K2CO3 (2.0 mmol), H2O (7.0 mL) and CTAB (cetyltrimethylammonium bromide) as an additive (0.5 mmol) at 80 °C, which obtained the product in a good yield (94%). In this study, CTAB was added for helping reactions in water. CTAB was introduced as an additive for enhancing the reactivity.10 CTAB as the enhancing agent is described in the literature such that only cationic surfactant has enhancing effect by chelating to the phosphine free organometallic Pd sources.10 We have examined the coupling reaction of substituted styrenes with various aryl iodides containing electron-withdrawing or electron-donating groups. As shown in Table 3, substituted styrenes were converted into the corresponding products in high yields under ligand-free and aerobic conditions.
Table 3 Heck coupling reaction of different aryl iodides with styrene derivativesa
Compared with the other literature works on the Suzuki and Heck coupling reactions, the notable features of our method are:
• The reaction system is simple;
• Elimination of toxic reagents and homogeneous catalysts;
• The yields of the products are high;
• The use of plant extract as an economic and effective alternative represents an interesting, fast and clean synthetic route for the large scale synthesis of Au/Pd bimetallic nanoparticles;
• The Au/Pd NPs catalyst can be easily recovered;
• According to the UV-vis results, the synthesized Au/Pd NPs by this method are quite stable and can be kept under inert atmosphere for several months.
Catalyst recyclability
The work-up procedure is straightforward due to the heterogeneous nature of the catalyst. To evaluate the reusability of Au/Pd bimetallic nanoparticles, the catalyst after completion of the reaction was separated and was washed with ethanol, dried and the recycled catalyst was saved for the next reaction. The recycled catalyst could be reused four times with no loss of activity (Table 2). Thus, the nanocatalyst is stable during the Suzuki coupling reaction. The reusability of the catalyst was also studied for the Heck coupling reaction under the present reaction conditions. The catalytic activity did not decrease considerably after four catalytic cycles (Fig. 9).
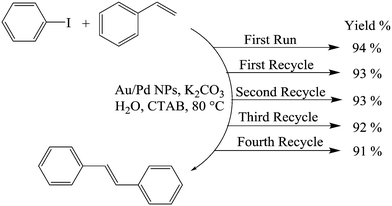 |
| Fig. 9 Reusability of Au/Pd NPs for Heck coupling reaction. | |
Conclusion
In summary, we have developed a ‘green’ strategy for the one-step room temperature synthesis of Au/Pd bimetallic nanoparticles, in which Euphorbia condylocarpa M. bieb root extract acts as the reductant as well as the stabilizer. The formation of Au/Pd bimetallic nanoparticles was possibly facilitated reduction of metal ions by flavonoid and phenolics acids present in the Euphorbia condylocarpa M. bieb root extract. Compared with other synthetic methods, the distinct advantage of our method is that the Au/Pd bimetallic NPs can be synthesized by one-step reduction. The formation of Au/Pd nanoparticles with flavonoid and phenolics acids takes place via the following steps: (1) complexation with Au and Pd metal salts, (2) simultaneous reduction of Au and Pd metal, and (3) capping with oxidized polyphenols/caffeine. Our method was clean, nontoxic and environment friendly. Also, we have established that the ligand-free heterogeneous catalyst [Au/Pd] is a highly efficient, stable and recyclable catalyst for the Suzuki and Heck coupling reactions. The present method has the advantages of readily available starting materials, straightforward and simple work-up procedures, elimination of ligand, high yields, tolerance for a wide variety of functionality, and excellent reusability of the catalyst.
Experimental
High-purity chemical reagents were purchased from the Merck and Aldrich chemical companies. All materials were of commercial reagent grade. Melting points were determined in open capillaries using a BUCHI 510 melting point apparatus and are uncorrected. 1H NMR and 13C NMR spectra were recorded on a Bruker Avance DRX-400 spectrometer at 400 and 100 MHz, respectively. FT-IR spectra were recorded on a Nicolet 370 FT/IR spectrometer (Thermo Nicolet, USA) using pressed KBr pellets. The element analyses (C, H, N) were obtained from a Carlo ERBA Model EA 1108 analyzer carried out on Perkin-Elmer 240c analyzer. X-ray diffraction (XRD) measurements were carried out using a Philips powder diffractometer type PW 1373 goniometer (Cu Kα = 1.5406 Å). The scanning rate was 2° min−1 in the 2θ range from 10 to 80°. Scanning electron microscopy (SEM) was performed on a Cam scan MV2300. EDS (S3700N) was utilized for chemical analysis of prepared nanostructures.
Preparation of Euphorbia condylocarpa M. bieb root extract
50 g of dried powder of E. condylocarpa M. bieb was extracted using boiling in 300 mL double distillated water for 10, 15, 20 and 25 min and aqueous extract was centrifuged in 7000 rpm to obtain the supernatant as extract.
Total phenolic content of the E. condylocarpa M. bieb
The amount of total phenolics in obtained aqueous extracts before and after interaction to obtain Au/Pd NPs at times ranging 10, 15, 20 and 25 min was determined with Folin–Ciocalteu reagent according to the method with slight modification using gallic acid as a standard.11a Briefly, 1.0 mL of sample solution was added in a 100 mL volumetric flask that contained about 60 mL distilled water. Then, 5.0 mL of Folin–Ciocalteu reagent was added and the content of the flask was mixed completely. After 5 min, 15.0 mL Na2CO3 (20%) was added and the volume was made up to 100 mL using distilled water. The mixture was allowed to stand for 2 h with shaking. The absorbance was measured at 760 nm. The total phenolic content was determined as mg of gallic acid equivalent (GAE) using an equation obtained from the standard gallic acid calibration graph.
Total antioxidant ability
The amount of antioxidant capacity was determined using FRAP assay, which depends upon the reduction of ferric tripyridyltriazine (Fe(III)–TPTZ) complex to the ferrous tripyridyltriazine (Fe(II)–TPTZ) by a reductant at low pH. (Fe(II)–TPTZ) has an intensive blue colour and can be monitored at 593 nm.11b Briefly, the stock solutions included 300 mM acetate buffer (3.1 g C2H3NaO2·3H2O and 16 mL C2H4O2), pH 3.6, 10 mM TPTZ (2,4,6-tripyridyl-s-triazine) solution in 40 mM HCl, and 20 mM FeCl3·6H2O solution. The fresh working solution was prepared freshly by mixing 25 mL acetate buffer, 2.5 mL TPTZ solution, and 2.5 mL FeCl3·6H2O solution and then warmed at 37 °C before using. Aqueous solution of known Fe(II) concentration as standard solution was used for calibration (in a range of 0.1 to 1 mM). Assay: blank FRAP reagent, sample: 1.5 mL FRAP reagent and 50 μL aqueous extracts obtained at different times. The reaction was monitored up to 4 min at 593 nm, at 37 °C. The Fe(II) standard solution was tested in a parallel process. Calibrations were made by a calibration curve. The standard curve was linear between 1 to 10 mmol also additional dilution was needed if the FRAP value was over the linear range of the standard curve. The results are expressed in mmol Fe2+ per mg sample.
Green synthesis of Au/Pd bimetallic nanoparticles using Euphorbia condylocarpa M. bieb root extract
In a typical synthesis of Au/Pd NPs, 20 mL of the above extract was added dropwise to 40 mL of a well mixed solution of HAuCl4 (0.2 mM)/PdCl2 (1 mM) with constant stirring at 50 °C for 2 hours. After 20 min the color of the solution changed from yellow to dark brown due to excitation of surface plasmon resonance which indicates the formation of Au/Pd NPs. Reduction of bimetallic mixture was completed around 45 minutes, (Fig. 4). Then the colored solution of bimetallic nanoparticles was centrifuged at 7000 rpm for 25 min to completely precipitation of Au/Pd NPs. The obtained precipitation was then washed three times with chloroform and ethanol, respectively.
General procedure for Suzuki coupling reaction
Under nitrogen atmosphere, an oven-dried round-bottomed flask was charged with catalyst (0.2 mol%), aryl iodide (1.0 mmol), K2CO3 (2.0 mmol), phenylboronic acid (1.1 mmol) and water (20 mL). The reaction mixture was stirred at 80 °C for 24 h. After completion of the reaction, the reaction mixture was cooled to room temperature and the catalyst was separated. The resulting reaction mixture was extracted with ethyl acetate. The organic phase was separated, evaporated under reduced pressure and the residue was purified by flash chromatography on silica gel to give the desired coupling product. All the products are known compounds and the spectral data and melting points were identical to those reported in the literature.12
Biphenyl (Table 2, entry 1). 1H NMR (400 MHz, CDCl3): δH 7.62 (d, J = 8.1 Hz, 4H), 7.48 (t, J = 7.7 Hz, 4H), 7.38 (t, J = 7.7 Hz, 2H).
4-Methylbiphenyl (Table 2, entries 2 and 7). 1H NMR (400 MHz, CDCl3): δH 7.59 (t, J = 7.7 Hz, 2H), 7.50 (d, J = 8.0 Hz, 2H), 7.45–7.39 (m, 2H), 7.34 (t, J = 7.7 Hz, 1H), 7.26 (t, J = 8.0 Hz, 2H), 2.39 (s, 3H).
1,1′-Biphenyl-4-carbonitrile (Table 2, entries 3 and 8). 1H NMR (400 MHz, CDCl3): δH 7.75–7.66 (m, 4H), 7.60 (d, J = 8.1 Hz, 2H), 7.51–7.40 (m, 3H).
3-Nitrobiphenyl (Table 2, entry 4). 1H NMR (400 MHz, CDCl3): δH 8.47 (s, 1H), 8.23–8.19 (m, 1H), 7.94 (d, J = 8.1 Hz, 1H), 7.66–7.61 (m, 3H), 7.55–7.50 (m, 2H), 7.47–7.43 (m, 1H).
4-Nitrobiphenyl (Table 2, entry 5). 1H NMR (400 MHz, CDCl3): δH 8.32 (d, J = 8.6 Hz, 2H), 7.76 (d, J = 8.6 Hz, 2H), 7.65 (t, J = 8.7 Hz, 2H), 7.52–7.42 (m, 3H).
4-Fluorobiphenyl (Table 2, entry 6). 1H NMR (400 MHz, CDCl3): δH 7.53–7.47 (m, 4H), 7.39 (t, J = 7.7 Hz, 2H), 7.30 (t, J = 7.4 Hz, 1H), 7.10 (t, J = 8.7 Hz, 2H).
Biphenyl-4-carboxaldehyde (Table 2, entry 9). 1H NMR (400 MHz, CDCl3): δH 10.2 (s, 1H), 7.98 (d, J = 8.8 Hz, 2H), 7.78 (d, J = 8.8 Hz, 2H), 7.66 (d, J = 8.8 Hz, 2H), 7.56–7.35 (m, 3H).
4-Acetylbiphenyl (Table 2, entry 10). 1H NMR (400 MHz, CDCl3): δH 8.05 (d, J = 8.0 Hz, 2H), 7.70 (d, J = 8.0 Hz, 2H), 7.65 (d, J = 8.0 Hz, 2H), 7.50–7.37 (m, 3H), 2.64 (s, 3H).
General procedure for Heck coupling reaction
To a stirred solution of 1.0 mmol of aryl iodide in 7 mL of H2O, 1.5 mmol of styrene, 2.0 mmol of K2CO3 and 0.2 mol% of Au/Pd bimetallic nanoparticles were added and the mixture was heated on an oil bath at 80 °C for 8 h under inert atmosphere (N2). After completion of the reaction (as monitored by TLC), the reaction mixture was cooled to room temperature, the catalyst was separated and the product was extracted with EtOAc. The organic phase was separated, dried over anhydrous MgSO4, and evaporated under reduced pressure, and the residue was subjected to gel permeation chromatography to afford pure products. All the products are known compounds and the spectral data and melting points were identical to those reported in the literature.13
(E)-1,2-Diphenylethene (Table 3, entry 1). 1H NMR (400 MHz, DMSO-d6): δH 7.71–7.12 (m, 10H), 7.10 (s, 2H).
(E)-1-(4-Chlorophenyl)-2-phenylethene (Table 3, entry 2). 1H NMR (400 MHz, DMSO-d6): δH 7.48–7.25 (m, 9H), 7.10 (s, 2H).
(E)-1-(4-Methylphenyl)-2-phenylethene (Table 3, entry 3). 1H NMR (400 MHz, DMSO-d6): δH 7.66–7.02 (m, 9H), 7.01 (s, 2H), 2.30 (s, 3H).
(E)-1-(4-Nitrophenyl)-2-phenylethene (Table 3, entry 4). 1H NMR (400 MHz, DMSO-d6): δH 8.45–8.10 (m, 2H), 7.82–7.21 (m, 7H), 7.19 (s, 2H).
(E)-1-(3-Nitrophenyl)-2-phenylethene (Table 3, entry 5). 1H NMR (400 MHz, DMSO-d6): δH 8.39 (s, 1H), 8.11 (d, J = 8.3 Hz, 1H), 7.82 (d, J = 8.1 Hz, 1H), 7.57–7.26 (m, 6H), 7.22 (s, 1H), 7.17 (s, 1H).
Acknowledgements
We gratefully acknowledge the Iranian Nano Council and the University of Qom for the support of this work.
References
- A. Fihri, M. Bouhrara, B. Nekoueishahraki and J. M. Basset, Chem. Soc. Rev., 2011, 40, 5181 RSC.
- I. Favier, D. Madec, E. Teuma and M. Gomez, Curr. Org. Chem., 2011, 15, 3127 CrossRef CAS.
-
(a) R. Chinchilla and C. Nájera, Chem. Rev., 2007, 107, 874 CrossRef CAS PubMed;
(b) R. H. Heck, Palladium Reagents for Organic Synthesis, Academic Press, New York, 1985 Search PubMed;
(c) J. Hassan, M. Sévignon, C. Gozzi, E. Schulz and M. Lemaire, Chem. Rev., 2002, 102, 1359 CrossRef CAS PubMed.
-
(a) M. Nasrollahzadeh, A. Ehsani and A. Rostami-Vartouni, Ultrason. Sonochem., 2014, 21, 275 CrossRef CAS PubMed;
(b) M. Nasrollahzadeh, A. Ehsani and M. Maham, Synlett, 2014, 25, 505 CrossRef PubMed.
- J. Michalik, M. Narayana and L. Kevan, J. Phys. Chem., 1985, 89, 4553 CrossRef CAS.
- S. Wei, Z. Dong, Z. Ma, J. Sun and J. Ma, Catal. Commun., 2013, 30, 40 CrossRef CAS PubMed.
-
(a) E. S. Abdel-Halim, M. H. El-Rafie and S. S. Al-Deyab, Carbohydr. Polym., 2011, 85, 692 CrossRef CAS PubMed;
(b) A. R. Jasbi, Phytochemistry, 2006, 67, 1977 CrossRef PubMed;
(c) S. P. Dubey, M. Lahtinen and M. Sillanpa, Process Biochem., 2010, 45, 1065 CrossRef CAS PubMed;
(d) G. Zhan, J. Huang, M. Du, I. Abdul-Rauf, Y. Ma and Q. Li, Mater. Lett., 2011, 65, 2989 CrossRef CAS PubMed;
(e) X. Huang, H. Wu, S. Pu, W. Zhang, X. Liao and B. Shi, Green Chem., 2011, 13, 950 RSC.
-
(a) A. Rizk, Bot. J. Linn. Soc., 1987, 94, 293 CrossRef PubMed;
(b) A. R. Jasbi, Phytochemistry, 2006, 67, 1977 CrossRef PubMed;
(c) Y. V. Roshchin and N. P. Kir'yalov, Chem. Nat. Compd., 1970, 6(4), 501 CrossRef;
(d) M. Nasrollahzadeh, S. M. Sajadi, M. Maham, P. Salaryan, A. Enayati, S. A. Sajjadi and K. Naderi, Chem. Nat. Compd., 2011, 47, 434 CrossRef CAS;
(e) M. Nasrollahzadeh, F. Babaei, S. M. Sajadi and A. Ehsani, Spectrochim. Acta, Part A, 2014, 132, 423 CrossRef CAS PubMed.
-
(a) M. Nasrollahzadeh, M. Maham, A. Ehsani and M. Khalaj, RSC Adv., 2014, 4, 19731 RSC;
(b) M. Nasrollahzadeh, A. Rostami-Vartouni, A. Ehsani and M. Moghadam, J. Mol. Catal. A: Chem., 2014, 387, 123 CrossRef CAS PubMed;
(c) M. Nasrollahzadeh, A. Zahraei, A. Ehsani and M. Khalaj, RSC Adv., 2014, 4, 20351 RSC;
(d) D. Habibi, M. Nasrollahzadeh, L. Mehrabi and S. Mostafaee, Monatsh. Chem., 2013, 144, 725 CrossRef CAS PubMed;
(e) M. Nasrollahzadeh, A. Azarian, A. Ehsani and A. Zahraei, Tetrahedron Lett., 2014, 55, 2813 CrossRef CAS PubMed;
(f) D. Habibi, S. Heydari and M. Nasrollahzadeh, J. Chem. Res., 2012, 36, 573 CrossRef CAS;
(g) P. Fakhri, B. Jaleh and M. Nasrollahzadeh, J. Mol. Catal. A: Chem., 2014, 383–384, 17 CrossRef CAS PubMed;
(h) M. Nasrollahzadeh, RSC Adv., 2014, 4, 29089 RSC;
(i) M. Nasrollahzadeh, M. Enayati and M. Khalaj, RSC Adv., 2014, 4, 26264 RSC;
(j) M. Nasrollahzadeh, A. Azarian, A. Ehsani and M. Khalaj, J. Mol. Catal. A: Chem., 2014, 394, 205 CrossRef CAS PubMed;
(k) M. Nasrollahzadeh, M. Maham and M. M. Tohidi, J. Mol. Catal. A: Chem., 2014, 391, 83 CrossRef CAS PubMed.
- S. Bhattacharya, A. Srivastava and S. Sengupta, Tetrahedron Lett., 2005, 46, 3557 CrossRef CAS PubMed.
-
(a) V. L. Singleton and J. A. Rossi, Am. Chem. Soc. Symp. Ser., 1965, 26, 47 CrossRef PubMed;
(b) I. F. F. Benzie and J. J. Strain, Anal. Biochem., 1996, 239, 70 CrossRef CAS PubMed.
-
(a) P. K. Mandali and D. K. Chand, Catal. Commun., 2013, 31, 16 CrossRef CAS PubMed;
(b) T. S. A. Heugebaert, S. De Corte, T. Sabbe, T. Hennebel, W. Verstraete, N. Boon and C. V. Stevens, Tetrahedron Lett., 2012, 53, 1410 CrossRef CAS PubMed;
(c) Y.-Y. Peng, J. Liu, X. Lei and Z. Yin, Green Chem., 2010, 12, 1072 RSC.
-
(a) H. Li, Z. Zhu, H. Li, P. Li and X. Zhou, J. Colloid Interface Sci., 2010, 349, 613 CrossRef CAS PubMed;
(b) L. Wan and C. Cai, Transition Met. Chem., 2011, 36, 747 CrossRef CAS PubMed.
|
This journal is © The Royal Society of Chemistry 2014 |