DOI:
10.1039/C8QM00424B
(Research Article)
Mater. Chem. Front., 2019,
3, 151-160
Rational design of aggregation-induced emission sensor based on Rhodamine B for turn-on sensing of trivalent metal cations, reversible data protection, and bioimaging†
Received
24th August 2018
, Accepted 16th November 2018
First published on 19th November 2018
Abstract
A novel AIE-active molecule (TPEThRB) composed of a tetraphenylethene unit, Rhodamine spirolactam, as a receptor, and thiophene ring as a conjugated electron-rich linker was obtained. It can be utilized to effectively detect trivalent metal cations and H+ ions, accompanied with visible color change and turn-on fluorescence enhancement. The four species can be further recognized individually through UV-Vis analysis. Moreover, compared to the conventional manner of metal ion chelation-induced ring-opening form of Rhodamine B derivative, the interaction of TPEThRB with trivalent cations is ascribed to the synergy of proton-induced open-cycle and the subsequent coordination interaction due to the steric effect and acidic hydrolysis of trivalent metal cations. In addition, in view of its acidochromism property with reversible color and fluorescence changes, AIE-active TPEThRB may be a prominent smart material for data protection by employing trifluoroacetic acid vapor as the chromogenic reagent and diethylamine vapor as the eraser. Owing to the AIE characteristics and the remarkable photophysical response characteristics towards trivalent metal ions in vitro, TPEThRB is suitable for fluorescence ratio imaging of trivalent metal cations in living cells.
Introduction
Due to the involvement of organic fluorescent materials in widespread applications, such as organic light-emitting diodes (OLEDs), fluorescent sensors, mechano-sensors and bioimaging, they have received significant attention.1 Considering the inherent sensing merits of visualization, sensitivity and selectivity, fluorescent molecules have played a great role in detecting various analytes in living organisms.2 Trivalent metal cations, M3+ = Al3+, Cr3+, Fe3+, have important influence on multiple physiological and pathological processes. For example, high levels of Al3+ ions can give rise to degeneration of the cranial nerves and biological function damage, including cognitive deterioration, mental disorder and metabolic disturbance of calcium and phosphorus.3 Abnormal content of Cr3+ ions can trigger diabetes and cardiovascular diseases.4 The deficiency of Fe3+ ion, an essential trace element, can cause many physiological abnormalities such as hypoimmunity, decreased resistance to infection and anemia.5 Therefore, research on fluorescent materials for effectively detecting trivalent metal cations is of great significance.
To date, there are many reports with respect to fluorescent materials used for detecting metal ions;6 however, many of them are turn-off type sensors, resulting in output information inevitably suffering from background fluorescence interference and disadvantages in biosensing and bioimaging fields.7 Compared to turn-off sensors, turn-on sensors with excellent performance are more valuable, in particular for biological applications.8 Here, spirocyclic derivatives of Rhodamine B have been brought into focus because of their capability to switch between two structural forms by responding towards specific species; these two forms are fluorescence-off spirolactam form and fluorescence-on open-cycle amide form, and this has a close relationship with the decoration of molecular structure.9 The optimization of synthetic strategies, including the modification of amino groups of xanthene unit, carboxyphenyl ring and carboxylic acid group, enriched the structural composition of rhodamine dyes, which widened the application in specific fluorescence sensing field.10 For example, Prof. D. Das et al. reported a fluorescent sensor based on Rhodamine B and pyrene for detecting Al3+ in 2013.11 It was a turn-on type probe because it could transform the spirolactam form of Rhodamine B moiety into the amide form through chelation between the sensor and Al3+ ions. Moreover, considering the advantage in the convenience of recognition, fluorescence materials with sensitivity towards external stimuli provide a prospective platform to act as smart species for information security protection and data memory.12 Meanwhile, in terms of biosensors and labelling dyes, the superior emitting ability of materials in aggregated state is of great significance. However, the excellent photophysical property of many fluorescent sensors in aggregated state is weak in contrast to those in solution state due to aggregation caused quenching (ACQ), which originates from strong intermolecular π–π interactions constructing non-radiation decay channel generally.13 As a promising solution, aggregation-induced emission (AIE) effect14 was reported by Prof. B. Z. Tang in 2001 and fluorescent materials based on AIE property have been attracting significant attention for sensors and solid-state luminescence.15 For instance, in 2016, Prof. A. Misra et al. reported aggregation-induced emission enhancement (AIEE) of a fluorescent sensor, pyrene-based Schiff base, for the detection of trace-level Al3+ with detection limit of 8.64 nM. The sensor exhibited around 200-fold fluorescence enhancement with the addition of Al3+ ions because of the chelation-enhanced fluorescence and AIEE effect.16 As a consequence, sensors with the capabilities of turn-on detection of metal cations and brilliant emission in an aggregated state for practical applications are worthy of further investigation. However, very few Rhodamine B derivative-based AIE fluorescent sensors have been reported for applications of turn-on detection of metal cations.
In this study, we designed and synthesized a novel AIE-active fluorescent sensor, TPEThRB, which is composed of a tetraphenylethene unit, spirocyclic Rhodamine B derivative, and an electron-rich thiophene ring. Tetraphenylethene fragment, as a traditional AIE fluorophore, can modulate TPEThRB molecule with AIE property and offer notable steric effect via its propeller-like configuration. The rhodamine segment acts as the indicator of trivalent metal cations via the structural change from loop-locked spirolactam with no emission to open-loop amide with fluorescence emission. The thiophene unit as a conjugated bridge enriches the electron-donating ability and improves the interaction of TPEThRB molecule with metal ions.
Using turn-on fluorescence enhancement for trivalent metal cations and sensitive response towards H+ ions with clear contrast of visible color and emission, the uses of TPEThRB for chemosensing, data protection and bioimaging are explored. Compared to conventional manner of metal ion chelation-induced ring-opening process of spirocyclic derivatives of Rhodamine B,17 trivalent metal cations (Al3+, Cr3+ and Fe3+) with Lewis acid characteristics are specifically recognized by turn-on fluorescence and absorption analyses, which may be assigned to the synergy effect of protonated open-cycle and coordination interaction. Owing to the excellent luminescence properties in solid state and the response to H+ ions with clear fluorescence and visible color changes, the circle of data writing, reading and erasing based on TPEThRB molecule can be achieved by means of trifluoroacetic acid vapor as the chromogenic reagent and diethylamine vapor as the eraser. Furthermore, in terms of effective emission in intracellular environment and the remarkable red shift of maximum fluorescent peak, the application of TPEThRB sensor in bioimaging of trivalent metal ions in HeLa cells was investigated. The results of ratio fluorescence imaging indicated that TPEThRB is suitable for fluorescent imaging of trivalent metal cations in living cells. The multifunctional AIE-active fluorescent materials have triggered intense research interest and the performances of TPEThRB were investigated thoroughly in this study.
Results and discussion
The target molecule TPEThRB was synthesized through facile Schiff-base condensation reaction of compounds 1 and 2, as shown in Scheme 1. The compounds 1 and 2 were obtained by relevant reference methods.18 The molecular structure was characterized by 1H and 13C NMR spectra, HR-MS spectra, a single crystal X-ray diffractometer, and theoretical calculation.
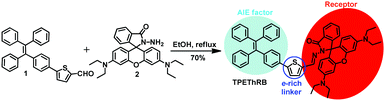 |
| Scheme 1 Synthetic route of compound TPEThRB. | |
AIE behavior and photophysical property
To demonstrate the AIE characteristics of TPEThRB molecule, fluorescent spectra of CH3CN–H2O mixture with different water fractions (fw) were investigated. The dilute CH3CN solution of TPEThRB molecule exhibited weak blue fluorescence with maximum emission peak at 467 nm (Fig. 1a) and the emission spectra exhibited negligible variation when fw in CH3CN–H2O mixture was lower than 50%. When fw increased to 60%, an intense blue-green fluorescence band with maximum emission peak at 492 nm appeared, and the maximum emission intensity of TPEThRB in CH3CN–H2O mixture with fw = 70% was around 11-fold higher than that in pure CH3CN solution (Fig. 1b).
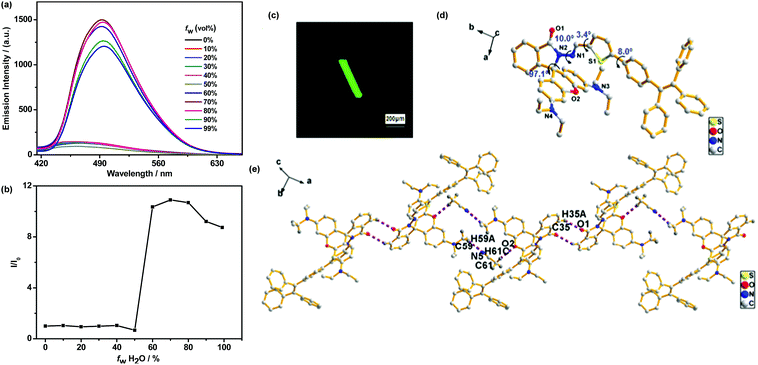 |
| Fig. 1 (a) Fluorescence spectra of TPEThRB (10 μM) in CH3CN–H2O solution with different water fractions (fw). (b) Plot of (I/I0) vs. fw, where I0 and I are the emission intensities of TPEThRB in CH3CN and CH3CN–H2O mixture. (c) Confocal laser scanning image. (d) Single-crystal structure of TPEThRB (CCDC 1814395) and (e) chain crystallography diagram.†TPEThRB single crystal was incubated in CH3CN solution and crystallographic data were collected at 296 K. | |
The AIE property was further verified by the fluorescence quantum yield (ΦF) and UV-Vis absorption spectra. The ΦF value of TPEThRB was 0.19 in dilute CH3CN solution and 6.24 in solid state, demonstrating clear enhancement in solid-state fluorescence quantum yield (αAIE = 32.8). Furthermore, the UV-Vis absorption spectra of TPEThRB in CH3CN–H2O mixtures with high water contents displayed Mie scattering effect, indicating the formation of nano-aggregates (Fig. S4, ESI†). The sizes of TPEThRB in the CH3CN–H2O mixtures with high water contents were investigated by dynamic light scattering (DLS). The DLS data demonstrated that the sizes of aggregates decrease with increasing volume fractions of water: 458, 447, 387, and 381 nm for fw = 60%, 70%, 80% and 90%, respectively (Fig. S5, ESI†); this is in agreement with the results of many other reported AIE systems.19 Herein, it is confirmed that TPEThRB exhibits AIE characteristics, which may play a great role in broadening the application of TPEThRB in the fields of sensor, data protection and bioimaging.
Fortunately, a single crystal of TPEThRB was obtained, which was assigned to the triclinic crystal system with a space group of P
. The fluorescence image from confocal laser scanning microscope showed that the crystal emitted green fluorescence and had a column-like appearance (Fig. 1c). Bright fluorescence in aggregated state and the highly twisted molecule conformation (Fig. 1d) are consistent with AIE property. In addition, ordered molecule arrangement and antiparallel packing with thiophene ring were confirmed by X-ray single-crystal analysis (Fig. S6, ESI†). The regular orientation and alignment of the single crystal of TPEThRB are derived from multiple interactions containing hydrogen bond interactions and π–π stacking. For example, the 1D chain structure (Fig. 1e) was constructed by C–H⋯N [C59–H59A⋯N5, 2.53 Å, 3.40(1) Å, 149°] and C–H⋯O [C61–H61C⋯O2, 2.53 Å, 3.38(1) Å, 147°] hydrogen bonds between the host molecules and non-bonding CH3CN and C–H⋯O [C35–H35A⋯O1, 2.55 Å, 3.31(1) Å, 139°] hydrogen bonds between adjacent host molecules. Moreover, the π–π stacking interaction between thiophene rings with a distance of 3.943(2) Å could contribute to the formation of the 2D layer structure. The C–H⋯π interaction with a distance of 3.634 (6) Å between the thiophene ring and the ethyl group was also observed. The ordered single-crystal construction of TPEThRB with column-like appearance may offer the possibility to extend applications in optical devices.
Optical response of TPEThRB probe towards ions
To check the performance of TPEThRB as a fluorescent sensor, fluorescence spectra of TPEThRB with trivalent metal cations, M3+ = Al3+, Cr3+, Fe3+, and H+ ion in CH3CN–H2O mixture (3
:
2, v/v) were investigated. As shown in Fig. 2, with the addition of trivalent metal cations and H+ ions, similar maximum emissions at 588 nm for trivalent metal cations and at 582 nm for H+ ion were observed and the mixtures presented visible color change from colorless to pink. The interaction between the sensor and the four species could reach the terminal state within six minutes at the most (Fig. S7, ESI†), accompanied with turn-on maximum fluorescence intensities (60-fold for H+, 29-fold for Al3+, 28-fold for Cr3+ and 17-fold for Fe3+), and the fluorescence quantum yields of TPEThRB in the absence and presence of metal ions were also measured: 0.17 for blank TPEThRB solution, 1.20 for H+ ion, 0.67 for Al3+ ion, 0.51 for Cr3+ ion and 0.27 for Fe3+ ion. Furthermore, on the basis of Job's plot (Fig. S8, ESI†) of [I′/I0′ − 1] versus the concentration of trivalent metal cations and H+, the estimated detection limits (LOD) by 3σ/s method were 3.2 μM for Fe3+, 4.8 μM for Al3+, 11.9 μM for Cr3+ and 0.93 μM for H+ (Fig. S9, ESI†); here, I′ and I0′ were assigned to the maximum emission intensities at 588 nm and at 582 nm in the presence and absence of trivalent metal cations and H+ ion, σ is the standard deviation of blank measurements, and s is the slope of the Job's plot. The data of LOD are at moderate level compared with that for reported sensors for trivalent metal ions (Table S1, ESI†).20 The evident fluorescence changes in the long wavelength region displayed the sensitive response of TPEThRB to the four species. In addition to more remarkable enhancement of maximum emission at 582 nm, the H+ ion has a relatively large influence on the maximum emission in the short wavelength region of TPEThRB than trivalent metal cations. The presence of H+ ion contributed to the structural transformation of the loop-locked spirolactam into an open-loop amide, resulting in extended conjugation length and improvement in intramolecular charge transfer. Accordingly, there was a moderate red shift of the maximum emission peak from 460 to 490 nm with slight increase in fluorescence intensity. In contrast, the presence of trivalent metal ions, Fe3+, Al3+ and Cr3+, had negligible effect on the emission in short wavelength region of TPEThRB due to coordination interaction. Moreover, to evaluate the reversibility of TPEThRB responding to trivalent metal ions, fluorescence spectra with the addition of disodium salt of EDTA (EDTA-2Na) were investigated. Compared to notable fluorescence enhancement of maximum emission peaks at 588 nm in the presence of trivalent metal ions, the addition of EDTA-2Na (5 equiv.) into TPEThRB–M3+ complexes resulted in 6 nm blue shift of maximum characteristic emission peak from 588 to 582 nm accompanied with sharp decrease in emission intensity (Fig. 3a). The results indicated that the coordination interaction between TPEThRB and trivalent metal ions was removed owing to better coordination affinity between EDTA-2Na and trivalent metal ions, but the effect of acidic hydrolysis on TPEThRB was still observed, which is in agreement with the proposed response mechanism of the synergy of proton-induced open-cycle and the following coordination interaction.
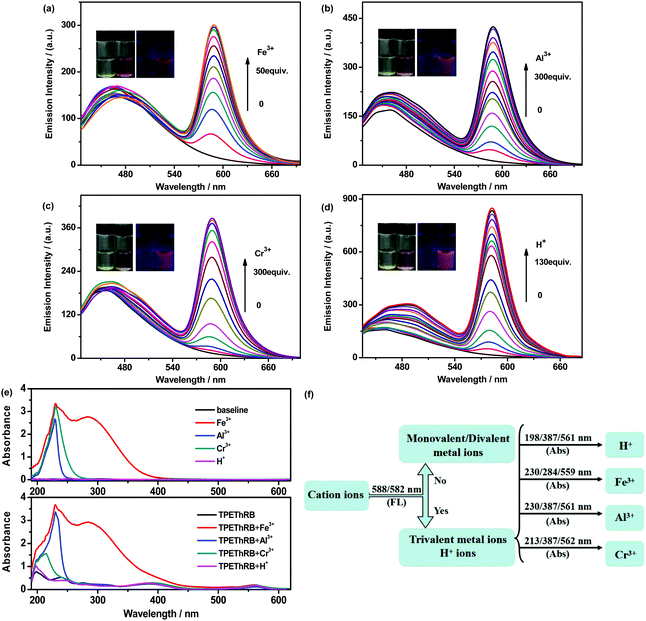 |
| Fig. 2 Fluorescence titration spectra of compound TPEThRB (10 μM) in CH3CN–H2O solution with different species (a) Fe3+, (b) Al3+, (c) Cr3+, (d) H+. Inset: The photographs of TPEThRB in CH3CN–H2O solution with Fe3+, Al3+, Cr3+ and H+ under natural light (left) and UV lamp illumination (right). (e) UV-Vis absorption spectra of compound TPEThRB (10 μM) with four species, H+, Al3+, Cr3+ and Fe3+ in CH3CN–H2O solution and only H+, Al3+, Cr3+ and Fe3+ in CH3CN–H2O solution. (f) Process scheme to recognize H+, Al3+, Cr3+, Fe3+ by using fluorescence and UV-Vis absorption spectra. | |
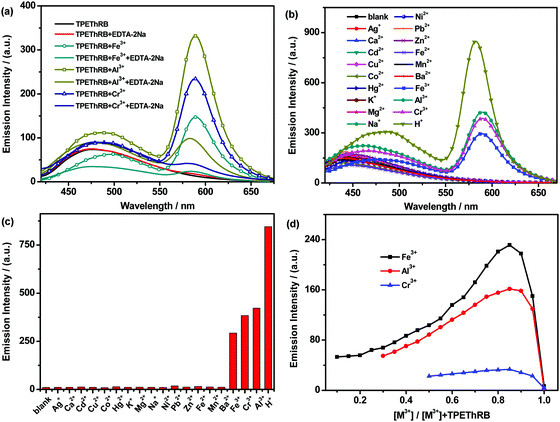 |
| Fig. 3 (a) Fluorescence spectra of TPEThRB by addition of trivalent metal ions and EDTA-2Na. (b) UV-Vis absorption spectra of TPEThRB with monovalent and divalent metal ions. (c) Histogram of fluorescence emission of compound TPEThRB (10 μM) in CH3CN–H2O solution with different valence cations and (d) the Job's plot of [M3+]/[M3+] + [TPEThRB] vs. emission intensity with a total concentration of [TPEThRB] + [M3+] = 90 μM; M3+ = Fe3+, Al3+ and Cr3+. | |
To obtain better insights into the specific response capability of TPEThRB sensor towards trivalent metal cations and H+ ions, UV-Vis absorption spectra in CH3CN–H2O mixture (3
:
2, v/v) were explored. Distinct characteristic absorption peaks appeared in the presence of trivalent metal ions and H+ ion. Based on selective matching with characteristic absorption peaks together with fluorescence spectra, it is possible to further distinguish the four ions from each other. The appearance of maximum emission peak at 588 and 582 nm is adopted to divide trivalent metal ions and H+ ion from others. In view of the intrinsic maximum absorption at 230 and 284 nm for Fe3+ as well as that at 230 nm for Al3+ and Cr3+ in CH3CN–H2O mixture, maximum absorption peaks at 559, 284 and 230 nm could be used to identify Fe3+ ion, maximum absorption peaks at 561, 387 and 230 nm may distinguish Al3+ ions, and maximum absorption peaks at 562, 387 and 213 nm could verify Cr3+ ion. In addition, the maximum absorption peaks at 561, 387 and 198 nm were assigned to H+ ion. Therefore, with the combination of the analyses of fluorescence and UV-Vis absorption spectra, we infer that the TPEThRB sensor can sensitively and individually recognize trivalent metal ions and H+ ions.
Selectivity
It is well-known that there are two forms for Rhodamine B derivatives: loop-locked spirolactam with no emission and open-loop amide with fluorescence emission.21 Upon responding towards analytes with structural transformation from the former type into the latter one, the characteristic absorption peak and characteristic fluorescence peak in the long wavelength range will be observed. Owing to the requirement of specifically detecting target species for sensors, the fluorescence and UV-Vis absorption spectra of TPEThRB interacting with various ions were investigated, and these ions include Ag+, K+, Na+, Ca2+, Cd2+, Cu2+, Co2+, Hg2+, Mg2+, Ni2+, Pb2+, Zn2+, Fe2+, Mn2+, Ba2+, Fe3+, Al3+, Cr3+ and H+. The results displayed that characteristic maximum emission peak (Fig. 3b) and characteristic maximum absorption peak (Fig. 2e and Fig. S10, ESI†) in the long wavelength region were only noticeably observed for the mixture of TPEThRB molecule with trivalent metal cations and H+ ion. Compared to the trivalent metal ions and H+ ion giving rise to open-loop response, the tested monovalent and divalent metal ions exerted no impact on the structural change of spirocyclic Rhodamine B subunit and the molecule still retained the primary loop-locked spirolactam form, due to which emission spectra and UV-Vis absorption spectra remained unchanged in the long wavelength region. Thus, TPEThRB sensor exhibits excellent selectivity concurrently with fine response capability (Fig. 3c), which is in favor of application in bioimaging.
To clarify the specific recognition of trivalent metal cations from monovalent and divalent metal cations, the response mechanism was further studied. The Job's plots of the emission intensity versus the molar fraction of M3+ in the mixture of M3+ and TPEThRB with total concentration of 90 μM exhibited the same maximum molar fraction at 0.85 (Fig. 3d), which illustrated approximate 1
:
6 ratio for the TPEThRB–M3+ complex. The abnormal stoichiometry indicated that the ring-opening is not solely induced by the coordination interaction with metal ions. Considering the steric effect modulated by propeller-like conformation of TPE moiety, compact structural architecture and nonresponse towards monovalent/divalent metal ions, the interaction affinity of TPEThRB towards metal ions may not be strong enough to trigger the conventional metal ion chelation-induced ring-opening of spirocyclic Rhodamine B subunit.22 With the assistance of acidic condition produced by the hydrolysis of trivalent metal cations, Lewis acid characteristics of Al3+, Cr3+ and Fe3+ may play a great role in the interaction of TPEThRB sensor with trivalent metal ions. As for the ability of generating protons through acidic hydrolysis, it is well known that Fe3+ is the most capable, followed by Al3+ and Cr3+ in that order.23 The order is in agreement with the estimated detection limit of TPEThRB sensor for the three trivalent metal ions. With regard to the fluorescence spectra of TPEThRB with trivalent metal ions in CH3CN–PBS mixture (10 mM, pH = 7.4), there was no obvious fluorescent response until the concentrations of trivalent metal ions were at a relatively high level (Fig. S11, ESI†), indicating that acidic condition is important to trigger the response. Meanwhile, compared to the 1H NMR spectra of blank TPEThRB sample, the –CH
N– proton displayed an evident downfield shift from 8.83 to 9.46 ppm with the addition of H+ ions. However, the characteristic –CHO– proton (9.86 ppm) of compound 1 was not observed (Fig. S12, ESI†), implying that the acidic condition results in spirolactam ring-opening rather than the hydrolysis of Schiff base because compound 1 was not regenerated. The above results further indicate that the interaction between the trivalent metal ions and TPEThRB can be ascribed to the synergy of protonated open-cycle and coordination interaction rather than the conventional mechanism of metal chelation reaction alone (Scheme 2).
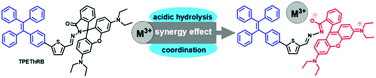 |
| Scheme 2 The proposed response mechanism of TPEThRB towards M3+. | |
Besides the appearance of characteristic absorption peak and fluorescence peak, the transformation to an open-loop amide form is conducive to alleviate the packed configuration and modulate electron distribution. The extended spatial construction of the molecule and the electron-enriched amide in combination with the conjugated thiophene linker can improve the coordination affinity between TPEThRB sensor and trivalent metal ions. The coordination interactions with the trivalent metal ions have significant influence on their UV-Vis absorption spectra, contributing to further recognize the responsive four species distinctly. Additionally, the 1H NMR spectra of TPEThRB were recorded with trivalent metal ions to further clarify the interaction between probe and metal ions (Fig. 4). The influence of the trivalent metal ions on chemical shifts was clear. In particular, the signal peak of –CH
N– proton broadened and shifted upfield to different degrees, which is consistent with a previous report that demonstrated the ring-opening of Rhodamine spirolactam and coordination interaction.24
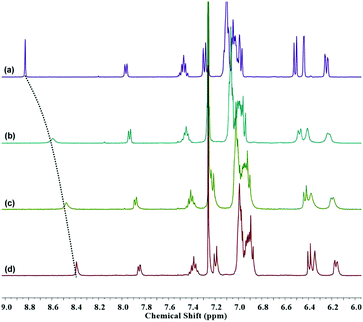 |
| Fig. 4
1H NMR spectra (in CDCl3–CD3OD) of TPEThRB in the (a) absence and presence of (b) Fe3+, (c) Al3+ and (d) Cr3+ ions. | |
Theoretical calculation
To maintain the optimized conformation, appropriate spatial arrangement can diminish the structural tension originating from the propeller-like configuration of TPE unit and compact architecture connection. To better understand the steric effect on the molecule configuration and response mechanism, DFT calculations with the B3LYP method have been performed (Fig. S13, ESI†). The calculated results of spatial configuration showed that the tricyclic subunit of spirocyclic Rhodamine B moiety was almost perpendicular to bicyclic subunit. The result is in agreement with that of single crystal analysis. The orbital electron distribution showed that the highest occupied molecular orbital (HOMO) is mainly located in tetraphenylethene and conjugated bridge, and the lowest unoccupied molecular orbital (LUMO) is distributed on partial tetraphenylethene, conjugated bridge and bicyclic segment of the spirocyclic Rhodamine B moiety. Due to almost perpendicular configuration, the tricyclic subunit of spirocyclic Rhodamine B moiety has no effective contribution towards orbital distribution, which largely impedes the extension of electron delocalization. Moreover, it is the lone pair electrons of nitrogen atom situated in tricyclic subunit that is the starting point to take part in electron rearrangement. The perpendicular configuration and steric hindrance inevitably inhibit triggering of metal ion chelation-induced ring-opening, resulting in no response to the monovalent and divalent metal ions. In contrast, the open-loop form induced by the acidic hydrolysis of trivalent metal ions effectively decreases the steric effect and increases the conjugation length, which is in favour of the interaction between TPEThRB sensor and trivalent metal ions.
Data protection
Materials with photophysical property of long emission lifetime and multi-stimuli response property, such as temperature, solvent, illumination, mechanical force and vapor, for data recording, encryption and decryption are of great value in the fields of information recording and security technologies.25 As for luminogens, which are sensitive to acid vapor, it is interesting to exploit their acidochromic property with colorimetric and fluorescent variations for practical applications.26
The AIE-active TPEThRB can respond to H+ with evident fluorescence and color changes; thus, a promising strategy to realize data recording, encryption and decryption by adopting TPEThRB as the smart material has been actualized. A strip of filter paper as a substrate was immersed in tetrahydrofuran (THF) solution of TPEThRB for a few seconds and then, we took it out and dried it by volatilizing to make TPEThRB deposit on the filter paper strip. The filter paper strip loaded with TPEThRB was covered by a template with engraving hollowed words ‘AIE’ and fumigated with trifluoroacetic acid (TFA) vapor for one minute. After removing the hollowed template, amaranthine pattern ‘AIE’ appeared on the filter paper strip together with clear contrast of fluorescent change between the blue-green emission of substrate and red-orange emission of the words ‘AIE’ (Fig. 5a and b). Moreover, the ‘AIE’ pattern on the strip of filter paper disappeared thoroughly after being exposed to diethylamine (DEA) vapor for a few seconds. The above processes represent the data writing and erasing process.
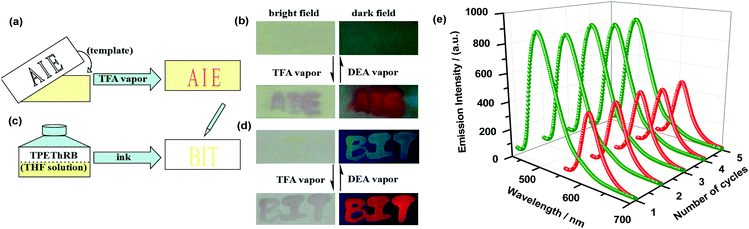 |
| Fig. 5 (a) Schematic diagrams and (b) photographs of data protection based on TPEThRB in template manner under natural light (up) and UV lamp (down); (c) diagram and (d) corresponding photograph of ink writing; (e) fluorescence spectra of TPEThRB exposed to TFA vapor and DEA vapor and reversible cycles. | |
Similarly, the THF solution of TPEThRB could also be used as a kind of encrypted ink to write words ‘BIT’ on a blank filter paper. The faint yellow words ‘BIT’ are illegible under natural light (Fig. 5c and d), whereas words with intensive blue-green fluorescent emission were clearly observed under UV lamp illumination owing to the outstanding AIE feature of the TPEThRB molecule, where the background blue luminescence originated from the blank filter paper margin under 365 nm UV light irradiation (Fig. S14, ESI†). After exposure to TFA vapor for one minute, the filter paper strip distinctly presented amaranthine words ‘BIT’ with orange-red emission; then, after fumigation with DEA vapor for a few seconds, the words ‘BIT’ on the strip of filter paper returned to the original state. The above results indicated that TFA vapor acted as a chromogenic reagent to make information emerge clearly, and DEA vapor acted as an eraser to clean out the information conversely, which could be repeated many times (Fig. 5e). Due to the performances of writing, reading and erasing based on the stimuli-responsive AIE molecules, they can serve as promising candidates for the research of data recording and information security protection.
Bioimaging
Materials with AIE characteristics are advantageous for applications in intracellular environment.27 Owing to the remarkable fluorescent responses of TPEThRB molecule towards the trivalent metal ions in CH3CN–H2O solution (Fig. S15, ESI†), the ability of TPEThRB sensor for the detection of trivalent metal cations (M3+ = Al3+, Cr3+, Fe3+) in HeLa cells was investigated by confocal fluorescence microscopy (Fig. 6). The green fluorescence channel of 450–550 nm and red fluorescence channel of 550–650 nm were selected to illustrate the performance of TPEThRB in bioimaging. The images showed that for the blank contrast group, the green fluorescence channel was bright and red fluorescence channel was dark when HeLa cells were incubated with TPEThRB sensor (10 μM) for 30 min at 37 °C. After trivalent metal ions, i.e., Al3+ (50 μM), Cr3+ (50 μM) and Fe3+ (25 μM) ions were added in the three blank culture media for incubation for another 30 min, both fluorescence channels of three test groups were visibly lightened. The ratio images of test groups having trivalent metal cations presented a striking contrast to the single channel images of the control group. The results indicated that TPEThRB sensor was capable of responding to trivalent metal ions in living cells, which is consistent with its performance in vitro. The AIE characteristics make the TPEThRB molecule retain its fine photophysical property and ratio fluorescence response ability towards trivalent metal cations within intracellular surroundings. Therefore, the AIE-active TPEThRB molecule can be a promising sensor for bioimaging of trivalent metal ions in HeLa cells.
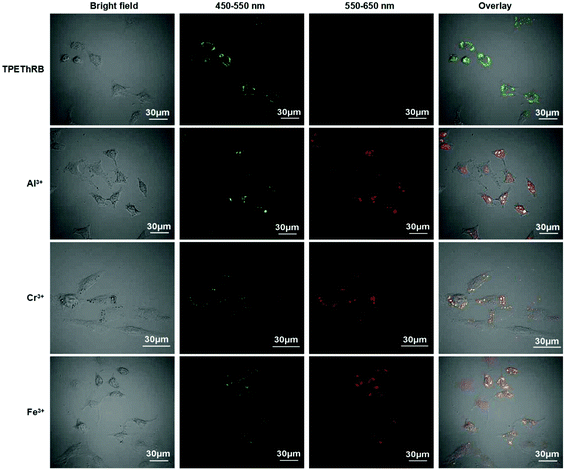 |
| Fig. 6 The confocal laser scanning images of HeLa cells incubated with TPEThRB (10 μM) and Al3+ (50 μM), Cr3+ (50 μM) and Fe3+ (25 μM) including bright-field images, fluorescence (450–550 nm channel and 550–650 nm channel) images and overlay images. | |
Conclusion
In summary, we have rationally designed and synthesized a turn-on AIE-active sensor, TPEThRB, containing tetraphenylethene with AIE characteristics, Rhodamine spirolactam as the receptor of target analytes and thiophene ring as the electron-rich conjugated linker. TPEThRB sensor can exhibit sensitive and specific turn-on response to trivalent metal cations with visible color change owing to the structural change of spirocyclic Rhodamine B moiety from a loop-locked spirolactam form to an open-loop amide form. Based on the abnormal stoichiometry of the interaction between TPEThRB and trivalent metal cations, we propose that the effective response may be ascribed to the synergy of protonated open-cycle and coordination interaction because of the steric effect caused by the propeller-like conformation of TPE unit and compact conjugated connection. A comprehensive investigation of analyses of acidic hydrolysis capability, efficient fluorescent response towards H+ ions, response delay towards trivalent metal cations in CH3CN–PBS mixture and theoretical calculation can contribute to verify this assumption. In view of excellent acidochromism property with outstanding AIE feature, the utility of TPEThRB molecule for reversible data protection was realized on the substrate of filter paper; TFA vapor and DEA vapor acted as the chromogenic reagent and eraser, respectively. Furthermore, based on the advantage of AIE characteristics and the remarkable 128 nm red-shift of maximum emission in the presence of trivalent metal ions, the collected red fluorescence channel of bioimaging in HeLa cells was lightened by the addition of trivalent metal cations. Hence it is demonstrated that TPEThRB is a viable chemosensor for trivalent metal cations in living cells. Our results indicate that AIE-active Rhodamine B-based materials will be promising candidates for multifunctional practical applications in fluorescence sensors, information protection and bioimaging fields.
Experimental section
1H and 13C NMR spectra were recorded on a Bruker ARX-400 (400 MHz) spectrometer in CDCl3 as reference using tetramethylsilane (TMS; δ = 0) as an internal standard. ESI MS was performed on an AGILENT Q-TOF 6520 liquid chromatograph mass spectrometer. Single crystal XRD was measured on an Agilent Gemini Ultra X-ray diffractometer. Fluorescence spectra and UV-Vis absorption spectra were obtained using F-7000 fluorescence spectrophotometer and UH5300 UV-Vis spectrophotometer, respectively. The theoretical calculation was performed using the Gaussian 09 suite of programs. The optimizations of the structures were performed by using B3LYP DFT. Fluorescence images of HeLa cells and single crystal of TPEThRB molecule were measured with an Olympus FluoView FV 1000 laser scanning confocal microscope.
Synthesis of compound TPEThRB
Compound 1 (0.13 g, 0.29 mmol) was added into 20 mL absolute ethanol solution of compound 2 (0.11 g, 0.25 mmol) and then, the mixture was refluxed for 8 h. After cooling to room temperature, ethanol was removed and the crude product was purified by silica gel column chromatography eluting with dichloromethane
:
petroleum ether (2
:
1) to obtain the product as a yellow solid (0.18 g, 70%). 1H NMR (400 MHz, CDCl3): δ (ppm), 8.83 (s, 1H, –N
CH–), 7.94–7.99 (d, 1H, J = 7.2 Hz, Ar-H), 7.43–7.51 (m, 2H, Ar-H), 7.27–7.31 (d, 2H, J = 8.0 Hz, Ar-H), 7.07–7.14 (m, 10H, Ar-H), 6.96–7.06 (m, 10H, Ar-H), 6.49–6.54 (d, 2H, J = 8.8 Hz, Ar-H), 6.42–6.46 (d, 2H, J = 2.0 Hz, Ar-H), 6.22–6.28 (dd, 2H, J = 8.8 Hz, 2.0 Hz, Ar-H), 3.27–3.37 (q, 8H, J = 7.2 Hz, CH2), 1.13–1.18 (t, 12H, J = 7.2 Hz, CH3). 13C NMR (100 MHz, CDCl3): δ (ppm), 164.7, 153.3, 151.5, 148.9, 146.4, 143.7, 143.6, 143.50, 143.48, 142.2, 141.3, 140.3, 140.2, 133.2, 132.0, 131.8, 131.4, 131.34, 131.31, 130.6, 129.5, 128.3, 128.0, 127.8, 127.7, 127.6, 126.6, 126.55, 126.48, 125.0, 123.8, 123.3, 122.8, 108.0, 106.1, 98.0, 66.2, 44.3, 12.6. HR-MS (ESI) (m/z): calculated for C59H52N4O2S: 881.3889, found: 881.3888 (M + H+).
Conflicts of interest
There are no conflicts to declare.
Acknowledgements
This work was financially supported by grants from the Natural Science Foundation of China (No. 21672023, 21472012, 21374010); J.-L. W. was supported by the Thousand Youth Talents Plan of China and Beijing Natural Science Foundation (2152027). The authors thank Prof. Yuping Dong (Beijing Institute of Technology) for fruitful discussion. The authors thank Analysis & Testing Center, Beijing Institute of Technology for NMR and TGA experiments.
References
-
(a) T. Shan, Y. Liu, X. Tang, Q. Bai, Y. Gao, Z. Gao, J. Li, J. Deng, B. Yang, P. Lu and Y. Ma, ACS Appl. Mater. Interfaces, 2016, 8, 28771–28779 CrossRef CAS PubMed
;
(b) J. Yang, L. Li, Y. Yu, Z. Ren, Q. Peng, S. Ye, Q. Li and Z. Li, Mater. Chem. Front., 2017, 1, 91–99 RSC
;
(c) J. Yang, J. Huang, Q. Li and Z. Li, J. Mater. Chem. C, 2016, 4, 2663–2684 RSC
;
(d) H. Luo, S. Chen, Z. Liu, C. Zhang, Z. Cai, X. Chen, G. Zhang, Y. Zhao, S. Decurtins, S.-X. Liu and D. Zhang, Adv. Funct. Mater., 2014, 24, 4250–4258 CrossRef CAS
;
(e) Z. Li, X. Yan, F. Huang, H. Sepehrpour and P. J. Stang, Org. Lett., 2017, 19, 5728–5731 CrossRef CAS PubMed
;
(f) J. J. Gruskos, G. Zhang and D. Buccella, J. Am. Chem. Soc., 2016, 138, 14639–14649 CrossRef CAS PubMed
;
(g) J. Li, Y. Chen, T. Chen, J. Qiang, Z. Zhang, T. Wei, W. Zhang, F. Wang and X. Chen, Sens. Actuators, B, 2018, 268, 446–455 CrossRef CAS
;
(h) D. Wang, H. Su, R. T. K. Kwok, G. Shan, A. C. S. Leung, M. M. S. Lee, H. H. Y. Sung, I. D. Williams, J. W. Y. Lam and B. Z. Tang, Adv. Funct. Mater., 2017, 27, 1704039 CrossRef
;
(i) G.-F. Zhang, H. Wang, M. P. Aldred, T. Chen, Z.-Q. Chen, X. Meng and M.-Q. Zhu, Chem. Mater., 2014, 26, 4433–4446 CrossRef CAS
;
(j) T. Jadhav, B. Dhokale, S. M. Mobin and R. Misra, J. Mater. Chem. C, 2015, 3, 9981–9988 RSC
.
-
(a) F. He, Y. L. Tang, S. Wang, Y. L. Li and D. B. Zhu, J. Am. Chem. Soc., 2005, 127, 12343–12346 CrossRef CAS PubMed
;
(b) M. T. Gabr and F. C. Pigge, Mater. Chem. Front., 2017, 1, 1654–1661 RSC
.
-
(a) E. House, J. Collingwood, A. Khan, O. Korchazkina, G. Berthon and C. Exleya, J. Alzheimer's Dis., 2004, 6, 291–301 CAS
;
(b) G. Berthon, Coord. Chem. Rev., 2002, 228, 319–341 CrossRef CAS
.
-
(a) H. Arakawa, R. Ahmad, M. Naoui and H.-A. Tajmir-Riahi, J. Biol. Chem., 2000, 275, 10150–10153 CrossRef CAS PubMed
;
(b) J. B. Vincent, Nutr. Rev., 2000, 58, 67–72 CrossRef CAS
.
-
(a) S. K. Sahoo, D. Sharma, R. K. Bera, G. Crisponi and J. F. Callan, Chem. Soc. Rev., 2012, 41, 7195–7227 RSC
;
(b) X. Yang, X. Chen, X. Lu, C. Yan, Y. Xu, X. Hang, J. Qu and R. Liu, J. Mater. Chem. C, 2016, 4, 383–390 RSC
.
-
(a) G. Balamurugan, S. Velmathi, N. Thirumalaivasan and S. P. Wu, Analyst, 2017, 142, 4721–4726 RSC
;
(b) A. Anwara, A. Minhazc, N. A. Khana, K. Kalantari, A. B. M. Afifid and M. R. Shahb, Sens. Actuators, B, 2018, 257, 875–881 CrossRef
;
(c) H. Li, P. Zhang, L. P. Smaga, R. A. Hoffman and J. Chan, J. Am. Chem. Soc., 2015, 137, 15628–15631 CrossRef CAS PubMed
;
(d) L. Li, B. Yu and T. You, Biosens. Bioelectron., 2015, 74, 263–269 CrossRef CAS PubMed
;
(e) P. C. Dhara, A. Pal, P. Mohanty and B. Bag, Sens. Actuators, B, 2015, 219, 308–314 CrossRef
;
(f) A. K. Dwivedi, M. Pandeeswar and T. Govindaraju, ACS Appl. Mater. Interfaces, 2014, 6, 21369–21379 CrossRef CAS PubMed
.
-
(a) D. Ma, B. Li, Z. Cui, K. Liu, C. Chen, G. Li, J. Hua, B. Ma, Z. Shi and S. Feng, ACS Appl. Mater. Interfaces, 2016, 8, 24097–24103 CrossRef CAS PubMed
;
(b) A. S. Klymchenko, Acc. Chem. Res., 2017, 50, 366–375 CrossRef CAS PubMed
;
(c) E. A. Owens, M. Henary, G. E. Fakhri and H. S. Choi, Acc. Chem. Res., 2016, 49, 1731–1740 CrossRef CAS PubMed
;
(d) S. Shanmugaraju and P. S. Mukherjee, Chem. Commun., 2015, 51, 16014–16032 RSC
.
-
(a) L. Wang, W. Li, W. Zhi, D. Ye, Y. Wang, L. Ni and X. Bao, Dyes Pigm., 2017, 147, 357–363 CrossRef CAS
;
(b) Q. Sun, W. Wang, Z. Chen, Y. Yao, W. Zhang, L. Duan and J. Qian, Chem. Commun., 2017, 53, 6432–6435 RSC
;
(c) D. Karak, A. Banerjee, S. Lohar, A. Sahana, S. K. Mukhopadhyay, S. S. Adhikari and D. Das, Anal. Methods, 2013, 5, 169–172 RSC
;
(d) K. Gu, Y. Xu, H. Li, Z. Guo, S. Zhu, S. Zhu, P. Shi, T. D. James, H. Tian and W.-H. Zhu, J. Am. Chem. Soc., 2016, 138, 5334–5340 CrossRef CAS PubMed
.
-
(a) G. Sivaraman and D. Chellappa, J. Mater. Chem. B, 2013, 1, 5768–5772 RSC
;
(b) Z. Li, Z. Xue, Z. Wu, J. Han and S. Han, Org. Biomol. Chem., 2011, 9, 7652–7654 RSC
;
(c) M. Yu, R. Yuan, C. Shi, W. Zhou, L. Wei and Z. Li, Dyes Pigm., 2013, 99, 887–894 CrossRef CAS
;
(d) H. N. Kim, M. H. Lee, H. J. Kim, J. S. Kim and J. Yoon, Chem. Soc. Rev., 2008, 37, 1465–1472 RSC
.
- M. Beija, C. A. M. Afonso and J. M. G. Martinho, Chem. Soc. Rev., 2009, 38, 2410–2433 RSC
.
- A. Sahana, A. Banerjee, S. Lohar, A. Banik, S. K. Mukhopadhyay, D. A. Safin, M. G. Babashkina, M. Bolte, Y. Garcia and D. Das, Dalton Trans., 2013, 42, 13311–13314 RSC
.
-
(a) J. Y. Hu, J. Wang, R. Liu, Y. Li, J. P. Lu and H. J. Zhu, Dyes Pigm., 2018, 153, 84–91 CrossRef CAS
;
(b) S.-W. Cheng, T. Han, T.-Y. Huang, Y.-H. C. Chien, C.-L. Liu, B. Z. Tang and G.-S. Liou, ACS Appl. Mater. Interfaces, 2018, 10, 18281–18288 CrossRef CAS PubMed
.
-
(a) L. Zong, Y. Xie, C. Wang, J.-R. Li, Q. Li and Z. Li, Chem. Commun., 2016, 52, 11496–11499 RSC
;
(b) D. Li, Y. Zhang, Z. Fan, J. Chen and J. Yu, Chem. Sci., 2015, 6, 6097–6101 RSC
;
(c) Y. Yuan and B. Liu, Chem. Sci., 2017, 8, 2537–2546 RSC
;
(d) M. Huang, R. Yu, K. Xu, S. Ye, S. Kuang, X. Zhu and Y. Wan, Chem. Sci., 2016, 7, 4485–4491 RSC
;
(e) S. Li, M. Gao, S. Wang, R. Hu, Z. Zhao, A. Qin and B. Z. Tang, Chem. Commun., 2017, 53, 4795–4798 RSC
.
- J. Luo, Z. Xie, J. W. Y. Lam, L. Cheng, H. Chen, C. Qiu, H. S. Kwok, X. Zhan, Y. Liu, D. Zhu and B. Z. Tang, Chem. Commun., 2001, 1740–1741 RSC
.
-
(a) S. Chen, Q. Chen, Q. Li, J. An, P. Sun, J. Ma and H. Gao, Chem. Mater., 2018, 30, 1782–1790 CrossRef CAS
;
(b) W. Qin, Z. Yang, Y. Jiang, J. W. Y. Lam, G. Liang, H. S. Kwok and B. Z. Tang, Chem. Mater., 2015, 27, 3892–3901 CrossRef CAS
;
(c) S. Tian, G. Liu, X. Wang, T. Wu, J. Yang, X. Ye, G. Zhang, J. Hu and S. Liu, ACS Appl. Mater. Interfaces, 2016, 8, 3693–3702 CrossRef CAS PubMed
;
(d) G. Liang, F. Ren, H. Gao, F. Zhu, Q. Wu and B. Z. Tang, J. Mater. Chem. A, 2017, 5, 2115–2122 RSC
;
(e) H. Liu and H. Liu, J. Mater. Chem. A, 2017, 5, 9156–9162 RSC
.
- M. Shyamal, P. Mazumdar, S. Maity, G. P. Sahoo, G. Salgado-Morán and A. Misra, J. Phys. Chem. A, 2016, 120, 210–220 CrossRef CAS
.
- S. Park, W. Kimb, K. M. K. Swamy, H. Y. Lee, J. Y. Jung, G. Kima, Y. Kima, S.-J. Kima and J. Yoon, Dyes Pigm., 2013, 99, 323–328 CrossRef CAS
.
-
(a) M. Gao, Y. Li, X. Chen, S. Li, L. Ren and B. Z. Tang, ACS Appl. Mater. Interfaces, 2018, 10, 14410–14417 CrossRef CAS
;
(b) Y. Cheng, J. Dai, C. Sun, R. Liu, T. Zhai, X. Lou and F. Xia, Angew. Chem., Int. Ed., 2018, 57, 3123–3127 CrossRef CAS PubMed
.
-
(a) L. Wang, Y. Shen, Q. Zhu, W. Xu, M. Yang, H. Zhou, J. Wu and Y. Tian, J. Phys. Chem.
C, 2014, 118, 8531–8540 CrossRef CAS
;
(b) M. Shyamal, S. Maity, P. Mazumdar, G. P. Sahoo, R. Maity and A. Misra, J. Photochem. Photobiol., A, 2017, 342, 1–14 CrossRef CAS
;
(c) L. Wang, Y. Shen, Q. Zhu, W. Xu, M. Yang, H. Zhou, J. Wu and Y. Tian, J. Phys. Chem. C, 2014, 118, 8531–8540 CrossRef CAS
.
-
(a) A. J. Weerasinghe, C. Schmiesing, S. Varaganti, G. Ramakrishna and E. Sinn, J. Phys. Chem. B, 2010, 114, 9413–9419 CrossRef CAS PubMed
;
(b) W. Lin, L. Long, L. Yuan, Z. Cao and J. Feng, Anal. Chim. Acta, 2009, 634, 262–266 CrossRef CAS
;
(c) N. Narayanaswamy and T. Govindaraju, Sens. Actuators, B, 2012, 161, 304–310 CrossRef CAS
;
(d) J. Wang, Y. Fan, H.-W. Lee, C. Yi, C. Cheng, X. Zhao and M. Yang, ACS Appl. Nano Mater., 2018, 1, 3747–3753 CrossRef CAS
;
(e) Y. Yang, X. Song, C. Xu, Y. Wang, G. Zhang and W. Liu, Dalton Trans., 2018, 47, 11077–11083 RSC
;
(f) L.-M. Liu and Z.-Y. Yang, Inorg. Chim. Acta, 2018, 469, 588–592 CrossRef CAS
;
(g) H. J. Jang, J. H. Kang, D. Yun and C. Kim, J. Fluoresc., 2018, 28, 785–794 CrossRef CAS
;
(h) H. Xu, M. Fang, C.-S. Cao, W.-Z. Qiao and B. Zhao, Inorg. Chem., 2016, 55, 4790–4794 CrossRef CAS
;
(i) S. Zeng, S.-J. Li, X.-J. Sun, M.-Q. Li, Y.-Q. Ma, Z.-Y. Xing and J.-L. Li, Spectrochim. Acta, Part A, 2018, 205, 276–286 CrossRef CAS
;
(j) M.-C. Tu, D. Rajwar, G. Ammanath, P. Alagappan, U. H. Yildiz and B. Liedberg, Anal. Chim. Acta, 2016, 912, 105–110 CrossRef CAS
;
(k) X. Huang, C. Fan, Z. Wang, X. Zhan, M. Pei and Z. Lu, Inorg. Chem. Commun., 2015, 57, 62–65 CrossRef CAS
;
(l) T. Rasheed, C. Li, L. Fu, F. Nabeel, C. Yu, L. Gong and Y. Zhou, J. Mol. Liq., 2018, 272, 440–449 CrossRef CAS
;
(m) H. Jin, J. Xu, L. Zhang, B. Ma, X. Shi, Y. Fan and L. Wang, J. Solid State Chem., 2018, 268, 168–174 CrossRef CAS
;
(n) V. Bravo, S. Gil, A. M. Costero, M. N. Kneeteman, U. Llaosa, P. M. E. Mancini, L. E. Ochando and M. Parra, Tetrahedron, 2012, 68, 4882–4887 CrossRef CAS
;
(o) N. Manjubaashini, T. D. Thangadurai, G. Bharathi and D. Nataraj, J. Fluoresc., 2018, 202, 282–288 CAS
.
- H. N. Kim, M. H. Lee, H. J. Kim, J. S. Kim and J. Yoon, Chem. Soc. Rev., 2008, 37, 1465–1472 RSC
.
- X. Chen, T. Pradhan, F. Wang, J. S. Kim and J. Yoon, Chem. Rev., 2012, 112, 1910–1956 CrossRef CAS PubMed
.
- X. Chen, X. Y. Shen, E. Guan, Y. Liu, A. Qin, J. Z. Sun and B. Z. Tang, Chem. Commun., 2013, 9, 1503–1505 RSC
.
- X. Zeng, L. Dong, C. Wu, L. Mu, S.-F. Xu and Z. Tao, Sens. Actuators, B, 2009, 141, 506–510 CrossRef CAS
.
-
(a) H. Sun, S. Liu, W. Lin, K. Y. Zhang, W. Lv, X. Huang, F. Huo, H. Yang, G. Jenkins, Q. Zhao and W. Huang, Nat. Commun., 2014, 5, 4601 CrossRef
;
(b) A. Kishimura, T. Yamashita, K. Yamaguchi and T. Aida, Nat. Mater., 2005, 4, 546–549 CrossRef CAS
;
(c) T. Mutai, H. Satou and K. Araki, Nat. Mater., 2005, 4, 685–687 CrossRef CAS
;
(d) Y. Wu, Y. Xie, Q. Zhang, H. Tian, W. Zhu and A. D. Q. Li, Angew. Chem., Int. Ed., 2014, 53, 2090–2094 CrossRef CAS
;
(e) D. J. Wales, Q. Cao, K. Kastner, E. Karjalainen, G. N. Newton and V. Sans, Adv. Mater., 2018, 30, 1800159 CrossRef
;
(f) T. Butler, W. A. Morris, J. Samonina-Kosicka and C. L. Fraser, ACS Appl. Mater. Interfaces, 2016, 8, 1242–1251 CrossRef CAS
;
(g) Z. Song, R. Liu, Y. Li, H. Shi, J. Hu, X. Cai and H. Zhu, J. Mater. Chem. C, 2016, 4, 2553–2559 RSC
;
(h) Y. Li, L. Chen, Y. Ai, E. Y.-H. Hong, A. K.-W. Chan and V. W.-W. Yam, J. Am. Chem. Soc., 2017, 139, 13858–13866 CrossRef CAS
;
(i) S. Mo, Q. Meng, S. Wan, Z. Su, H. Yan, B. Z. Tang and M. Yin, Adv. Funct. Mater., 2017, 27, 1701210 CrossRef
;
(j) C. Wang and Z. Li, Mater. Chem. Front., 2017, 1, 2174–2194 RSC
.
-
(a) P. Xue, J. Ding, Y. Shen, H. Gao, J. Zhao, J. Sun and R. Lu, J. Mater. Chem. C, 2017, 5, 11532–11541 RSC
;
(b) M. E. Genovese, A. Athanassiou and D. Fragouli, J. Mater. Chem. A, 2015, 3, 22441–22447 RSC
;
(c) F. Nisic, A. Colombo, C. Dragonetti, M. Fontani, D. Marinotto, S. Righetto, D. Roberto and J. A. G. Williams, J. Mater. Chem. C, 2015, 3, 7421–7427 RSC
.
-
(a) D. D. La, S. V. Bhosale, L. A. Jones and S. V. Bhosale, ACS Appl. Mater. Interfaces, 2018, 10, 12189–12216 CrossRef CAS PubMed
;
(b) N. N. Li, J. Z. Li, P. Liu, D. Pranantyo, L. Luo, J. C. Chen, E.-T. Kang, X. F. Hu, C. M. Li and L. Q. Xu, Chem. Commun., 2017, 53, 3315–3318 RSC
.
Footnote |
† Electronic supplementary information (ESI) available: Characterization of NMR and MS spectra, UV-Vis absorption and fluorescence spectra, and crystallographic data. CCDC 1814395. For ESI and crystallographic data in CIF or other electronic format see DOI: 10.1039/c8qm00424b |
|
This journal is © the Partner Organisations 2019 |
Click here to see how this site uses Cookies. View our privacy policy here.