DOI:
10.1039/D3TB02912C
(Review Article)
J. Mater. Chem. B, 2024,
12, 7977-8006
Innovative approaches to wound healing: insights into interactive dressings and future directions
Received
10th December 2023
, Accepted 29th May 2024
First published on 6th June 2024
Abstract
The objective of this review is to provide an up-to-date and all-encompassing account of the recent advancements in the domain of interactive wound dressings. Considering the gap between the achieved and desired clinical outcomes with currently available or under-study wound healing therapies, newer more specific options based on the wound type and healing phase are reviewed. Starting from the comprehensive description of the wound healing process, a detailed classification of wound dressings is presented. Subsequently, we present an elaborate and significant discussion describing interactive (unconventional) wound dressings. Latter includes biopolymer-based, bioactive-containing and biosensor-based smart dressings, which are discussed in separate sections together with their applications and limitations. Moreover, recent (2–5 years) clinical trials, patents on unconventional dressings, marketed products, and other information on advanced wound care designs and techniques are discussed. Subsequently, the future research direction is highlighted, describing peptides, proteins, and human amniotic membranes as potential wound dressings. Finally, we conclude that this field needs further development and offers scope for integrating information on the healing process with newer technologies.
Introduction
Wound assessment has been a persistent therapeutic challenge for the medical community since ancient times. Wounds are usually associated with increased morbidity and significant mortality.1 In 2022, the World Health Organization (WHO) reported that about 4.4 million people worldwide lose their lives annually due to injuries, both unintentional and caused by violence. Collectively, this accounts for almost 8% of all mortalities.2 These data are noteworthy compared to cancer deaths (≈10 million deaths globally as of 2020), one of the leading cause of death worldwide.3 A wound can be defined as any injury to the epithelial lining of the skin that is caused by physical or thermal damage. This type of injury can lead to various mental health issues, including anxiety, depression, or stress, which may impose a considerable effect on the balanced lifestyle and well-being of an individual.4
Wound healing is an intricate and dynamic physiological process that involves numerous cellular events to rejuvenate damaged tissues.5 It relies on the interaction among extracellular matrix (ECM) molecules, mediators, fibroblasts, keratinocytes, and invading leukocyte subtypes within the human body.6 These components work together in a coordinated manner to restore the integrity of injured tissue and regenerate what has been lost. The process of wound healing can be divided into four sequential stages: hemostasis, inflammation, proliferation, and maturation.7Table 1 provides a detailed overview of the different phases of wound healing, including the duration of each phase, the specific events that occur during each phase to prepare the tissue for healing, and visual illustrations depicting the wound in each phase. Each stage of wound healing is interconnected and interdependent. In this case, a disruption or imbalance in any stage can lead to impaired healing or the formation of chronic wounds.
Table 1 Phases of wound healing
Phase |
Events |
Pictorial illustration |
Importance |
The time frames for each stage mentioned in this table are approximate and can vary depending on the size of wound, location, and individual factors.
|
Hemostasis |
Vasoconstriction: reduced blood flow and prevention of bleeding |
|
This stage begins immediately after tissue injury and aims to stop bleeding and promote blood clot formation. Platelets and clotting factors come into action, forming a clot that seals the wound and creates a provisional matrix. This initial matrix acts as a scaffold for the subsequent stages of healing. |
Time spana: 5–10 minutes8 |
Primary hemostasis: platelet aggregation |
|
|
Secondary hemostasis: formation of fibrin resulting in a strong blood clot.6,9 |
|
Inflammatory |
Swelling, redness and pain. |
|
Following hemostasis, the inflammation stage begins. Although inflammation is often associated with negative connotations, it is an essential and carefully regulated process in wound healing. Inflammation serves multiple purposes, including removing debris, clearing pathogens, and initiating the recruitment of various cell types necessary for tissue repair. Inflammatory cells such as neutrophils and macrophages play a critical role in this stage, releasing growth factors and cytokines that stimulate subsequent phases of healing. |
Time span: 0–4 days10 |
Chemotactic movement of neutrophils. |
|
Macrophages engulf bacteria, apoptotic neutrophils and necrotic tissues.11 |
Proliferative (reconstruction/repair phase) |
Stimulation of angiogenesis by macrophages. |
|
During this stage, new tissue formation occurs, primarily driven by the activity of fibroblasts and keratinocytes. Fibroblasts produce collagen, the main component of the ECM, which provides strength and support for wound healing. Alternatively, keratinocytes contribute to re-epithelialization, where they migrate and proliferate to cover the wound surface. |
Time span: 3–24 days10 |
Characterised by migration and proliferation of fibroblasts. |
|
Fibroblasts (produce collagen) are stimulated by macrophages and differentiate into myofibroblasts (important in wound contraction process).12 |
Remodelling/maturation |
Indicated by wound contraction and collagen remodelling. |
|
The final stage of wound healing is maturation. In this phase, the newly formed tissue undergoes remodelling and maturation to attain its optimal strength and functionality. Collagen fibers reorganize and align along the lines of tension, making the healed tissue more resistant to stress. Excessive scar tissue formation is minimized through a balance of collagen synthesis and degradation. |
Time span: 24 days–2 years8 |
Increase in the wound tensile strength (cannot regain the full strength of original tissue). |
|
Formation of a scar.9,12 |
Wound dressings play a crucial role in the healing process by preventing the wound from becoming worse or getting infected, as well as facilitating the development of granulation tissue at the wound site.13 They not only provide protection but also promote natural healing.14 With the aim of treating various types of wounds, numerous dressings have been developed and used in clinics. The use of wound dressings offers diverse benefits, including superficial protection of the wound surface, maintenance of a moist wound healing environment, appropriate temperature regulation, removal of excess fluid, and alleviation of discomfort during dressing changes.15 To ensure effective treatment, wound dressings must consider various wound-related factors, such as the type and depth of the wound and the amount of exudate.16 However, if a dressing fails to sufficiently soak the exudate, it can cause maceration, increasing the chances of infection. Alternatively, dressings with high absorbing capacity may disrupt the tissue moisture balance and cause irritation.17 Therefore, improper wound treatment and unsuitable dressing choices can create a barrier to effective wound healing.13
Passive conventional dressings, such as bandages and gauzes, are essential wound care products primarily used for drying and cleaning wounds to control bleeding and shield against external contaminants.18 However, these dressings have significant limitations and issues when applied to highly exudating chronic wounds, which can lead to discomfort and pain during dressing removal. Therefore, these dressings are more appropriate for dry wounds.19 Furthermore, these dressings are unsuitable for wounds with excessive exudate given that they quickly become saturated, risking leakage and infection.20 Additionally, they may adhere to the wound surface, potentially causing harm during removal, and disrupting the healing process.21 Moreover, their limited breathability, incompatibility with some topical treatments, and the risk of secondary trauma when changed frequently highlight their limitations.22 Consequently, healthcare professionals are now exploring advanced wound care solutions tailored to specific wound types and conditions to effectively mitigate these challenges and optimize the healing process.23
Interactive or modern wound dressings represent a significant advancement in wound care, offering a tailored and advanced approach to treatment compared to traditional passive dressings.24 Unlike passive dressings, interactive dressings actively engage with the wound environment.25 Typically, they are composed of specialized materials, such as hydrogels, foams, and silicone, which contribute to the healing process. Interactive dressings demonstrate proficiency in maintaining an optimal moisture balance within the wound, preventing excessive dryness and excessive moisture.26 Their ability to promote the formation of granulation tissue is a key feature, providing a supportive scaffold for the growth of blood vessels and epithelial cells, ultimately leading to wound closure.27 Moreover, interactive dressings effectively manage exudate, preventing saturation and leakage, reducing the need for frequent dressing changes, and minimizing the risk of infection.28 Their transparency facilitates wound monitoring without disturbing the wound bed, and they adhere gently to the wound surface, causing minimal pain and trauma during removal.26 These attributes make interactive dressings a superior choice in wound care, particularly for highly exudating chronic wounds, complex cases, and situations where optimizing the healing process is paramount.
This review is dedicated to exploring the recent innovations in interactive wound dressings, as well as examining patents and providing an update on their clinical status. Furthermore, we analyze the effectiveness of dressings, evaluate treatment advantages and limitations, and highlight promising new materials for future wound care applications. To exemplify the transformative potential of advanced interactive dressings in wound care, two notable examples stand out. Firstly, 3D patches of manuka honey-gelatin possess potent antibacterial properties effective against both Gram-negative and Gram-positive bacteria, thereby addressing common wound infections, while concurrently promoting angiogenesis and the production of skin cells.29 Additionally, there is a smart and flexible wound bandage equipped with sensors for detecting uric acid (UA), pH, and temperature at the wound site. This innovative bandage has shown exceptional clinical performance, delivering precision and stability in the monitoring of UA, pH, and temperature levels, both in controlled environments and real-world scenarios.30 These examples emphasize the promising future of interactive dressings in revolutionizing wound care by integrating sensors and drug delivery capabilities. These innovations enable personalized therapy and real-time monitoring, underlining the critical importance of ongoing research to unlock the full therapeutic potential of wound healing dressings, ultimately benefiting both patients and healthcare providers.
Wound management and dressing selection
The term “wound management” refers to all facets of patient care related to wounds, including initial injury assessment, infection control, fluid loss management, tissue regeneration, wound closure, scar development and remodelling.31 There are several wound-care items on the market, such as simple protecting layers, hydrogels, metal ion-impregnated dressings, and antimicrobial dressings, which aid in wound healing by promoting the closure of the wound surface.32 The choice of wound care product is influenced by several factors, including the wound characteristics such as type of wound, its colour, depth, shape, size, amount of exudate, location, and the care setting. Given that acute and chronic wounds differ significantly in terms of their etiology, different approaches to wound care management need to be adopted.12 With a wide range of products available on the market, choosing the right dressing can be challenging. The choice of wound care product will often change at each stage of wound healing given that wounds are dynamic and go through distinct phases of healing, as depicted in Fig. 1.33 For instance, possible dressing options to treat wounds in the hemostasis phase of wound healing involve hydrocolloids and transparent films, and their commercially available products include Comfeel®, Tegasorb®, Oxyband®, Bioclusive®, and Polyskin II.32 Further, factors such as ease of use, cost-effectiveness, and patient compliance are also considered to identify the most suitable product.34
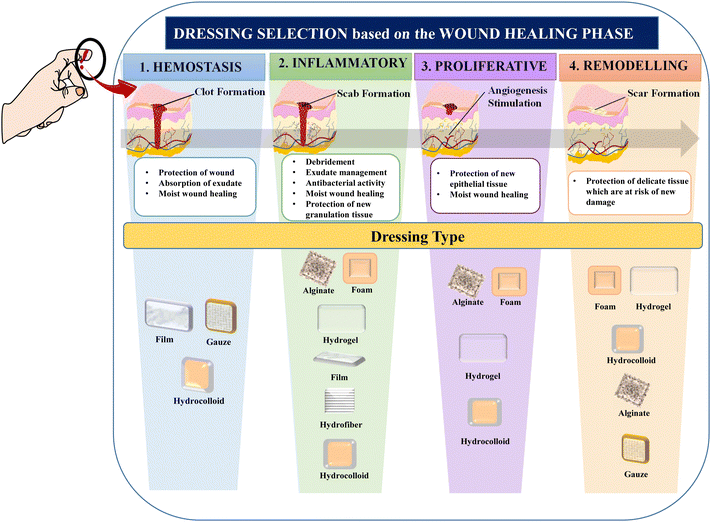 |
| Fig. 1 Dressing selection based on wound healing phase. | |
Dressings can be broadly classified into two categories, traditional and modern dressings, considering the advanced innovations in manufacturing techniques and associated properties.25,35 However, dressings can also be categorised based on factors such as clinical performance, material origin and type,36 functions and interaction with the wound surface,37 physical forms,38 and adaptability to changing wound conditions.14,39 Other classification criteria include skin replacement products, wound healing devices, and traditional, contemporary, and advanced dressings.7 The various classes of wound dressings based on these parameters are summarised in Fig. 2.
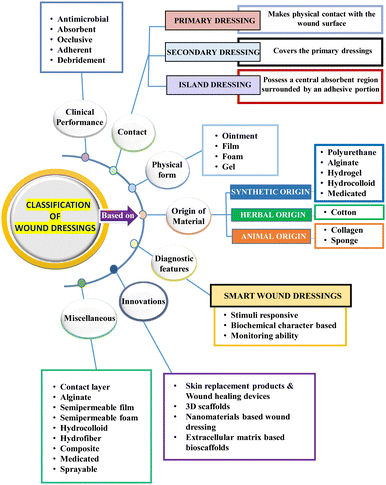 |
| Fig. 2 Summary of the classification of wound dressings based on different parameters. | |
Interactive wound dressings
Interactive wound dressings are dressings that actively interact with the wound surface to enhance the healing process and water vapor transmission rate (WVTR).40 These dressings include unconventional dressings such as biopolymer-based dressings, bioactive-supplemented dressings, and smart dressings41 (Fig. 3). Biopolymer-based dressings, such as films, foams, hydrogels, and hydrocolloids, serve as a barrier against microorganisms entering the wound environment.4 Bioactive dressings may be loaded with bioactive agents, exhibiting biodegradability and biocompatibility, while actively interacting with the wound surroundings.42,43 The commonly used bioactive agents include vitamins, antibiotics, and growth factors, which aid in the wound healing process.44 Smart dressings, a newer concept in wound healing, utilize technological advancements and biomaterials embedded with sensors to sense and monitor the healing process in real-time, providing an optimal healing environment.7,14
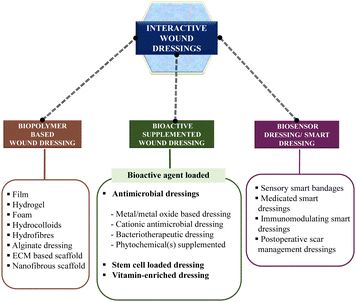 |
| Fig. 3 Classification of interactive wound dressings in this review. | |
Biopolymer-based dressings
Biopolymers or biomaterials are widely used in the design of wound dressings due to their high biocompatibility, biodegradability, bioactivity, and non-toxicity.45 A biomaterial is defined as any combination of synthetic or naturally derived polymers, excluding drugs, which can be utilized as a whole or as part of a system to treat, augment, or replace any tissue, organ, or physiological process within the body (Fig. 4).46,47 A wide range of biomaterials is available to meet the challenges of customization and provide specialised solutions for tissue regeneration in various circumstances, such as wound healing.48,49 Biomaterials for wound healing can be categorised depending on their source, chemical composition, type of dressing, and application.18,46 Natural biomaterials, such as collagen, alginate, chitosan, and silk, have gained prominence due to their inherent biocompatibility and their ability to promote tissue regeneration and maintain a favourable wound environment.50 They naturally degrade, aligning with the wound healing process, which eliminates the need for removal and reduces the risk of additional harm.51 Furthermore, natural biomaterials may have antimicrobial properties and help maintain a moist wound environment, which are critical factors in optimizing the healing process.52 In contrast, synthetic biomaterials such as polycaprolactone, while offering versatility and ease of manufacture, often necessitate removal procedures, which can pose a risk of additional wound damage.53 This inherent difference underscores the preference for natural biomaterials in many wound dressing applications, given that they contribute to a more seamless and minimally invasive healing process.54,55
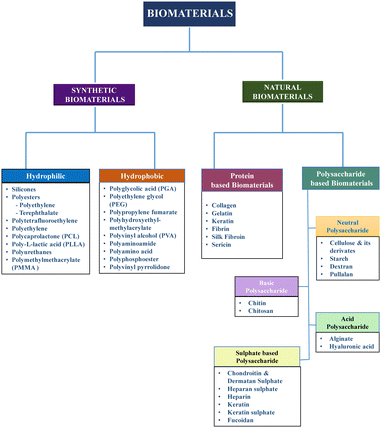 |
| Fig. 4 Classification of biomaterials. | |
In each phase of wound healing, several growth factors and cytokines play a role in advancing the wound to the next healing stage. These biological factors are responsible for promoting cell proliferation, angiogenesis, collagen synthesis, and other processes essential for tissue regeneration.55 However, the hostile environment inside a non-healing wound frequently causes the disappearance or degradation of essential growth factors and cytokines.56 Therefore, the administration of growth factors and cytokines to wounds via biomaterials has been studied for regenerative purposes.57 Furthermore, the effectiveness of polymeric biomaterials can be enhanced by incorporating medicinal compounds, growth factors, and antimicrobial medications, given that they can expedite wound closure by inducing collagen deposition and vascularization.58 Different wound dressings have been developed using these biomaterials, which are elaborated in the following sections.
Film dressings
Film dressings are thin, transparent, flexible, and semi-permeable sheets used in wound care to protect the wound from external contaminants while allowing moisture vapor transmission and gas exchange.59 One side of these sheets is coated with adhesive, allowing them to securely cling to the wound surface.60 Film dressings are commonly made from various polymers, with polyurethane emerging as a widely favoured option.61 Other polymers such as silicone and hydrocolloid are also utilized in the manufacturing of film dressings.62 The specific formulation may vary depending on the brand and type of film dressing. In the study by Mistry et al. on diabetic wound healing, it was found that chitosan–polygalacturonic acid polyelectrolyte complex films significantly accelerated wound closure and promoted hair growth in diabetic mice.63 These dressings offer convenience in wound management due to their unique characteristics. For example, their elasticity allows them to fit any body contour, and their thin and flexible nature makes them easy to apply, even in difficult-to-reach areas such as joints.64 Films can be utilised for direct medicine administration to the wound site given that they are permeable to gas and impermeable to liquid and germs. They allow some moisture to evaporate, act as a barrier against external contamination, and support wound bed inspection without removing the dressing.60 These dressings are utilized mainly for wounds that do not exude heavily.9 The composition and thickness of the film affect its mean vapour transmission rate (MVTR), which determines how much moisture can pass through it.65 Adding adhesive to the plastic film reduces the MVTR, potentially leading to the build-up of exudate. Therefore, understanding the MVTR is crucial when using film dressings.9 Hence, maceration from wound exudate is a drawback of film dressings.66 Film dressings are cost-effective, soft, transparent, resilient, and adhesive.67 Films are appropriate for superficial pressure wounds.68 Commercially available film dressings for various indications, are shown below in Table 2.
Table 2 Comprehensive overview of commercial wound dressing products
Type of dressings |
Commercial products |
Indications |
Pros |
Cons |
Ref. |
Biopolymer wound dressings |
Film dressings |
Tegaderm® OpSite® |
For small, minimally exudative wounds as a primary dressing |
Allows monitoring of skin, conformable, cheap; absorbs significant volume of exudate, remains in position for many days |
Fluid will become trapped and macerate; allogenic; scab formation and dryness in the presence of low exudates |
8, 69 and 70
|
Mepilex® |
As a secondary dressing for other wounds |
Bioclusive® |
Moderately to heavily exuding wounds |
Kendall™ Polyskin™ |
|
Transeal® |
|
OmniDerm® |
|
Mepore® |
|
Carrafilm™ |
|
Blisterfilm™ |
|
|
Hydrogel dressings |
Hydrosorb® |
Necrotic wounds, low to medium exudating wounds, leg ulcers and pressure sores |
Maintains moist environment, allows vapour and oxygen exchange, aids tissue debridement, biocompatible |
Fluid accumulation leads to maceration or infection of skin mechanically weak |
8, 32, 41, 70 and 71
|
Intrasite® |
SAF-gel® |
Gel purilon® |
Aquasite® |
Aquaform® |
Carrasyn® |
Nu-Gel® |
2nd skin® |
Clearsite® |
Elasto-Gel™ |
FlexiGel™ |
Hypergel® |
Kendall™ |
Curafil™ |
Kendall™ Curagel™ |
Normlgel® |
Burn shield hydrogel® |
Tegagel™ |
Transigel™ |
Vigilon® |
XCell hydrogel® |
Geliperm® |
|
Foam dressings |
Biatain Ag® |
Ulcers or other chronic wounds, mainly stage 1 pressure ulcer |
Promote autolytic debridement, conformable; prevents skin deterioration |
Relatively expensive; often cause localized erythematous reaction |
8, 32, 41, 46 and 70
|
Mepilex® Border |
First-degree burns |
Allevyn® |
As secondary dressings for foams, hydrogels, and alginates |
Tegaderm® |
|
Lyofoam® |
|
PermaFoam® |
|
Mepilex® |
|
Cavi-Care® |
|
Betafoam® |
|
Biopatch® |
|
Aquacel™ |
|
Flexzan® |
|
Kendall™ Curafoam™ |
|
Kendall™ Hydrasorb® |
|
PolyMem® |
|
MitraFlex™ |
|
|
Hydrocolloid dressings |
DuoDERM® |
Light to moderate exuding wounds, sloughing or granulating wounds, ulcers, leg ulcers, stoma sites |
Formation of gel provides moisture and fluid exchange from the injury site; promote autolytic debridement |
Not suited for infected or heavily exuding wounds; cannot be used on dry wounds |
8, 32, 41, 46, 70 and 71
|
CombiDERM® |
GranuGel® |
Aquacel® |
Granuflex® |
Comfeel® |
Tegasorb® |
Oxyband® |
Cutinova® |
Hydrocol® |
NuDerm® |
Replicare® |
Karayahesive® |
Restore® |
|
Hydrofiber dressings |
Aquacel® Ag |
Chronic ulcers including pyoderma gangrenosum, deep exuding pressure ulcers, severe injuries, diabetic wounds and second-degree burns |
Highly absorbent; non-adherent |
Usually requires a secondary dressing |
8, 70 and 72
|
Aquacel® Extra™ |
Exufiber® |
|
Alginate dressings |
Kaltostat® |
Second degree burns, wounds with moderate to heavy exudates |
High absorption capacity; maintains moist wound environment; promotes clotting |
Secondary dressing is required |
8, 32, 41, 46, 70 and 71
|
Algidity® |
Ultra last® |
Sorbsan® |
Algosteril® |
KendallTM |
CurasorbTM |
Kalginate® |
Melgisorb® |
SeaSorb® |
Sorbalgon® |
Kaltocarb® |
Kaltogel® |
|
ECM-based scaffolds |
AlloDerm® |
Skin regeneration, chronic wound treatment, and tissue repair. |
Enhance wound healing, promote tissue regeneration, and improve scar quality |
Limited scalability, potential immunogenicity, variable scaffold quality, and higher costs |
73 and 74
|
Oasis® |
GraftJacket® |
Allopatch HD™ |
CardioCel® |
Synergraft® |
|
Nanofibrous scaffolds |
Bioweb™ |
Chronic wounds, acute wounds, burns, and skin grafts |
Enhance cell adhesion, natural matrix mimicry, controlled drug release, versatility, and promotes healing. |
Challenges in achieving consistent scaffold morphology, scalability, cytotoxicity, integrity maintenance, and higher costs |
75 and 76
|
Hydrogel dressings
Hydrogel dressings offer several therapeutic benefits, including a calming effect, reduced risk of infection, and promotion of moist wound healing.77 These benefits are closely linked to the unique properties of hydrogels. Hydrogel dressings are created using a three-dimensional network scaffold of hydrophilic polymer that has good water and biological fluid absorption capacity without disintegrating.78 By absorbing a significant amount of fluid from the injury site, hydrogel-based dressings provide a soothing effect and reduce the infection risk.77 Furthermore, their ability to maintain a moist environment promotes optimal wound healing conditions (Table 2).79 Hydrogels possess other properties that contribute to their therapeutic benefits. They can serve as effective drug delivery systems due to their high porosity and the ability to incorporate and release medications at the wound site.80 The cross-linked networks of hydrogels, formed through reversible physical interactions such as hydrogen bonds and van der Waals forces, allow for controlled release.81 Additionally, hydrogels have emerged as highly competitive candidates due to their unique capability to mimic the ECM (extracellular matrix), providing a suitable environment for cell growth and tissue regeneration.77
Natural polymers such as gelatin, chitosan, alginate, hyaluronic acid, and dextran are typically used for the manufacturing of hydrogel dressings owing to their favourable biocompatibility and biodegradability.82 The technique of manufacturing is particularly crucial given that the ultimate form, stability, and mechanical behaviour of the gel may be determined by the backbone chemistry and crosslinking procedure.83 Crosslinking can be achieved chemically (through polymerization, radiation, small-molecule or polymer–polymer crosslinking), or physically (via ionic interaction, hydrophobic interaction, hydrogen bonding, stereo complexation, and supramolecular chemistry).84 Material choice is perhaps the most important factor influencing the properties of hydrogels.85 Recently, Yang et al. developed hydrogen-bonding supramolecular hydrogels with promising mechanical strength and shape memory properties for postoperative antiadhesion application, showcasing a superior performance.86 Xin Zhao et al. conducted a study focusing on the development of injectable hydrogels with unique properties. These hydrogels exhibit anti-swelling characteristics, high strength, and wet adhesion. They also demonstrate a rapid gelling process, facilitating sutureless wound closure and scar-free repair of infectious wounds.87 By understanding the interplay between the therapeutic benefits and the properties of hydrogels, researchers and healthcare professionals can harness the potential of hydrogel dressings for enhanced wound care and improved patient outcomes.88
Foam dressings
Foam dressings are versatile wound care products that come in the form of hydrophilic or hydrophobic foam.89 They are typically made from polyurethane or silicone, which sometimes can be equipped with an adhesive border that aids in the thermal insulation of the wound bed and transportation of oxygen and moisture vapour.4 This enables the exudate to pass through the semi-permeable, non-adherent surface and into the insulated foam. Highly absorbent polyurethane foams are made up of two or three layers, comprising a hydrophilic wound contact surface and a hydrophobic backing.60 Due to the presence of a semipermeable backing, they aid in the uniform distribution of exudate throughout the absorbent layer and prevent outside leakage.69 This design helps maintain the integrity of the dressing and reduces the risk of contamination.90 Silastic foam dressings, a type of silicone-based rubber foam, offer flexibility and conform to the shape of wounds.4 This feature allows for better wound coverage and promotes wound healing by maintaining a close and secure contact between the dressing and wound bed.91 Compared to traditional gauze dressings, foam dressings provide greater comfort during nursing time and dressing changes.92 This is an important consideration given that patient comfort plays a crucial role in overall wound management and compliance with treatment protocols.21 Foam dressings are versatile and considered appropriate for mild to severely exuding wounds and lower leg ulcers, and are also recommended for granulating wounds.4 Their effectiveness in managing these types of wounds has been supported by clinical practice and recommendations from healthcare professionals. Additionally, foam dressings are commercialized under different names, as listed in Table 2.
Hydrocolloid dressings
The most commonly used moisture-retaining dressings are hydrocolloid dressings (Table 2). These dressings contain a combination of gelling agents (gelatin, sodium carboxymethyl cellulose, and pectin), elastic materials, and adhesives.93 They are supported by an appropriate carrier, such as film and foam, to form a self-adhesive, absorbent and a waterproof lining.33 Hydrocolloid wound dressings are typically made up of two layers, an outer semi-occlusive layer and an inner hydrocolloid layer.9 Hydrocolloids have the potential to adhere to both the moist and dry surfaces of wounds. Upon interaction with the wound exudates, hydrocolloids swell, leading to the formation of a cohesive gel-like substance at the site of injury.90 In addition, their occlusive nature provides resistance to the entry of water, oxygen and air contaminants, which facilitates the process of granulation and angiogenesis.94 Depending on the amount of exudate and the type of hydrocolloid dressing, hydrocolloid interaction can reduce the dressing frequency to up to seven days wear time.33
Hydrofiber dressings
Hydrofiber dressings are fabricated using sodium carboxymethylcellulose, which forms a gel-like substance on interaction with exudating wounds.95 These dressings are available in the form of sheets or ribbons of sodium carboxymethylcellulose with high absorbing capacity.22 In the process of absorbing wound exudate, hydrofibres change into gels that help maintain a moist environment, while promoting autolytic debridement.28 Hydrofiber ribbons can be packed into deep concave regions, making them particularly beneficial for deep wounds.96 However, they should only be packed up to 4/5th of the wound area because they swell as they turn into a gel. After application, the hydrofiber dressings must be changed every three days.97 Additionally, they have been shown to work well on donor sites with partial thickness and second-degree burns.70 The commercially available products of hydrofiber dressings together with their indications, advantages, and associated drawbacks are depicted in Table 2.
Alginate dressings
Alginate dressings are biodegradable and highly absorbent dressings derived from naturally occurring calcium and sodium salts of alginic acid found in seaweed rich in mannuronic acid and guluronic acid.98 When applied to wounds, alginate dressings exchange sodium ions on the wound surface with the calcium ions from alginate to produce a protective layer, and thus provide a moist wound environment and minimize bacterial infections at the wound site.98 Most alginates have a fibrous structure, which means that if there is not enough wound exudate to gel the fibres, leftover fibres may remain in the wound.99 Given that they prompt a foreign body response, they may lead to an inflammatory response.98 The therapeutic effectiveness of alginate dressings is affected by the proportion of other polymers used in combination with alginate, the type of cross linkers used, the length of time the crosslinks are formed, the type of excipients used, the incorporation of nanoparticles, and the presence of antibacterial agents.98 Moreover, the benefits and drawbacks of alginate dressings are listed in Table 2.
ECM-based scaffolds
An innovative approach, namely extracellular matrix (ECM)-based scaffolds, is intricately linked to the ongoing advancements in natural biomaterials for wound care and tissue regeneration.100 ECM-based scaffolds derived from natural resources such as dermal proteins are typically generated by decellularizing mammalian tissues, retaining the native ECM and its signalling proteins.57,101 However, various factors, such as the choice of donor tissue,102 the decellularization procedure,103 and post-decellularization processing steps, including terminal sterilization and chemical crosslinking, influence the quality and efficacy of ECM scaffolds.104 The quality of the ECM scaffold plays a crucial role in constructive healing responses and clinical outcomes.105 For instance, researchers have successfully created ECM scaffolds by combining sericin, a naturally occurring silk protein, with ECM obtained from human placenta, demonstrating non-toxicity, angiogenic properties, and wound healing efficacy.106 This innovative biomaterial offers potential applications in tissue regeneration, showcasing the advantages of natural biomaterials in advancing wound care (Table 2).
Nanofibrous scaffolds
Nanofibrous scaffolds have emerged as promising candidates for wound care and skin tissue regeneration due to their unique properties.107 These scaffolds offer biological compatibility, biodegradability, adhesive properties, moisture absorption, and mechanical strength.108 Nanofibrous scaffolds fabricated with both natural and synthetic polymers have shown promise in developing drug delivery systems, enabling the controlled release of bioactive compounds such as growth factors, vitamins, antimicrobial substances, antioxidants, and hormones.109 This is achieved through techniques such as electrospinning of blended polymers or coaxial spinning of two different polymers together with drugs and active agents.110 These scaffolds serve as artificial extracellular matrices, providing a natural environment for tissue formation.111 Compared to other forms of scaffolds, nanofibrous scaffolds offer enhanced cell adhesion, proliferation, and differentiation efficiency, which are attributed to their high surface-to-volume ratio.112 Additionally, the incorporation of nanomaterials, such as CuO/Ag nanoparticles (AgNPs), within electrospun cellulose acetate nanofibers has shown potential bacteriostatic and bactericidal properties.113 These capabilities highlight the versatility and potential applications of nanofibrous scaffolds in wound healing and tissue regeneration (Table 2).
Bioactive-supplemented wound dressings
Bioactive-supplemented wound dressings target specific bioactive compounds including antimicrobial agents, stem cells, growth factors and vitamins to the wound site for accelerating the healing process and creating a dynamic interaction with the wound surface.114 The bioactive dressings employed in wound healing comprise a range of options, such as antimicrobial-boosted dressings, dressings loaded with stem cells, and vitamin-enriched dressings.
Supplemented antimicrobial dressings
Although microbial bioburden has long been acknowledged as a potential hindrance to wound healing, biofilms are now regarded as a major microbiological obstruction.115 Biofilms are extracellular polymeric substances that are self-produced by the surface-attached microbial communities, shielding the microbes from dangers outside their environment, and thus preventing the action of antimicrobial medications.116 It has been suggested that biofilms contribute to chronic inflammation in wounds, defective granulation-tissue development, and epithelialization.117,118 Antimicrobial-impregnated dressings can be utilised for superficial wounds that are highly susceptible to infection.70 Hydrofiber technology has been used to create a novel, next-generation antibacterial dressing (NGAD) that can regulate exudate as well as two major local barriers to wound healing, i.e., biofilm and infection.118 Recently, Xun Wang et al. created a multifunctional hydrogel dressing containing cypate-conjugated antimicrobial peptides (AMP-cypates), liposome-encapsulated perfluorodecalin, and recombinant type III collagen, which showed antimicrobial action to aid healing of chronic wounds in a hypoxic environment.119 These dressings include metal or metal oxide-based dressings, cationic antimicrobial-based dressing, bacteriotherapeutic dressing, and phytochemical(s) supplemented dressing.
Metal- or metal oxide-based dressing
Metal and metal oxide-based bioactive augmented wound dressings include metallic or metal oxide components (silver, copper, zinc) in wound dressings, which are frequently used for antibacterial or therapeutic properties. These dressings can help promote wound healing and prevent infections.120
Silver is regarded as a broad-spectrum antimicrobial agent with potential application for superficial infections. Hydrogels, alginates and foams can be impregnated with silver particles in addition to conventional topical wound treatments.70 Small amounts of silver are released by slow-release dressings that have been impregnated with silver, and the chloride ions present in wound exudate immediately bind with these silver ions, exhibiting structural changes to the bacterial cell membrane, as follows: (a) binding to tissue proteins; (b) denaturing bacterial DNA/RNA and preventing replication; (c) stimulating the synthesis of reactive oxygen species (ROS); (d) interacting with bacterial proteins; and (e) inhibiting the respiratory chain and interfering with respiratory enzymes.11,121 According to research, Acticoat, a three- or five-layered silver mesh-based dressing containing silver nanocrystals, reduces wound infection, lessens the need for dressing changes, lowers the levels of matrix metalloproteinase activity, bioburden and wound exudate, and expedites wound healing in chronic conditions in a cost-effective way.122 Precautions for the usage of these dressings over epithelializing and proliferating wounds should be taken, given that in vitro studies have shown that Acticoat is toxic to squamous cells and fibroblasts.122 Further, the study by Christopher Doherty et al. compared the in vitro and in vivo effectiveness of different silver-based dressings against Staphylococcus aureus (S. aureus) and Pseudomonas aeruginosa biofilm models and reported their varying effects on biofilms depending on their physicochemical properties123 (Fig. 5).
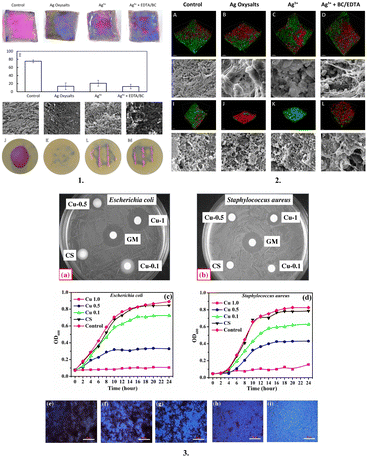 |
| Fig. 5 Metal- or metal oxide-based dressing: (1) Visualisation of P. aeruginosa biofilms after treatment with silver dressings. (A)–(D) Viability of bacteria within P. aeruginosa biofilms grown on the porcine skin was visualised with the PrestoBlue viability dye after 24 h treatment with silver dressings or non-antimicrobial control dressings. Viable bacteria appear pink, whereas non-viable bacteria and the porcine skin appear blue. (E) Viable bacteria (pink staining) were quantified using Image Pro version 10. (F)–(I) Scanning electron microscopy images of P. aeruginosa biofilms grown on the porcine skin and treated with silver dressings or non-antimicrobial control dressing for 24 h. SEM scale bar = 5 μm. (J)–(M) Colony biofilms grown on filter membranes and stained with PrestoBlue viability dye following 24 h incubation with silver dressings. (2) Visualisation of in vivo wound biofilms after 3 days treatment with control or silver dressings. Confocal images of P. aeruginosa (A)–(D) and S. aureus (I)–(L) stained with Toto 1 (green) to visualise the extracellular nucleic acids, a component of the biofilm extracellular polymeric substance, and Syto 60 (red), which stains intracellular nucleic acids. Scanning electron microscopy images of P. aeruginosa (E)–(H) and S. aureus (M)–(P) biofilm-infected wounds after 3 days of treatment with control and silver dressings. SEM scale bars = 5 μm. Confocal imaging scale bars = 50 μm; (3) Inhibition zone against (a) E. coli and (b) S. aureus and growth inhibition curve in TGY broth against (c) E. coli and (d) S. aureus. Biofilm development on the surface of films (e) CS, (f) Cu 0.0, (g) Cu 0.1, (h) Cu 0.5 and (i) Cu 1.0. White scale bars represent 80 μm. Reproduced from ref. 123 and 124 with permission from [Springer, Elsevier], 2023. | |
Zinc is essential in regulating the wound healing remodelling process at all stages. This encompasses activities such as repairing the cell membrane, managing oxidative stress, coordinating blood clotting, moderating inflammation and immune response, facilitating tissue re-epithelialization, promoting the growth of new blood vessels (angiogenesis), and participating in the formation of fibrous tissue and scars.125
Copper, a trace mineral, plays a pivotal role in numerous wound healing processes.126 It actively encourages wound healing through several mechanisms.126 Firstly, it fosters the growth of new blood vessels (angiogenesis) by elevating the levels of hypoxia-induced factor 1alpha (HIF-1α), vascular endothelial growth factor (VEGF), and copper-dependent transcription factor Atox1.127 Moreover, copper supports the expression of integrin, a protein vital for cell adhesion and signalling.128 It also stimulates dermal fibroblasts to secrete essential structural proteins such as fibrinogen, elastin, and collagen, while contributing to the stabilization of these proteins.129 Furthermore, copper plays a pivotal role in upregulating copper-dependent enzymes and polysaccharides, including lysyl oxidase, metalloproteinases, glycosaminoglycans, and small proteoglycans, all crucial for processes such as matrix remodeling, cell proliferation, and re-epithelization. Lastly, copper facilitates the migration of both skin and stem cells, which is imperative for effective wound healing.130 Recently, Mishra et al. synthesised copper-incorporated microporous chitosan–polyethylene glycol hydrogels loaded with naproxen, and the results showed superior antibacterial activity with sustained drug release, excellent keratinocyte cell response, and inhibition of biofilm formation.124
Cationic antimicrobial-based dressings
A cationic antimicrobial-based dressing is a form of wound dressing that contains cationic antimicrobial compounds to aid in the prevention or treatment of wound infections.131 There are several examples of cationic antimicrobial-based dressings used in wound care including polyhexamethylene biguanide-containing dressing, chlorhexidine, and iodine-supplemented dressings (Fig. 6).
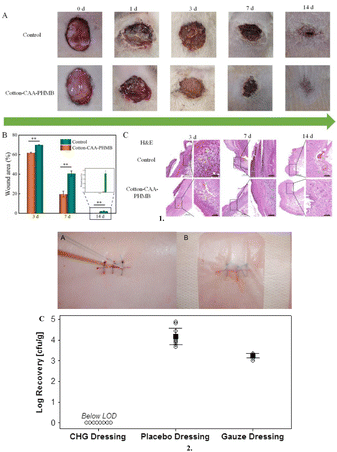 |
| Fig. 6 Cationic antimicrobial-based dressings: 1. (A) Images post-surgery at different time points, (B) Statistics of the wound area during the healing process and (C) H&E staining of wound tissue from both groups on day 3, 7 and 14 after surgery (scale bar 500 μm and 100 μm). 2. (A) Representative photograph of a sutured incision being inoculated with MRSA (methicillin-resistant Staphylococcus aureus) suspension, (B) Photograph of postoperative wound with chlorhexidine gluconate dressing applied, and (C) MRSA recovered from the wound specimens excised 3 days postoperatively. Individual data points are represented with open circles. The means and 95% confidence intervals are represented by black squares and lines. All 8 CHG dressing data points were below the LOD. Cotton-CAA PHMB, Cotton-chloroacetic acid-polyhexamethylene biguanide; cfu, colony-forming units; CHG, chlorhexidine gluconate; LOD, limit of detection; and MRSA, methicillin-resistant Staphylococcus aureus. Reproduced from ref. 132 and 133 with permission from [Elsevier], 2023. | |
Iodine is considered a broad-spectrum antimicrobial that enters the cell wall of bacteria and disrupts the cell membrane by breaking the hydrogen bonds, which eventually results in cell death.134 Iodine dressings exist in two distinct forms of sheets and gels.70 Iodine dressings are available as povidone iodine (acts as a reservoir of free active iodine135 and cadexomer iodine, as well as several commercial formulations).70 Povidone–iodine, an antiseptic, is infused with gauze. A more recent composition of dextran beads called cadexomer-iodine (a 3-D starch lattice containing 0.9% iodine)69 promotes the sustained release of iodine, which lowers the bacterial burden and aids in the debridement of the wound by absorbing up to 7 times its weight in exudate. Shaydier Argel et al. assessed bacterial nanocellulose loaded with povidone–iodine as a bioactive dressing for skin and soft tissue infections and demonstrated the antibacterial and anti-inflammatory properties of the dressing.136
Polyhexamethylene biguanide (PHMB) is a biguanide, a strong base that is strongly positively charged at physiological pH.137 PHMB functions similarly to iodine in that it penetrates bacterial cells and breaks the cell membrane, allowing potassium ions and cytosolic components to flow out and cause cell death.138 To treat acute and chronic wounds that are heavily colonised and locally infected, PHMB is the most frequently used antiseptic.132,135 Chlorhexidine-containing dressings are especially useful in surgical wounds, burns, and chronic wounds, where the risk of infection is high.139 By breaking the cell membrane, chlorhexidine kills a wide range of microorganisms, including bacteria and fungi. This aids in the regulation of bioburden inside the wound environment, thereby helping in the overall healing process.140 A recent study by Mana et al. examined the efficacy of a postoperative wound dressing containing chlorhexidine gluconate against methicillin-resistant Staphylococcus aureus (MRSA) in an in vivo porcine incisional wound model and concluded that it showed an excellent antimicrobial activity against MRSA contaminating a surgical wound.133
Bacteriotherapeutic dressings
Dysbiosis in the skin microbiota is inherently associated with wounds due to the exposure of the subcutaneous tissue to a wide variety of opportunistic microorganisms and activation of the immune response. The application of topical bacteriotherapeutics, both pre- and post-biotics, has been reported to restore the skin eubiosis, prevent infection, reduce inflammation and enhance healing.77,78 There are numerous reports wherein different probiotic impregnated dressings have shown superior wound healing activity in different stages of wound healing compared to conventional dressings79,81 (Fig. 7).
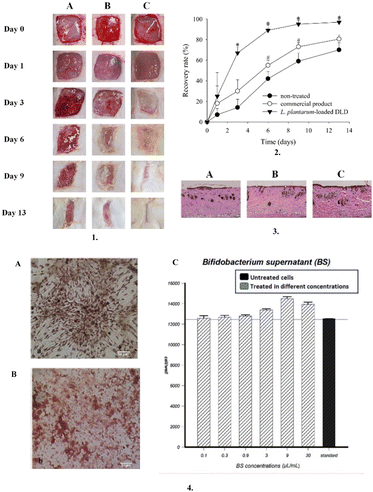 |
| Fig. 7 Bacteriotherapeutic dressings: 1. Representative images of P. aeruginosa-infected wound model: (A) non-treated, (B) commercial product, and (C) L. plantarum-loaded DLD. 2. Wound recovery rate (%) of the P. aeruginosa-infected wound model. *p < 0.05 compared with non-treated and commercial product, #p < 0.05 compared with non-treated. 3. Representative histopathological profiles of wounds recovery in P. aeruginosa-infected model: (A) non-treated, (B) commercial product, and (C) L. plantarum-loaded DLD. 4. (A) Rat bone marrow stromal cells after 14 days of treatment with Bifidobacterium bifidum supernatant; stained with Alizarin red, (B) Rat bone marrow stromal cells after 14 days of treatment with Bifidobacterium bifidum supernatant; stained with Oil red-O (400× using the inverted microscopic system), and (C) Effect of the logarithmic concentrations of Bifidobacterium bifidum supernatant on rat bone marrow stromal cells. The MTT assay showed that the highest proliferation occurred in 9 μL mL−1 concentration. The control group included cells with standard culture medium without Bifidobacterium bifidum supernatant and bacterial cell mass as a negative control. Reproduced from ref. 141 and 142 with permission from [Elsevier, Shiraz University of Medical Sciences], 2023. | |
Nisin is one of the most widely reported postbiotics for augmenting the wound healing effect.71,92,143 The recent trend of incorporating postbiotics in dressing materials consisting of probiotics such as chitosan, gums, and alginate has also shown promise.94 Furthermore, due to the biocompatibility and positive benefits on skin health, probiotics are now again receiving attention in pharmaceutical research.81,117 Throughout their growth, these living microorganisms produce compounds such as bacteriocins and organic acids, which have antibacterial properties and aid in accelerating the wound healing process.144 Recently, Jung Suk Kim et al. created a dual-layer wound dressing (DLD) that contains Lactobacillus plantarum (L. plantarum) by encasing a hydrogel in a hydrocolloid and proved that it is an efficient wound treatment system compared to commercial products due to its superior mechanical and wound healing qualities.141 Moreover, owing to the therapeutic efficacy of probiotics by boosting immune cell production, invading inflammatory cells, and possessing antimicrobial properties, the study by Atena Bazjoo et al. demonstrated that the biodegradable film made of chitosan and Bifidobacterium bifidum cell-free supernatant accelerated wound healing in rat models.142,145
Phytochemical-supplemented dressings
Phytochemical-supplemented dressings are wound dressings enriched with phytochemicals, which are bioactive molecules derived from plants. These dressings including curcumin-, honey- or essential oil-supplemented dressings use the inherent healing abilities of phytochemicals to accelerate wound healing.
Curcumin is obtained from the rhizome of Curcuma longa and is frequently utilised in both acute and chronic wound healing with anti-inflammatory, anti-infection, and antioxidant properties.146 Moreover, it fights free radicals by acting as an antioxidant and has been proven to be a potential candidate for reducing reactive oxygen species (ROS) and can accelerate healing due to its diketone structure and phenolic hydroxyl groups.42 Gabriela Isopencu et al. in their study demonstrated the antimicrobial activity of turmeric extract-loaded bacterial cellulose–carboxymethyl cellulose composites against S. aureus, Escherichia coli (E. coli), and Candida albicans (C. albicans) and revealed the significantly high absorbing capacity of these composite dressings for exudates of chronic wounds.147
Honey is recommended for use in wound dressings due to its medicinal qualities, which include the capacity to nourish wounds topically, reduce inflammation, stimulate granulation and angiogenesis, and epithelialize wounds.148 Honey can prevent the development of microorganisms, which is ascribed to its acidity, endogenous hydrogen peroxide, and a high sugar to water ratio.70 Due to the high sugar content in honey, it exerts an osmotic pressure, which inhibits bacterial development and cell proliferation.149 With an acidic pH (3.4–6.1), honey may help macrophages destroy bacteria and prevent the growth of microbial biofilms.149 Honey has proven to be effective in the management of burns and ulcers, especially ulcers related to diabetes such as DFU (diabetic foot ulcers).150 Using an extrusion-based printing process, Ana Brites et al. developed antibacterial manuka honey-gelatin 3D patches with antibacterial properties against both Gram-negative (E. coli) and Gram-positive bacteria (Staphylococcus epidermidis and S. aureus), which are common in wound infection.29 Furthermore, it promoted angiogenesis and increased the production of human skin fibroblasts and epidermal keratin cells29 (Fig. 8).
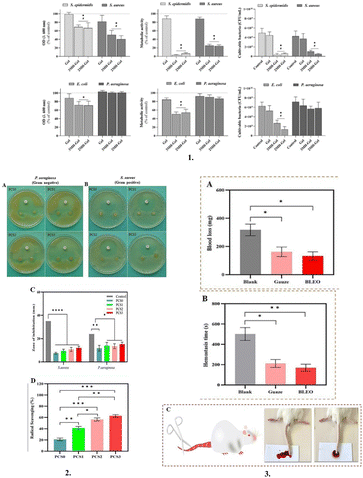 |
| Fig. 8 Phytochemical supplemented dressings: 1. Antibacterial activity of 3D hydrogels (Gel, 3MH-Gel, and 2MH-Gel) against S. epidermidis, S. aureus, E. coli and P. aeruginosa, after 24 h of incubation. Optical density (OD), metabolic activity (resazurin reduction) and CFU counting. * Significantly different from the control (C+, normal growth medium). # Significantly different from the Gel patch. 2. Antibacterial inhibitory effects of PCS (polyvinyl alcohol/chitosan/Saqez essential oil) bilayer hydrogels against (A) S. aureus and (B) P. aeruginosa (Disk diameter = 6 mm). (C) Zone of inhibition diagram of PCS bilayer hydrogels and (D) Antioxidant activity of PCS bilayer hydrogels. 3. (A) Results of the blood loss in the bilayer hydrogel with SEO using the mouse tail amputation model (bleeding blood volume in mg), (B) Results of the hemostasis time in the bilayer hydrogel with SEO (Saqez essential oil) using the mouse tail amputation model (bleeding blood time in seconds), and (C) Images of the hemostatic ability and effectiveness of bilayer hydrogel. The right-side image is BLEO sample and the left-side image is control (with SEO using the mouse tail amputation model). Reproduced from ref. 29 and 151 with permission from [Elsevier], 2023. | |
Essential oils are volatile natural mixtures that contain volatile secondary plant metabolites with antibacterial, anti-inflammatory, antioxidant, anti-allergic, anti-inflammatory, and chemoprotective properties.152 It has been reported that essential oils attack the phospholipids in bacterial cell membranes and the lipids present on their cell walls, increase the rate of permeation, and eventually cause cell death, leading to a drop in pH level, as well as the termination of cellular processes including ATP production, gene transcription and translation.153In vitro and in vivo analysis of a novel bilayer hydrogel dressing fabricated by incorporating Saqez essential oil, polyvinyl alcohol and chitosan demonstrated enhanced antibacterial activity and potent antioxidant properties, coupled with their non-toxic nature, biocompatibility, and effective hemostatic properties.151
Stem cell-loaded dressings
Stem cell-based therapies can contribute to tissue repair and regeneration by the incorporation of stem cells into dressing materials, enabling these cells to differentiate into various types based on the wound.154
They have emerged as a new paradigm in managing wound treatment and skin repair.155 However, in general, stem cells used in skin tissue engineering have some drawbacks, including immunological sensitivity, a decreased survival rate, and slower rates of proliferation and differentiation.156 Thus, to overcome these challenges, preclinical studies have suggested the use of various stem cells, including that from the umbilical cord, bone marrow, and placenta, together with natural and synthetic polymeric substances, such as gelatin, hyaluronic acid, poly(lactic-co-glycolic acid), and polycaprolactone, to create stem cell 3D scaffolds, which are equivalent to ECM.156 ECM is a noncellular component of tissues that acts as a scaffold for cells and plays a critical role in regulating growth, homeostasis, inflammation, and repair,157 and helps in providing a suitable environment for wound healing for optimum functioning around the wound bed.46,158 In the study by Mohanty et al., they developed a bioconjugate of human epidermal growth factor and curcumin loaded with mesenchymal stem cells for in vivo diabetic wound healing, which enhanced the production of granulation tissue, collagen, and angiogenesis (Fig. 9).159
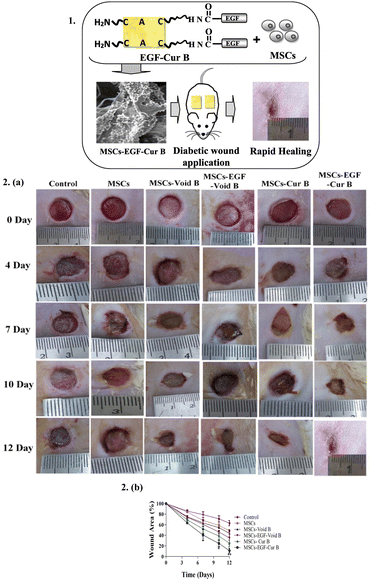 |
| Fig. 9 Stem cell loaded dressings: (1) Schematic representation of a human epidermal growth factor-curcumin bandage bioconjugate (EGF-Cur B) loaded with mesenchymal stem cells (MSCs) for in vivo diabetic wound healing. (2) Effective wound healing activity of MSCs-EGF-Cur B after topical application on full thickness diabetic wound in a rat. (a) Macroscopic appearance of diabetic wounds of a rat treated with NS (as control), MSCs, MSCs-void B, MSCs-EGF-void B, MSCs-Cur B, and MSCs-EGF-Cur B at different days of post-wounding. (b) Dermal wound area at different post-wounding days as a percentage calculated with respect to the original size. Data as mean ± SEM, n = 5; p < 0.05* and p < 0.005**, All groups vs. Control. Reproduced from ref. 159 with permission from [Elsevier], 2023. | |
Vitamin-enriched dressings
Vitamin-enriched dressings, another type of bioactive wound dressing, offer advanced therapeutic benefits by delivering essential vitamins directly to the wound site, promoting the healing process.160 Studies have demonstrated the efficacy of vitamin-enriched dressings in various wound healing applications. These dressings are often loaded with vitamin C, vitamin A, and vitamin E, each known for their unique roles in supporting wound repair and skin health.160 For instance, vitamin A contributes significantly to wound healing by increasing fibroblast proliferation and aiding in the formation of granulation tissue.161 Vitamin E provides antioxidant protection, stabilizes granulation tissue, and stimulates re-epithelialization, which are crucial for wound closure.162 For example, combining vitamin C with propolis resulted in synergistic effects on wound closure, while vitamin B12-enriched nanofibrous dressings accelerated wound healing and re-epithelialization processes (Fig. 10).163,164 Additionally, vitamin D3-loaded hydrogels enhanced cell proliferation and the wound closure rates.165 By incorporating vitamins in biomaterial-based dressings, researchers aimed to overcome challenges such as low bioavailability and rapid oxidation, ensuring sustained release and optimal therapeutic efficacy.166 These advancements hold promise for improving wound care outcomes, particularly in chronic wound management, by harnessing the regenerative potential of vitamins within innovative dressing formulations.
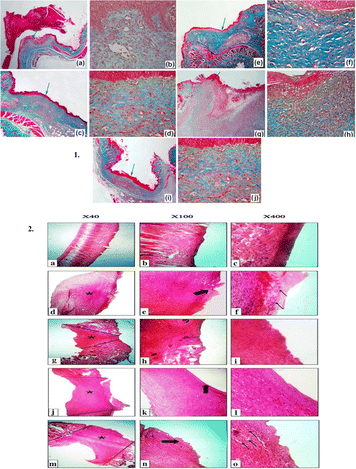 |
| Fig. 10 Vitamin-enriched dressings: (1) Histological profiles of wound area in diabetic (STZ) mice after 15 days of treatment with the cellulose-based films using MT staining. (a) and (b) Control of the diabetic (STZ) group. (c) and (d) Cel-PVA (cellulose–polyvinyl alcohol) treatment, (e) and (f) Cel-PVA/VitC treatment, (g) and (h) Cel-PVA/Prop (cellulose–polyvinyl alcohol/propolis) treatment and (i) and (j) Cel-PVA/VitC/Prop treatments. Note: the images presented in the left column have low magnification (40×), whereas the images presented in the right column have high magnification (400×). Green arrows indicate the selected regions of each image. (2) H&E-stained microscopic sections of healed incisions in rats at 14 days. (a), (b), and (c) Positive control, (d), (e), and (f) sterile gauze-treated wound, (g), (h), and (i) alginate hydrogel without vitamin D3, (j), (k), and (l) alginate hydrogel/3000 IU vitamin D3, and (m), (n), and (o) alginate hydrogel/30 000 IU vitamin D3. Reproduced from ref. 164 and 165 with permission from [Elsevier], 2024. | |
Biosensor dressings/smart dressings
A smart and multipurpose dressing with on-demand therapeutic properties, strong adhesive and antimicrobial properties, extensive wireless communication, and real-time sensing by monitoring physiological conditions (temperature and moisture) along with relevant biomarkers (pH, glucose, uric acid, oxygen, nitric oxide, inflammatory factors, toxins, and enzymes secreted by bacteria) has been developed in response to the growing need for rapid wound healing.167–171 Advancements in technology and knowledge of the interactions among biochemical factors, cells, and the surfaces of biomaterials have led to the development of theranostic wound dressings.172 Furthermore, with the rapid growth of wearable wound monitor technology for temperature and pH monitoring, wound diagnosis and care have improved.173 According to a study, changes in wound temperature by 2.2 °C may be a warning sign of imminent wound degeneration,174 and keeping the pH at 7.4 at the site of the injury gives room for bacterial growth and can lead to subsequent wound infection.175 Chronic wounds have a pH between 7 and 9, and this alkaline environment makes them more prone to bacterial colonisation.176 Recently, Nan Pan et al. electrospun curcumin with a polycaprolactone matrix and generated a pH-responsive film, which showed potent wound healing capabilities.177
Sensory smart bandages
To speed up the decision-making process for wound care, sensors in the wound environment can provide crucial information.178 They can also reduce the frequency of changing the wound dressing. Additionally, there are substantial advantages in terms of decreased healthcare costs and hospital stays.41,179
The pH of the wound affects a wide range of significant processes, including angiogenesis, protease activity, oxygen release, bacterial toxicity, biofilm development, fibroblast proliferation and migration, antibiotic efficiency, and take-rate in skin grafting (Fig. 11).180 Given that the pH of the wound fluctuates depending on the stage of wound healing, non-invasive, pH testing of the wound can be helpful to assess the wound condition and the effectiveness of the therapeutic approach.181 Normal healthy skin has a pH in the range of 4–6, which is slightly acidic.182 However, when the skin is damaged, the internal body pH of 7.4 is exposed, which disturbs this acidic environment.183 Numerous studies have examined the possibility of using wound dressings as an indicator for the pH condition of the wound. For instance, Arafa et al. prepared an environmentally safe pH-sensing wound dressing by incorporating Curcuma longa extract in an itaconic acid-grafted hydrogel made of hydroxyethyl cellulose.184 A colour change from clearly yellow to dark red was observed for pH values above 7. It was determined that the manufactured wound dressing can visibly display the pH of the wound bed, in addition to having the therapeutic effects of CLE.184 Furthermore, to track the condition of the wound, Jankowska et al. inserted a fluorescent pH-sensing dye in the hydrogel and showed that the hydrogel had high sensitivity for varying pH.168
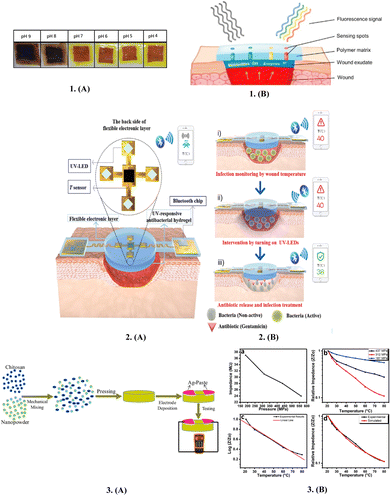 |
| Fig. 11 Sensory smart bandages: 1. (A) Smart therapeutic pH-sensitive wound dressing based on CLE (Curcuma longa extract) and HEC (hydroxyethylcellulose) hydrogel at different pH values (4–9). (B) Scheme of the sensing principle for non-invasive wound monitoring based on the detection of pH-values. 2. Schematics of the structures and working principles of smart flexible electronic-integrated wound dressing, (A) Flexible electronic device is integrated with a sensor for monitoring temperature and four UV-LEDs for emitting UV light (365 nm) to trigger the release of antibiotics from the UV-responsive antibacterial hydrogel. (B) Conceptual view of the integrated system for infected-wound monitoring and on-demand treatment: (i) real-time monitoring of wound temperature and providing an alert of hyperthermia caused by infection; (ii) turning on UV-LEDs to trigger the release of antibiotics; and (iii) infection inhibition by the released antibiotics, resulting in decreased wound temperature. 3. (A) Schematic diagram of the fabricated sensors. (B) Relationship between (a) initial impedance and fabrication pressure, (b) relative impedance and temperature, (c) log of impedance and temperature and (d) comparison between simulated and experimental results of the fabricated sensors. Reproduced from ref. 168 and 184–186 with permission from [Elsevier, Wiley], 2023. | |
One of the most crucial and promising signs is temperature, which is intimately related to the inflammation and infection states at the wound site.187 Before any other visible symptom, abnormal fluctuations in wound temperature may be considered a preliminary indication of infection.185 For instance, temperatures between 36 °C and 38 °C suggest a decrease in infection and make it easier for more water molecules to enter the film, leading to activation of humidity sensors with great sensitivity. However, temperatures below 33 °C cause fibroblasts, neutrophils, and epithelial cells to function less actively, delaying the healing of wounds.185 Furthermore, in wound assessment, the relevance of portable infrared sensor-based technologies that offer structural assessment of clinical tissues by thermal imaging is growing.185 Pang et al. created a smart versatile double-layered dressing with adaptable electrical parts paired with an antimicrobial hydrogel to offer real-time assessment of temperature at the injury site and deliver antibiotics by in situ UV irradiation.185
Oxygen is a critical mediator in the healing of wounds and is necessary for the deposition of collagen, epithelialization, fibroplasia, angiogenesis, and infection resistance.188 The inability of the peripheral vasculature to properly carry O2-rich blood to the tissue, which results in wound hypoxia, is one of the main problems frequently associated with chronic wounds.189,190 Moreover, in non-healing wound exudate, the partial pressure of oxygen is between 5 and 20 mm Hg, while in healthy tissue, it is between 30 and 50 mm Hg.191 In the study by Mostafalu et al., they designed and implemented a flexible smart bandage with embedded flexible oxygen sensors, small readout electronics, and a wireless data transfer system.192 Flexible oxygen sensors were created, and it was demonstrated that they had good linear output and sensitivity. To act as a mechanically robust wound bandage, the bandage was further 3D printed utilising an elastomeric material with high strength and flexibility.191
Moisture balance is crucial for achieving ideal wound healing conditions.193 It has been demonstrated that the right amount of moisture in the wound is essential for initiating the re-epithelialization and granulation processes.193 However, it is important to recognise that excessive hydration, particularly when coupled with a high level of matrix metalloproteinases, can cause wound maceration, which can be typical in chronic wounds and will impede the healing process.194 Chani et al. created an impedimetric humidity and temperature sensor based on organic–inorganic nanocomposites using chitosan and CuMn2O4-spinel nanopowder. The findings showed that impedance change serves as the foundation for the temperature and humidity sensing mechanisms.186 The absorption and adsorption of water molecules are attributed to the decrease in impedance, which occurs in response to rising humidity.194 Furthermore, Rani et al. created a metal oxide platform-based highly nanoporous niobium oxide (Nb2O5) film with distinctive three-dimensional vein-like structures.195 They concluded that the formation of this nanoporous vein structure across the film promotes the surface area available for sites for water molecule adsorption and makes it easier for more water molecules to penetrate the film, leading to humidity sensors with high sensitivity.196
To enable wound monitoring, several sophisticated flexible bandages equipped with various sensors have been created. However, most of them are still at the level of sensor integration, which do not address the issues and difficulties encountered in real-world applications, such as the lack of multi-task detection systems on dressings or the requirement for additional external batteries or power supplies for detection.185 Smart bandages with an embedded flexible multipurpose sensor that can diagnose the wound status by accurately detecting various wound parameters (uric acid (UA), pH, and temperature) simultaneously have the potential to revolutionise the concept of wound management and significantly impact the outcomes of therapy.30,185,197 To detect UA, pH, and temperature at the wound site, Sharifuzzaman et al. created a smart, stretchable, and flexible multifunctional sensor-integrated wound bandage based on an MXene-functionalized porous graphene scaffold.30 This bandage demonstrated excellent clinical linearity, sensitivity, repeatability, and stability for detecting UA, pH, and temperature in both analytical and real samples (Fig. 12).30
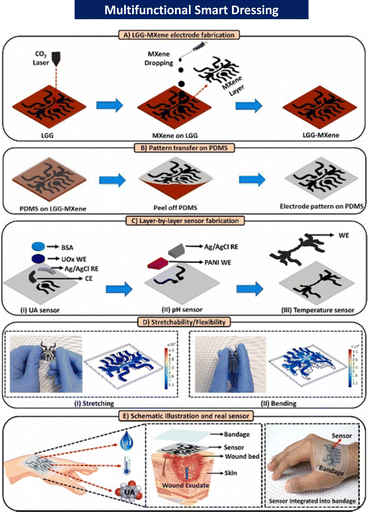 |
| Fig. 12 Stepwise fabrication scheme of the proposed stretchable and flexible smart bandage with an integrated multifunctional sensor. MXene (a novel class of 2D multilayered nanosheets); LGG-MXene (laser-guided graphene-MXene); PDM (polydimethylsiloxane); BSA (bovine serum albumin); WE (LGG-MXene/PDMS); CE (LGG-MXene/PDMS); RE (Ag/AgCl); UOx (uricase enzyme); and PANI (pH-sensitive membrane). Reproduced from ref. 30 with permission from [Elsevier], 2023. | |
Medicated smart dressings
A promising method to treat wound infections and hasten the healing process involves the real-time monitoring of the wound status and prompt therapy delivery with smart wound dressings.30 On-demand therapy with actively regulated drug delivery appears to be more crucial for wound healing than wound monitoring.198 Extensive efforts have been undertaken to incorporate responsive drug delivery systems in smart wound dressings for more precise administration and on-demand therapy.30 For an instance, Lou at el. created a smart flexible wound dressing with integrated electronics that can track the temperature of the wound in real time to detect infections early and deliver immediate relief via the UV-activated release of antibiotics185 (Fig. 13).
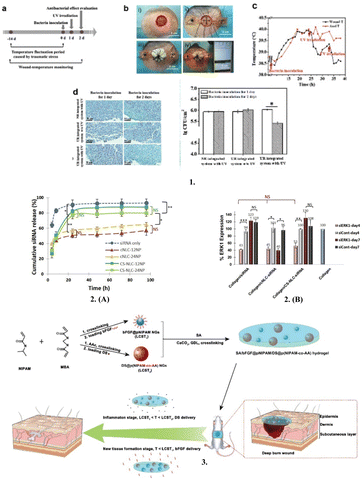 |
| Fig. 13 Medicated smart dressings (1) In vivo studies on infection monitoring and on-demand treatment. (a) Timeline of the animal experiments. (b) Operational procedure: (i) wound creation, (ii) implantation of the integrated system, (iii) pressure dressing, and (iv) real-time wound-temperature monitoring. (c) Curve of the recorded wound temperatures by the integrated system in real time. Anal temperature was measured as a reference. (d) Gram staining (left) and statistical analysis of bacteria densities (right) of the infected wounds treated by different groups. 2. (A) In vitro release of fluorescently labeled free siRNA (siRNA only) or siRNA complexed with either cationic (cNLC-12NP, cNLC-24NP) or chitosan (CS-NLC-12NP, CS-NLC-24NP) particles from collagen scaffolds. (B) Down-regulation of ERK 1 in NIH 3T3 cells when incubated on the different collagen scaffolds for 4 or 7 days. 3. Synthesis of SA/bFGF@pNIPAM/DS@p(NIPAM-co-AA) (sodium alginate/basic fibroblast growth factor-loaded poly(N-isopropylacrylamide)/diclofenac sodium-loaded@p(N-isopropylacrylamide-co-acrylic acid)) composite hydrogel and stepwise delivery of DS and bFGF in wound repair by tailoring the temperature. Reproduced from ref. 185, 199 and 200 with permission from [Elsevier], 2023. | |
Chronic wounds frequently become difficult to treat because of the biofilm created by bacterial infections. Moreover, there is a limited number of available treatments due to bacterial medication resistance.201 Thus, to overcome these limitations, numerous studies are being conducted to create new types of wound dressing that can deliver treatments on demand, while also allowing for continuous evaluation of the wound status.202 For instance, recently Juan Ma et al. proposed a novel hydrogel-forming microneedle fabricated by integrating polyvinyl alcohol hydrogels with a nitric oxide (NO)-releasing agent and graphene oxide at freezing temperatures.201 This heat-sensitive, light-regulated microneedle patch releases nitric oxide, which is an antimicrobial agent, directly into the wound and prevents bacterial growth.201 In another study by Chen et al., an amphiphilic pentapeptide fabricated pH-switchable antimicrobial supramolecular hydrogel was prepared for effective biofilm elimination and wound healing promotion. Thus, an effective way for treating clinically infected wounds may be provided by the suggested synergistic strategy of reducing biofilm growth and promoting healing.203
Research on nucleic acids including the transfer of silencing RNA (siRNA), microRNA, extrachromosomal plasmid DNA, and oligonucleotides including aptamers and antisense oligonucleotides into specialised cells and affected tissues has recently led to the advancement of new treatment approaches for effective wound care.204 Furthermore, the utilisation of siRNA and miRNA-based wound healing has gained popularity during the past few years among nucleic acid-based wound healing techniques.205 These nucleic acids can regulate injury by focusing on a particular gene associated with irritation, cell motility, angiogenesis, epithelialization, and oxidative stress, among other methods for the repair and regeneration of chronic wounds.206,207 Tezgel et al. prepared collagen loaded with cationic nanostructured lipid carriers nanoparticles and showed that it has extended siRNA release capability and demonstrates in vitro downregulation of ERK-1 protein, which is important in cell proliferation and wound healing.199
Chronic ulcers may heal more quickly with the direct infusion of growth factors, and thus scarring after healing may be completely avoided or drastically diminished.208 These growth factors are secreted by local cells and are crucial for the normal healing of cutaneous wounds; however, injured tissue frequently lacks them severely.208 Furthermore, the creation of a biomembrane with regulated growth factor release using two biomaterials, sericin and alginate, and a platelet derivative was studied by Nardini et al. as a potential advanced medical procedure to promote wound healing.209 In another study by Lin et al., a hydrogel dressing fabricated using alginate and poly(N-isopropylacylamide) was shown to release sequentially diclofenac sodium during the inflammation phase and basic fibroblast growth factors at the tissue development stage.200
Mesenchymal stromal cells (MSCs) and their exosomes have recently attracted increasing attention from academics due to their capacity to produce skin cells such as fibroblasts and keratinocytes as well as their distinct ability to reduce inflammation at the site of wounds.210 Additionally, exosomes (a category of extracellular vesicles) surround a variety of bioactive substances, including lipids, proteins, messenger RNA (mRNA), transfer RNA (tRNA), long non-coding RNAs (lncRNAs), mitochondrial DNA (mtDNA), and microRNAs (miRNAs).211 Recently, in the study by Ferroni et al., human mesenchymal stem cell–loaded 3D printed methacrylated hyaluronic acid patches demonstrated enhanced human fibroblast and human endothelial cell proliferation, migration, angiogenic potential, and expression of certain markers associated with the wound healing process.212 Furthermore, Shiekh et al. reported the development and assessment of an exosome-laden oxygen-releasing antioxidant wound dressing called OxOBand to promote wound closure and skin regeneration in diabetic wounds.213 It was concluded that OxOBand containing adipose-derived stem cell (ADSC) exosomes and antioxidant polyurethane (PUAO) in the form of highly porous cryogels with sustained oxygen releasing capabilities is a considerably more recent treatment approach for improving diabetic wound healing and may open the door for cutting-edge treatment strategies to manage diabetic ulcers (Fig. 14).213
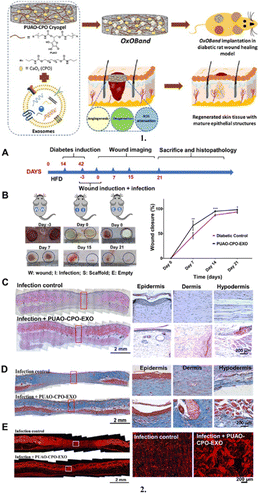 |
| Fig. 14 Exosome-laden oxygen-releasing antioxidant wound dressing-OxOband: (1) Schematic representation of the formation of OxOBand from oxygen-releasing antioxidant PUAO-CPO (polyurethane antioxidant-calcium peroxide) cryogels with adipose-derived stem cells exosomes. 2. Exosome-laden oxygen-releasing antioxidant scaffold enhanced wound closure, regeneration, and prevented infection in infectious diabetic wounds. (A) Experimental design. Rats were fed a high-fat diet, and diabetes was induced by streptozocin. After 4 weeks of diabetic induction, wounds were created on the back of the rat. Wounds were infected with 1 × 108 bacterial count of P. aeruginosa and S. aureus in the ratio of 1 : 1. Infection and ulceration were allowed to develop for 3 days after which wounds were treated with PUAO-CPO-EXO scaffolds, and the contralateral wound was kept empty. Regeneration was observed for 21 days. (B) Representative images of wound closure after 7, 15, and 21 days. PUAO-CPO-EXO-treated wounds showed a faster wound closure compared to the empty wound. Also, PUAO-CPO-EXO scaffolds prevented subsequent infection. (C) Histological H&E-stained tissues demonstrated granulation tissue formation and complete wound closure (scale bar: 2 mm) (left). Magnified images showing epidermal remodelling and formation of mature epithelial structures (scale bar: 200 μm) (right). (D) Masson's trichrome stained wound tissue demonstrated enhanced collagen deposition (blue color) in exosome-laden oxygen-releasing antioxidant scaffold (PUAO-CPO-EXO)-treated animals compared to diabetic infection control (scale bar: 2 mm) (left). The hair follicle and gland formation were also observed (scale bar: 200 μm) (right). (E) Picrosirius red staining showing enhanced collagen remodelling in exosome-laden PUAO-CPO-EXO-treated wounds. Reproduced from ref. 213 with permission from [Elsevier], 2023. | |
Immunomodulating smart dressings
Chronic wounds arise due to dysregulated inflammation, impacting the wound microenvironment.187 Immunomodulatory biomaterials play a vital role by delivering factors such as stem cells, growth hormones, and cytokines, which are crucial for activating inflammatory cells such as macrophages (Fig. 15).214,215 These macrophages exhibit two critical phenotypes, i.e., M1, which is pro-inflammatory and essential for pathogen elimination (classical activation), and M2, which is anti-inflammatory and aids tissue repair (alternative activation).216 Environmental variations prompt macrophages to switch phenotypes, regulated by cytokines and growth factors. However, under conditions such as obesity or diabetes, M1 polarization dominates, leading to prolonged inflammation.217 In diabetic wounds, this imbalance delays healing, highlighting the importance of macrophage coordination in transitioning from pro-inflammatory to anti-inflammatory phenotypes for effective wound repair.205,217 Recently, Zhou et al. proposed a novel spatiotemporal on–off immunomodulatory therapy (SOIT) for the treatment of biofilm-infected diabetic wounds by targeting NLRP3 (nucleotide-binding domain, leucine-rich–containing family, pyrin domain–containing-3) inflammasomes.218 In their study, they created a dual-control release nanocomposite hydrogel (MGO@GM). The hydrogel was fabricated using methacrylate gelatin (Gel-MA), graphene oxide (GO) nanosheets, and mesoporous silicone nanospheres (MSN) loaded with metformin (M). GO was released in the first step under near IR activation. The previously upregulated NLRP3 and the resulting inflammatory response eliminated bacterial biofilm infection in the diabetic wound.218 They also initiated the production of reactive oxygen species (ROS). In the second phase, metformin was released after the degradation of MGO@GM. This helped lower the local hyperglycemia, downregulation of NLRP3, and M2 repolarization of macrophages and tissue repair, all of which reduced inflammation and assisted in the regeneration of wound tissue.218
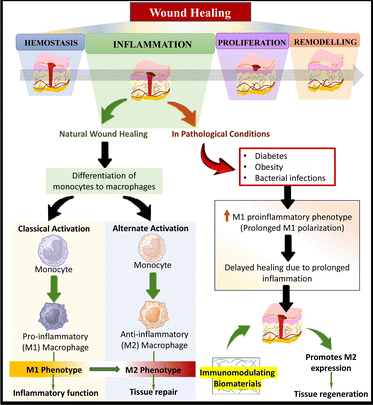 |
| Fig. 15 Mechanism of action of immunomodulating smart dressings. | |
Postoperative scar management dressings
Skin scarring is a common undesirable by-product of healing cutaneous wounds, and because of the development of keloid scars or hypertrophic scar contractures, it can significantly impair functional ability.219 It has long been understood that by imitating the scarless wound healing process of the foetal system, adequate inhibition or control of inflammatory response will be useful for reducing scars.220 For instance, interleukin-6 (IL-6) and tumour necrosis factor alpha (TNF-a), which are pro-inflammatory cytokines that are primarily released by M1 macrophages, can interfere with one or more phases of the wound healing process and cause prolonged inflammation, resulting in additional tissue damage, including tissue fibrosis.221 However, M2 macrophages support tissue regeneration through anti-inflammation, immunological regulation, and tissue remodelling.222,223 In the recent study by Dai et al., many in vitro tests were performed to establish the effectiveness and possible mechanism of umbilical cord mesenchymal stem cell extract in a rat excisional wound and further concluded that this innovative SCE-hydrogel wound dressing can regenerate wound tissue and suppress hypertrophic scar development in a rabbit ear scar model, primarily proving its viability, effectiveness, and adaptability for prospective clinical translation (Fig. 16).224
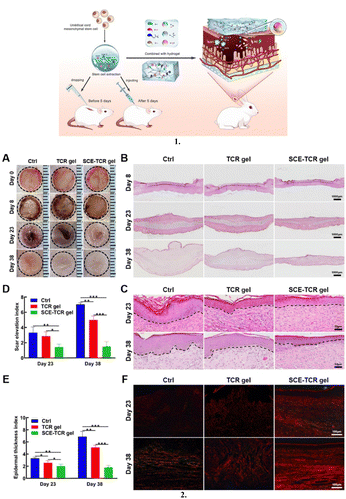 |
| Fig. 16 Postoperative scar management dressings: (1) Schematic illustration of umbilical cord mesenchymal stem cell extract (UC-SCE) preparation and its anti-scarring functional verification in a rat wound model, and the design of a novel hydrogel wound dressing and its efficacy proof for UC-SCE delivery and hypertrophic scar inhibition in a rabbit ear wound model. (2) SCE-TCR (stem cell extract-tandem crosslinking reaction) hydrogel promoted rabbit ear wound tissue regeneration with less scar formation. (A) Gross observation of wound healing and formed hypertrophic scar tissues at postoperative days 0, 8, 23, and 38. (B) Histology of hypertrophic scar formation under different treatments (control, TCR (tandem crosslinking reaction) gel, and SCE-TCR gel) at postoperative days 8, 23, and 38. Magnification × 40 and bar = 1000 μm. (C) Corresponding H&E (hematoxylin and eosin) staining of the epidermis of rabbit ear wounds with different treatments (control, TCR gel, and SCE-TCR gel) at postoperative days 8, 23, and 38. Magnification × 200 and bar = 50 μm. (D) Scar Elevation Index (SEI) and (E) Epidermal Thickness Index (ETI) of hypertrophic scar with different treatments (control, TCR gel, and SCE-TCR gel) at postoperative days 23 and 38. (F) Polarized microscopy images of Sirius red stained wound tissues with different treatments (control, TCR gel, and SCE-TCR gel) at postoperative days 23 and 38. Magnification × 200 and bar = 100 μm. (*p < 0.05, **p < 0.01, and ***p < 0.001). Reproduced from ref. 224 with permission from [Elsevier], 2023. | |
Clinical trials within last 5 years
To obtain information on clinical trials conducted in the last 5 years for the evaluation, comparison and effectiveness of various wound dressings, a search was conducted using the keyword “Wound Dressing” on clinicalTrials.gov.225 The initial search yielded 1325 clinical trials, of which completed trials totaling 412 were screened. The number of on-going clinical trials on wound dressings indicates that there is a developing interest by researchers in the domain of wound care. This is also expressed in the global advanced wound dressing market, which was valued at USD 7.4 billion in 2022 and is expected to expand at a compound annual growth rate (CAGR) of 4.5% from 2023 to 2030.
Most of the 412 completed trials describe wound healing therapies, devices, and conventional dressings and only 26 describe novel dressings including smart dressings, bioactive-supplemented dressings and biopolymer-based dressings used for healing burns and diabetic foot ulcers, which are currently described here (Fig. 17). Some interesting clinical trials include the one entitled ‘Bioactive smart dressings for diabetic foot ulcers: randomized controlled trial’ assessing the efficacy of a cryogel dressing (Trigel) for the treatment of chronic diabetic foot ulcers. Another clinical trial with the title ‘Antimicrobial dressing versus standard dressing in obese women undergoing cesarean delivery’ compared the efficacy of a chlorohexidine gluconate (CHG) impregnated wound dressing (ReliaTect™ Post-Op Dressing) and standard wound care dressing in women undergoing cesarean delivery.
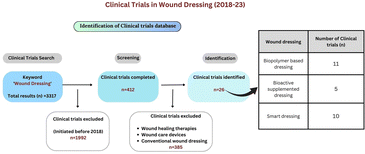 |
| Fig. 17 Clinical trials in wound dressings (2018–23). | |
The restricted number of clinical trials exploring innovative wound dressings, despite their potential advantages over traditional options, can be ascribed to several factors. Firstly, we believe that the formulation development and pre-clinical research in this area is still in its nascent stage. Once the scope and application of such innovative dressings are established, then more frequent clinical trials will be initiated. Further obstacles include obtaining research funding, navigating intricate regulatory processes, and industry reluctance to take risks. All of these may impede the commencement and completion of trials for these novel dressings. Prevailing practices and limited awareness in wound care coupled with the time-intensive development of new dressings, transfer of technology to industry and its scaling-up viability may further contribute to the limited focus on trials for these pioneering approaches. Overcoming these challenges is pivotal to fostering increased research efforts, and ultimately integrating these innovative dressings, which offer distinct therapeutic benefits, into mainstream wound care practices.
Patents of unconventional wound dressings
To study the vast amount of intellectual property and understand the intricate network of innovation woven throughout the wound care sector, a search was conducted using the keywords “Wound dressing” and timeframe 2021 to 2023 on Google Patents,226 The Lens,227 and Espacenet.228 This search yielded a total of 124
547 patents (Fig. 18).
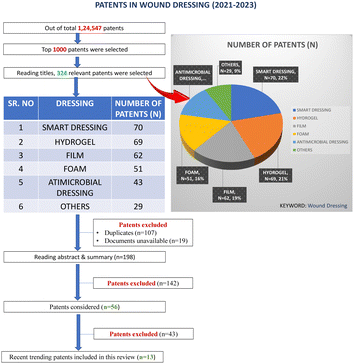 |
| Fig. 18 Patents on wound dressings (2021–2023). | |
The intent was to identify the trend in these patent applications on unconventional wound dressings incorporating contemporary technology and design. To narrow the search and note the latest trend, only the top 1000 patents were selected, among which, the patents related to devices, apparatus, methods, textile materials, composition, wound treatment systems, data collection protocols, wound healing methods, products and techniques in wound dressings were considered out of the scope, and hence excluded. This funnelled down to 324 patents, out of which 107 were duplicates in terms of filing in different zones and details of 19 others were unavailable, while upon reading the abstract and summary, another 142 applications were considered less relevant. Hence, a total of 56 were finally screened.
The trend in these patents revealed a significant focus on smart dressings, accounting for the highest number of patents. This category reflects a growing interest in integrating advanced technologies into wound care solutions. Hydrogel dressings are another prominent area, which is valued for their moisture retention and healing properties. Film dressings, providing a protective yet breathable barrier, are another significant category. A variety of other dressing types was also listed, indicating that versatile advancements are happening in the wound care sector. This underscores the fact that multifunctional solutions are now created in wound management, which are also clinically tested or awaiting physician, patient and regulatory approval.
Expanding on this trend, some of the patents, as mentioned in Table 3, exemplify the innovative strides in wound care technology. For instance, patents are targeting continuous monitoring of wound pH, promoting angiogenesis and collagen synthesis, and accelerating wound healing through electronic circuitry, showcasing the diverse range of advancements in the field. Furthermore, patents facilitating convenient self-health monitoring for diabetic patients and offering eco-friendly wound dressing solutions reflect the ongoing evolution of wound care technology. These patents collectively represent a significant leap forward in addressing various challenges in wound treatment and healing.
Table 3 Recent trends in patents on unconventional wound dressings (2021–2023)
S. no |
Patent number (Ref.) |
Applications and significant features |
Brief product description |
1 |
US11559438B2229 |
Offers improved sensor monitoring of wounds. |
A highly stretchable base with electronic parts like sensors and a range of electronic circuits. |
|
2 |
CN112566592B230 |
Enhanced wound sensor monitoring was observed. |
Devices and procedures for encapsulating a section of a wound dressing with a biocompatible material. |
|
3 |
CN110960352B231 |
Continuous monitoring of changes in wound pH, prevents wound infection. |
The smart dressing is structured as a transparent patch with a pH sensor unit and a wound contact layer and is fabricated by blending and electrospinning the dye-loaded silicon dioxide nanoparticles, polyethylene oxide, and alginate polymer. |
|
4 |
US11351153B2232 |
Exhibits increased antifungal and antibacterial effects of imidazole and activated creatinine respectively. |
A synergistic mixture of an antifungal drug in the imidazole family and activated creatinine is provided in an antimicrobial composition. |
|
5 |
CN113509590B233 |
Better biocompatibility and reliability; promotes angiogenesis and collagen synthesis; lesser chances of infection and scar formation. |
Characterised by encasing mesenchymal stem cell exosomes in hyaluronic acid hydrogel, wherein latter is made by periodic refrigerating and melting hyaluronic acid aqueous solution, followed by crosslinking with carbodiimide hydrochloride and adipic dihydrazide. |
|
6 |
US11540950B2234 |
Assist in reliable wound monitoring and operation. |
Wound dressing is a three-layered structure; an adhesive layer with a distal side and a proximal surface (attached to the wound surface), an absorbent core layer comprising an electrode assembly with several electrodes and sensor points positioned on its distal side, and a top layer. |
|
7 |
CN111686092B235 |
Convenient for self-health monitoring, individual diagnosis, and therapy dressing of diabetic and other wound patients; concurrently release drugs in response to oxidative stimulation and monitor active oxygen in the wounds; high-efficiency drug delivery. |
Wound dressing is obtained by mixing porous silicone particles with graphene quantum dot solution, oscillating, centrifuging, and cleaning to create porous silicone/graphene quantum dot composite particles, and then incorporating these particles into a 0.5–5 mg mL−1 polypeptide drug solution. |
|
8 |
US11484640B2236 |
Accelerates wound healing process; benefits a wide variety of wounds including abdominal, incisional, and open wounds. |
A dressing with electronic circuitry capable of wirelessly communicating a dressing identifier or dressing details to a control system in a pump assembly, can further automatically change operating conditions depending on the information obtained. |
|
9 |
AU2021105727A4237 |
Biocompatible dressing that is non-toxic, simple to apply and remove, prevents dehydration, shows blood compatibility, maintains the appropriate level of moist environment around the wound to allow for gas permeability, and shows antimicrobial properties. |
Biomaterials-based composite materials made of silk fibroin, polyvinyl alcohol, and zinc oxide nanoparticles are synthesised into wound dressing materials. |
|
10 |
CN110755678B238 |
Gelatin antibacterial hydrogel wound dressing was prepared by a safe and eco-friendly method and showed improved bacterial resistance. |
Dressing is made by: (i) preparing a nano-based solution of silver and gelatin; (ii) forming polyvinyl alcohol, chitosan and gelatin mixture; (iii) creating a printing solution; (iv) printing 3D hydrogel on non-woven material; and (v) crosslinking the sample prepared in step (iv). |
|
11 |
CN113262105B239 |
No requirement to charge and detach wound dressing. |
A piezoelectric wound dressing including an encapsulating material, electrodes, a piezoelectric material (inorganic such as BaTiO3, GaN, ZnSnO3 or organic such as polyvinylidene fluoride), a substrate, and an adhesive. |
|
12 |
CN114618006B240 |
Good response to humidity and pressure. |
A flexible sensor for wound intelligent monitoring is characterised by an electrode layer placed on the surface of a functional layer in the dressing; the dressing is made of chitosan hemostatic sponge for the substrate, and the functional layer is made of a citric acid-thermoplastic elastomer polyurethane composite nanofiber for the functional layer. |
|
13 |
CN113289053B241 |
Antibacterial effect, promotes circulation and development of fibroblastic cells, and effective wound healing. |
A hydrogel body, two-dimensional borolene nanosheets loaded in the hydrogel body, and a substance that can react with boric acid to release Zn2+ are the distinguishing features of an antibacterial hydrogel wound dressing. |
Future considerations
Due to the complicated pathophysiological processes involved in wound healing, the use of conventional treatment methods has yielded satisfactory outcomes to date. The dressings available in the market hold certain limitations when applied to burns or chronic wounds.22 For instance, these dressings may not be transparent, thereby resulting in a poor interpretation of the wound status. Furthermore, they can harm the dermal layer, cause scarring, and poor oxygen absorption. In addition, most of these dressings are unaffordable and can cause adverse effects if used with other drugs.23
The statistics and information gathered from searches for patents and clinical studies indicate a significant surge in research and innovation related to wound dressing. The extensive exploration of these domains reveals a strong interest among scientists in advancing this field. The human amniotic membrane (HAM) serves as an effective wound dressing due to its anti-inflammatory and anti-fibrotic properties.242 It promotes epithelialization, inhibits infection through antibacterial activity and preserves moisture for an ideal healing environment.243 Furthermore, HAM contains angiogenic elements that promote the formation of new blood vessels.244 Its compatibility with the human body and observed reduction in pain make it a potential material for wound care.245 Ongoing research continues to improve its application in a variety of wound healing circumstances. Recently, Kafili et al. demonstrated that printable decellularized amniotic membrane-derived hydrogels supplemented with sodium alginate and LAPONITE® nanoplatelets exhibited adequate structural integrity and biocompatibility, underlining their potential for therapeutic applications.246
In wound dressings, peptides and proteins play critical roles in cell signalling, proliferation, and extracellular matrix synthesis.247 They induce angiogenesis, have antibacterial properties, and control inflammation, all of which aid in wound healing.248 These biomolecules also control collagen synthesis, tissue healing, and matrix metalloproteinase activity.249 Zhou et al. developed a natural peptide hydrogel with outstanding efficacy in promoting wound healing in MRSA-infected burn wounds, demonstrating strong in vitro and in vivo antimicrobial effects and biocompatibility.250 It offered a novel approach for designing and synthesizing inherently antimicrobial or multifunctional peptide hydrogels.250 By utilising these diverse functions for improved wound care and quicker healing, peptides and proteins can be incorporated into wound dressings to increase their effectiveness.
Conclusions
Interest in the field of wound dressings has surged in recent years due to the rising prevalence of lifestyle and metabolic diseases such as diabetes and obesity, as well as the aging population worldwide. Consequently, infected and chronic wounds pose significant clinical, social, and economic challenges. Conventional passive dressings have been proven to be insufficient to meet current wound healing demands, emphasizing the need for interactive wound dressings.
The innovative materials and technologies presented above aim to tackle the existing challenges. Employing newer delivery options for extended effects is advocated to promote healing and reduce the need for daily dressing changes. Biosensors can aid in monitoring infections and healing progress to identify the need to change the dressing when indicated. Alternatively, appropriate antibiotics and antimicrobials, including phytochemicals, especially when incorporated in suitable carriers can address resistant infections. Advanced materials such as hydrogels and biological components mimicking natural healing processes are also being explored, together with nanofibrous and extracellular matrix-based scaffolds.
Infected wounds present a serious threat, leading to high morbidity and mortality. Bacteriotherapeutic agents such as probiotics and postbiotics can restore healthy flora, minimizing infection and inflammation to facilitate wound healing. Novel concepts such as vitamin enrichment at the wound site and the use of stem cells for tissue repair and regeneration were also discussed. This comprehensive analysis of clinical studies conducted over the past five years, together with recent patents, provides valuable insights into the dynamic trends in interactive wound dressing technology. This analysis is indispensable for the scientific community, offering crucial understanding of the efficacy, market potential, and patient needs to develop tailored solutions.
Author contributions
Radhika Yadav and Rohtash were involved in conceptualization, data curation, methodology, writing – original draft, writing – review & editing of the manuscript. Muskan was involved in conceptualization, data curation, and writing – original draft. Bakr Ahmed contributed to conceptualization, methodology, visualization, writing – review & editing. Kamal Dua, Monica Gulati, Pushvinder Jit Singh, Sachin Singh, Suneel Kumar, and Rohan M. Shah were involved in visualization, writing – review & editing. Parneet Kaur Deol, and Indu Pal Kaur worked on conceptualization, methodology, supervision, and writing – review & editing. All authors have read and approved the final manuscript.
Conflicts of interest
There are no conflicts to declare.
Acknowledgements
This research was supported by Rutgers Busch Biomedical Grant 2021 (# AWD00009147).
References
- M. E. Okur, I. D. Karantas, Z. Şenyiğit, N. Üstündağ Okur and P. I. Siafaka, Recent trends on wound management: New therapeutic choices based on polymeric carriers, Asian J. Pharm. Sci., 2020, 15, 661–684 CrossRef PubMed.
- WHO urges more effective prevention of injuries and violence causing 1 in 12 deaths worldwide. https://www.who.int/news/item/29-11-2022-who-urges-more-effective-prevention-of-injuries-and-violence--causing-1-in-12-deaths-worldwide.
- Cancer. https://www.who.int/news-room/fact-sheets/detail/cancer.
- S. Dhivya, V. V. Padma and E. Santhini, Wound dressings – A review, Biomedicine, 2015, 5, 24–28 CrossRef PubMed.
- M. Rodrigues, N. Kosaric, C. A. Bonham and G. C. Gurtner, Wound healing: A cellular perspective, Physiol. Rev., 2019, 99, 665–706 CrossRef CAS PubMed.
- H. N. Wilkinson and M. J. Hardman, Wound healing: Cellular mechanisms and pathological outcomes, Open Biol., 2023, 10, 341–370 Search PubMed.
- E. Rezvani Ghomi, S. Khalili, S. Nouri Khorasani, R. Esmaeely Neisiany and S. Ramakrishna, Wound dressings: Current advances and future directions, J. Appl. Polym. Sci., 2019, 136, 47738 CrossRef.
- E. E. Tudoroiu,
et al., An Overview of Cellulose Derivatives-Based Dressings for Wound-Healing Management, Pharmaceuticals, 2021, 14, 1215 CrossRef CAS PubMed.
- B. Hawthorne,
et al., Enhancing wound healing dressing development through interdisciplinary collaboration, J. Biomed. Mater. Res., Part B, 2021, 109, 1967–1985 CrossRef CAS PubMed.
- F. Yang, X. Bai, X. Dai and Y. Li, The biological processes during wound healing, Regener. Med., 2021, 16, 373–390 CrossRef CAS PubMed.
- A. Oliveira, S. Simões, A. Ascenso and C. P. Reis, Therapeutic advances in wound healing, J. Dermatol. Treat., 2022, 33, 2–22 CrossRef CAS PubMed.
- H. A. Wallace, B. M. Basehore and P. M. Zito, Wound Healing Phases, StatPearls, 2023 Search PubMed.
- M. Minsart, S. Van Vlierberghe, P. Dubruel and A. Mignon, Commercial wound dressings for the treatment of exuding wounds: an in-depth physico-chemical comparative study, Burns Trauma, 2022, 10, tkac024 CrossRef PubMed.
- R. Dong and B. Guo, Smart wound dressings for wound healing, Nano Today, 2021, 41, 101290 CrossRef CAS.
- A. G. Niculescu and A. M. Grumezescu, An Up-to-Date Review of Biomaterials Application in Wound Management, Polymers, 2022, 14, 421 CrossRef CAS PubMed.
- A. E. Stoica, C. Chircov and A. M. Grumezescu, Nanomaterials for Wound Dressings: An Up-to-Date Overview, Molecules, 2020, 25, 2699 CrossRef CAS PubMed.
- E. Chamanga, Effectively managing wound exudate, Br. J. Community Nurs., 2015, 20, S8–S10 CrossRef PubMed.
- A. Bianchera, O. Catanzano, J. Boateng and L. Elviri, The Place of Biomaterials in Wound Healing, Ther. Dressings Wound Healing Appl., 2020, 2, 337–366 Search PubMed.
- B. Rogina-Car, S. Kovačević, I. Schwarz and K. Dimitrovski, Microbial Barrier Properties of Cotton Fabric—Influence of Weave Architecture, Polymers, 2020, 12, 1570 CrossRef CAS PubMed.
- I. R. Sweeney, M. Miraftab and G. Collyer, A critical review of modern and emerging absorbent dressings used to treat exuding wounds, Int. Wound J., 2012, 9, 601 CrossRef PubMed.
- C. Shi,
et al., Selection of Appropriate Wound Dressing for Various Wounds, Front. Bioeng. Biotechnol., 2020, 8, 511530 Search PubMed.
- A. Sood, M. S. Granick and N. L. Tomaselli, Wound Dressings and Comparative Effectiveness Data, Adv. Wound Care, 2014, 3, 511 CrossRef PubMed.
- R. Laurano, M. Boffito, G. Ciardelli and V. Chiono, Wound dressing products: A translational investigation from the bench to the market, Eng. Regen., 2022, 3, 182–200 Search PubMed.
- J. A. Ataide,
et al., Nanotechnology-Based Dressings for Wound Management, Pharmaceuticals, 2022, 15, 1286 CrossRef CAS PubMed.
- V. Brumberg, T. Astrelina, T. Malivanova and A. Samoilov, Modern Wound Dressings: Hydrogel Dressings, Biomedicines, 2021, 9, 1235 CrossRef CAS PubMed.
- K. Nuutila and E. Eriksson, Moist Wound Healing with Commonly Available Dressings, Adv. Wound Care, 2021, 10, 685 CrossRef PubMed.
- M. Alhajj, P. Bansal and A. Goyal, Physiology, Granulation Tissue, StatPearls, 2022 Search PubMed.
- C. D. Weller, V. Team and G. Sussman, First-Line Interactive Wound Dressing Update: A Comprehensive Review of the Evidence, Front. Pharmacol., 2020, 11, 495485 Search PubMed.
- A. Brites,
et al., Fabrication of antibacterial and biocompatible 3D printed Manuka-Gelatin based patch for wound healing applications, Int. J. Pharm., 2023, 632, 122541 CrossRef CAS PubMed.
- M. Sharifuzzaman,
et al., Smart bandage with integrated multifunctional sensors based on MXene-functionalized porous graphene scaffold for chronic wound care management, Biosens. Bioelectron., 2020, 169, 112637 CrossRef CAS PubMed.
- S. Chhabra, N. Chhabra, A. Kaur and N. Gupta, Wound Healing Concepts in Clinical Practice of OMFS, J. Maxillofac. Oral Surg., 2017, 16, 403 CrossRef PubMed.
- C. Martin,
et al., Current trends in the development of wound dressings, biomaterials and devices, Pharm. Pat. Anal., 2013, 2, 341–359 CrossRef CAS PubMed.
- C. Weller and G. Sussman, Wound Dressings Update, J. Pharm. Pract. Res., 2006, 36, 318–324 CrossRef.
- K. A. Al-Gharibi, S. Sharstha and M. A. Al-Faras, Cost-Effectiveness of Wound Care: A concept analysis, Sultan Qaboos Univ. Med. J., 2018, 18, e433 CrossRef PubMed.
- K. J. B. Kus and E. S. Ruiz, Wound Dressings – A Practical Review, Curr. Dermatol. Rep., 2020, 9, 298–308 CrossRef.
- D. Queen, H. Orsted, H. Sanada and G. Sussman, A dressing history, Int. Wound J., 2004, 1, 59–77 CrossRef PubMed.
- L. van Rijswijk, Ingredient-based wound dressing classification: a paradigm that is passe and in need of replacement, J. Wound Care, 2013, 15, 11–14 CrossRef PubMed.
- A. F. Falabella, Debridement and wound bed preparation, Dermatol. Ther., 2006, 19, 317–325 CrossRef PubMed.
- J. S. Boateng, K. H. Matthews, H. N. E. Stevens and G. M. Eccleston, Wound healing dressings and drug delivery systems: A review, J. Pharm. Sci., 2008, 97, 2892–2923 CrossRef CAS PubMed.
- R. Xu,
et al., Controlled water vapor transmission rate promotes wound-healing via wound re-epithelialization and contraction enhancement, Sci. Rep., 2016, 6, 1–12 CrossRef PubMed.
- E. R. Ghomi, M. Niazi and S. Ramakrishna, The evolution of wound dressings: From traditional to smart dressings, Polym. Adv. Technol., 2023, 34, 520–530 CrossRef.
- S. Alven, X. Nqoro and B. A. Aderibigbe, Polymer-Based Materials Loaded with Curcumin for Wound Healing Applications, Polymers, 2020, 12, 2286 CrossRef CAS PubMed.
- Y. P. Afsharian and M. Rahimnejad, Bioactive electrospun scaffolds for wound healing applications: A comprehensive review, Polym. Test., 2021, 93, 106952 CrossRef CAS.
- V. Vivcharenko and A. Przekora, Modifications of Wound Dressings with Bioactive Agents to Achieve Improved Pro-Healing Properties, Appl. Sci., 2021, 11, 4114 CrossRef CAS.
- I. Gardikiotis, F. D. Cojocaru, C. T. Mihai, V. Balan and G. Dodi, Borrowing the Features of Biopolymers for Emerging Wound Healing Dressings: A Review, Int. J. Mol. Sci., 2022, 23, 8778 CrossRef CAS PubMed.
- N. Tiwari,
et al., Recent progress in polymeric biomaterials and their potential applications in skin regeneration and wound care management, J. Drug Delivery Sci. Technol., 2023, 82, 104319 CrossRef CAS.
- P. Aramwit, Introduction to biomaterials for wound healing, Wound Healing Biomater., 2016, 2, 3–38 Search PubMed.
- M. M. Farag, Recent trends on biomaterials for tissue regeneration applications: review, J. Mater. Sci., 2023, 58, 527–558 CrossRef CAS.
-
A. Spoială, C.-I. Ilie, D. Ficai and A. Ficai, Biomaterials, in Biomaterials and Tissue Engineering. Stem Cell Bio Regen Med 74, ed. O. Gunduz, C. Egles, R. A. Pérez, D. Ficai and C. B. Ustundag, 2023, pp. 97–129 Search PubMed.
- S. Prete,
et al., Natural and Synthetic Polymeric Biomaterials for Application in Wound Management, J. Funct. Biomater., 2023, 14, 455 CrossRef CAS PubMed.
- R. A. Hortensius and B. A. C. Harley, Naturally derived biomaterials for addressing inflammation in tissue regeneration, Exp. Biol. Med., 2016, 241, 1015 CrossRef CAS PubMed.
- Y. Liang, Y. Liang, H. Zhang and B. Guo, Antibacterial biomaterials for skin wound dressing, Asian J. Pharm. Sci., 2022, 17, 353–384 CrossRef PubMed.
- M. Mir,
et al., Synthetic polymeric biomaterials for wound healing: a review, Prog. Biomater., 2018, 7, 1 CrossRef CAS PubMed.
- R. Naomi, H. Bahari, P. M. Ridzuan and F. Othman, Natural-Based Biomaterial for Skin Wound Healing (Gelatin vs. Collagen): Expert Review, Polymers, 2021, 13, 2319 CrossRef CAS PubMed.
- D. Chicharro-Alcántara,
et al., Platelet Rich Plasma: New Insights for Cutaneous Wound Healing Management, J. Funct. Biomater., 2018, 9, 10 CrossRef PubMed.
- K. Raziyeva,
et al., Immunology of Acute and Chronic Wound Healing, Biomolecules, 2021, 11, 700 CrossRef CAS PubMed.
- R. Z. Murray, Z. E. West, A. J. Cowin and B. L. Farrugia, Development and use of biomaterials as wound healing therapies, Burns Trauma, 2019, 7, 2 Search PubMed.
- W. V. Sathyaraj,
et al., Therapeutic Efficacy of Polymeric Biomaterials in Treating Diabetic Wounds—An Upcoming Wound Healing Technology, Polymers, 2023, 15, 1205 CrossRef CAS PubMed.
- H. M. Nguyen, T. T. Ngoc Le, A. T. Nguyen, H. N. Thien Le and T. T. Pham, Biomedical materials for wound dressing: recent advances and applications, RSC Adv., 2023, 13, 5509–5528 RSC.
- V. Brumberg, T. Astrelina, T. Malivanova and A. Samoilov, Modern Wound Dressings: Hydrogel Dressings, Biomedicines, 2021, 9, 1235 CrossRef CAS PubMed.
- K. Nuutila and E. Eriksson, Moist Wound Healing with Commonly Available Dressings, Adv. Wound Care, 2021, 10, 685 CrossRef PubMed.
- P. Trucillo and E. Di Maio, Classification and Production of Polymeric Foams among the Systems for Wound Treatment, Polymers, 2021, 13, 1608 CrossRef CAS PubMed.
- P. Mistry, S. Kumar, R. S. Schloss, F. Berthiaume and N. A. Langrana, Chitosan-polygalacturonic acid complex dressing improves diabetic wound healing and hair growth in diabetic mice, Biochem. Biophys. Res. Commun., 2024, 696, 149502 CrossRef CAS PubMed.
- A. Ahmed and J. Boateng, Calcium alginate-based antimicrobial film dressings for potential healing of infected foot ulcers, Ther. Delivery, 2018, 9, 185–204 CrossRef CAS PubMed.
- R. Xu,
et al., Controlled water vapor transmission rate promotes wound-healing via wound re-epithelialization and contraction enhancement, Sci. Rep., 2016, 6, 1–12 CrossRef PubMed.
- I. Savencu, S. Iurian, A. Porfire, C. Bogdan and I. Tomuta, Review of advances in polymeric wound dressing films, React. Funct. Polym., 2021, 168, 105059 CrossRef CAS.
- J. G. Hodge, D. S. Zamierowski, J. L. Robinson and A. J. Mellott, Evaluating polymeric biomaterials to improve next generation wound dressing design, Biomater. Res., 2022, 26, 1–39 CrossRef PubMed.
-
O. Sarheed
et al., Antimicrobial Dressings for Improving Wound Healing. Wound Healing – New insights into Ancient Challenges, 2016, pp. 373–98 Search PubMed.
- V. Jones, J. E. Grey and K. G. Harding, Wound dressings, BMJ, 2006, 332, 777–780 CrossRef PubMed.
- K. C. Broussard and J. G. Powers, Wound dressings: Selecting the most appropriate type, Am. J. Clin. Dermatol., 2013, 14, 449–459 CrossRef PubMed.
- K. Nuutila and E. Eriksson, Moist Wound Healing with Commonly Available Dressings, Adv. Wound Care, 2021, 10, 685–698 CrossRef PubMed.
- B. Joergensen, S. Blaise and A. S. Svensson, A randomised, open-label, parallel-group, multicentre, comparative study to compare the efficacy and safety of Exufiber® with Aquacel® Extra™ dressings in exuding venous and mixed aetiology leg ulcers, Int. Wound J., 2022, 19, 22–38 CrossRef PubMed.
- C. H. Lin, K. Hsia, H. Ma, H. Lee and J. H. Lu,
In Vivo Performance of Decellularized Vascular Grafts: A Review Article, Int. J. Mol. Sci., 2018, 19, 2101 CrossRef PubMed.
- M. Parmaksiz, A. Dogan, S. Odabas, A. E. Elçin and Y. M. Elçin, Clinical applications of decellularized extracellular matrices for tissue engineering and regenerative medicine, Biomed. Mater., 2016, 11, 022003 CrossRef PubMed.
- H. A. Owida,
et al., Recent Applications of Electrospun Nanofibrous Scaffold in Tissue Engineering, Appl. Bionics Biomech., 2022, 2022, 1953861 Search PubMed.
- C. N. M. Ryan,
et al., An academic, clinical and industrial update on electrospun, additive manufactured and imprinted medical devices, Expert Rev. Med. Devices, 2015, 12, 601–612 CrossRef CAS PubMed.
- S. Jiang,
et al., Breathable, antifreezing, mechanically skin-like hydrogel textile wound dressings with dual antibacterial mechanisms, Bioact. Mater., 2023, 21, 313–323 CAS.
- S. Alven, S. Peter and B. A. Aderibigbe, Polymer-Based Hydrogels Enriched with Essential Oils: A Promising Approach for the Treatment of Infected Wounds, Polymers, 2022, 14, 3772 CrossRef CAS PubMed.
- A. Mohandas, P. T. Sudheesh Kumar, B. Raja, V. K. Lakshmanan and R. Jayakumar, Exploration of alginate hydrogel/nano zinc oxide composite bandages for infected wounds, Int. J. Nanomed., 2015, 10, 53–66 CAS.
- N. H. Thang, T. B. Chien and D. X. Cuong, Polymer-Based Hydrogels Applied in Drug Delivery: An Overview, Gels, 2023, 9, 523 CrossRef CAS PubMed.
- A. U. Vakil, M. Ramezani and M. B. B. Monroe, Antimicrobial Shape Memory Polymer Hydrogels for Chronic Wound Dressings, ACS Appl. Bio Mater., 2022, 5, 5199–5209 CrossRef CAS PubMed.
- C. Chircov,
et al., Chitosan-Dextran-Glycerol Hydrogels Loaded with Iron Oxide Nanoparticles for Wound Dressing Applications, Pharmaceutics, 2022, 14, 2620 CrossRef CAS PubMed.
- S. Bashir,
et al., Fundamental Concepts of Hydrogels: Synthesis, Properties, and Their Applications, Polymers, 2020, 12, 1–60 Search PubMed.
- M. Bustamante-Torres,
et al., Hydrogels Classification According to the Physical or Chemical Interactions and as Stimuli-Sensitive Materials, Gels, 2021, 7, 182 CrossRef CAS PubMed.
- H. Chamkouri, A Review of Hydrogels, Their Properties and Applications in Medicine, Am. J. Biomed. Sci. Res., 2021, 11, 485–493 CrossRef.
- Y. Yang,
et al., H-Bonding Supramolecular Hydrogels with Promising Mechanical Strength and Shape Memory Properties for Postoperative Antiadhesion Application, ACS Appl. Mater. Interfaces, 2020, 12, 34161–34169 CrossRef CAS PubMed.
- X. Zhao,
et al., Injectable Antiswelling and High-Strength Bioactive Hydrogels with a Wet Adhesion and Rapid Gelling Process to Promote Sutureless Wound Closure and Scar-free Repair of Infectious Wounds, ACS Nano, 2023, 17, 22015–22034 CrossRef PubMed.
- S. Jin,
et al., Progress of Hydrogel Dressings with Wound Monitoring and Treatment Functions, Gels, 2023, 9, 694 CrossRef CAS PubMed.
- P. Trucillo and E. Di Maio, Classification and Production of Polymeric Foams among the Systems for Wound Treatment, Polymers, 2021, 13, 1608 CrossRef CAS PubMed.
- H. M. Nguyen, T. T. Ngoc Le, A. T. Nguyen, H. N. Thien Le and T. T. Pham, Biomedical materials for wound dressing: recent advances and applications, RSC Adv., 2023, 13, 5509 RSC.
- S. P. Sonar, N. A. Kumar, N. Ali, A. Moharana and S. Centre, A comprehensive review on wound dressing usage in clinical settings, Int. J. Surg. Med., 2022, 8, 16–26 Search PubMed.
- H. Vermeulen, D. T. Ubbink, A. Goossens, R. De Vos and D. A. Legemate, Systematic review of dressings and topical agents for surgical wounds healing by secondary intention, Br. J. Surg., 2005, 92, 665–672 CrossRef CAS PubMed.
-
A. Nussinovitch, Hydrocolloids for coatings and adhesives. Handbook
of Hydrocolloids, Woodhead Publishing, 2nd edn, 2009, pp. 760–806 Search PubMed.
- M. Uzun, A review of wound management materials, J. Text. Eng. Fash. Technol., 2018, 4, 53–59 Search PubMed.
- I. R. Sweeney, M. Miraftab and G. Collyer, A critical review of modern and emerging absorbent dressings used to treat exuding wounds, Int. Wound J., 2012, 9, 601–612 CrossRef PubMed.
- Y. Barnea, J. Weiss and E. Gur, A review of the applications of the hydrofiber dressing with silver (Aquacel Ag®) in wound care, Ther. Clin. Risk Manage., 2010, 6, 21 CAS.
- M. Walker, S. Lam, D. Pritchard and C. A. Cochrane, Biophysical properties of a Hydrofiber® cover dressing, Wounds, 2010, 6, 9 Search PubMed.
- B. A. Aderibigbe and B. Buyana, Alginate in Wound Dressings, Pharmaceutics, 2018, 10, 42 CrossRef PubMed.
- A. Froelich,
et al., Alginate-Based Materials Loaded with Nanoparticles in Wound Healing, Pharmaceutics, 2023, 15, 1142 CrossRef CAS PubMed.
- P. Kolimi, S. Narala, D. Nyavanandi, A. A. A. Youssef and N. Dudhipala, Innovative Treatment Strategies to Accelerate Wound Healing: Trajectory and Recent Advancements, Cells, 2022, 11, 2439 CrossRef CAS PubMed.
- M. C. Cramer and S. F. Badylak, Extracellular Matrix-Based Biomaterials and Their Influence Upon Cell Behavior, Ann. Biomed. Eng., 2020, 48, 2132–2153 CrossRef PubMed.
- S. T. LoPresti and B. N. Brown, Effect of source animal age upon macrophage response to extracellular matrix biomaterials, J. Immunol. Regener. Med., 2018, 1, 57–66 CrossRef PubMed.
- Y. Efraim,
et al., 3D Structure and Processing Methods Direct the Biological Attributes of ECM-Based Cardiac Scaffolds, Sci. Rep., 2019, 9, 1–13 CrossRef CAS PubMed.
- A. Neishabouri, A. Soltani Khaboushan, F. Daghigh, A. M. Kajbafzadeh and M. Majidi Zolbin, Decellularization in Tissue Engineering and Regenerative Medicine: Evaluation, Modification, and Application Methods, Front. Bioeng. Biotechnol., 2022, 10, 805299 CrossRef PubMed.
- A. D. McInnes, M. A. J. Moser and X. Chen, Preparation and Use of Decellularized Extracellular Matrix for Tissue Engineering, J. Funct. Biomater., 2022, 13, 240 CrossRef CAS PubMed.
- J. Bhoopathy,
et al., Sericin/Human Placenta-Derived Extracellular Matrix Scaffolds for Cutaneous Wound Treatment—Preparation, Characterization, In Vitro and In Vivo Analyses, Pharmaceutics, 2023, 15, 362 CrossRef CAS PubMed.
- Z. Jiang,
et al., Nanofiber Scaffolds as Drug Delivery Systems Promoting Wound Healing, Pharmaceutics, 2023, 15, 1829 CrossRef CAS PubMed.
-
G. Rivero, M. D. Popov Pereira da Cunha, P. C. Caracciolo and G. A. Abraham, Nanofibrous scaffolds for skin tissue engineering and wound healing applications, Tissue Engineering Using Ceramics and Polymers, Woodhead Publishing, 3rd edn, 2022, pp. 645–681 Search PubMed.
-
L. Bacakova
et al., Nanofibrous Scaffolds for Skin Tissue Engineering and Wound Healing Based on Synthetic Polymers, Applications of Nanobiotechnology, IntechOpen, 2020, p. 1 Search PubMed.
- W. Qian,
et al., Dual Drug Release Electrospun Core-Shell Nanofibers with Tunable Dose in the Second Phase, Int. J. Mol. Sci., 2014, 15, 774–786 CrossRef PubMed.
- S. Anjum,
et al., Electrospun Biomimetic Nanofibrous Scaffolds: A Promising Prospect for Bone Tissue Engineering and Regenerative Medicine, Int. J. Mol. Sci., 2022, 23, 9206 CrossRef CAS PubMed.
- M. A. Fernandez-Yague,
et al., Biomimetic approaches in bone tissue engineering: Integrating biological and physicomechanical strategies, Adv. Drug Delivery Rev., 2015, 84, 1–29 CrossRef CAS PubMed.
- G. Kumar, F. G. Khan, M. I. Abro, U. Aftab and A. W. Jatoi, Development of cellulose acetate/CuO/AgNP nanofibers based effective antimicrobial wound dressing, Compos. Commun., 2023, 39, 101550 CrossRef.
- Z. Hussain, H. E. Thu, A. N. Shuid, H. Katas and F. Hussain, Recent Advances in Polymer-based Wound Dressings for the Treatment of Diabetic Foot Ulcer: An Overview of State-of-the-art, Curr. Drug Targets, 2017, 19, 527–550 CrossRef PubMed.
- A. G. Goswami, S. Basu, T. Banerjee and V. K. Shukla, Biofilm and wound healing: from bench to bedside, Eur. J. Med. Res., 2023, 28, 1–18 CrossRef PubMed.
- Y. Jiang, M. Geng and L. Bai, Targeting Biofilms Therapy: Current Research Strategies and Development Hurdles, Microorganisms, 2020, 8, 1–34 Search PubMed.
- D. G. Metcalf and P. G. Bowler, Biofilm delays wound healing: A review of the evidence, Burns Trauma, 2013, 1, 5–12 CrossRef PubMed.
- M. Walker, D. Metcalf, D. Parsons and P. Bowler, A real-life clinical evaluation of a next-generation antimicrobial dressing on acute and chronic wounds, J. Wound Care, 2014, 24, 11–22 CrossRef PubMed.
- X. Wang,
et al., Collagen hydrogel with multiple antimicrobial mechanisms as anti-bacterial wound dressing, Int. J. Biol. Macromol., 2023, 232, 123413 CrossRef CAS PubMed.
- A. Kwiatkowska,
et al., Composite Membrane Dressings System with Metallic Nanoparticles as an Antibacterial Factor in Wound Healing, Membranes, 2022, 12, 215 CrossRef CAS PubMed.
- M. Konop, T. Damps, A. Misicka and L. Rudnicka, Certain Aspects of Silver and Silver Nanoparticles in Wound Care: A Minireview, J. Nanomater., 2016, 2016, 7614753 Search PubMed.
- J. Fong and F. Wood, Nanocrystalline silver dressings in wound management: a review, Int. J. Nanomed., 2006, 1, 441 CrossRef CAS PubMed.
- C. Doherty,
et al., Anti-biofilm effects and healing promotion by silver oxynitrate-based dressings, Sci. Rep., 2023, 13, 2014 CrossRef CAS PubMed.
- S. K. Mishra, D. S. Mary and S. Kannan, Copper incorporated microporous chitosan-polyethylene glycol hydrogels loaded with naproxen for effective drug release and anti-infection wound dressing, Int. J. Biol. Macromol., 2017, 95, 928–937 CrossRef CAS PubMed.
- P. H. Lin,
et al., Zinc in Wound Healing Modulation, Nutrients, 2017, 10, 16 CrossRef PubMed.
- E. Melamed,
et al., Healing of Chronic Wounds by Copper Oxide-Impregnated Wound Dressings—Case Series, Medicina, 2021, 57, 296 CrossRef PubMed.
- G. Borkow,
et al., Molecular mechanisms of enhanced wound healing by copper oxide-impregnated dressings, Wound Repair Regen., 2010, 18, 266–275 CrossRef PubMed.
- A. Das,
et al., Endothelial Antioxidant-1: a Key Mediator of Copper-dependent Wound Healing in vivo, Sci. Rep., 2016, 6, 1–16 CrossRef PubMed.
- N. Philips,
et al., Beneficial Regulation of Fibrillar Collagens, Heat Shock Protein-47, Elastin Fiber Components, Transforming Growth Factor-β1, Vascular Endothelial Growth Factor and Oxidative Stress Effects by Copper in Dermal Fibroblasts, Connect. Tissue Res., 2012, 53, 373–378 CrossRef CAS PubMed.
- Z. Ahmed, A. Briden, S. Hall and R. A. Brown, Stabilisation of cables of fibronectin with micromolar concentrations of copper: in vitro cell substrate properties, Biomaterials, 2004, 25, 803–812 CrossRef CAS PubMed.
- F. Yousefian,
et al., Antimicrobial Wound Dressings: A Concise Review for Clinicians, Antibiotics, 2023, 12, 1434 CrossRef CAS PubMed.
- C. Guo,
et al., Polyhexamethylene biguanide chemically modified cotton with desirable hemostatic, inflammation-reducing, intrinsic antibacterial property for infected wound healing, Chin. Chem. Lett., 2022, 33, 2975–2981 CrossRef CAS.
- T. S. C. Mana,
et al., Preliminary analysis of the antimicrobial activity of a postoperative wound dressing containing chlorhexidine gluconate against methicillin-resistant Staphylococcus aureus in an in vivo porcine incisional wound model, Am. J. Infect. Control, 2019, 47, 1048–1052 CrossRef PubMed.
- R. G. Sibbald and J. A. Elliott, The role of Inadine in wound care: a consensus document, Int. Wound J., 2017, 14, 316–321 CrossRef PubMed.
- P. J. Alves,
et al., Update on the role of antiseptics in the management of chronic wounds with critical colonisation and/or biofilm, Int. Wound J., 2021, 18, 342–358 CrossRef PubMed.
- S. Argel,
et al., Assessment of Bacterial Nanocellulose Loaded with Acetylsalicylic Acid or Povidone–Iodine as Bioactive Dressings for Skin and Soft Tissue Infections, Pharmaceutics, 2022, 14, 1661 CrossRef CAS PubMed.
- H. Fjeld and E. Lingaas, Polyhexanide – Safety and efficacy as an antiseptic, Tidsskr. Nor. Laegeforen., 2016, 136, 707–711 Search PubMed.
- S. Sowlati-Hashjin, M. Karttunen and P. Carbone, Insights into the Polyhexamethylene Biguanide (PHMB) Mechanism of Action on Bacterial Membrane and DNA: A Molecular Dynamics Study, J. Phys. Chem. B, 2020, 124, 4487–4497 CrossRef CAS PubMed.
- A. Kapanya,
et al., Synthesis of polymeric hydrogels incorporating chlorhexidine gluconate as antibacterial wound dressings, J. Biomater. Sci., Polym. Ed., 2020, 31, 895–909 CrossRef CAS PubMed.
- K. Schmidt, C. Estes, A. McLaren and M. J. Spangehl, Chlorhexidine Antiseptic Irrigation Eradicates Staphylococcus epidermidis From Biofilm: An In Vitro Study, Clin. Orthop. Relat. Res., 2018, 476, 648 CrossRef PubMed.
- J. S. Kim,
et al., Development of guar gum-based dual-layer wound dressing containing Lactobacillus plantarum: Rapid recovery and mechanically flexibility, Int. J. Biol. Macromol., 2022, 221, 1572–1579 CrossRef CAS PubMed.
- M. Saberian,
et al., The Effect of Bifidobacterium Bifidum Supernatant and Cell Mass on the Proliferation Potential of Rat Bone Marrow-Derived Stromal Cells, Iran. J. Med. Sci., 2020, 45, 269–276 Search PubMed.
- C. Chircov,
et al., Chitosan-Dextran-Glycerol Hydrogels Loaded with Iron Oxide Nanoparticles for Wound Dressing Applications, Pharmaceutics, 2022, 14, 2620 CrossRef CAS PubMed.
- P. F. Hillman, C. Lee and S. J. Nam, Microbial Natural Products with Wound-Healing Properties, Processes, 2022, 11, 30 CrossRef.
- A. Bazjoo, P. Jafari, A. Marjani and N. Akbari, Effect of cell-free supernatant of Bifidobacterium bifidum combined with chitosan biodegradable film on full thickness wound healing in rats, Physiol. Pharmacol., 2022, 26, 468–479 Search PubMed.
- M. Barchitta,
et al., Nutrition and Wound Healing: An Overview Focusing on the Beneficial Effects of Curcumin, Int. J. Mol. Sci., 2019, 20, 1119 CrossRef CAS PubMed.
- G. Isopencu,
et al., Bacterial Cellulose—Carboxymethylcellulose Composite Loaded with Turmeric Extract for Antimicrobial Wound Dressing Applications, Int. J. Mol. Sci., 2023, 24, 1719 CrossRef CAS PubMed.
- A. M. Scagnelli, Therapeutic Review: Manuka Honey, J. Exot. Pet. Med., 2016, 25, 168–171 CrossRef.
- P. H. S. Kwakman,
et al., How honey kills bacteria, FASEB J., 2010, 24, 2576–2582 CrossRef CAS PubMed.
- R. Kateel, P. Adhikari, A. J. Augustine and S. Ullal, Topical honey for the treatment of diabetic foot ulcer: A systematic review, Complement Ther. Clin. Pract., 2016, 24, 130–133 CrossRef PubMed.
- A. Rezaei, H. Ehtesabi and S. Ebrahimi, Incorporation of Saqez essential oil into polyvinyl alcohol/chitosan bilayer hydrogel as a potent wound dressing material, Int. J. Biol. Macromol., 2023, 226, 383–396 CrossRef CAS PubMed.
- Y. X. Seow, C. R. Yeo, H. L. Chung and H. G. Yuk, Plant Essential Oils as Active Antimicrobial Agents, Crit. Rev. Food Sci. Nutr., 2014, 54, 625–644 CrossRef CAS PubMed.
- D. Simões,
et al., Recent advances on antimicrobial wound dressing: A review, Eur. J. Pharm. Biopharm., 2018, 127, 130–141 CrossRef PubMed.
- W. Kim, Y. Gwon, S. Park, H. Kim and J. Kim, Therapeutic strategies of three-dimensional stem cell spheroids and organoids for tissue repair and regeneration, Bioact. Mater., 2023, 19, 50–74 CAS.
- R. Hazrati, S. Davaran and Y. Omidi, Bioactive functional scaffolds for stem cells delivery in wound healing and skin regeneration, React. Funct. Polym., 2022, 174, 105233 CrossRef CAS.
- P. Das, S. Manna, S. Roy, S. K. Nandi and P. Basak, Polymeric biomaterials-based tissue engineering for wound healing: a systemic review, Burns Trauma, 2023, 11, 58 Search PubMed.
- X. Shao, I. N. Taha, K. R. Clauser, Y. Gao (Tom) and A. Naba, MatrisomeDB: the ECM-protein knowledge database, Nucleic Acids Res., 2020, 48, D1136–D1144 CrossRef CAS PubMed.
- Q. L. Loh and C. Choong, Three-Dimensional Scaffolds for Tissue Engineering Applications: Role of Porosity and Pore Size, Tissue Eng., Part B, 2013, 19, 485–502 CrossRef CAS PubMed.
- C. Mohanty and J. Pradhan, A human epidermal growth factor-curcumin bandage bioconjugate loaded with mesenchymal stem cell for in vivo diabetic wound healing, Mater. Sci. Eng., C, 2020, 111, 110751 CrossRef CAS PubMed.
- V. Vivcharenko and A. Przekora, Modifications of Wound Dressings with Bioactive Agents to Achieve Improved Pro-Healing Properties, Appl. Sci., 2021, 11, 4114 CrossRef CAS.
- R. Zinder, R. Cooley, L. G. Vlad and J. A. Molnar, Vitamin A and Wound Healing, Nutr. Clin. Pract., 2019, 34, 839–849 CrossRef PubMed.
- A. Ehterami,
et al., Chitosan/alginate hydrogels containing Alpha-tocopherol for wound healing in rat model, J. Drug Delivery Sci. Technol., 2019, 51, 204–213 CrossRef CAS.
- S. Farzanfar, G. S. kouzekonan, R. Mirjani and B. Shekarchi, Vitamin B12-loaded polycaprolacton/gelatin nanofibrous scaffold as potential wound care material, Biomed. Eng. Lett., 2020, 10, 547–554 CrossRef PubMed.
- G. T. Voss,
et al., Polysaccharide-based film loaded with vitamin C and propolis: A promising device to accelerate diabetic wound healing, Int. J. Pharm., 2018, 552, 340–351 CrossRef CAS PubMed.
- A. Ehterami,
et al., A promising wound dressing based on alginate hydrogels containing vitamin D3 cross-linked by calcium carbonate/d-glucono-δ-lactone, Biomed. Eng. Lett., 2020, 10, 309–319 CrossRef PubMed.
- A. Ehterami,
et al., Chitosan/alginate hydrogels containing Alpha-tocopherol for wound healing in rat model, J. Drug Delivery Sci. Technol., 2019, 51, 204–213 CrossRef CAS.
- M. Mehrali,
et al., Blending Electronics with the Human Body: A Pathway toward a Cybernetic Future, Adv. Sci., 2018, 5, 1700931 CrossRef PubMed.
- D. A. Jankowska,
et al., Simultaneous detection of pH value and glucose concentrations for wound monitoring applications, Biosens. Bioelectron., 2017, 87, 312–319 CrossRef CAS PubMed.
- Y. Gao,
et al., A flexible multiplexed immunosensor for point-of-care in situ wound monitoring, Sci. Adv., 2021, 7, eabg9614 CrossRef CAS PubMed.
- R. Li,
et al., A flexible and physically transient electrochemical sensor for real-time wireless nitric oxide monitoring, Nat. Commun., 2020, 11, 1–11 CrossRef PubMed.
- J. Zhu,
et al., Smart bioadhesives for wound healing and closure, Bioact. Mater., 2023, 19, 360–375 Search PubMed.
- S. Saberianpour,
et al., Development of theranostic wound dressings: harnessing the knowledge of biospecific interactions at the biomaterial interface to promote healing and identify biomarkers, Expert Rev. Med. Devices, 2023, 20, 163–165 CrossRef CAS PubMed.
- N. Tang,
et al., Wearable Sensors and Systems for Wound Healing-Related pH and Temperature Detection, Micromachines, 2021, 12, 430 CrossRef PubMed.
- A. M. Wijlens, S. Holloway, S. A. Bus and J. J. van Netten, An explorative study on the validity of various definitions of a 22 °C temperature threshold as warning signal for impending diabetic foot ulceration, Int. Wound J., 2017, 14, 1346–1351 CrossRef PubMed.
- X. Deng, M. Gould and M. A. Ali, A review of current advancements for wound healing: Biomaterial applications and medical devices, J. Biomed. Mater. Res., Part B, 2022, 110, 2542–2573 CrossRef CAS PubMed.
- M. Qin, H. Guo, Z. Dai, X. Yan and X. Ning, Advances in flexible and wearable pH sensors for wound healing monitoring, J. Semicond., 2019, 40, 111607 CrossRef CAS.
- N. Pan, J. Qin, P. Feng, Z. Li and B. Song, Color-changing smart fibrous materials for naked eye real-time monitoring of wound pH, J. Mater. Chem. B, 2019, 7, 2626–2633 RSC.
- S. Wang,
et al., Responsive hydrogel dressings for intelligent wound management, BMEMat, 2023, 1, e12021 CrossRef.
- H. Derakhshandeh, S. S. Kashaf, F. Aghabaglou, I. O. Ghanavati and A. Tamayol, Smart Bandages: The Future of Wound Care, Trends Biotechnol., 2018, 36, 1259–1274 CrossRef CAS PubMed.
- A. R. Sadeghi-Avalshahr, A. M. Molavi, S. Nokhasteh and Z. Harati, Recent advances in fabrication of smart dressings for real-time monitoring of pH in chronic wounds—a review, Polym. Bull., 2023, 80, 5831–5859 CrossRef CAS.
- F. Mariani,
et al., Advanced Wound Dressing for Real-Time pH Monitoring, ACS Sens., 2021, 6, 2366–2377 CrossRef CAS PubMed.
- H. Lambers, S. Piessens, A. Bloem, H. Pronk and P. Finkel, Natural skin surface pH is on average below 5, which is beneficial for its resident flora, Int. J. Cosmet. Sci., 2006, 28, 359–370 CrossRef CAS PubMed.
- T. R. Dargaville,
et al., Sensors and imaging for wound healing: A review, Biosens. Bioelectron., 2013, 41, 30–42 CrossRef CAS PubMed.
- A. A. Arafa, A. A. Nada, A. Y. Ibrahim, M. K. Zahran and O. A. Hakeim, Greener therapeutic pH-sensing wound dressing based on Curcuma Longa and cellulose hydrogel, Eur. Polym. J., 2021, 159, 110744 CrossRef CAS.
- Q. Pang,
et al., Smart Flexible Electronics-Integrated Wound Dressing for Real-Time Monitoring and On-Demand Treatment of Infected Wounds, Adv. Sci., 2020, 7, 1902673 CrossRef CAS PubMed.
- M. T. S. Chani, K. S. Karimov, S. B. Khan, N. Fatima and A. M. Asiri, Impedimetric humidity and temperature sensing properties of chitosan-CuMn2O4 spinel nanocomposite, Ceram. Int., 2019, 45, 10565–10571 CrossRef CAS.
- P. Schilrreff and U. Alexiev, Chronic Inflammation in Non-Healing Skin Wounds and Promising Natural Bioactive Compounds Treatment, Int. J. Mol. Sci., 2022, 23, 4928 CrossRef CAS PubMed.
- S. O’Callaghan, P. Galvin, C. O’Mahony, Z. Moore and R. Derwin, ‘Smart’ wound dressings for advanced wound care: a review, J. Wound Care, 2020, 29, 394–406 CrossRef PubMed.
- M. Ochoa,
et al., Integrated sensing and delivery of oxygen for next-generation smart wound dressings, Microsyst. Nanoeng., 2020, 6, 1–16 CrossRef PubMed.
- C. K. Sen, Wound healing essentials: Let there be oxygen, Wound Repair Regen., 2009, 17, 1–18 CrossRef PubMed.
- S. Schreml,
et al., Oxygen in acute and chronic wound healing, Br. J. Dermatol., 2010, 163, 257–268 CrossRef CAS PubMed.
- P. Mostafalu,
et al., Smart Bandage for Monitoring and Treatment of Chronic Wounds, Small, 2018, 14, 1703509 CrossRef PubMed.
- S. D. Milne,
et al., A wearable wound moisture sensor as an indicator for wound dressing change: an observational study of wound moisture and status, Int. Wound J., 2016, 13, 1309–1314 CrossRef PubMed.
- E. Crowe, C. Scott, S. Cameron, J. H. Cundell and J. Davis, Developing Wound Moisture Sensors: Opportunities and Challenges for Laser-Induced Graphene-Based Materials, J. Compos. Sci., 2022, 6, 176 CrossRef CAS.
- R. Abdul Rani,
et al., High Surface Area to Volume Ratio 3D Nanoporous Nb2O5 for Enhanced Humidity Sensing, J. Electron. Mater., 2019, 48, 3805–3815 CrossRef CAS.
- N. Rani Raju,
et al., Multifunctional and Smart Wound Dressings—A Review on Recent Research Advancements in Skin Regenerative Medicine, Pharmaceutics, 2022, 14, 1574 CrossRef CAS PubMed.
- A. Pal,
et al., Early detection and monitoring of chronic wounds using low-cost, omniphobic paper-based smart bandages, Biosens. Bioelectron., 2018, 117, 696–705 CrossRef CAS PubMed.
- R. Tiwari and K. Pathak, Local Drug Delivery Strategies towards Wound Healing, Pharmaceutics, 2023, 15, 634 CrossRef CAS PubMed.
- Ö. Tezgel,
et al., Collagen scaffold-mediated delivery of NLC/siRNA as wound healing materials, J. Drug Delivery Sci. Technol., 2020, 55, 101421 CrossRef.
- X. Lin,
et al., An alginate/poly(N-isopropylacrylamide)-based composite hydrogel dressing with stepwise delivery of drug and growth factor for wound repair, Mater. Sci. Eng., C, 2020, 115, 111123 CrossRef CAS PubMed.
- C. J. Ma,
et al., Light-regulated nitric oxide release from hydrogel-forming microneedles integrated with graphene oxide for biofilm-infected-wound healing, Biomater. Adv., 2022, 134, 112555 CrossRef CAS PubMed.
- H. Derakhshandeh,
et al., A Wirelessly Controlled Smart Bandage with 3D-Printed Miniaturized Needle Arrays, Adv. Funct. Mater., 2020, 30, 1905544 CrossRef CAS PubMed.
- H. Chen,
et al., pH-Switchable Antimicrobial Supramolecular Hydrogels for Synergistically Eliminating Biofilm and Promoting Wound Healing, ACS Appl. Mater. Interfaces, 2022, 14, 18120–18132 CrossRef CAS PubMed.
- P. Sharma,
et al., Nucleic acid-based therapeutics for dermal wound healing, Int. J. Biol. Macromol., 2022, 220, 920–933 CrossRef CAS PubMed.
- D. Chouhan, N. Dey, N. Bhardwaj and B. B. Mandal, Emerging and innovative approaches for wound healing and skin regeneration: Current status and advances, Biomaterials, 2019, 216, 119267 CrossRef CAS PubMed.
- J. A. Kulkarni,
et al., The current landscape of nucleic acid therapeutics, Nat. Nanotechnol., 2021, 16, 630–643 CrossRef CAS PubMed.
- M. Hueso,
et al., Ncrnas in therapeutics: Challenges and limitations in nucleic acid-based drug delivery, Int. J. Mol. Sci., 2021, 22, 11596 CrossRef CAS PubMed.
- O. Catanzano, F. Quaglia and J. S. Boateng, Wound dressings as growth factor delivery platforms
for chronic wound healing, Expert Opin. Drug Delivery, 2021, 18, 737–759 CrossRef CAS PubMed.
- M. Nardini,
et al., Growth Factors Delivery System for Skin Regeneration: An Advanced Wound Dressing, Pharmaceutics, 2020, 12, 120 CrossRef CAS PubMed.
- D. Bian, Y. Wu, G. Song, R. Azizi and A. Zamani, The application of mesenchymal stromal cells (MSCs) and their derivative exosome in skin wound healing: a comprehensive review, Stem Cell Res. Ther., 2022, 13, 1–17 CrossRef PubMed.
- D. K. Jeppesen,
et al., Reassessment of Exosome Composition, Cell, 2019, 177, 428–445 CrossRef CAS PubMed.
- L. Ferroni,
et al., Exosomes of mesenchymal stem cells delivered from methacrylated hyaluronic acid patch improve the regenerative properties of endothelial and dermal cells, Biomater. Adv., 2022, 139, 213000 CrossRef CAS PubMed.
- P. A. Shiekh, A. Singh and A. Kumar, Exosome laden oxygen releasing antioxidant and antibacterial cryogel wound dressing OxOBand alleviate diabetic and infectious wound healing, Biomaterials, 2020, 249, 120020 CrossRef CAS PubMed.
- K. Dixit, H. Bora, J. Lakshmi Parimi, G. Mukherjee and S. Dhara, Biomaterial mediated immunomodulation: An interplay of material environment interaction for ameliorating wound regeneration, J. Biomater. Appl., 2023, 37, 1509–1528 CrossRef CAS PubMed.
- S. Butenko,
et al., Engineering Immunomodulatory Biomaterials to Drive Skin Wounds toward Regenerative Healing, Cold Spring Harbor Perspect. Biol., 2022, 15, a041242 CrossRef PubMed.
- E. Apeku, M. M. Tantuoyir, R. Zheng and N. Tanye, Exploring the polarization of M1 and M2 macrophages in the context of skin diseases, Mol. Biol. Rep., 2024, 51, 1–9 CrossRef PubMed.
- A. Apte, K. W. Liechty and C. Zgheib, Immunomodulatory biomaterials on chemokine signaling in wound healing, Front. Pharmacol., 2023, 14, 1084948 CrossRef CAS PubMed.
- J. Zhou,
et al., Spatiotemporal On–Off Immunomodulatory Hydrogel Targeting NLRP3 Inflammasome for the Treatment of Biofilm-Infected Diabetic Wounds, Adv. Funct. Mater., 2023, 33, 2211811 CrossRef CAS.
- A. Al-Attar, S. Mess, J. M. Thomassen, C. L. Kauffman and S. P. Davison, Keloid pathogenesis and treatment, Plast. Reconstr. Surg., 2006, 117, 286–300 CrossRef CAS PubMed.
- B. Nikahval, A. Oryan, P. Memarian and A. Kamali, Use of ovine acellular peritoneal matrix combined with honey and ovine fetal skin extract in the healing of full-thickness infected burn wounds in a rat model, Vet. Res. Forum, 2020, 11, 355–363 Search PubMed.
- A. Shaikh-Kader, N. N. Houreld, N. K. Rajendran and H. Abrahamse, Levels of Cyclooxygenase 2, Interleukin-6, and Tumour Necrosis Factor-α in Fibroblast Cell Culture Models after Photobiomodulation at 660
nm, Oxid. Med. Cell. Longevity, 2021, 2021, 6667812 Search PubMed.
- Y. Nazir,
et al., High Efficiency In Vitro Wound Healing of Dictyophora indusiata Extracts via Anti-Inflammatory and Collagen Stimulating (MMP-2 Inhibition) Mechanisms, J. Fungi, 2021, 7, 1100 CrossRef CAS PubMed.
- A. B. Sousa, A. P. Águas, M. A. Barbosa and J. N. Barbosa, Immunomodulatory biomaterial-based wound dressings advance the healing of chronic wounds via regulating macrophage behavior, Regener. Biomater., 2022, 9, rbac065 CrossRef CAS PubMed.
- W. Dai,
et al., A novel TCR hydrogel wound dressing loaded with UC-SMC extract enhances skin regeneration and prevents wound scarring, Appl. Mater Today, 2023, 32, 101785 CrossRef.
- Home | ClinicalTrials.gov. https://clinicaltrials.gov/.
- Google Patents. https://patents.google.com/.
- The Lens – Free & Open Patent and Scholarly Search. https://www.lens.org/.
- Espacenet – patent search. https://worldwide.espacenet.com/.
- Integrated sensor enabled wound monitoring and/or therapy dressings and systems, 2018.
- Component positioning and packaging of wound dressing for implementing sensors, 2019.
- Intelligent dressing and preparation method thereof, 2019.
- Antimicrobial compositions containing a synergistic combination of activated creatinine and an imidazole antifungal agent, 2017.
- Wound dressing with exosome combined with hyaluronic acid and preparation method and application thereof, 2021.
- Moisture sensing wound dressing, 2019.
- Preparation method of porous silicon graphene quantum dot composite drug-loaded particles, wound dressing, preparation method of wound dressing and application of wound dressing, 2020.
- Negative pressure wound therapy dressing recognition, wound status detection, and therapy adjustment, 2020.
- A method of preparation of Silk Fibroins coated with Hybrid chitosan-ZnO nanoparticles for wound dressing, 2021.
- 3D printing antibacterial hydrogel wound dressing based on green in situ reduction, 2019.
- Wound dressing based on piezoelectric effect and preparation method thereof, 2021.
- Intelligent wound dressing, preparation method and application thereof, and flexible sensor, 2022.
- Antibacterial hydrogel wound dressing loaded with two-dimensional material and nanoparticles and preparation method thereof, 2021.
- Z. Hu,
et al., Biological importance of human amniotic membrane in tissue engineering and regenerative medicine, Mater. Today Bio, 2023, 22, 100790 CrossRef CAS PubMed.
- N. Fitriani, G. Wilar, A. C. Narsa, A. F. A. Mohammed and N. Wathoni, Application of Amniotic Membrane in Skin Regeneration, Pharmaceutics, 2023, 15, 748 CrossRef CAS PubMed.
- J. R. Munoz-Torres,
et al., Biological properties and surgical applications of the human amniotic membrane, Front. Bioeng. Biotechnol., 2023, 10, 1067480 CrossRef PubMed.
- Z. Jahanafrooz, B. Bakhshandeh, S. Behnam Abdollahi and E. Seyedjafari, Human amniotic membrane as a multifunctional biomaterial: recent advances and applications, J. Biomater. Appl., 2022, 37, 1341–1354 CrossRef PubMed.
- G. Kafili, E. Tamjid, H. Niknejad and A. Simchi, Development of printable nanoengineered composite hydrogels based on human amniotic membrane for wound healing application, J. Mater. Sci., 2023, 58, 12351–12372 CrossRef CAS.
- X. Wang, H. Duan, M. Li, W. Xu and L. Wei, Characterization and mechanism of action of amphibian-derived wound-healing-promoting peptides, Front. Cell Dev. Biol., 2023, 11, 1219427 CrossRef PubMed.
- H. Haidari, L. Melguizo-Rodríguez, A. J. Cowin and Z. Kopecki, Therapeutic potential of antimicrobial peptides for treatment of wound infection, Am. J. Physiol.: Cell Physiol., 2023, 324, C29–C38 CrossRef CAS PubMed.
- A. Ebrahimi, M. Reza Farahpour, S. Amjadi, M. Mohammadi and H. Hamishehkar, Nanoliposomal peptides derived from Spirulina platensis protein accelerate full-thickness wound healing, Int. J. Pharm., 2023, 630, 122457 CrossRef CAS PubMed.
- J. Zhou,
et al., An injectable, natural peptide hydrogel with potent antimicrobial activity and excellent wound healing-promoting effects, Nano Today, 2023, 49, 101801 CrossRef CAS.
Footnote |
† Both authors contributed equally. |
|
This journal is © The Royal Society of Chemistry 2024 |