DOI:
10.1039/C7RA08939B
(Review Article)
RSC Adv., 2017,
7, 46999-47016
Unveiling a versatile heterocycle: pyrazoline – a review
Received
12th August 2017
, Accepted 22nd September 2017
First published on 5th October 2017
Abstract
The design and synthesis of novel fluorescent heterocyclic dyes is a “hotspot” research area, due to their favourable photophysical properties and crucial role in charge transfer processes, which could allow huge advances in the fields of physics, chemistry and biology. One group of electron-rich nitrogen carriers, pyrazolines, are enjoying brisk growth, since they combine exciting electronic properties with the potential for dynamic applications. Concerning multi-functional applications, pyrazolines could provide a new range of applications that would revolutionize various fields. As reported in many studies, the potential applications of pyrazolines are vast and ever growing, but they have yet to make in-roads into real-life applications. This review provides an overview on the current status of pyrazoline derivatives in terms of synthesis, physico-chemical properties and various applications in order to realize their full potential for practical applications, and to trigger the synthesis and study of novel pyrazoline based compounds with much promise. We also present our conclusions and outlook for the future.
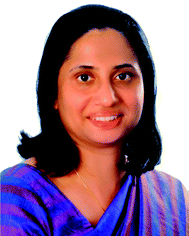 Beena Varghese | Beena Varghese received her Master's degree in Analytical Chemistry from Mahatma Gandhi University (India) in 2004. She completed her PhD (2015) and Post-doctoral fellowship (2016) at Sultan Qaboos University (Oman) in the research group of Prof. Salma Al-Kindy. Presently, she is working as a visiting faculty member in the Department of Chemistry at Sultan Qaboos University, and her research interests include novel fluorescent labels, their analytical applications, and fluorescent organic nanoparticles based on electron rich heterocyclic rings for application in biomedicine, sensors and in opto-electronics. |
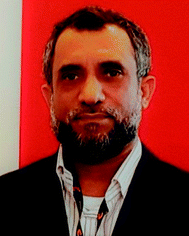 Saleh N. Al-Busafi | Saleh Nasser Al-Busafi obtained his MSc (1994) and a PhD (1999) in Organic Chemistry from the University of Reading, Reading, United Kingdom. He has been a member of the RSC (MRSC) since 2012. He joined the Department of Chemistry at Sultan Qaboos University in Sultanate of Oman, in 1991, where he is now an Associate Professor. His research interests include isolation of natural products and the synthesis of fluorescent organic labels. |
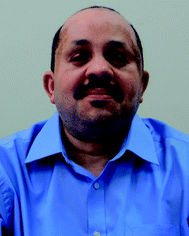 FakhrEldin O. Suliman | FakhrEldin Suliman obtained an MSc (1992) and a PhD (1996) in Analytical Chemistry from King Fahd University of Petroleum and Minerals, Dhahran, Saudi Arabia. He was awarded a Science and Technology Agency (STA) Fellowship at the National Institute for Environmental Studies, Japan, in 1998. He joined the Department of Chemistry at Sultan Qaboos University in Sultanate of Oman, in 1999 where he is now Professor and Head of Department. His research interests include developing fluorescent probes, miniaturization of analytical techniques, and supramolecular chemistry. |
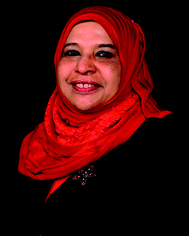 Salma M. Z. Al-Kindy | Salma Al-Kindy obtained her B.Sc (Honors) (1982) in Chemistry from American University of Cairo, Egypt and PhD in Chemistry from Loughborough University, England in 1987. She started her academic career in 1989 at Sultan Qaboos University (Oman) and received a lot of academic awards and international recognition. Currently, she is the Dean of the College of Science, Sultan Qaboos University and Professor of Analytical Chemistry. Prof. Salma's research interest has been in developing analytical protocols for the monitoring of analytes in complex matrices and in developing sensitive and selective methods for the assay of pharmaceutical components using microfluidic systems. |
1. Introduction
1.1. A quick look at the general-to-specific background of pyrazolines
In the impulse to build novel molecules possessing vibrant, versatile and beneficial properties, an elegant member of the heterocyclic family called pyrazoline was found to be appealing for researchers. From those advances, a wide array of synthetic structures comprising the pyrazoline moiety have been successfully designed and developed, which exhibit diverse and tunable properties depending on the mode of synthesis and the molecular structures (Fig. 1). Pyrazolines are also proving to be highly versatile performers in various applications such as brightening agents in synthetic fibers, papers and textiles, fluorescent probes in some elaborate chemo-sensors, recognition of transition metal ions, hole-transport material, organic electronics, electrophotography and electroluminescence.1–11 It therefore comes as no surprise that as a privileged scaffold, pyrazolines are widely incorporated into the structures of numerous important medical and biochemical agents that have been effectively utilized as anti-bacterial, anti-fungal, anti-viral, anti-parasitic, anti-tubercular, anti-inflammatory, anti-cancer, anesthetic, analgesic and insecticidal agents.12–26
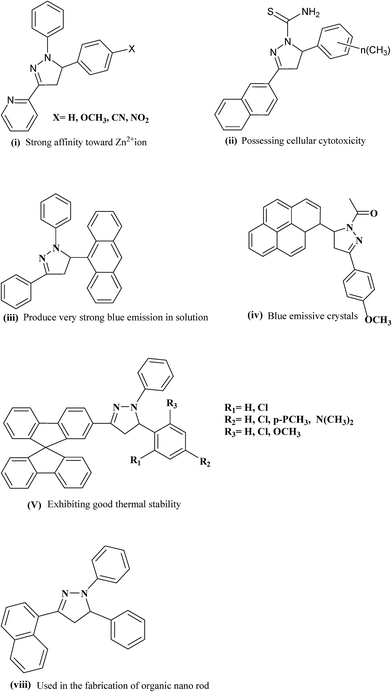 |
| Fig. 1 Structures of some pyrazoline derivatives: (i) 3-(2-pyridyl)-2-pyrazoline; (ii) 3-naphthalen-2-yl-5-methoxyphenyl-pyrazoline-1-carbothioamide; (iii) 1,3-diphenyl-5-(9-anthryl)-2-pyrazoline; (iv) 1-acetyl-3-(4-methoxyphenyl)-5-(1-pyrenyl)-pyrazoline; (v) 1,5-diphenyl-3-spirobifluorenyl pyrazoline; (vi) 3-(1-benzothiazol-2-yl-5-furan-2yl-4,5-dihydro-1H-pyrazol-3yl)-4-hydroxy-1H-quinoline-2-one; (vii) 1,3,5-triphenyl-2-pyrazoline (viii) 1,5-diphenyl-3-(naphthalene-4-yl)-1H-pyrazoline. | |
The name pyrazole was given by Ludwig Knorr in 1883 and refers to the class of simple aromatic ring organic compounds of the heterocyclic series, characterized by a 5-membered ring structure composed of three carbon atoms and two nitrogen atoms in adjacent positions.27
In the 19th century, Fischer and Knoevenagel synthesized and characterized 2-pyrazolines, utilizing the remarkably simple reflux reaction of α,β-unsaturated aldehydes and ketones with phenyl hydrazine in acetic acid, which became one of the most popular methods. There have been many efforts to utilize pyrazoline as a synthetic building block in the design of good lead compounds in the library of new heterocyclic compounds; however, its green status is questionable.28–33 In the chemical literature these days, there have been more attempts at showcasing the synthetic procedures targeting pyrazoline compounds that are claimed to be green by the authors.34–39
Since the release of a bright blue-light emitting pyrazoline molecule with tunable photophysical properties, several innovative compounds on this scaffold have been introduced for various applications. The theoretical prediction of a great variety of molecular properties such as molecular structures, vibrational frequencies, atomization energies, ionization energies, electric and magnetic properties and reaction paths is likely to provide new fundamental insight as well as to realize the full potential of applications. In terms of electron donating ability, a pyrazoline derivative relies on the nitrogen with lone pairs located in position-1 (N-1) in the pyrazoline ring; also, varying the substituents attached to the N-1 atom was found to display relevant changes in the photophysical properties of pyrazoline compounds. The advantages of modulating the optical properties of pyrazoline compounds can also be refined by altering the substitution pattern in the C-3 and C-5 phenyl rings.40 There is a long-standing interest in the photophysics of N-phenyl substituted five membered rings and their derivatives in aqueous/non-aqueous solvents as well as in various micro heterogeneous media, due to the possibility for charge transfer (CT) emission. On studying the effect of the non-conjugated 5-position substituent of pyrazoline-based photosensitizers on the photophysical characteristics and performance of dye-sensitized solar cells (DSSCs), a trend of increasing absorption maxima, electrochemical characteristics and DSSC performances was observed with increasing electron-donating ability.41
The pervasiveness of this heterocycle leads to multiple useful properties and has stimulated the need for facile and efficient ways to make these heterocyclic cores. Moreover, the incorporation of the pyrazoline moiety and other electron-donors/acceptors into one molecule is one of the most actively pursued areas of research involving fascinating diverse heterocycles, and seems to be a good choice for many applications. The incorporation of different substituents into the pyrazoline parent nucleus is quite interesting, since it increases the conjugation length, due to the presence of an additional double bond in alternate arrangements with the phenyl ring, prompting researchers to synthesize and elucidate the effects of substituents on the absorption and fluorescence properties of this class of compounds.42 Compared to the unsubstituted parent molecule, the incorporation of electron-donors and electron-acceptors into one molecule leads to endowment with structural diversity, stability and highly improved optical properties. Of the factors influencing the nonlinear optical features of push–pull type pyrazoline based chromophores, pride of place must go to the position as well as the number and type of the electron-acceptor groups attached to the molecular structure (Fig. 2).43
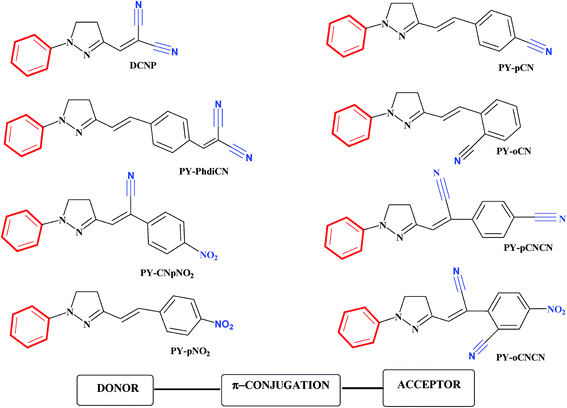 |
| Fig. 2 Chemical structures of some push–pull type pyrazoline based chromophores. The donor (D) and the acceptor (A) are marked in red and blue, respectively. The compounds shown are (3-(1,1-dicyanoethenyl)-1-phenyl-4,5-dihydro-1H-pyrazole) (DCNP); (E)-2-(4-(1-phenyl-4,5-dihydro-1H-pyrazol-3-yl)vinyl)benzonitrile (PY-pCN); (E)-2-(4-(2-(1-phenyl-4,5-dihydro-1H-pyrazol-3-yl)vinyl)benzylidene)malononitrile (PY-PhdiCN); (E)-2-(2-(1-phenyl-4,5-dihydro-1H-pyrazol-3-yl)vinyl)benzonitrile (PYoCN); ((Z)-2-(4-nitrophenyl)-3-(1-phenyl-4,5-dihydro-1H-pyrazol-3-yl)acrylonitrile) (PYCNpNO2); (Z)-2-(1-cyano-4-(1-phenyl-4,5-dihydro-1H-pyrazol-3-yl)vinyl)benzonitrile (PY-pCNCN); (E)-3-(4-nitrostyryl)-1-phenyl-4,5-dihydro-1H-pyrazole (PY-pNO2); (Z)-2-(1-cyano-2-(1-phenyl-4,5-dihydro-1H-pyrazol-3-yl)vinyl)benzonitrile (PY-oCNCN).43 | |
The geometries of many pyrazoline derivatives have been characterized crystallographically and are shown to be driven by the establishment of CH–π interactions, consequently resulting in the stacking of the molecules to be packed as a crystal; these types of interactions have been found to be strong enough to stabilize a particular conformation of molecules for their higher-order self-assembly.44–47 The significant findings from the detailed crystal analysis of some pyrazoline molecules suggest that the stacking modes of molecules and intermolecular electronic interactions provide significant prospects in the tuning of solid-state fluorescence.48,49 One of the well-known characteristics of the whole family of pyrazoline derivative compounds is the existence of geometrical isomers, first noticed by Tsitovich during the synthesis of 5,5-dimethylpyrazolines nearly five decades ago.50 (E)-3-(4-Nitrostyryl)-1-phenyl-4,5-dihydro-1H-pyrazole (PY-pNO2) provides a lasting example of the existence of two different polymorphic forms as the bent cis-type and the rod-like trans-type; an indication of two ground state photo isomers of PY-pNO2 in solution. Quantum chemical calculations gave insight into the switching of trans 1 → cis 1 and trans 2 → cis 2 isomers, and also predicted the possibility of four ground state conformers trans 1, trans 2, cis 1 and cis 2 with different ground state energies (Fig. 3).51 It has been well recognized for some time that stereochemistry plays a decisive role in controlling the insecticidal effects of some carbamoylated and acylated pyrazolines.52
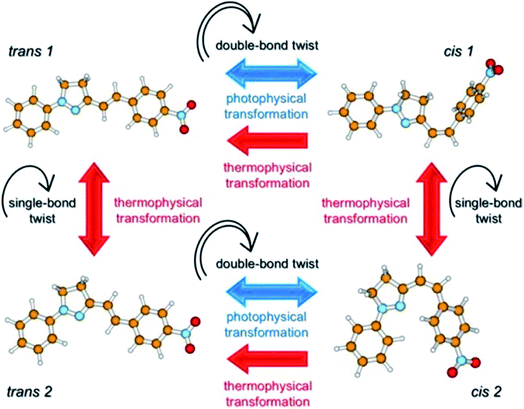 |
| Fig. 3 Scheme showing the photophysics of PY-pNO2. The transitions due to double bond twisting are possible only via photophysical transformation after photon absorption, while those due to single bond twisting may occur thermally. Reprinted with permission from ref. 51. Copyright 2016 American Chemical Society. | |
The tautomeric behaviors of some pyrazole-carbothioamide and pyrazole-carboxamide compounds have been discussed in detail, with the support of both experimental and computational studies, using density functional theory (DFT) and FT-Raman and NMR techniques.53 Several studies have described the thermal stability and electrochemical performance of compounds clubbed with pyrazolines with specific applications.
The fact that the pyrazoline ring itself is a photoactive unit should be taken into account as an important aspect for its electrochemical applications. Cyclic and Osteryoung square wave voltammetry studies indicated that some pyrazolino [60] fullerenes (Fig. 4) had improved electron affinity in comparison with the parent fullerenes. Promising data have been obtained from second harmonic a.c. (alternating current) voltammetry of 2-pyrazoline structures bonded to tin oxide (SnO2) surface.54–56
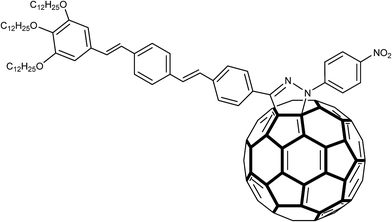 |
| Fig. 4 Structure of a pyrazolino [60] fullerene in which an oligophenylenevinylene (OPV) trimeric subunit was attached to C60 through a pyrazoline ring. | |
It is well documented that pyrazolines outperform many legendary functional units in terms of synthesis, properties and novel applications; in this article we will review the role that the pyrazolines have played in the development of some significant research areas that focus on functional small organic molecules based on the pyrazoline core skeleton. This review begins by highlighting some key synthetic procedures targeting pyrazoline derivatives and then briefly introducing some green synthetic approaches. We survey the applications by looking at how intramolecular charge transfer processes (ICT) in pyrazoline rings occur and become associated with other ICT units for a comprehensive gathering. Also, representative references are discussed by focusing on the intrinsic blue emission and solvatochromic behavior of pyrazoline derivatives. We then briefly cover how as a tunable functional unit, the pyrazoline ring at present offers the greatest versatility and the successful application of pyrazoline derivatives in several fields. Finally, we discuss our personal view on the possible future research opportunities to improve the performance in various exciting research fields.
2. Pyrazolines: understanding the most popular heterocyclic ring
2.1. Synthetic routes: conventional to green
Pyrazolines are extremely valuable nitrogen-containing heterocyclic compounds that exist in a variety of chemical and biological agents and enhance their activities; as such, various reaction protocols have been worked out for their synthesis. Among the methods employed in the synthesis of pyrazolines, an especially popular procedure is based on the reaction of α,β-unsaturated aldehydes and ketones with hydrazine reagents under various conditions. In this method, hydrazones are formed as intermediates, which can be subsequently cyclized to 2-pyrazolines in the presence of a suitable cyclizing agent like acetic acid. Thus far, this simple and convenient procedure has remained one of the most popular methods for the preparation of 2-pyrazolines (Scheme 1) and modified versions of this synthetic strategy have been reported.57–60 1,3-Dipolar cycloaddition was employed in the synthesis of 4,5-dihydro-3-(substituted imidazole)-5-substituted-1-phenyl-1H-pyrazoline. In the second step of this reaction, the nitrile imine was obtained by the oxidative dehydrogenation of the phenyl hydrazone derivative with chloramines-T (CAT), and the olefin-trapped nitrile imine led to the resulting compound in good quality and yield under refluxing conditions in ethanol. The synthesis of fine crystals of 3,4,5-triethoxycarbonyl-2-pyrazoline by the introduction of palladium chloride (PdCl2) to ethyl diazoacetate (EDA) in a high-pressure reactor at ambient temperature seems to be an interesting synthetic approach. It was reported that the “one-pot procedure” can be useful to surmount challenging classical heating methods, and was well demonstrated in the synthesis of 3-[4′-(4′′-nitrophenoxy)-phenyl]-5-(substituted aryl)-2-pyrazoline-1-carboxaldehydes. The interested reader is referred to some articles and previous reviews on synthesis for additional details.61–66
 |
| Scheme 1 General synthesis of pyrazoline compounds. (a) Aldehyde, KOH/K2CO3, EtOH, reflux, 2 h. (b) Phenyl hydrazine, ethanol, 3 h, dil. sulphuric acid. | |
Under the framework of “Green Chemistry”, researchers address the new challenge of synthesizing pyrazoline derivatives by adopting new reaction conditions that reduce the use of volatile organic solvents and hazardous toxic chemicals. A new catalytic application of tungstate sulfuric acid (TSA) as a highly efficient and green solid acid catalyst to synthesize pyrazoles was proposed.67 Cellulose sulfonic acid (CSA), was also found to be able to cyclize α,β-unsaturated hydrazones to pyrazolines in excellent yields under green reaction conditions.68 The examples of “on water” synthesis of N-unsubstituted pyrazoles, 2-pyrazoline derivatives under solvent-free conditions, using the grinding technique, ultrasonic irradiation to yield fluorinated pyrazoles and the microwave (MW) irradiation technique with short workup procedure, direct us towards a positive path to green alternative methods, over the conventional synthesis.69–72
The idea that replacing a ‘non-green’ solvent and reagents in a process with a ‘green’ one is highly attractive, but the major drawback of these methods is that obtaining most of the reagents and solvents used in the green synthesis is a herculean task.
2.2. ICT and novel D–π–A systems
The intramolecular charge transfer process (ICT) has attracted the most attention for finding potential applications of pyrazoline derivatives. From this single starting point have come investigations on the opto-electronic properties, which involve many physico-chemical modifications that can be precisely achieved through fluorescence spectroscopy along with other techniques. A key theme involved in the charge transfer mechanism of pyrazoline is the competition between the N1 → N2 → C3 conjugate charge transfer and the N1 → C5 non-conjugate charge transfer (Fig. 5). Since these types of compounds have a heterocyclic nitrogen atom, transitions involving the non-bonding nitrogen electrons have similar properties to those of π–π* transitions, as the n orbital generally overlaps the π-orbital of the adjacent carbon atom. Along with the charge transfer, increased charge densities may also be developed for the atoms N-2 and C-3 of the pyrazoline ring in the first excited state S1. However, the introduction of substituents on the phenyl radical in position 5 of the pyrazoline ring seems to not be conjugated with the basic chromophoric system of the molecule and is almost orthogonal to it.73
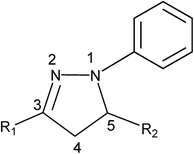 |
| Fig. 5 Pyrazoline ring. | |
Modulation of the photophysical properties for obtaining the desired applications have usually been achieved by incorporating other chromophores into pyrazolines as the fusion partner or changing the substituents on the side-chain via various synthetic methods. 1-Phenyl-3-(4-methylphenyl)-5-phenyl-2-pyrazoline and 1-phenyl-3-(4-methyl-phenyl)-5-(2,4-dichlorophenyl)-2-pyrazoline are two structures that have the same parent nucleus with different substituents on the 5-phenyl ring, which show a significant contrast in the wavelength maxima and intensity.74 The electron-donors ensure efficient charge transfer over electron-acceptors in pyrazoline systems, demonstrated by a comparative study on a series of π-conjugated, rigid, rod pyrazoline derivatives. It is known from the better thermal stability, red-shifted absorption spectra, and lower HOMO and LUMO energy levels that the pyrazoline derivatives with electron contributors exhibit improved photovoltaic properties.75 In addition, the different heterocyclic terminal groups such as thiophene, furan and pyridine, etc., also successfully induce major effects on the optical properties of compounds with the pyrazoline skeleton.76 Notably, a tremendous number of conjugated systems constituting the electron-donating (D) and electron-accepting (A) groups through a π-conjugated linker (D–π–A) have been developed; this is an efficient strategy in tailoring the properties arising from pyrazoline ICT, e.g., (fluorene-2-yl)-(9-ethylcarbazole-3-yl)ketene and 1-phenyl-3-(fluorenone-2-yl)-5-(9-ethylcarbazole-3-yl)-2-pyrazoline.77
Rigid π-fused benzene rings are ideal candidates to illustrate the effect of structural modifications that have been made in the pyrazolinic carbon to bring out efficient emission properties including both color and photophysical parameters. Fused benzene rings with relatively flexible frameworks, such as naphthalene and anthracene, have been chosen to reveal the impact of strong charge withdrawing systems at the C-3 and C-5 position, respectively, for the expansion of conjugation. 3-Naphthyl-1-phenyl-5-(4-carboxyphenyl)-2-pyrazoline (NPCP) was designed in such a way that the phenyl substituted pyrazoline ring (electron donor) acts as a channel between the naphthalene moiety (electron acceptor) and the electron withdrawing carboxylic group as side chains, and was found to have a number of advantageous photophysical properties, compared to over other members of this family. The displayed absorptions and emission wavelengths in different solvents suggest the manifestation of the π–π* transitions with considerable charge-transfer character, which arise from the pyrazoline ring and its flow to the naphthalene unit, thereby enhancing the delocalization of π-electrons throughout the system (Fig. 6).78
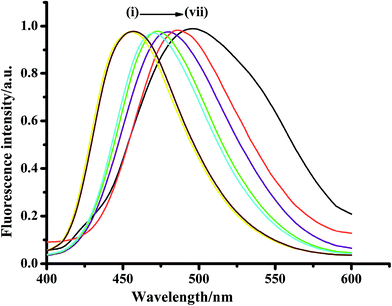 |
| Fig. 6 Fluorescence profile of NPCP in different homogeneous solvents (1 × 10−6 mol L−1); λexc = 370 nm. (i) n-Heptane, (ii) n-cyclohexane, (iii) dioxane, (iv) THF, (v) MeOH, (vi) ACN, (vii) water. Reproduced from ref. 78. Copyright 2015 with permission from Elsevier. | |
While fusing the anthracene, in 5-(9-anthryl)-3-(4-nitrophenyl)-1-phenyl-2-pyrazoline (ANPP), it turned out that there was competition between the photo-induced intramolecular energy transfer from the anthryl to the pyrazoline moiety. There was also charge transfer from N1 to C3 in the pyrazoline moiety in the excited state, which was exemplified by the fluorescence spectra at two different wavelengths of 420 and 365 nm; the existence of spiro-conjugated charge transfer between the fused moieties was also claimed in these systems.79
2.3. Solvatochromism
For many practical applications, pyrazoline derivatives are used as solutions and the influence of solvents on their optical properties are of great significance; therefore, the study of their behavior in various solvents is of profound interest, and has been outlined in many articles. The solvent polarity in addition to some specific interactions, may significantly influence the photophysical behavior of pyrazoline compounds and are thus reflected in the spectral shifts of the solutions.80,81 The solvent dependent radiative transitions and relaxation dynamics of the pyrazoline molecule from S1 and S2 states are most likely considered as the key elements in these phenomena. In the 3-pyrazolyl-2-pyrazoline derivative (PYZ), S0 → S1 and S0 → S2 electronic transitions were interpreted as a perfect pathway for the electronic spectra in the ground state, whereas the radiative emissions and relaxation processes from S1 and S2 states of PYZ establish the modifications of the excited state in solvents like water, ethylene glycol and glycerol. Similarly, radiative transitions from the S1 state would act as a main type of transition in polar aprotic and protic solvents. Based on all the features proposed, there is no doubt that the solute solvent specific interactions are influential for modulating the steady state emission spectra and relaxation dynamics of PYZ in different solvents.82
One of the most important reasons why there is a large spectral shift in the emission spectra of pyrazoline compounds is their increased excited state dipole moments, which are fundamentally different from those in the ground state. An increase in the dipole moment of 2.83 D units was reported for 1,5-diphenyl-3-(N-ethylcarbazole-3-yl)-2-pyrazoline (DEP). This increase was calculated using the Lippert–Mataga equation and features the influence of viscosity and temperature on the fluorescence properties of DEP.83 The solvatochromic models, which include the orientation polarizability (Δf) and the Kamlet–Taft solvent parameters, are comprised of π* (dipolarity/polarizability), α (hydrogen bond donating capacity) and β (hydrogen bond accepting ability), and provide a satisfactory description of the solvatochromic behavior of the pyrazole-nicotinamide drug, nifenazone (NIF). The important factors contributing to the overall effect are the polarity of the medium, hydrogen bonding capability and potential delocalization of electron density within the pyrazole ring, and cause NIF to behave according to the different conditions. The performed Density Functional Theory (DFT) and Time-Dependent DFT (TD-DFT) calculations at the CAM-B3LYP/6-31G+(d) level of theory, coupled with the integral equation formalism polarizable continuum model (IEF-PCM) support the experimental findings.84
2.4. Electroluminescence
Current trends in electroluminescent devices emphasize the direct impact of small organic molecules in accelerating the fabrication of organic electroluminescent (EL) devices, having high luminous efficiency, a wide selection of emission colors via molecular design, low cost and easy processing. In order to realize the functionality of the EL devices, compounds based on pyrazolines should satisfy the specific physicochemical property requirements, and also demonstrate good ability for film formation by the conventional vacuum vapor deposition/vacuum thermo evaporation/wet process and sufficient thermal stability.85–87 Inspired by efficient hole-transport ability, blue emission and high quantum yield, pyrazoline hybrids fused with structurally diverse moieties have been considered as potential candidates for electroluminescent emitters. In PLEDs (polymer light emitting diodes) with varying concentrations of pyrazolate-bridged binuclear platinum(II) complexes as dopants, a stable near infrared (NIR) emission with a peak at 692 nm and a shoulder at 753 nm has been achieved, leading to better device performance with the maximum external quantum efficiency (EQE) of 0.15% and a radiant intensity of 19.10 μW cm−2.88 It is of great significance to find numerous pyrazoline derivatives that are pronouncedly different in structure, being used as optoelectronic materials in organic light-emitting devices.89–91 Additional advantages can sometimes be gained by constructing multichromophore light-emitting compounds, showing good prospects for application in LED applications.
In the case of a pyrazoline–triphenylamine motif, there is a tremendous possibility for the emission of different colored fluorescence. The exhibited emission bands at 524–539 nm and the demonstrated high thermal stabilities with decomposition temperatures (Td) from 400 to 480 °C, revealed the increase in the degree of conjugation and the electron effect. The charge flow from the triphenylamine moiety from the HOMO to LUMO (pyrazoline, benzene and naphthalimide for different compounds) was emphasized by theoretical calculations.92 Great strides have been made towards the synthesis of bis/tris chromophores containing the pyrazoline moiety, proving its suitability in OLEDs as a hole-transporting material with a red shift on increasing solvent polarity and the high value of quantum yields with suitable HOMO ranges (e.g. −5.78 to −5.55 eV).93,94 Due to the increased solubility in common solvents, some poly(phenylene ethynylene) (PPE-type) conjugated co-polymers (C10-PPE-DPP and C10-PPE-TPP), containing diketopyrrolopyrrole (DPP) and triphenylpyrazoline (TPP) units synthesized by the Heck–Sonogashira coupling methodology were expected to be better light emitting diode materials (LEDs). These polymers were found to be capable of forming smooth and uniform films, and the produced yellow/red emission with large Stokes shift is in agreement with their twisted molecular structures, determined via quantum chemical calculations.95
2.5. Chemo-sensing
Chemo-sensors blended with pyrazoline moieties bearing inherent binding atoms for target analytes have been utilized for multi-purpose sensing applications, and are a rapidly expanding field. The sensing capability of pyrazoline derivatives in terms of fluorescence is regulated by a number of mechanisms such as charge transfer (CT) transitions, photo-electron transfer (PET), chelation enhanced fluorescence (CHEF), fluorescence resonance energy transfer (FRET), and excimer formation.96–101 There are precise regulations concerning many chemicals used in the chemical industry and energy field. Transition or heavy metal ions are crucial because they pose severe risks to human health and the environment, and in most cases they tend to accumulate in the food chain or natural water sources and are usually toxic. An admirable sensing ability was found for some pyrazoline-based probes for the detection of hydrazine in the solution and gaseous states in the range of 1.99 ppb, which is lower than the U.S. Environmental Protection Agency standard (10 ppb). Another probe, 2-[4-(3,5-diphenyl-4,5-dihydro-pyrazol-1-yl)-benzylidene]-malononitrile, effectively detects hydrazine at pH 5.0–9.0 with an emission wavelength of 520 nm and Stokes shift of 140 nm; it provides a wide range of applicability including multicolor labeling applications, as it can avoid most of the background influence.102,103
A pyrazoline derivative, 2-(4-chloro-2-(1-(6-chloropyridazin-3-yl)-5-phenyl-4,5-dihydro-1H-pyrazol-3-yl)phenoxy)acetic acid, was reported as an effective “turn on” fluorescent sensor for zinc ions. This sensor formed a 1
:
1 complex with Zn2+ and showed fluorescence enhancement with good tolerance of other metal ions.104 Another probe, 5-(2-(allyloxy)phenyl)-3-(2-hydroxy-5-methylphenyl)-4,5-dihydro-1H-pyrazole-1-carbothioamide, was found to possess the ability to penetrate the membrane easily, and the practicality of the proposed chemo sensor for the determination of intracellular zinc ions was carried out in living neuron cells (Fig. 7).105
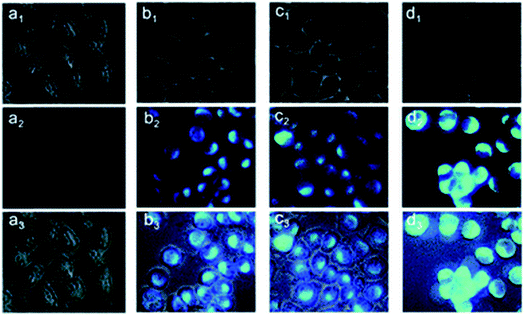 |
| Fig. 7 Fluorescence microscopy images of living PC12 cells. (a1–a3): images of cells incubated with a 10 μM probe for 30 min at 37 °C. Cells were exposed to pyrithione (pyr, concentration equivalent to 1/2 of added Zn2+) in the presence of increased concentrations of extracellular Zn2+ as (b1–b3) 10, (c1–c3) 25 and (d1–d3) 50 μM, following incubation with probe L for 30 min. (a1–d1) Bright-field, (a2–d2) fluorescent, (a3–d3) overlay images. Scale bar = 10 μm. Reproduced from ref. 105 with permission from The Royal Society of Chemistry. | |
Some pyrazoline-based fluorescent sensors provide somewhat higher selectivity and sensitivity for Al3+ and Cu2+ in aqueous solution and DMF
:
H2O = 1
:
1 (v/v), respectively, and also show good selectivity towards the respective ions, over other competitive metal ions; the fluorescence intensity was increasingly quenched (“turn-off” sensor).106,107 1-[(5-Phenyl-1H-pyrazole-3-ylimino)-methyl]-naphthalen-2-ol (HL), selective for Al(III) ions, utilizing “turn-on” fluorescence shifting achieved 32 nM for Al3+ within a very short response time (15–20 s), which is superior to all previously reported chemo sensors (Fig. 8).108 Based on the exploitation of Cu2+ as an ON–OFF–ON signaling motif, a pyrazoline-based probe responded to Cu2+ in aqueous medium in a “turn-off” fluorescent manner and was successfully used for the real-time tracking of Cu2+ in living cells.109 In order to enrich the literature of pyrazoline as sensors, gold(I) complexes based on thiocarbamoyl-pyrazoline ligands and heteroleptic copper(I) complexes based on mixed phosphine/thiocarbamoyl-pyrazoline ligands were synthesized and characterized and photophysical studies were reported.110,111
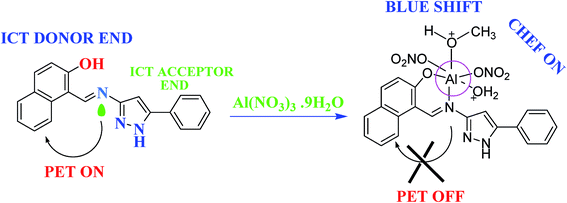 |
| Fig. 8 Probable mechanistic pathway for sensing Al(III) ions.108 | |
In view of the high performance of 3-hydroxyflavones as ratiometric fluorescent probes, the research on 3-hydroxychromone with a bulky 1,3,5-triphenyl-2-pyrazoline moiety exhibited a fairly better response and showed good linear plots of the normal-to-photo tautomer emission intensity ratio with the solvent polarity.112 A newly designed pyrazoline probe, 2-(3-(2-((tert-butyldiphenylsilyl)oxy)phenyl)-5-(4-methoxyphenyl)-4,5-dihydro-1H-pyrazol-1-yl)benzo[d]thiazole, was found to be excellent for practical applications such as fluoride content examination in environmental water samples and in toothpaste, and has relevance due to the increasing importance of fluoride ions in everyday life.113 Tetraaryl pyrazoles containing a polymerizable vinyl functional group were developed and found to be quite attractive for the detection of 2,4,6-trinitrophenol (TNP) or picric acid in the aggregate state. An aggregation induced emission enhancement (AIEE) phenomenon contributes to the fluorescence quenching on adding the aliquots of picric acid in water and shows the detection limit of ∼23 ng, which is comparable to other literature reported AIE/AIEE based polymers.114 It has been recently reported that a thiazol-substituted pyrazoline-based organic fluorescent nanoparticle (OFNs) (N1) could sense picric acid sensitively and selectively via an acid–base interaction induced electron transfer process. It was found that the fluorescence of N1 can be easily quenched by the addition of 2,4,6-trinitrophenol (TNP) in aqueous medium, and a detection limit to TNP of 0.002 ppm was obtained.115
Indeed, it should be noted that pyrazoline chemosensors for environmental monitoring have been far less studied compared to the other applications. Recently, Mohan et al. published a research paper featuring the quinoline appended pyrazoline derivative, 3-(1-benzothiazol-2-yl-5-furan-2yl-4,5-dihydro-1H-pyrazol-3yl)-4-hydroxy-1H-quinoline-2-one (QFP) as a viable chemosensor for fluorescence imaging of Ni2+ ions in live cells. The successful analytical application of QFP in the determination of Ni2+ ions in effluent water from industrial waste provides the additional conclusion that pyrazoline chemosensors have a positive future in the environmental monitoring of real samples.116
2.6. Non-linear optical (NLO) properties
The pyrazoline derivatives have received significant consideration for applications in device fabrication exploiting amplified spontaneous emission/lasing phenomena and two-photon absorption. An experimental investigation on the third-order NLO properties of a series of pyrazoline derivatives has demonstrated the ability to maximize the nonlinear responses by tailored modification of their molecular structure. On comparing the third order nonlinear optical parameters and the thicknesses of all investigated thin polymeric systems, the nonlinearity seemed to be promoted by changing the acceptor from –CN to –NO2, whereas there was no effect with the nitrile group;117 a detailed study was conducted on a series of 3-(4-n-decyloxyphenyl)-1-(p-X-phenyl)-2-pyrazolines with different substituents on the 1-phenyl ring. Following the quantum chemical DFT based calculations, the molecules having highly polar end-groups (PY-oCNNO2 and PirazFPHdiCN) were identified as possessing non-linear optical properties (NLO), and were exploited as dopants in the poly (methyl methacrylate) (PMMA) matrix, thus illustrating the light amplification properties and spectral narrowing of their luminescence spectra.118 With respect to 3-(1,1-dicyanoethenyl)-1-phenyl-4,5-dihydro-1H-pyrazole (DCNP) molecules dispersed in poly(methyl methacrylate) (PMMA) matrices, a significant difference in the luminescence properties was observed for thin films of PMMA containing dyes with changes in the acceptor–donor group, which was demonstrated by stimulated emission and random lasing processes. In such systems, the pumping threshold levels for the observation of random lasing emission was found to vary from about 1.5 mJ cm−2 to nearly 70 μJ cm−2 and for stimulated emission from 16 mJ cm−2 to 5 mJ cm−2 (Fig. 9).119 In a series of 3-(4-n-decyloxyphenyl)-1-(p-X-phenyl)-2-pyrazolines with different substituents on the 1-phenyl ring, which itself is in a parallel orientation, the terminal groups –OCH3, –H, –Cl and –COOH play decisive roles in the compounds being designated as promesogenic materials and having large Stokes shifts and high quantum yields.120
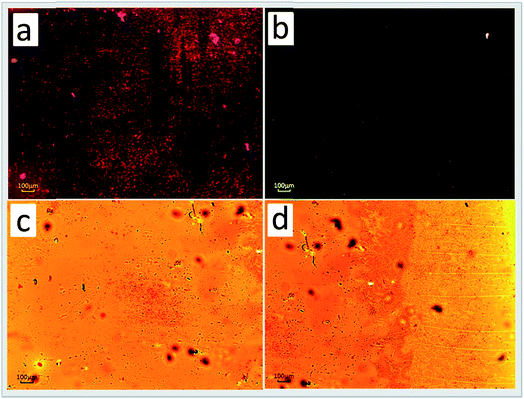 |
| Fig. 9 Optical microscope photographs of (a) DCNP, (b) PY-oCNNO2 and (c), (d) PY-pNO2 compounds. The large (a, c) and small (b) groups of little crystals suspended in the polymeric layer are clearly seen. Layer cracks (d) are caused by the slow evaporation process of the solvent facilitating the nucleation and growth of microcrystals. Reprinted with permission from ref. 119. Copyright 2014 American Chemical Society. | |
2.7. Biological activities
The utilization of the pyrazoline fragment has increased, although it is synthetically more challenging, in view of the broad chemical diversity, stability and a wide-range of biological activities, leading to more potent activity, clinical value and less toxicity for pharmaceutical applications. As such, eighteen novel 1-N-substituted-3,5-diphenyl-2-pyrazoline derivatives, a series of twenty 1-(4-sulfamylphenyl)-3-trifluoromethyl-5-indolyl pyrazolines, some 2-naphthyl pyrazolines and exclusive fluorine substituted pyrazoline derivatives were designed, synthesized and screened for cyclooxygenase (COX-1, COX-2) inhibitory, anti-inflammatory, analgesic and antimicrobial activities; some of them possessed acceptable activity.121–124 In particular, a series of 1,3,5-trisubstituted pyrazolines appears to have antimalarial properties, in addition to the potential for other applications; these compounds target the malaria parasite by inhibiting the haem detoxification process.125
In the treatment of Alzheimer's and Parkinson's diseases, the inhibitory potency shown by a collection of 1-N-substituted thiocarbamoyl-3-phenyl-5-thienyl-2-pyrazolines towards monoamine oxidase B and cholinesterase is promising.126 Most importantly, one of the sub-fields of pyrazoline use is the bio-orthogonal synthesis for in vivo/vitro protein labeling.127–130
Our coverage here is only on selective articles. Although studies on pyrazoline derivatives have been carried out for several years, researchers are still looking for novel pyrazoline compounds possessing biological activities.131–138 Indoxacarb is the first commercialized pyrazoline-type sodium-channel blocker insecticide, testifying to the importance of optimizing pyrazoline-type chemistry with regards to insecticidal efficacy, safety towards non-target organisms and the environment.139,140 There are some groups that have adopted the molecular hybridization method to explore pyrazoline-type insecticides in an attempt to enhance environmental degradation, reduce bioaccumulation, and improve efficacy.141,142
2.8. Bio-imaging/sensing
Research into the development of dyes based on common species with pyrazolines has been conducted and there has been modification in the fluorescence of many common species with significant enhancement, indicating the importance of pyrazoline hybrids in the bio-imaging of living cells.143,144 The quality of the bio-imaging of a pseudo-peptide, oligo(3,4,5-triethoxycarbonyl-2-pyrazoline), with fewer than 70 glycine residues mimicking the green fluorescent protein (GFP) was found to be excellent and exhibits remarkable fluorescence properties, compared to the monomer, 2-pyrazoline.145 The advantages of the high conversion rates (ϕf = 0.52), folded methylene chain (–CH2–CH2–CH2–CH2–) and the presence of the azo group on the rigid pyrazoline ring facilitate the excellent fluorescence efficiency of the oligomer, 1,4-butanediol-bis(5-carbonyl-3-carbethoxy-2-pyrazoline). This contributes to two-way conversion luminescence (TWCL), and boosts the fluorescence intensity in cell imaging.146 The cleavage of the 2,4-dinitrobenzenesulfoyl moiety (DNBS) from the N-(4-(1,5-diphenyl-4,5-dihydro-1H-pyrazol-3-yl)phenyl)-2,4-dinitrobenzene sulfonamide probe was responsible for blocking the photoinduced electron transfer (PET) pathway from the pyrazoline to the DNBS moiety. By virtue of the benefits of switching on the blue emission of the pyrazoline with 488-fold fluorescence enhancement, it showed higher sensitivity and selectivity to glutathione (GSH). Such amplification of the fluorescent signal provides an effective means for imaging intracellular biothiols with strong blue fluorescence, and demonstrates practical application by detecting GSH in calf serum.147
Besides the focus on the development of molecules for imaging, researchers have also made use of the pyrazoline derivatives to design new and efficient drugs to monitor some key molecules of the cell nucleus. One of the best examples, 1-phenyl-3-biphenyl-5-(N-ethylcarbazole-3-yl)-2-pyrazoline (PBEP) has the ability to insert into DNA pairs by the intercalative binding mode. This compound possesses a fluorescence that is quenched dramatically and immediately by the addition of DNA, due to the formation of a stable complex. The advantage of PBEP over other compounds is that it can be appended onto calf thymus DNA (ct DNA) and salmon sperm DNA (ss DNA) with binding constants with the magnitude of 104 L mol−1, and the binding site size is about 1 per base pair.148 On the other hand, because of the influence of various environmental factors associated with physiological processes, the detection by single emission phenomenon is hampered by the challenge of perturbed fluorescence, which questions the precise analyses under biological conditions. To this end, Forster resonance energy transfer (FRET) based biosensors have opened up possibilities, in terms of the eradication of interference from background signals and the fluctuation of detection conditions. A unique pH-driven Forster resonance energy transfer (FRET) based biosensor was obtained after the assembly of an anticancer drug, doxorubicin (DOX), with a donor fluorophore, pyrazoline (PYZ). This PYZ–DOX couple represents a straightforward route to cell imaging, and also controls and delivers anticancer drugs to cancer cells by simply monitoring the pH of the medium.149
2.9. Host–guest assembly
It is widely accepted that the reduced polarity and restricted space provided by the nano cages of supramolecules have the potential to become the molecular container for entrapped pyrazoline derivatives, thereby enhancing the blue light emission efficiency. To do so, the proper fitting of the guest to the host cavity is required. Moreover, there are significant advantages to the use of pyrazolines in biomedical applications as they offer better solubility, stability and bioavailability. The inclusion of (1′-(4-bromophenyl)-3a′,4′,5′,6′,6a′-hexahydrocyclopentapyrazoline)-3-methyl-1-phenyl-1H-pyrazole-4-carbonitrile (PZ) in β and γ-CDs was verified both experimentally and theoretically. The small cavity size causes PZ to orient itself between the hydrophobic bucket-like cavities of two β-CDs to form the 1
:
2 complex, whereas the loose fit leads to 1
:
1 complexation with γ-CD.150 Specifically, the pyrazoline derivative [5-(-1-(4-bromo-phenyl)-3a′,7a′-hexahydro-1H-indazol-3-yl)-3-methyl-1-phenyl-1H-pyrazole-4-carbonitrile] (PZ) in trans form displays an emission band at 396 nm, and disrupts the embedding of a second β-CD in order to avoid steric congestion and consequently features 1
:
1 complexation. The fluorescence spectra of the cis-isomer was centered at 475 nm; it also partially engaged two β-CD, leading to 1
:
2 complexation.151
An emission intensity increase along with a blue shift confirmed the penetration of a labeled drug, 3-naphthyl-1-phenyl-5-(5-fluoro-2-nitrophenyl)-2-pyrazoline (NPFP)–phenylephrine, into the β-CD cavity (Fig. 10). The type of complexation in lower and higher concentrations of CDs was also defined through the analysis of data obtained from the Benesi–Hildebrand double reciprocal linear plot and steady-state anisotropy. The extent of protection and depth of inclusion from the surrounding water generates the 1
:
1/1
:
2 complexes in different host concentrations. From a theoretical point of view, the stability of the (NPFP)–phenylephrine-CDs was excellent as interpreted from the calculated thermodynamic parameters obtained from molecular modelling studies.152 In a parallel effort, the general concept of the nearness of pyrazolinic protons to H-3 and H-5 protons of β/γ CDs has been visualized by 1H NMR and 2D NMR spectroscopy, which outlined a detailed knowledge of the dynamics and host–guest interactions of the earlier mentioned dye NPCP, and CDs of varying cavity dimensions (α-, β- and γ-CDs).153
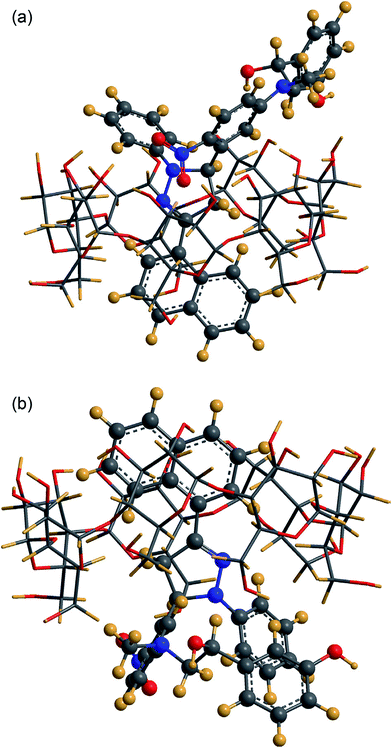 |
| Fig. 10 Geometry of the most favorable inclusion complex of NPFP–phenylephrine β-CD. Reproduced from ref. 152 with permission from Elsevier. | |
2.10. Organic micro/nano particles
Nowadays, researchers have taken the opportunity to construct nanostructures with purposely designed molecules, and broaden their scope to new avenues that were not previously accessible. Since organic photonic materials were meant to provide multiple useful applications, this propelled the preparation of pyrazoline micro/nanoparticles, which have captured more and more interest.154 The generality of the size-tunable emission concept of organic nanoparticles has been further illustrated by organic nanoparticles of 1,3-diphenyl-5-(2-anthryl)-2-pyrazoline (DAP) and 1,3-diphenyl-5-pyrenyl-2-pyrazoline (DPP). DAP has average diameters ranging from 40 to 160 nm, and was found to display size-dependent bathochromic absorption transition and the split band of the higher-energy band of anthracene. The blue shifted emission of the pyrazoline chromophore with an increase in the particle size occurs due to the restraint of the vibronic relaxation and the configuration reorganization process of the electronically excited state. Attention has also been directed to the formed exciplex between pyrazoline and anthracene moieties; in particular, their intensities increase with the growth of the nanoparticles.155 In DPP nanoparticles, the size-dependent effects also exist; as a result of the surface effects, they exhibit multiple emission properties ranging from near-UV to green, which can be tuned by alteration of both the excitation wavelength and nanoparticle size.156
In organic thin films of stearic acid/pyrazoline nanoparticles, the Langmuir monolayer of stearic acid/pyrazoline nanoparticle was specifically chosen to deposit onto the solid substrate, and its dispersion in the Langmuir–Blodgett (LB) film was investigated by TEM, AFM and top view imaging. The extremely thin LB films offer a linear increase in the optical absorbance at around 370 nm on increasing the multilayers for a given pressure-area isotherm at the air/water interface.157 The rod-like cetyltrimethylammonium bromide (CTAB) micelles lie at the heart of many studies in nano and micrometer-scale materials and are widely used as templates in the synthesis of inorganic nano rods or nano wires, due to the simplicity and low cost. Concomitant with this effort, Hongbing Fu and co-workers showed that 1,3-diphenyl-2-pyrazoline (DP) nano fibers could be produced in the presence of CTAB in the aqueous phase by the self-inducing template-growth approach. The rapid dissolution of DP particles into the hydrocarbon core of spherical CTAB micelles causes the transition to rod-like shape, which acts as a template for the growth of cylindrical DP nanofibers. It is reported that the self-assembly process of DP fibers as J-type aggregates involves the dominant crystal-packing forces.158
In the fabrication of highly monodisperse pyrazoline nanoparticles, stearic acid has been considered as a surfactant to assist as a charge controlling additive to control the electrophoric mobility of the particles in the encapsulation of pyrazoline nanoparticles to methyl methacrylate (MMA) and ethylene glycol dimethacrylate (EGDMA) (dispersion–polymerization) in a methanol–water mixture. The 400 nm electrophoretic particles were prepared with 1.5 wt% of stearic acid surfactant to deliver an electrophoric mobility of −7.513 × 10−5 cm2 V−1 s−1.159
The chemical oxidative polymerization of aniline using 1-(N-butyl-1,8-naphthalimide-4′-yl)-3-(4-methoxyl-phenyl)-5-phenyl-pyrazoline nanofibres (BMPP) as templates was found to be highly favorable to produce BMPP/polyaniline core–shell nanofibers with net-like structures, possessing better electrochemical properties than BMPP nanofibers.160 It has been proposed that the pristine nano rods of 1,5-diphenyl-3-(naphthalene-4-yl)-1H-pyrazoline (DPNP) can accommodate 2,5-bis(2-(N-hexadecylpyridinium-4-yl)-vinyl)pyrrole iodide (dye) through an adsorption mechanism; a switching of ξ-potential from negative to positive, constituting the dye/DPNP core/diffuse-shell nano rods also rendered fluorescence resonance energy transfer (FRET) from the DPNP core to the dye shell (Fig. 11).161 Such systems open up venues for multifunctional nano-materials with potential applications in optoelectronics.
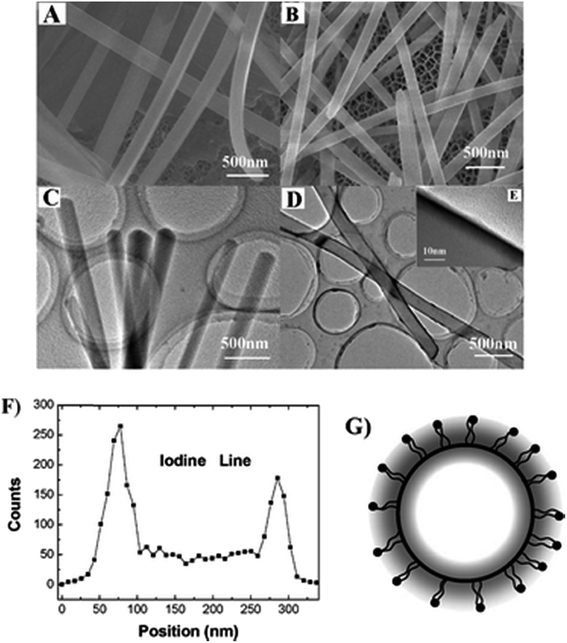 |
| Fig. 11 (A) SEM and (C) TEM images of the pristine nanorods of DPNP; (B) SEM and (D) low-magnification TEM and (E) high magnification TEM images of DPNP/dye 1 core/diffuse-shell nanorods; (F) HRTEM-assisted EDS line of elemental iodine analysis across the section of a single core/diffuse-shell nanorod. (G) Schematic illustration of the section of the core/diffuse-shell nanorod. Scale bars: (A–D) 500 nm; (E) 10 nm. Reproduced from ref. 161 with the permission from The Royal Society of Chemistry. | |
2.11. More applications
A mixture of co-aggregates of 1,3,5-triphenyl-2-pyrazoline (TPP) and 1,4-dicyanonaphthalene (DCN), while considering the quenching of TPP fluorescence by DCN accompanied by a broad and structureless emission at about 560 nm, revealed the formation of an exciplex rather than an intermolecular charge-transfer complex (ICT) in the excited state. The obvious effect of changing the DCN content can be observed from the emission color of the mixed co-aggregates. The theoretical calculations are consistent with the experimental observations, which support the occurrence of photo-induced electron transfer (PET) through an electronic transition from the lowest unoccupied molecular orbital (LUMO) of the excited TPP to that of the ground-state of DCN (Fig. 12).162 The information gained in this work was later well supported through detailed theoretical studies by Yulan Dai and co-workers.163
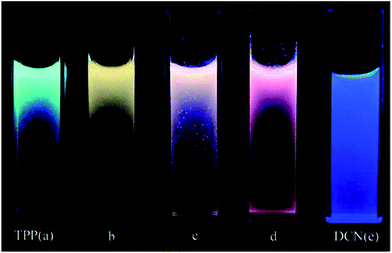 |
| Fig. 12 Photographs of the suspensions of mixed co-aggregates with different TPP/DCN molar ratios taken under a UV lamp (355 nm): (a) pure TPP aggregates; (b) (TPP/DCN) 4 : 1; (c) (TPP/DCN) 2 : 1; (d) (TPP/DCN) 1 : 1; and (e) pure DCN aggregates. Reprinted with permission from ref. 162. Copyright 2008 American Chemical Society. | |
Interesting work was carried out to demonstrate the possibility of using pyrazole derivatives as efficient acceptors for the organic solar cells, with the application of polythiophene as the donor, and luminophores from the azaheterocyclic groups as acceptors, and bulk hetero structures were fabricated. It was then more precisely evidenced experimentally and theoretically that the maximum attainable short current density Isc = 39.14 μA, fill factor FF = 37.42% and energy conversion efficiency QE = 1.19% were obtained for solar cell structures having active layers with absorption coefficients kc = 0.29.164 Furthermore, a detailed experimental and theoretical study was conducted on photophysical and charge transfer properties of some pyrazoline derivatives, synthesized by a simple and cost-effective synthetic procedure. As a prominent advantage, these compounds were found to exhibit UV-visible absorption peaks below 400 nm, avoiding the problem of interference from the donor absorption that usually falls in the visible to NIR region. Moreover, these compounds were widely investigated as hole transport materials (HTMs), and it was found that the HOMO energy levels between −4.8 and −5.2 eV, and the charge carrier mobility of the dimethoxy substituted phenyl moiety in the order of 4 × 10−5 cm2 V−1 s−1 underline its suitability as an efficient HTM.165
One of the biggest challenges in the development of organic electrical memory materials involves the lack of thermal stability and the inconvenience of device fabrication. An organic small molecule 4-(1,3-diphenyl-4,5-dihydro-1H-pyrazol-5-yl)-N,N-dimethylaniline (ATPP) bearing a pyrazoline moiety is shown to have high promise as an electrical memory material. In this stunning application, an electro-active film of ATPP was fabricated by thermal evaporation and then prepared as a sandwich memory device, indium tin oxide/ATPP/Al (ITO/ATPP/Al). The performance of the device was particularly demanding, due to the switch-off phenomenon at the compliance current of 0.1 A, also stable static random-access memory (SRAM) behavior and excellent stability in test conditions because of the introduction of (polystyrene) PS.166
A simple, rapid and efficient micro extraction method for the extraction and determination of some 2-pyrazoline derivative compounds in aqueous samples was developed. When considering the current trends in analytical procedures, the optimal conditions observed such as sampling temperature (70 °C), stirring rate (1250 rpm), pH (5.0), nature and volume of the organic solvent (8.0 μL, 1-undecanol), the extraction time (30 min) and the achieved detection limit (5–10 μg L−1) are big pluses in the further analytical applications of pyrazoline derivatives in a greener way. Additionally, the established method provides an effective avenue for the determination of compounds in real life samples including serum and urine.167 Another key step towards novel applications of pyrazoline dyes involves the medium sensitivity and its suitability for bio-analytical applications, but development of this area is still in its early stages. Recently, we have been able to develop an analytical method using 3-naphthyl-1-phenyl-5-(4-carboxyphenyl)-2-pyrazoline (NPCP) as the pre-column derivatization agent for labelling the NH2 group. The feasibility of rapid separations of model analytes allows relatively high selectivity and sensitivity towards amino groups under milder reaction conditions such as moderate derivatization temperature, less derivatization time and no multiple derivatives or by-products. The detection responses of the NPCP-amides were attainable in the range of 35 μg L−1, highlighting its competency with the main stream fluorescent labelling agents for tagging amino groups.168 The researchers are of the hope that graphene, one of the “materials on demand”, would revolutionize the electronics and transform our day-to-day lives. Among the various covalently modified graphene oxides, one involving an amide unit as a bridge between graphene oxide (GO) and a pyrazoline dye, 3-naphthyl-1-phenyl-5(4-aminophenyl)-2-pyrazoline (NPAP), displayed excellent photophysical properties due to the charge flow between the two fragments. This type of GO modification involving pyrazoline compounds will surely pave the way for a range of applications involving graphene based structures with tunable and enhanced optoelectronics.169
The repetitive yet diverse nature of research on the pyrazoline moiety and the corresponding compounds is apparent. To date, a fair number of articles have been published and specific articles describing particular applications are cited. This review makes no attempt to be exhaustive, but highlights some representative reports for citing the multifaceted applications of pyrazoline derivatives.
3. Conclusions and future view
It is amazing what a small molecule scaffold like pyrazoline, adapted in heterocyclic chemistry, has achieved. The abundance of research aimed at finding ways to utilize these compounds to replace some heterocyclic rings that are not as efficient is justified by many recent publications describing their unique properties, together with the simplicity of their synthesis and modification. Although these efforts have been very successful, there is still much to be done. Some efforts have been made to develop safe, non-toxic and environmentally friendly synthetic procedures, with the least possible consumption of materials and energy and less generation of waste, but the use of special reagents or solvents limit the viability. Therefore, green approaches should be oriented in such a way that reagents are made commercially available and are cost effective. After an overview of the photophysics of many reported pyrazoline compounds, it was noticed that there is a general trend of designing molecules with simple substitutions at the C-3 and N-1 positions of the pyrazoline ring with commonly reported systems. There are still lots of unreported bulky systems with versatile optical properties or electron withdrawing/donating ability, and there is lots of room for improvement in the extension of charge transfer (CT) systems. In our lab, some fruitful attempts have been developed to incorporate a diverse set of substitutions on the phenyl ring at C-3, and we are also planning to utilize different polycyclic aromatic hydrocarbons at the N-1 position, thereby making the system more rigid and fluorescent.
In addition, theoretical calculations have proved to be an effective way to design target molecules and to explain the electron processes in this area, and we hope more and more theoretical chemists will participate in this promising direction. Regarding the pyrazoline based probes and the realization of practical applications in fluorescence bio-imaging technology, it is essential to produce dyes having high quantum yield, enhanced photo stability and biocompatibility; however, we think more work is required for further improvement in this area. Significant progress in the discovery of novel chemical classes of pyrazoline drugs against some target microorganisms has been made in medicinal chemistry. Along these lines, the launch of many pyrazoline compounds having immense clinical value is also expected, but researchers should also focus on clinical trials, which would offer important insight to address key biological problems. Challenges still remain regarding their poor aqueous solubility, which has hindered their potential use in drug development. In this regard, the enhancement of the photophysics of some pyrazoline derivatives, accomplished by host–guest interactions with macrocyclic hosts, highlights an effective strategy to increase the solubility, stability and bioavailability of medicaments. In contrast to organic solvents, the use of various supramolecular systems or micro heterogeneous media will continue to be an active area of research to ensure the biological safety during the use of pyrazoline compounds in bio-related fields. Taking into account simple chiral separations of bio-analytes using macrocyclic systems, the investigations of interactions of some dye molecules with cyclodextrins has attracted wide attention from bio-analytical chemists, which helps them to design separation protocols based on supramolecular chemistry.
Ongoing research shows that pyrazoline molecules are very promising for the fabrication of nano-electronic and bio-electronic devices in the near future. The past few years have witnessed an increase in research interest in graphene (along with graphene derivatives), and functionalization is the best way to achieve the best performance from graphene oxide (GO). In recent years, huge progress has been made in terms of modulating the photophysical properties of graphene oxide through surface functionalization using fluorescent dyes. However, the perplexing question still remains as to why pyrazoline dyes were not widely used for the covalent modification of graphene oxide sheets in literature, even though they stand out among the five membered heterocycles due to their simple structure, blue light emitting efficiency, large extinction coefficient, high quantum yields and excellent medium sensitivity. A challenging issue is also to explore these types of organic molecules to meet the needs of the industry and the society at large. For example, new designs of the pyrazoline core skeleton, which are well known for efficient photon absorption and charge collection may be beneficial for the development of dye-sensitized solar cells (DSSC). It was reported in many articles that one of the nitrogen atoms in the heterocyclic ring of pyrazolines forms an electron-donating π-conjugated system, so it is able to act as a hole-transporting material (HTM) when used in electro luminescent devices (ELD) and this feature is expected to bring a revolution in material chemistry. In light of the high sensitivity and selectivity for detecting metal ions, the sensory systems developed on the pyrazoline backbone have proved to be among the most useful chemical platforms for the design of chemical sensors and will have wider applicable scope in addressing the issues in terms of human health and the environment. In the past few years, huge progress was registered in terms of converting organic dye molecules to nanoparticles. These nanoparticles sit in the spotlight because they promise to be a fascinating class of materials and are well suited to many applications like bio-analysis, photo-catalysis, pharmacology, photonics, and microelectronics. Following the paths as with other organic dyes, many academic research groups are actively working on pyrazoline dyes seeking to improve the physico-chemical properties with the desired morphology and they have shown that the π-conjugated organic micro- or nanostructures hold promise.
The availability of a wide variety of fluorescent probes and the development of sensitive analytical techniques have made fluorescence labelling an essential facet of chemical biology and biomedical science. Despite many applications of pyrazoline derivatives in various fields, there are still just a few reported applications in bio-analytical research. The probes on the pyrazoline backbone will play the principal role in the future, as proved by recent applications of such types of molecules for tagging the –NH2 group. Once again, significant advances in analytical sensing are needed to realize the potential of pyrazoline derivatives. A quick overview of all the domains where pyrazoline derivatives find their applications support the fact that these five-membered nitrogen containing heterocyclic rings have been employed to design various compounds according to individual demands for specific applications. We are optimistic that pyrazoline based compounds will likely lead to offshoot technologies and niche applications.
Conflicts of interest
There are no conflicts to declare.
Acknowledgements
We would like to acknowledge Sultan Qaboos University (SQU) for all financial support.
References
- A. Wagner, C. W. Schellhammer and S. Peterson, Angew. Chem., Int. Ed., 1966, 5, 699 CrossRef CAS.
- S. Hua, S. Zhang, Y. Hua, Q. Tao and A. Wu, Dyes Pigm., 2013, 96, 509 CrossRef.
- C. K. Kumar, R. Trivedi, L. Giribabu, S. Niveditha, K. Bhanuprakash and B. Sridhar, J. Organomet. Chem., 2015, 780, 20 CrossRef.
- T. T. Zhang, F. W. Wang, M. M. Li, J. T. Liu, J. Y. Miao and B. X. Zhao, Sens. Actuators, B, 2013, 186, 755 CrossRef CAS.
- H. B. Shi, S. J. Ji and B. Bian, Dyes Pigm., 2007, 73, 394 CrossRef CAS.
- P. Wang, N. Onozawa-Komatsuzaki, Y. Himeda, H. Sugihara, H. Arakawa and K. Kasuga, Tetrahedron Lett., 2001, 42, 9199 CrossRef CAS.
- V. Cherpak, P. Stakhira, S. Khomyak, D. Volynyuk, Z. Hotra, L. Voznyak, G. Dovbeshko, O. Fesenko, V. Sorokin, A. Rybalochka and O. Oliynyk, Opt. Mater., 2011, 33, 1727 CrossRef CAS.
- K. Cyprych, L. Sznitko, O. Morawski, A. Miniewicz, I. Rau and J. Mysliwiec, J. Phys. D: Appl. Phys., 2015, 48, 195101 CrossRef.
- T. Vandana, V. Ramkumar and P. Kannan, Opt. Mater., 2016, 58, 514 CrossRef CAS.
- E. Arbaciauskiene, K. Kazlauskas, A. Miasojedovas, S. Jursenas, V. Jankauskas, W. Holzerd, V. Getautisa and A. Sackus, Synth. Met., 2010, 160, 490 CrossRef CAS.
- M. Jin, Y. J. Liang, R. Lu, X. H. Chuai, Z. H. Yi, Y. Y. Zhao and H. J. Zhang, Synth. Met., 2004, 140, 37 CrossRef CAS.
- J. V. Mehta, S. B. Gajera, P. Thakor, V. R. Thakkar and M. N. Patel, RSC Adv., 2015, 5, 85350 RSC.
- A. Burmudzij, Z. Ratkovi, J. Muskinja, N. Jankovic, B. Rankovic, M. Kosanic and S. Đorđevi, RSC Adv., 2016, 6, 91420 RSC.
- V. K. Mishra, M. Mishra, V. Kashaw and S. K. Kashaw, Bioorg. Med. Chem., 2017, 25, 1949 CrossRef CAS PubMed.
- J. Ramírez-Prada, S. M. Robledo, I. D. Velez, M. P. Crespo, J. Quiroga, R. Abonia, A. Montoya, L. Svetaz, S. Zacchino and B. Insuasty, Eur. J. Med. Chem., 2017, 131, 237 CrossRef PubMed.
- D. Havrylyuk, B. Zimenkovsky, O. Vasylenko, C. W. Day, D. F. Smee, P. Grellier and R. Lesyk, Eur. J. Med. Chem., 2013, 66, 228 CrossRef CAS PubMed.
- S. C. Karad, V. B. Purohit, P. Thakor, V. R. Thakkar and D. K. Raval, Eur. J. Med. Chem., 2016, 112, 270 CrossRef CAS PubMed.
- D. N. Rana, M. T. Chhabria, N. K. Shah and P. S. Brahmkshatriya, Med. Chem. Res., 2014, 23, 2218 CrossRef CAS.
- G. Mótyán, F. Kovács, J. Wölfling, A. Gyovai, I. Zupkó and E. Frank, Steroids, 2016, 112, 36 CrossRef PubMed.
- M. A. Abdel-Sayed, S. M. Bayomi, M. A. El-Sherbeny, N. I. Abdel-Aziz, K. E. H. ElTahir, G. S. G. Shehatou and A. A. M. Abdel-Aziz, Bioorg. Med. Chem., 2016, 24, 2032 CrossRef CAS PubMed.
- M. S. Muneera and J. Joseph, J. Photochem. Photobiol., B, 2016, 163, 57 CrossRef CAS PubMed.
- M. Amir, H. Kumar and S. A. Khan, Bioorg. Med. Chem. Lett., 2008, 18, 918 CrossRef CAS PubMed.
- Y. Lee, B. S. Kim, S. Ahn, D. Koh, Y. H. Lee, S. Y. Shin and Y. Lim, Bioorg. Chem., 2016, 68, 166 CrossRef CAS PubMed.
- S. Y. Shin, S. Ahn, H. Yoon, H. Jung, Y. Jung, D. Koh, Y. H. Lee and Y. Lim, Bioorg. Med. Chem. Lett., 2016, 26, 4301 CrossRef CAS PubMed.
- R. S. Joshi, P. G. Mandhane, S. D. Diwakar, S. K. Dabhade and C. H. Gill, Bioorg. Med. Chem. Lett., 2010, 20, 3721 CrossRef CAS PubMed.
- P.-L. Zhao, F. Wang, M.-Z. Zhang, Z.-M. Liu, W. Huang and G.-F. Yang, J. Agric. Food Chem., 2008, 56, 10767 CrossRef CAS PubMed.
- L. Knorr, Ber. Dtsch. Chem. Ges., 1883, 16, 2597 CrossRef.
- R. A. Mekheimer, E. A. Ahmed and K. U. Sadek, Tetrahedron, 2012, 68, 1637 CrossRef CAS.
- J. Shun-Jun and S. Hai-Bin, Dyes Pigm., 2006, 70, 246 CrossRef.
- N. Ertan, Dyes Pigm., 2000, 44, 41 CrossRef.
- W.-Y. Liu, Y.-S. Xie, B.-X. Zhao, B.-S. Wang, H.-S. Lv, Z.-L. Gong, S. Lian and L.-W. Zheng, J. Photochem. Photobiol., A, 2010, 214, 135 CrossRef CAS.
- X. Q. Cao, X. H. Lin, Y. Zhu, Y. Q. Ge and J. W. Wang, Spectrochim. Acta, Part A, 2012, 98, 76 CrossRef CAS PubMed.
- B. Dong, M. Wang and C. Xu, Luminescence, 2013, 28, 628 CrossRef CAS PubMed.
- F. Liu, J.-F. Yang, H. Liu, W.-Z. Wei and Y.-M. Ma, J. Chin. Chem. Soc., 2016, 63, 254 CrossRef CAS.
- K. Manna and Y. K. Agrawal, Bioorg. Med. Chem. Lett., 2009, 19, 2688 CrossRef CAS PubMed.
- Z. N. Siddiqui, T. N. M. Musthafa, A. Ahmad and A. U. Khan, Bioorg. Med. Chem. Lett., 2011, 21, 2860 CrossRef CAS PubMed.
- M. A. Zolfigol, D. Azarifar, S. Mallakpour, I. Mohammadpoor-Baltork, A. Forghaniha, B. Malekia and M. Abdollahi-Alibeik, Tetrahedron Lett., 2006, 47, 833 CrossRef CAS.
- M. A. Zolfigol, D. Azarifar and B. Maleki, Tetrahedron Lett., 2004, 45, 2181 CrossRef CAS.
- S. Mallouk, K. Bougrin, H. Doua, R. Benhida and M. Soufiaoui, Tetrahedron Lett., 2004, 45, 4143 CrossRef CAS.
- G. J. Pant, P. Singh, B. S. Rawat, M. S. M. Rawat and G. C. Joshi, Spectrochim. Acta, Part A, 2011, 78, 1075 CrossRef PubMed.
- T. Mori, H. Saomoto, K. Machitani, K. Inoue, Y. Aoki, T. Koshitani, N. Koumura and T. N. Murakami, RSC Adv., 2016, 6, 13964 RSC.
- P. Singh, J. S. Negi, K. Singh, G. J. Pant, M. S. M. Rawat and G. C. Joshi, Synth. Met., 2012, 162, 1977 CrossRef CAS.
- A. Szukalski, B. Sahraoui, B. Kulyk, C. A. Lazar, A. M. Manea and J. Mysliwiec, RSC Adv., 2017, 7, 9941 RSC.
- Z. S. Sahin, U. Salgın-Goksen, N. Gokhan-Kelekci and S. Isık, J. Mol. Struct., 2011, 1006, 147 CrossRef CAS.
- F. Jian, P. Zhao, H. Guo and Y. Li, Spectrochim. Acta, Part A, 2008, 69, 647 CrossRef PubMed.
- H. Zhang, X. Hua, W. Dou and W. Liu, J. Fluorine Chem., 2010, 131, 883 CrossRef CAS.
- F. Anam, A. Abbas, K. M. Lo, Z. Rehman, S. Hameed and M. M. Naseer, New J. Chem., 2014, 38, 5617 RSC.
- B. Kupcewicz and M. Małecka, Cryst. Growth Des., 2015, 15, 3893 CAS.
- Q. Feng, M. Wang, B. Dong, C. Xu, J. Zhao and H. Zhang, CrystEngComm, 2013, 15, 3623 RSC.
- B. V. Ioffe and D. D. Tsitovich, Chem. Heterocycl. Compd., 1966, 1, 375 CrossRef.
- A. Szukalski, A. Miniewicz, K. Haupa, B. Przybyl, J. Janczak, A. L. Sobolewski and J. Mysliwiec, J. Phys. Chem. C, 2016, 120, 14813 CAS.
- R. Hasan, K. Nishimura, M. Okada, M. Akamatsu, M. Inoue and T. Ueno, Pestic. Sci., 1996, 46, 105 CrossRef CAS.
- F. B. Miguel, J. A. Dantas, S. Amorim, G. F. S. Andrade, L. A. S. Costa and M. R. C. Couri, Spectrochim. Acta, Part A, 2016, 152, 318 CrossRef CAS PubMed.
- L.-L. Tong, Y. He, L.-N. Niu, L.-L. Bao, Z.-C. Wei, Y. Liao and C.-B. Guo, Synth. Met., 2015, 210, 133 CrossRef CAS.
- A. F. Diaz and K. Kanazawa, J. Electroanal. Chem. Interfacial Electrochem., 1978, 86, 441 CrossRef CAS.
- F. Langa and F. Oswald, C. R. Chim., 2006, 9, 1058 CrossRef CAS.
- N. Ziani, K. Lamara, A. Sid, Q. Willem, B. Dassonneville and A. Demonceau, Eur. J. Chem., 2013, 4, 176 CrossRef CAS.
- W. Yang, Y. Hua, Y.-S. Yang, F. Zhang, Y.-B. Zhang, X.-L. Wang, J.-F. Tang, W.-Q. Zhong and H.-L. Zhu, Bioorg. Med. Chem., 2013, 21, 1050 CrossRef CAS PubMed.
- K. R. Ethiraj, P. Nithya, V. Krishnakumar, A. Jesil Mathew and F. Nawaz Khan, Res. Chem. Intermed., 2013, 39, 1833 CrossRef CAS.
- U. Salgın-Goksen, S. Yabanoglu-Ciftci, A. Ercan, K. Yelekci, G. Ucar and N. Gokhan-Kelekci, J. Neural Transm., 2013, 120, 863 CrossRef PubMed.
- S. Sharma, S. Kaur, T. Bansal and J. Gaba, Chem. Sci. Trans., 2014, 3, 861 Search PubMed.
- J.-T. Li, X.-H. Zhang and Z.-P. Lin, Beilstein J. Org. Chem., 2007, 3, 13 CrossRef PubMed.
- A. Lévai and J. Jekőb, ARKIVOC, 2007, i, 134 Search PubMed.
- C. Blackburn, M. O. Duffey, A. E. Gould, B. Kulkarni, J. X. Liu, S. Menon, M. Nagayoshi, T. J. Vos and J. Williams, Bioorg. Med. Chem. Lett., 2010, 20, 4795 CrossRef CAS PubMed.
- B. S. Dawane, S. G. Konda, G. G. Mandawad and B. M. Shaikh, Eur. J. Med. Chem., 2010, 45, 387 CrossRef CAS PubMed.
- G.-W. Wang, Y.-J. Li, R.-F. Peng, Z.-H. Liang and Y.-C. Liu, Tetrahedron, 2004, 60, 3921 CrossRef CAS.
- S. S. Rahmatzadeh, B. Karami and S. Khodabakhshi, J. Chin. Chem. Soc., 2015, 62, 17 CrossRef CAS.
- Z. Daneshfar and A. Rostami, RSC Adv., 2015, 5, 104695 RSC.
- V. Marković and M. D. Joksović, Green Chem., 2015, 17, 842 RSC.
- S. N. Shelke, G. R. Mhaske, V. D. B. Bonifácio and M. B. Gawande, Bioorg. Med. Chem. Lett., 2012, 22, 5727 CrossRef CAS PubMed.
- S. B. Zangade, S. S. Mokle, A. T. Shinde and Y. B. Vibhute, Green Chem. Lett. Rev., 2013, 6, 123 CrossRef CAS.
- A. Adhikari, B. Kalluraya, K. V. Sujith, K. Gouthamchandra, R. Jairam, R. Mahmood and R. Sankolli, Eur. J. Med. Chem., 2012, 55, 467 CrossRef CAS PubMed.
- G. Bai, J. Li, D. Li, C. Dong, X. Han and P. Lin, Dyes Pigm., 2007, 75, 93 CrossRef CAS.
- P. Zhao, R. Li, H. Wang, F. Jian and H. Guo, Spectrochim. Acta, Part A, 2009, 74, 87 CrossRef PubMed.
- G. B. Yang, Y. Wu, W. J. Tian, X. Zhou and A. M. Ren, Curr. Appl. Phys., 2005, 5, 327 CrossRef.
- V. Ramkumar and P. Kannan, Opt. Mater., 2015, 46, 605 CrossRef CAS.
- S. Song, D. Ju, J. Li, D. Li, Y. Wei, C. Dong, P. Lin and S. Shuang, Talanta, 2009, 77, 1707 CrossRef CAS PubMed.
- B. Varghese, S. N. Al-Busafi, F. O. Suliman and S. M. Z. Al-Kindy, J. Lumin., 2015, 159, 9 CrossRef CAS.
- M. Wang, J. Zhang, J. Liu, C. Xu and H. Ju, J. Lumin., 2002, 99, 79 CrossRef CAS.
- A. Sarkar, T. K. Mandal, D. K. Rana, S. Dhar, S. Chall and S. C. Bhattacharya, J. Lumin., 2010, 130, 2271 CrossRef CAS.
- S. Pramanik, P. Banerjee, A. Sarkar, A. Mukherjee, K. K. Mahalanabis and S. C. Bhattacharya, Spectrochim. Acta, Part A, 2008, 71, 1327 CrossRef PubMed.
- S. Chatterjee, P. Banerjee, S. Pramanik, A. Mukherjee, K. K. Mahalanabis and S. C. Bhattacharya, Chem. Phys. Lett., 2007, 440, 313 CrossRef CAS.
- J. F. Li, B. Guan, D. X. Li and C. Dong, Spectrochim. Acta, Part A, 2007, 68, 404 CrossRef CAS PubMed.
- A. D. Bani-Yaseen and M. Al-Balawi, Phys. Chem. Chem. Phys., 2014, 16, 15519 RSC.
- P. Stakhira, S. Khomyak, V. Cherpak, D. Volyniuk, J. Simokaitiene, A. Tomkeviciene, N. A. Kukhta, J. V. Grazulevicius, A. V. Kukhta, X. W. Sun, H. V. Demir, Z. Hotra and L. Voznyak, Synth. Met., 2012, 162, 352 CrossRef CAS.
- Q. Peng, J. Zou, G. Zeng, Z. Wen and W. Zheng, Synth. Met., 2009, 159, 1944 CrossRef CAS.
- Z. Lu, Q. Jiang, W. Zhu, M. Xie, Y. Hou, X. Chen, Z. Wang, D. Zou and T. Tsutsui, Synth. Met., 2000, 111, 425 CrossRef.
- N. Su, F. Meng, J. Chen, Y. Wang, H. Tan, S. Su and W. Zhu, Dyes Pigm., 2016, 128, 68 CrossRef CAS.
- V. Ramkumar and P. Kannan, Opt. Mater., 2015, 46, 314 CrossRef CAS.
- H. Shi, J. Dai, X. Zhang, L. Xu, L. Wang, L. Shi and L. Fang, Spectrochim. Acta, Part A, 2011, 83, 242 CrossRef CAS PubMed.
- X. H. Zhang, S. K. Wu, Z. Q. Gao, C. S. Lee, S. T. Lee and H.-L. Kwong, Thin Solid Films, 2000, 371, 40 CrossRef CAS.
- G. Chen, H.-Y. Wang, Y. Liu, X.-P. Xu and S.-J. Ji, Dyes Pigm., 2010, 85, 194 CrossRef CAS.
- B. Bian, S.-J. Ji and H.-B. Shi, Dyes Pigm., 2008, 76, 348 CrossRef CAS.
- H.-Y. Wang, J.-J. Shi, G. Chen, X.-P. Xu and S.-J. Ji, Synth. Met., 2012, 162, 241 CrossRef CAS.
- G. Zhang, K. Liu, Y. Lia and M. Yang, Polym. Int., 2009, 58, 665 CrossRef CAS.
- R.-R. Zhang, J.-F. Zhang, S.-Q. Wang, Y.-L. Cheng, J.-Y. Miao and B.-X. Zhao, Spectrochim. Acta, Part A, 2015, 137, 450 CrossRef CAS PubMed.
- M. Verma, A. F. Chaudhry, M. T. Morgan and C. J. Fahrni, Org. Biomol. Chem., 2010, 8, 363 CAS.
- J. Cody, S. Mandal, L. Yang and C. J. Fahrni, J. Am. Chem. Soc., 2008, 130, 13023 CrossRef CAS PubMed.
- D. Jeyanthi, M. Iniya, K. Krishnaveni and D. Chellappa, Spectrochim. Acta, Part A, 2016, 159, 231 CrossRef CAS PubMed.
- J. L. Bricks, A. Kovalchuk, C. Trieflinger, M. Nofz, M. Buschel, A. I. Tolmachev, J. Daub and K. Rurack, J. Am. Chem. Soc., 2005, 127, 13522 CrossRef CAS PubMed.
- K. Gayathri, K. Velmurugan, R. Nandhakumar, M. Malathi, S. Mathusalini, P. Subramaniam Mohan and R. Shankar, J. Photochem. Photobiol., A, 2017, 341, 136 CrossRef.
- L. Wang, F. Liu, H. Liu, Y. Dong, T. Liu, J. Liu, Y. Yao and X. Wan, Sens. Actuators, B, 2016, 229, 441 CrossRef CAS.
- X.-X. Zheng, S.-Q. Wang, H.-Y. Wang, R.-R. Zhang, J.-T. Liu and B.-X. Zhao, Spectrochim. Acta, Part A, 2015, 138, 247 CrossRef CAS PubMed.
- Z.-L. Gong, B.-X. Zhao, W.-Y. Liu and H.-S. Lv, J. Photochem. Photobiol., A, 2011, 218, 6 CrossRef CAS.
- Z. Zhang, F.-W. Wang, S.-Q. Wang, F. Ge, B.-X. Zhao and J.-Y. Miao, Org. Biomol. Chem., 2012, 10, 8640 CAS.
- S. Hu, J. Song, G. Wu, C. Cheng and Q. Gao, Spectrochim. Acta, Part A, 2015, 136, 1188 CrossRef CAS PubMed.
- S.-Q. Wang, Y. Gao, H.-Y. Wang, X.-X. Zheng, S.-L. Shen, Y.-R. Zhang and B.-X. Zhao, Spectrochim. Acta, Part A, 2013, 106, 110 CrossRef CAS PubMed.
- M. Mukherjee, S. Pal, S. Lohar, B. Sen, S. Sen, S. Banerjee, S. Banerjee and P. Chattopadhyay, Analyst, 2014, 139, 4828 RSC.
- M.-M. Li, S.-Y. Huang, H. Ye, F. Ge, J.-Y. Miao and B.-X. Zhao, J. Fluoresc., 2013, 23, 799 CrossRef CAS PubMed.
- A. Ferle, L. Pizzuti, S. D. Inglez, A. R. L. Caires, E. S. Lang, D. F. Back, A. F. C. Flores, A. M. Júnior, V. M. Deflon and G. A. Casagrande, Polyhedron, 2013, 63, 9 CrossRef CAS.
- L. R. V. Favarin, P. P. Rosa, L. Pizzuti, A. Machulek Jr, A. R. L. Caires, L. S. Bezerra, L. M. C. Pinto, G. Maia, C. C. Gatto, D. F. Back, A. Anjos and G. A. Casagrande, Polyhedron, 2017, 121, 185 CrossRef CAS.
- D. A. Svechkarev, I. V. Bukatich and A. O. Doroshenko, J. Photochem. Photobiol., A, 2008, 200, 426 CrossRef CAS.
- Y. Liu, S.-Q. Wang and B.-X. Zhao, RSC Adv., 2015, 5, 32962 RSC.
- V. Mukundam, A. Kumar, K. Dhanunjayarao, A. Ravi, S. Peruncheralathan and K. Venkatasubbaiah, Polym. Chem., 2015, 6, 7764 RSC.
- M. Ahmed, S. Hameed, A. Ihsan and M. M. Naseer, Sens. Actuators, B, 2017, 248, 57 CrossRef CAS.
- G. Subashini, R. Shankar, T. Arasakumar and P. S. Mohan, Sens. Actuators, B, 2017, 243, 549 CrossRef CAS.
- I. Papagiannouli, A. Szukalski, K. Iliopoulos, J. Mysliwiec, S. Couris and B. Sahraoui, RSC Adv., 2015, 5, 48363 RSC.
- J. Mysliwiec, A. Szukalski, L. Sznitko, A. Miniewicz, K. Haupa, K. Zygadlo, K. Matczyszyn, J. Olesiak-Banska and M. Samoc, Dyes Pigm., 2014, 102, 63 CrossRef CAS.
- A. Szukalski, L. Sznitko, K. Cyprych, A. Miniewicz and J. Mysliwiec, J. Phys. Chem. C, 2014, 118, 8102 CAS.
- J. Barbera, K. Clays, R. Gimenez, S. Houbrechts, A. Persoons and J. L. Serrano, J. Mater. Chem., 1998, 8, 1725 RSC.
- R. Fioravanti, A. Bolasco, F. Manna, F. Rossi, F. Orallo, F. Ortuso, S. Alcaro and R. Cirilli, Eur. J. Med. Chem., 2010, 45, 6135 CrossRef CAS PubMed.
- M. V. R. Reddy, V. K. Billa, V. R. Pallela, M. R. Mallireddigari, R. Boominathan, J. L. Gabriel and E. P. Reddy, Bioorg. Med. Chem., 2008, 16, 3907 CrossRef CAS PubMed.
- S. P. Sakthinathan, G. Vanangamudi and G. Thirunarayanan, Spectrochim. Acta, Part A, 2012, 95, 693 CrossRef CAS PubMed.
- S. Y. Jadhav, S. P. Shirame, S. D. Kulkarni, S. B. Patil, S. K. Pasale and R. B. Bhosale, Bioorg. Med. Chem. Lett., 2013, 23, 2575 CrossRef CAS PubMed.
- B. N. Acharya, D. Saraswat, M. Tiwari, A. K. Shrivastava, R. Ghorpade, S. Bapna and M. P. Kaushik, Eur. J. Med. Chem., 2010, 45, 430 CrossRef CAS PubMed.
- G. Ucar, N. Gokhan, A. Yesilada and A. A. Bilgin, Neurosci. Lett., 2005, 382, 327 CrossRef CAS PubMed.
- Z. Yu, T. Y. Ohulchanskyy, P. An, P. N. Prasad and Q. Lin, J. Am. Chem. Soc., 2013, 135, 16766 CrossRef CAS PubMed.
- Z. Yu, Y. Pan, Z. Wang, J. Wang and Q. Lin, Angew. Chem., Int. Ed., 2012, 51, 10600 CrossRef CAS PubMed.
- J. Wang, W. Zhang, W. Song, Y. Wang, Z. Yu, J. Li, M. Wu, L. Wang, J. Zang and Q. Lin, J. Am. Chem. Soc., 2010, 132, 14812 CrossRef CAS PubMed.
- W. Song, Y. Wang, J. Qu and Q. Lin, J. Am. Chem. Soc., 2008, 130, 9654 CrossRef CAS PubMed.
- M. S. Shah, S. U. Khan, S. A. Ejaz, S. Afridi, S. U. F. Rizvi, M. Najam-ul-Haq and J. Iqbal, Biochem. Biophys. Res. Commun., 2017, 482, 615 CrossRef CAS PubMed.
- V. V. Salian, B. Narayana, B. K. Sarojini, M. S. Kumar, G. S. Nagananda, K. Byrappa and A. K. Kudva, Spectrochim. Acta, Part A, 2017, 174, 254 CrossRef CAS PubMed.
- K. R. A. Abdellatif and W. A. A. Fadaly, Bioorg. Chem., 2017, 70, 57 CrossRef CAS PubMed.
- P. Ahmad, H. Woo, K.-Y. Jun, A. A. Kadi, H. A. Abdel-Aziz, Y. Kwon and A. F. M. M. Rahman, Bioorg. Med. Chem., 2016, 24, 1898 CrossRef CAS PubMed.
- Y.-S. Yang, B. Yang, Y. Zou, G. Li and H.-L. Zhu, Bioorg. Med. Chem., 2016, 24, 3052 CrossRef CAS PubMed.
- R. F. George, M. A. Fouad and I. E. O. Gomaa, Eur. J. Med. Chem., 2016, 112, 48 CrossRef CAS PubMed.
- A. Sid, A. Messai, C. Parlak, N. Kazancı, D. Luneau, G. Kesan, L. Rhyman, I. A. Alswaidan and P. Ramasami, J. Mol. Struct., 2016, 1121, 46 CrossRef CAS.
- A. K. Pandey, S. Sharma, M. Pandey, M. M. Alam, M. Shaquiquzzaman and M. Akhter, Eur. J. Med. Chem., 2016, 123, 476 CrossRef CAS PubMed.
- S. F. McCann, G. D. Annis, R. Shapiro, D. W. Piotrowski, G. P. Lahm, J. K. Long, K. C. Lee, M. M. Hughes, B. J. Myers, S. M. Griswold, B. M. Reeves, R. W. March, P. L. Sharpe, P. Lowder, W. E. Barnette and K. D. Wing, Pest Manag. Sci., 2001, 57, 153 CrossRef CAS PubMed.
- K. S. Silver and D. M. Soderlund, Pestic. Biochem. Physiol., 2005, 81, 136 CrossRef CAS.
- D. M. T. Chan, T. M. Stevenson, D. W. Piotrowski, C. R. Harrison, M. A. H. Fahmy, R. L. Lowe, K. L. Monaco, B. M. Reeves, M. P. Folgar and E. G. Esrey, Synthesis and Chemistry of Agrochemicals VI, ACS Symposium Series, American Chemical Society, Washington, DC, 2001 Search PubMed.
- N.-J. Fan, S.-P. Wei, J.-M. Gao and J.-J. Tang, J. Braz. Chem. Soc., 2015, 26, 389 CAS.
- S.-Y. Bai, X. Dai, B.-X. Zhao and J.-Y. Miao, RSC Adv., 2014, 4, 19887 RSC.
- A. Ciupa, P. A. D. Bank, M. F. Mahon, P. J. Wood and L. Caggiano, MedChemComm, 2013, 4, 956 RSC.
- X. Jia, Y. Li, L. Xiao and L. Liu, Polym. Chem., 2014, 5, 4781 RSC.
- C. Chen, Y. Li and L. Liu, RSC Adv., 2016, 6, 105723 RSC.
- S.-Q. Wang, Q.-H. Wu, H.-Y. Wang, X.-X. Zheng, S.-L. Shen, Y.-R. Zhang, J.-Y. Miao and B.-X. Zhao, Analyst, 2013, 138, 7169 RSC.
- J. Li, D. Li, Y. Han, S. Shuang and C. Dong, Spectrochim. Acta, Part A, 2009, 73, 221 CrossRef PubMed.
- D. K. Rana, S. Dhar and S. C. Bhattacharya, Phys. Chem. Chem. Phys., 2014, 16, 5933 RSC.
- S. S. Mati, S. Sarkar, S. Rakshit, A. Sarkar and S. C. Bhattacharya, RSC Adv., 2013, 3, 8071 RSC.
- S. S. Mati, S. Sarkar, P. Sarkar and S. C. Bhattacharya, J. Phys. Chem. A, 2012, 116, 10371 CrossRef CAS PubMed.
- B. Varghese, S. N. Al-Busafi, F. O. Suliman and S. M. Z. Al-Kindy, Spectrochim. Acta, Part A, 2015, 136, 661 CrossRef CAS PubMed.
- B. Varghese, S. N. Al-Busafi, F. O. Suliman and S. M. Z. Al-Kindy, Spectrochim. Acta, Part A, 2017, 173, 383 CrossRef CAS PubMed.
- S. W. Oh, D. R. Zhang and Y. S. Kang, Mater. Sci. Eng., C, 2004, 24, 131 CrossRef.
- D. Xiao, L. Xi, W. Yang, H. Fu, Z. Shuai, Y. Fang and J. Yao, J. Am. Chem. Soc., 2003, 125, 6740 CrossRef CAS PubMed.
- H. Fu, B. H. Loo, D. Xiao, R. Xie, X. Ji, J. Yao, B. Zhang and L. Zhang, Angew. Chem., Int. Ed., 2002, 41, 962 CrossRef CAS PubMed.
- H. Fu, D. Xiao, J. Yao and G. Yang, Angew. Chem., Int. Ed., 2003, 42, 2883 CrossRef CAS PubMed.
- S. W. Oh, J. H. Suh and Y. S. Kang, Colloids Surf., A, 2006, 284, 359 CrossRef.
- S. W. Oh, C. W. Kim, S.-M. Son and Y. S. Kang, J. Colloid Interface Sci., 2011, 357, 31 CrossRef CAS PubMed.
- Z. Xuan and L. Lu, Mater. Lett., 2011, 65, 754 CrossRef CAS.
- L. Kang, Y. Chen, D. Xiao, A. Peng, F. Shen, X. Kuang, H. Fu and J. Yao, Chem. Commun., 2007, 2695 RSC.
- F. Shen, A. Peng, Y. Chen, Y. Dong, Z. Jiang, Y. Wang, H. Fu and J. Yao, J. Phys. Chem. A, 2008, 112, 2206 CrossRef CAS PubMed.
- Y. Dai, M. Guo, J. Peng, W. Shen, M. Li, R. He, C. Zhu and S. H. Lin, Chem. Phys. Lett., 2013, 556, 230 CrossRef CAS.
- E. Gondek, Mater. Lett., 2013, 112, 94 CrossRef CAS.
- J. Ajantha, E. Varathan, V. Bharti, V. Subramanian, S. Easwaramoorthi and S. Chand, RSC Adv., 2016, 6, 786 RSC.
- C. Lu, Q. Liu, P. Gu, D. Chen, F. Zhou, H. Li, Q. Xu and J. Lu, Polym. Chem., 2014, 5, 2602 RSC.
- H. R. Sobhi, Y. Yamini, A. Esrafili and M. Adib, J. Pharm. Biomed. Anal., 2008, 48, 1059 CrossRef CAS PubMed.
- B. Varghese, S. N. Al-Busafi, F. O. Suliman and S. M. Z. Al-Kindy, Anal. Methods, 2016, 8, 2729 RSC.
- B. Varghese, S. H. Al-Harthi, S. N. Al-Busafi, F. O. Suliman, S. M. Z. Al-Kindy and M. T. Z. Myint, J. Lumin., 2017, 192, 527 CrossRef CAS.
|
This journal is © The Royal Society of Chemistry 2017 |
Click here to see how this site uses Cookies. View our privacy policy here.