DOI:
10.1039/D0QI00746C
(Research Article)
Inorg. Chem. Front., 2020,
7, 4445-4451
2.2.2-Cryptand as a bidentate ligand in rare-earth metal chemistry†‡
Received
24th June 2020
, Accepted 6th September 2020
First published on 13th October 2020
Abstract
The 2.2.2-cryptand ligand (crypt) that is heavily used in reductions of rare-earth metal complexes to encapsulate alkali metals has been found to function as a bidentate ligand to rare-earth metal ions in some cases. The X-ray crystal structures of the reduced dinitrogen metal complex, [{(R2N)2Ce(crypt-κ2-O,O′)}2(μ–η2:η2-N2)] (R = SiMe3), and the ytterbium metallocene, (C5Me5)2Yb(crypt-κ2-O,O′), are presented to demonstrate this binding mode. The implications of this available binding mode in rare-earth metal cryptand chemistry are discussed.
Introduction
One of the recent advances in rare-earth metal chemistry has been the discovery of crystallographically-characterizable molecular complexes of La(II), Ce(II), Pr(II), Gd(II), Tb(II), Ho(II), Er(II), Lu(II), and Y(II) according to syntheses such as those shown in eqn (1).1–9 | 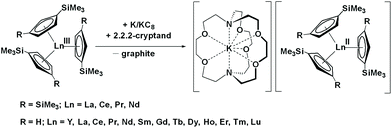 | (1) |
Prior to those discoveries, molecular complexes of +2 lanthanide ions were known only for Eu(II), Yb(II), Sm(II), Tm(II), Dy(II), and Nd(II).6,7,10–13 The reduction reactions that generated the new Ln(II) ions utilized the 2.2.2-cryptand ligand (crypt) extensively to encapsulate the alkali metal ion formed in the reaction. The crypt-chelated alkali metal units constituted good counter-cations for the (Cp′3LnII)1− and (Cp′′3LnII)1− anions (Cp′ = C5H4SiMe3; Cp′′ = C5H3(SiMe3)2), shown in eqn (1), which facilitated crystallographic characterization of the reaction products. Ln(II) metal complexes are now known with [M(crypt)]1+ counter-cations for M = Li, Na, K, Rb, and Cs in structures such as [Li(crypt)][Cp′3Ln],14 [Na(crypt)][Cp′3Ln],15 [K(crypt)][Cp′′3Ln],1,9 [K(crypt)][Cptet3Ln] (Ln = La, Ce, Pr, Nd, Sm, Gd, Tb, Dy); (Cptet = C5Me4H),16 [K(crypt)][Cpt3Dy]2 (Cpt = C5H4CMe3),17 [K(crypt)][CpMe3Dy] (CpMe = C5H4Me),18 [K(crypt)][Sc(OAr′)3] (OAr′ = OC6H2-t-Bu2-2,6-Me-4),19 [K(crypt)][((Ad,MeArO)3mes)Ln] (Ln = Nd, Gd, Dy, Er; (Ad,MeArO)3mes = (2-Ad-4-Me-C6H2O)3(2,4,6-Me3C6H2); Ad = 1-adamantyl),8 [K(crypt)][Y(OArAd,Ad,t-Bu)3] (OArAd,Ad,t-Bu = 2,6-Ad2-4-t-Bu-C6H2O),20 [Rb(crypt)][Ln(NR2)3] (Ln = Nd, Ho, Er),21 and [Cs(crypt)][Sc(NR2)3] (R = SiMe3).22 Additionally, the crypt ligand in [Li(crypt)]1+ counter-cations has also been found as crypt-κ6 in three different binding modes: N2O4,14,23 NO5,14,23–29 and O6.14
However, the crypt ligand can also encapsulate rare-earth metals ions as well as alkali metal ions. A variety of such metal complexes have been crystallographically-characterized involving both divalent and trivalent ions usually with one or two additional coordinated ligands that could be anions or neutral species. Examples include [LnII(crypt)(THF)x][Cp′3Ln] (x = 1, Ln = Sm, Eu; x = 0, Ln = Yb),30 [SmII(crypt)(DippForm)][DippForm] (DippForm = N,N′-bis(2,6-diisopropylphenyl)-formamidinate),31 [SmII(crypt)(PCO)2],31 [EuII(crypt)Cl][Cl],32 [YbII(crypt)I][I],33 [LnIII(crypt)(THF)][BPh4]2 (Ln = Sm, Eu),34 [LaIII(crypt)Cl2][Cl],35 [LnII(crypt)(DMF)x][X]2 (x = 2, Ln = Sm, Eu; x = 1, Ln = Yb; X = I, BPh4),36 [LnIII(crypt)(OTf)2][OTf] and [LnII(crypt)(OTf)2] (Ln = Nd, Sm)37 among others.38–42
We report here a new coordination mode for crypt with rare-earth metal ions that is intermediate between the two modes described above, i.e. it is in between the 8-coordinate mode with the rare-earth metal ion inside crypt and the situation in which crypt is not coordinating the rare-earth metal ion at all. We describe here two crystal structures that show that crypt can function as a bidentate ligand to rare-earth ions. This has interesting implications in the reaction chemistry of rare-earth metal complexes involving crypt.
Results and discussion
The first example of a bidentate crypt metal complex of a rare-earth metal ion was discovered while examining the reduction of dinitrogen with Ce(NR2)3 (R = SiMe3) and potassium graphite. Numerous LnA3/M reactions (A = anion; M = alkali metal) of this type have been performed in the past and generated both (N
N)2− and (N2)3− metal complexes.43–48 For example, crystallographically-characterized examples of [(R2N)2(THF)Ln]2(μ–η2:η2-N2) have been isolated in this way from reactions in THF for the smaller rare-earths, Ln = Nd, Gd, Tb, Dy, Ho, Y, Er, Tm, and Lu, eqn (2).44 | 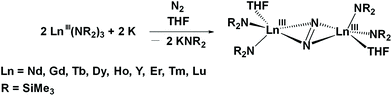 | (2) |
Analogous reactions with the larger lanthanides, La, Ce, and Pr, appeared to proceed similarly, but crystallographic confirmation of the products was not obtained. 1H and 15N NMR data on the La product were similar to those on the Y and Lu products.44,46 Hence, it was likely that (N
N)2− products of La, Ce, and Pr formed, but the metal complexes were too sterically unsaturated with these larger metals to crystallize well. Further evidence of reaction in eqn (2) with the large lanthanides was obtained by isolation and crystallographic characterization of the tetrakis(amide) products, [K(THF)6][Ln(NR2)4] for La and Pr and [Na(THF4)(Et2O)][Ce(NR2)4] for Ce. These tetrakis(amides) can form by complexation to the Ln(NR2)3 starting material of the (NR2)1− ligands lost by each metal in a successful dinitrogen reduction.44
When the LnA3/M reaction was conducted in Et2O using Ce(NR2)3 and KC8 in the presence of crypt, the (N
N)2− metal complex, [{(R2N)2Ce(crypt-κ2-O,O′)}2(μ–η2:η2-N2)], 1, was isolated and identified by X-ray diffraction, eqn (3), Fig. 1. This was the first cerium amide (N
N)2− metal complex that could be crystallized.
| 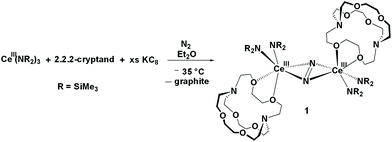 | (3) |
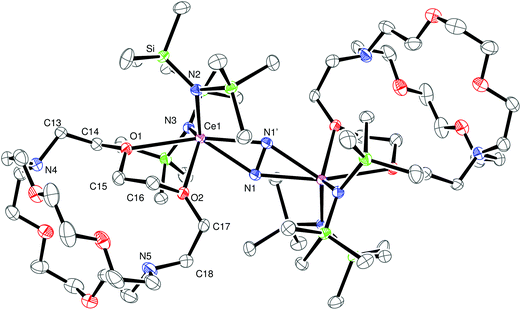 |
| Fig. 1 ORTEP representation of [{(R2N)2Ce(crypt-κ2-O,O′)}2(μ–η2:η2-N2)], 1, with thermal ellipsoids drawn at the 50% probability level. Hydrogen atoms and an Et2O in the lattice are excluded for clarity. | |
The crystal structure of 1, shows that it is a neutral metal complex with a side-on bound (N
N)2− and two amide ligands bound to each cerium. This is similar to the known series of THF adducts in eqn (2), [{(THF)(R2N)2Ln}2(μ–η2:η2-N2)],44 except instead of one THF molecule per metal, a crypt ligand coordinates to each metal through two oxygen atoms. In this case, crypt is acting as a κ2-O,O′ “exo-crypt” with binding similar to that of a dimethoxyethane (DME) ligand. Presumably, with the extra steric bulk of crypt versus THF, the Ce (N
N)2− product is sterically saturated enough to allow isolation and crystallization.
Compound 1 is sparingly soluble in both toluene and benzene. A 1H NMR spectrum was taken in both toluene-d8 and C6D6 (see ESI‡). The crypt resonances align with free cryptand in solution and only one resonance is seen for the methyl group of the silyl amides, which is shifted upfield.
The 1.233(4) Å N–N distance in 1 is similar to those in the metal complexes in eqn (2), 1.258(3)–1.305(6) Å, and is consistent with a double bond, i.e. (N
N)2−.49,50 The exo-crypt in 1 does not bind symmetrically and has Ce–O distances in 1 of 2.545(2) Å for Ce–O1 and 2.796(2) Å for Ce–O2. In comparison, the Ce–O(THF) distances in the cyclopentadienyl cerium dinitrogen complexes, [(C5Me5)2(THF)Ce]2[μ–η2:η2-N2] and [(C5Me4H)2(THF)Ce]2[μ–η2:η2-N2], are 2.607(4) Å and 2.589(3) Å, respectively.46 Hence, the crypt coordination could also be described as a monodentate ligand with a long secondary interaction. The 2.545(2) Å Ce–O(crypt) distance is similar to the 2.518(1) Å Nd–O(THF) distance in [(R2N)2(THF)Nd]2(μ–η2:η2-N2) when the 0.027 Å ionic radius increase from Nd to Ce is considered.51
Within the coordinated crypt, the 1.437(3)–1.443(3) Å C–O distances involving the coordinating O1 and O2 are within the error limits of the other 1.399(4)–1.424(4) Å C–O distances, as shown in Table 1. The C–C distances and the angles involving the bound oxygen atoms are similar to those in the rest of the crypt molecule.
Table 1 Selected distances (Å) and angles (°) for 1
Ce1–N3(amide) |
2.409(2) |
Ce1–N1 |
2.451(2) |
Ce1–N2(amide) |
2.411(2) |
Ce1–N1′ |
2.468(2) |
Ce1–O1 |
2.7957(18) |
Ce1–O2 |
2.5453(18) |
N1–N1′ |
1.233(4) |
|
|
Crypt O–C distance |
O1–C14 |
1.439(3) |
O1–C15 |
1.437(3) |
O2–C16 |
1.438(3) |
O2–C17 |
1.443(3) |
O3–C20 |
1.422(4) |
O3–C21 |
1.418(4) |
O4–C22 |
1.413(4) |
O4–C23 |
1.418(4) |
O5–C26 |
1.424(4) |
O5–C27 |
1.417(4) |
O6–C28 |
1.413(4) |
O6–C29 |
1.399(4) |
Crypt C–C distance |
C13–C14 |
1.525(4) |
C15–C16 |
1.485(4) |
C17–C18 |
1.512(4) |
C19–C20 |
1.504(4) |
C21–C22 |
1.489(5) |
C23–C24 |
1.507(5) |
C25–26 |
1.510(4) |
C27–C28 |
1.495(5) |
C29–C30 |
1.525(5) |
|
|
A second example of exo-crypt coordination was found in the reaction of (C5Me5)Yb(BPh4) with crypt in toluene. This reaction was explored to determine if ligand redistribution would occur to form an Yb(II)-in-crypt product.30 However, ligand rearrangement occurred to form a bis(cyclopentadienyl) metallocene which crystallized with crypt bound as a bidentate ligand, (C5Me5)2Yb(crypt-κ2-O,O′), 2, eqn (4), Fig. 2. The compound was identified by X-ray crystallography, but the data were not of high enough quality for a detailed structural discussion. In the metal complex 2, as in 1, the exo-crypt ligand coordinates like DME. X-ray crystal structures of similar formally eight coordinate (C5Me5)2YbL2 compounds are common with L = pyridine52 and with L2 = bipyridine and substituted bipyridines.53,54
| 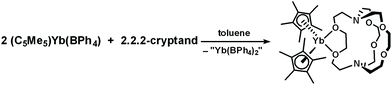 | (4) |
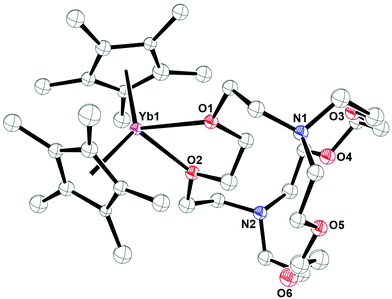 |
| Fig. 2 Structure of (C5Me5)2Yb(crypt-κ2-O,O′), 2, showing the bidentate binding of crypt. | |
Conclusion
Although 2.2.2-cryptand can stabilize alkali metal ions to generate favorable lattices for crystallization of rare-earth metal complexes and it can encapsulate rare-earth ions as an octadentate ligand, it can also function as a stabilizing bidentate κ2-chelating ligand for rare-earth metal ions as shown in [{(R2N)2Ce(crypt-κ2-O,O′)}2(μ–η2:η2-N2)], 1, and (C5Me5)2Yb(crypt-κ2-O,O′), 2. The diverse nature of these two metal complexes suggests that crypt could coordinate to rare-earth metal ions in a variety of different coordination environments.
Similar to these crypt-κ2-O,O′ binding modes, 18-crown-6 (18-c-6) rare-earth metal complexes are known in which not all O-donors are coordinated. Two scandium metal complexes containing [ScCl2(κ5-18-c-6)][X] (X = SbCl6 or FeCl4)55,56 and [Ce(κ2-18-c-6)][N(SiMe3)PhF]3 (PhF = pentafluorophenyl)57 have been reported.
To the extent that crypt binds like dimethoxyethane, it constitutes a sterically bulky variation of this commonly-used ether. The size and 3-dimensional nature of crypt means that it can substantially fill the coordination environment of a sterically unsaturated compound. In the case of the [{(R2N)2Ln}2(μ–η2:η2-N2)], which crystallizes for smaller metals, Nd–Lu, but not for Ce, it is possible that a single THF ligand was not enough to sterically saturate the coordination environment to yield single crystals for X-ray diffraction. Crypt coordination in 1 led to successful crystallization of this cerium (N
N)2− compound. Attempts to crystallize “[{(R2N)2Ce}2(μ–η2:η2-N2)]”, with DME have been unsuccessful.
The efficacy of crypt in 2 is somewhat different since the (C5Me5)2Yb unit crystallizes in the absence of any ethers and with one and two coordinating ligands.52–54,58–60 Hence, this is not the case of a sterically unsaturated unit that will not crystallize. The κ2-crypt just functions as other bidentate coordinating bases.
These results raise interesting possibilities in reaction chemistry. It is possible that crypt can coordinate to a rare-earth metal ion before alkali metal reduction occurs and before the alkali metal cation is formed and inserts into the crypt. Similarly, in the reactions that form Ln-in-crypt [Ln(crypt)]n+ cations,30,35–37 a preliminary step in the procedure could involve bidentate coordination of the crypt to the rare-earth metal ion before it inserts into the cavity. The importance of κ2-crypt coordination in any reaction coordinate will depend heavily on the specific system, the solvent, and any other ligands present in the reaction mixture. However, in non-polar solvents free of any other coordinating ligands, κ2-crypt could play an important role as a bidentate ligand that preferentially stabilizes species that are otherwise not isolable.
Experimental
All manipulations and syntheses described below were conducted with the rigorous exclusion of air and water using standard Schlenk line and glovebox techniques under an argon or dinitrogen atmosphere. Solvents were sparged with UHP argon and dried by passage through columns containing Q-5 and molecular sieves prior to use. Deuterated NMR solvents were dried over NaK alloy or Na/benzophenone, degassed by three freeze–pump–thaw cycles, and vacuum-transferred before use. 1H NMR spectra were recorded on a Bruker AVANCE 600 spectrometer (1H operating at 600 MHz) at 298 K, unless otherwise stated, and referenced internally to residual protio-solvent resonances. 2.2.2-Cryptand (Sigma-Aldrich) was placed under vacuum (10−3 Torr) before use. Ce(NR2)3
61 and (C5Me5)Yb(BPh4)62 were synthesized according to literature procedures.
[{(R2N)2Ce(crypt-κ2-O,O′)}2(μ–η2:η2-N2)], 1
A dinitrogen-saturated Et2O (3 mL) solution of Ce(NR2)3 (R = SiMe3) (60 mg, 0.1 mmol) and 2.2.2-cryptand (36 mg, 0.1 mmol), chilled to −35 °C, was added to a vial containing excess KC8 (20 mg 0.1 mmol), that had been chilled in a cold well with a liquid nitrogen bath. This generated a yellow solution. Bright yellow crystals were isolated by filtering and placing the Et2O solution in a −35 °C freezer for several days (20 mg, 24%). These were identified as 1 by X-ray diffraction.
(C5Me5)2Yb(crypt-κ2-O,O′), 2
In an argon atmosphere, the addition of a green toluene-solution of (C5Me5)Yb(BPh4) (50 mg, 0.08 mmol) to a toluene (2 mL) solution of 2.2.2-cryptand (30 mg, 0.08 mmol) generated a green mixture with white precipitate. The mixture was filtered to remove insoluble material and the green solution was layered into hexanes and placed in a −35 °C freezer. After 1 d, green X-ray quality crystals of 2 were formed.
Conflicts of interest
There are no conflicts to declare.
Acknowledgements
We thank the U. S. National Science Foundation for support of this research under CHE-1855328. We also thank Chen Sun for assistance with X-ray crystallography and David H. Woen for sample preparation.
Notes and references
- P. B. Hitchcock, M. F. Lappert, L. Maron and A. V. Protchenko, Lanthanum Does Form Stable Molecular Compounds in the +2 Oxidation State, Angew. Chem., Int. Ed., 2008,(47), 1488–1491 CrossRef CAS.
- M. R. MacDonald, J. W. Ziller and W. J. Evans, Synthesis of a Crystalline Molecular Complex of Y2+, [(18-Crown-6)K][(C5H4SiMe3)3Y], J. Am. Chem. Soc., 2011, 133(40), 15914–15917 CrossRef CAS.
- M. R. MacDonald, J. E. Bates, M. E. Fieser, J. W. Ziller, F. Furche and W. J. Evans, Expanding Rare-Earth Oxidation State Chemistry to Molecular Complexes of Holmium(II) and Erbium(II), J. Am. Chem. Soc., 2012, 134(20), 8420–8423 CrossRef CAS.
- M. R. Macdonald, E. Bates, J. W. Ziller, F. Furche and W. J. Evans, Completing the Series of +2 Ions for the Lanthanide Elements: Synthesis of Molecular Complexes of Pr+2, Gd+2, Tb+2, and Lu+2, J. Am. Chem. Soc., 2013, 135, 9857–9868 CrossRef CAS.
- M. E. Fieser, M. R. Macdonald, B. T. Krull, J. E. Bates, J. W. Ziller, F. Furche and W. J. Evans, Structural, Spectroscopic, and Theoretical Comparison of Traditional vs Recently Discovered Ln2+ Ions in the [K(2.2.2-Cryptand)][(C5H4SiMe3)3Ln] Complexes: The Variable Nature of Dy2+ and Nd2+, J. Am. Chem. Soc., 2015, 137(1), 369–382 CrossRef CAS.
- W. J. Evans, Tutorial on the Role of Cyclopentadienyl Ligands in the Discovery of Molecular Complexes of the Rare-Earth and Actinide Metals in New Oxidation States, Organometallics, 2016, 35, 3088–3100 CrossRef CAS.
-
D. H. Woen and W. J. Evans, Expanding the +2 Oxidation State of the Rare-Earth Metals, Uranium, and Thorium in Molecular Complexes, in Handbook on the Physics and Chemistry of Rare Earths, Elsevier B.V., 2016, pp. 1–57 Search PubMed.
- M. E. Fieser, C. T. Palumbo, H. S. La Pierre, D. P. Halter, V. K. Voora, J. W. Ziller, F. Furche, K. Meyer and W. J. Evans, Comparisons of Lanthanide/Actinide +2 Ions in a Tris(aryloxide)arene Coordination Environment, Chem. Sci., 2017, 8(11), 7424–7433 RSC.
- C. T. Palumbo, L. E. Darago, C. J. Windorff, J. W. Ziller and W. J. Evans, Trimethylsilyl versus Bis(trimethylsilyl) Substitution in Tris(cyclopentadienyl) Complexes of La, Ce, and Pr: Comparison of Structure, Magnetic Properties, and Reactivity, Organometallics, 2018, 37(6), 900–905 CrossRef CAS.
- L. R. Morss, Thermochemical Properties of Yttrium, Lanthanum, and the Lanthanide Elements and Ions, Chem. Rev., 1975, 76(6), 827–841 CrossRef.
- N. B. Mikheev and A. N. K. Complex, Formation of the Lanthanides and Actinides in Lower Oxidation States, Coord. Chem. Rev., 1991, 109, 1–59 CrossRef CAS.
- M. N. Bochkarev, Molecular Compounds of “New” Divalent Lanthanides, Coord. Chem. Rev., 2004, 248(9–10), 835–851 CrossRef CAS.
-
F. Nief, Molecular Chemistry of the Rare-Earth Elements in Uncommon Low-Valent States, in Handbook on the Physics and Chemistry of Rare Earths, 2010, pp. 241–300 Search PubMed.
- D. N. Huh, L. E. Darago, J. W. Ziller and W. J. Evans, Utility of Lithium in Rare-Earth Metal Reduction Reactions to Form Nontraditional Ln2+ Complexes and Unusual [Li(2.2.2-Cryptand)]1+ Cations, Inorg. Chem., 2018, 57(4), 2096–2102 CrossRef CAS.
-
M. E. Fieser, PhD Dissertation, University of California, Irvine, 2015.
- T. F. Jenkins, D. H. Woen, L. N. Mohanam, J. W. Ziller, F. Furche and W. J. Evans, Tetramethylcyclopentadienyl Ligands Allow Isolation of Ln(II) Ions across the Lanthanide Series in [K(2.2.2-Cryptand)][(C5Me4H)3Ln] Complexes, Organometallics, 2018, 37(21), 3863–3873 CrossRef CAS.
- M. A. Angadol, D. H. Woen, C. J. Windorff, J. W. Ziller and W. J. Evans,
tert-Butyl(cyclopentadienyl) Ligands Will Stabilize Nontraditional +2 Rare-Earth Metal Ions, Organometallics, 2019, 38(5), 1151–1158 CrossRef CAS.
- D. N. Huh, J. W. Ziller and W. J. Evans, Isolation of Reactive
Ln(II) Complexes with C5H4Me Ligands (CpMe) Using Inverse Sandwich Countercations: Synthesis and Structure of [(18-Crown-6)K(μ-CpMe)K(18-Crown-6)][CpMe3LnII] (Ln = Tb, Ho), Dalton Trans., 2018, 47(48), 17285–17290 RSC.
- S. A. Moehring, M. J. Beltrán-Leiva, D. Páez-Hernández, R. Arratia-Pérez, J. W. Ziller and W. J. Evans, Rare-Earth Metal(II) Aryloxides: Structure, Synthesis, and EPR Spectroscopy of [K(2.2.2-Cryptand)][Sc(OC6H2tBu2-2,6-Me-4)3], Chem. – Eur. J., 2018, 24(68), 18059–18067 CrossRef CAS.
- S. A. Moehring, M. Miehlich, C. J. Hoerger, K. Meyer, J. W. Ziller and W. J. Evans, A Room-Temperature Stable Y(II) Aryloxide: Using Steric Saturation to Kinetically Stabilize Y(II) Complexes, Inorg. Chem., 2020, 59(5), 3207–3214 CrossRef CAS.
- A. J. Ryan, L. E. Darago, G. Balasubramani, G. P. Chen, J. W. Ziller, F. Furche, J. R. Long and W. J. Evans, Synthesis, Structure, and Magnetism of Tris(amide) [Ln{N(SiMe3)2}3]1- Complexes of the Non-traditional +2 Lanthanide Ions, Chem. – Eur. J., 2018, 2, 7702–7709 CrossRef.
- D. H. Woen, G. P. Chen, J. W. Ziller, T. J. Boyle, F. Furche and W. J. Evans, Solution Synthesis, Structure, and CO2 Reduction Reactivity of a Scandium(II) Complex, [Sc(N(SiMe3)2)3]-, Angew. Chem., 2017, 129, 2082–2085 CrossRef.
- S. D. Han, J. L. Allen, E. Jónsson, P. Johansson, D. W. McOwen, P. D. Boyle and W. A. Henderson, Solvate Structures and Computational/Spectroscopic Characterization of Lithium Difluoro(oxalato)borate (LiDFOB) Electrolytes, J. Phys. Chem. C, 2013, 117(11), 5521–5531 CrossRef CAS.
- C. Tirla, N. Mézailles, L. Ricard, F. Mathey and P. Le Floch, Dianionic Platinadiphospholene Complexes, Inorg. Chem., 2002, 41(23), 6032–6037 CrossRef CAS.
- A. Moores, L. Ricard, P. Le Floch and N. Mézailles, First X-Ray Crystal Study and DFT Calculations of Anionic λ4-Phosphinines, Organometallics, 2003, 22(9), 1960–1966 CrossRef CAS.
- I. A. Guzei, L. C. Spencer, J. W. Su and R. R. Burnette, Low-Temperature Enantiotropic k2 Phase Transition in the Ionic 222-Cryptand Complex with LiClO4, Acta Crystallogr., Sect. B: Struct. Sci., 2007, 63(1), 93–100 CrossRef CAS.
- P. Alex Rudd, N. Planas, E. Bill, L. Gagliardi and C. C. Lu, Dinitrogen Activation at Iron and Cobalt Metallalumatranes, Eur. J. Inorg. Chem., 2013, 2(22–23), 3898–3906 CrossRef.
- M. K. Assefa, G. Wu and T. W. Hayton, Synthesis of a Terminal Ce(IV) Oxo Complex by Photolysis of a Ce(III) Nitrate Complex, Chem. Sci., 2017, 8(11), 7873–7878 RSC.
- E. Lu, J. T. Boronski, M. Gregson, A. J. Wooles and S. T. Liddle, Silyl-Phosphino-Carbene Complexes of Uranium(IV), Angew. Chem., Int. Ed., 2018, 57(19), 5506–5511 CrossRef CAS.
- D. N. Huh, C. M. Kotyk, M. Gembicky, A. L. Rheingold, J. W. Ziller and W. J. Evans, Synthesis of Rare-Earth-Metal-in-Cryptand Dications, [Ln(2.2.2-Cryptand)]2+, from Sm2+, Eu2+, and Yb2+ Silyl Metallocenes (C5H4SiMe3)2Ln(THF)2, Chem. Commun., 2017, 53(62), 8664–8666 RSC.
- S. Bestgen, Q. Chen, N. H. Rees and J. M. Goicoechea, Synthesis and Reactivity of Rare-Earth Metal Phosphaethynolates, Dalton Trans., 2018, 47(37), 13016–13024 RSC.
- C. U. Lenora, F. Carniato, Y. Shen, Z. Latif, E. M. Haacke, P. D. Martin, M. Botta and M. J. Allen, Structural Features of Europium(II)-Containing Cryptates That Influence Relaxivity, Chem. – Eur. J., 2017, 23(61), 15404–15414 CrossRef CAS.
- T. C. Jenks, A. N. W. Kuda-Wedagedara, M. D. Bailey, C. L. Ward and M. J. Allen, Spectroscopic and Electrochemical Trends in Divalent Lanthanides through Modulation of Coordination Environment, Inorg. Chem., 2020, 59(4), 2613–2620 CrossRef CAS.
- M. L. Marsh, F. D. White, D. S. Meeker, C. D. McKinley, D. Dan, C. Van Alstine, T. N. Poe, D. L. Gray, D. E. Hobart and T. E. Albrecht-Schmitt, Electrochemical Studies of Selected Lanthanide and Californium Cryptates, Inorg. Chem., 2019, 58(15), 9602–9612 CrossRef CAS.
- D. N. Huh, C. J. Windorff, J. W. Ziller and W. J. Evans, Synthesis of Uranium-in-Cryptand Complexes, Chem. Commun., 2018, 54(73), 10272–10275 RSC.
- D. N. Huh, J. W. Ziller and W. J. Evans, Facile Encapsulation of Ln(II) Ions into Cryptate Complexes from LnI2(THF)2 Precursors (Ln = Sm, Eu, Yb), Inorg. Chem., 2019, 58(15), 9613–9617 CrossRef CAS.
- D. N. Huh, S. R. Ciccone, S. Roy, J. W. Ziller, F. Furche and W. J. Evans, Synthesis of Ln(II)-in Cryptand Complexes by Chemical Reduction of Ln(III)-in-Cryptand Precursors, Angew. Chem., Int. Ed., 2020, 59, 16141–16146 CAS.
- J. H. Burns, Crystal and Molecular Structure of a Cryptate Complex of Samarium: C18H36O6N2Sm2(NO3)6 · H2O, Inorg. Chem., 1979, 18(11), 3044–3047 CrossRef CAS.
- B. M. Ciampolini, P. Dapporto and N. Nardi, Structure and Properties of Some Lanthanoid(III) Perchlorates with the Cryptand 4,7,13,16,21,24-Hexaoxa-1,10-diazabicyclo[8.8.8]hexacosane, J. Chem. Soc., Dalton Trans., 1979, 974–977 RSC.
- F. Benetollo, G. Bombieri, A. Cassol, G. De Paoli and J. Legendziewicz, Coordination Chemistry of Lanthanides with Cryptands. An X-Ray and Spectroscopic Study of the Complex Nd2(NO3)6 [C18H36O6N2] · H2O, Inorg. Chim. Acta, 1985, 110(1), 7–13 CrossRef CAS.
- G. Yang, S. Liu and Z. Jin, Coordination Chemistry and Structure Characterization of C18H36O6N2Eu2(NO3)6 · H2O, Inorg. Chim. Acta, 1987, 131(1), 125–128 CrossRef CAS.
- U. Heinze, T. Heinze and D. Klemm, Synthesis and Structure Characterization of Lanthanum [2,2,2]Cryptates, [LaCl[2,2,2](H2O)]Cl · H2O and [La(CF3SO3)[2,2,2](DMF)](CF3SO3)2, Macromol. Chem. Phys., 1999, 200(2), 896–902 CrossRef CAS.
- W. J. Evans, D. S. Lee, C. Lie and J. W. Ziller, Expanding the LnZ3/ Alkali-Metal Reduction System to Organometallic and Heteroleptic Precursors: Formation of Dinitrogen Derivatives of Lanthanum, Angew. Chem., Int. Ed., 2004, 43(41), 5517–5519 CrossRef CAS.
- W. J. Evans, D. S. Lee, D. B. Rego, J. M. Perotti, S. A. Kozimor, E. K. Moore and J. W. Ziller, Expanding Dinitrogen Reduction Chemistry to Trivalent Lanthanides via the LnZ3/Alkali Metal Reduction System: Evaluation of the Generality of Forming Ln2(μ-η2:η2-N2) Complexes via LnZ3/K, J. Am. Chem. Soc., 2004, 126(44), 14574–14582 CrossRef CAS.
- W. J. Evans, D. S. Lee and J. W. Ziller, Reduction of Dinitrogen to Planar Bimetallic M2(μ-η2:η2-N2) Complexes of Y, Ho, Tm, and Lu Using the K/Ln[N(SiMe3)2]3 Reduction System, J. Am. Chem. Soc., 2004, 126, 454–455 CrossRef CAS.
- W. J. Evans, D. B. Rego and J. W. Ziller, Synthesis, Structure, and 15N NMR Studies of Paramagnetic Lanthanide Complexes Obtained by Reduction of Dinitrogen, Inorg. Chem., 2006, 45, 10790–10798 CrossRef CAS.
- D. Rinehart, M. Fang, W. J. Evans and R. Long, A N23- Radical-Bridged Terbium Complex Exhibiting Magnetic Hysteresis at 14 K, J. Am. Chem. Soc., 2011, 133, 14236–14239 CrossRef.
- M. Fang, D. S. Lee, J. W. Ziller, R. J. Doedens, J. E. Bates, F. Furche and W. J. Evans, Synthesis of the (N2)3- Radical from Y2+ and Its Protonolysis Reactivity to Form (N2H2)2- via the Y[N(SiMe3)2]3/KC8 Reduction System, J. Am. Chem. Soc., 2011, 133(11), 3784–3787 CrossRef CAS.
- F. H. Allen, O. Kennard, D. G. Watson, L. Brammer, A. G. Orpen and R. Taylor, Tables of Bond Lengths Determined by X-Ray and Neutron Diffraction. Part 1. Bond Lengths in Organic Compounds, J. Chem. Soc., Perkin Trans. 2, 1987,(12), 1–19 RSC.
- M. E. Fieser, D. H. Woen, J. F. Corbey, T. J. Mueller, J. W. Ziller and W. J. Evans, Raman Spectroscopy of the N-N Bond in Rare Earth Dinitrogen Complexes, Dalton Trans., 2016, 45(37), 14634–14644 RSC.
- R. D. Shannon, Revised Effective Ionic Radii and Systematic Studies of Interatomie Distances in Halides and Chaleogenides, Acta Crystallogr., Sect. A: Cryst. Phys., Diffr., Theor. Gen. Crystallogr., 1976, 32, 751–767 CrossRef.
- T. D. Tilley, R. A. Andersen, B. Spencer and A. Zalkin, Bis(pentamethylcyclopentadienyl)bis(pyridine)ytterbium(II), Inorg. Chem., 1982, 21(7), 2647–2649 CrossRef CAS.
- M. Schultz, J. M. Boncella, D. J. Berg, T. D. Tilley and R. A. Andersen, Coordination of 2,2′-Bipyridyl and 1,10-Phenanthroline to Substituted Ytterbocenes: An Experimental Investigation of Spin Coupling in Lanthanide Complexes, Organometallics, 2002, 21(5), 460–472 CrossRef CAS.
- G. Nocton, C. H. Booth, L. Maron and R. A. Anderson, Influence of the Torsion Angle in 3,3′-Dimethyl-2,2′-bipyridine on the Intermediate Valence of Yb in (C5Me5)2Yb(3,3’-Me2-bipy), Organometallics, 2013, 32(19), 5305–5312 CrossRef CAS.
- G. R. Willey, M. T. Lakin and N. W. Alcock, Unusual Crown Ether Encapsulation of a Chloroscandium(III) Cation. Structure of Threaded [ScCl2(18-crown-6)][SbCl6], J. Chem. Soc., Chem. Commun., 1992, 21, 1619–1620 RSC.
- M. D. Brown, W. Levason, D. C. Murray, M. C. Popham, G. Reid and M. Webster, Primary and Secondary Coordination of Crown Ethers to Scandium(III). Synthesis, Properties and Structures of the Reaction Products of ScCl3(THF)3, ScCl3·6H2O and Sc(NO3)3·5H2O with Crown Ethers, Dalton Trans., 2003, 3(5), 857–865 RSC.
- H. Yin, J. R. Robinson, P. J. Carroll, P. J. Walsh and E. J. Schelter, κ2-Coordination of 18-crown-6 to Ce(III) Cations: Solution Dynamics and Reactivity, Chem. Commun., 2014, 50(26), 3470–3472 RSC.
- D. T. Tilley, R. A. Andersen, B. Spencer, H. Ruben and D. H. Templeton, Divalent Lanthanide Chemistry. Bis(pentamethylcyclopentadienyl)europium(II) and ytterbium(II) Derivatives: Crystal Structure of Bis(pentamethyIcyclopentadienyl)(tetrahydrofuran)ytterbium(II)-Hemitoluene at 176 K, Inorg. Chem., 1980, 19, 2999–3003 CrossRef.
- T. D. Tilley, J. M. Boncella, D. J. Berg, C. J. Burns, R. A. Andersen, G. A. Lawless, M. A. Edelman and M. F. Lappert, Bis[Bis(Trimethylsilyl)Amido]Bis(Diethyl Ether)Ytterbium and (Diethyl Ether)Bis(η5−Pentamethylcyclopentadienyl)Ytterbium, Inorg. Synth., 1990, 27, 146 CAS.
- M. Schultz, C. J. Burns, D. J. Schwartz and R. A. Andersen, Solid-State Structures of Base-Free Ytterbocenes and Inclusion Compounds of Bis(pentamethylcyclopentadienyl)ytterbium with Neutral Carboranes and Toluene: The Role of Intermolecular Contacts, Organometallics, 2000, 19(9), 781–789 CrossRef CAS.
- D. C. Bradley, J. S. Ghotra and F. A. Hart, Three-co-ordination in Lanthanide Chemistry: Tris[bis(trimethylsilyl)amido]lanthanide(III) Compounds, J. Chem. Soc., Chem. Commun., 1972, 349–350 RSC.
- W. J. Evans, T. M. Champagne and J. W. Ziller, Synthesis and Reactivity of Mono (pentamethylcyclopentadienyl) Tetraphenylborate Lanthanide Complexes of Ytterbium and Samarium: Tris(ring) Precursors to (C5Me5)Ln Moieties, Organometallics, 2007, 26(24), 1204–1211 CrossRef CAS.
Footnotes |
† Dedicated to Professor Xu Guangxian for his extensive efforts in research as “the father of Chinese rare-earth chemistry” and for his excellent teaching which has generated an ongoing legacy of outstanding students. |
‡ Electronic supplementary information (ESI) available. CCDC 2011829. For ESI and crystallographic data in CIF or other electronic format see DOI: 10.1039/d0qi00746c |
|
This journal is © the Partner Organisations 2020 |
Click here to see how this site uses Cookies. View our privacy policy here.