DOI:
10.1039/C6QO00095A
(Research Article)
Org. Chem. Front., 2016,
3, 809-812
Catalytic asymmetric α-amination of β-keto esters and β-keto amides with a chiral N,N′-dioxide–copper(I) complex†
Received
8th March 2016
, Accepted 27th April 2016
First published on 9th May 2016
Abstract
The highly efficient asymmetric α-amination of β-keto esters and β-keto amides was realized by using a chiral N,N′-dioxide/Cu(I) complex. Under mild reaction conditions, a series of corresponding chiral α-amino dicarbonyl compounds were obtained in excellent yields (up to 98%) with excellent enantioselectivities (up to 95% ee).
Introduction
Optically active amino acids are distributed widely in natural products and medicines, exhibiting important biological or medicinal activities.1 The asymmetric α-amination of 1,3-dicarbonyl compounds with azodicarboxylates is an efficient and simple strategy to construct optically active α-amino carbonyl compounds bearing a quaternary stereocenter.2 Therefore, this reaction has gained much attention.3–7 Since the pioneer work of Jørgensen and co-workers6b using a copper(II)-box catalyst to accomplish this transformation, different catalytic systems, including metal complexes,3,6 organocatalysts4 and phase-transfer catalysts,5 have recently been reported. However, most of these studies are concentrated on β-keto esters. The reports on the α-amination of β-keto amides are relatively rare7 although the amino group is useful for their further easy manipulation,8 due to the lower acidity of the α-hydrogen.9 So, developing new catalytic systems, efficient not only for the enantioselective α-amination of β-keto esters but also for that of β-keto amides, is still necessary and meaningful.
Copper, with mild Lewis acidity, has been proved to be particularly effective at activating the nitrogen atom.6,10 Several chiral copper(II) complexes have been applied in α-amination reactions.6 The application of copper(I) complexes in α-amination reactions needs to be developed. On the other hand, chiral N,N′-dioxides, developed by our group, have been proved to be efficient ligands or organocatalysts in various asymmetric transformations.11 Herein, we describe our efforts in developing an N,N′-dioxide/Cu(I) complex catalytic system for the enantioselective α-amination of β-keto esters as well as β-keto amides.
Results and discussion
Initially, we investigated several copper salts complexing with a L-pipecolic acid derived ligand L-PiEt2 to promote the α-amination of the β-keto ester 1c using commercially available diethyl azodicarboxylate 2a as the amination reagent in CH2Cl2 at 35 °C. As shown in Table 1, the L-PiEt2–Cu(OTf)2 complex provided the product in good yield but moderate enantioselectivity (73% yield, 46% ee; Table 1, entry 1), while the L-PiEt2–CuOTf·(C7H8)0.5 complex provided the product in excellent yield and good enantioselectivity (90% yield, 74% ee, Table 1, entry 2). Since Cu(I) afforded the product with better results than Cu(II) did, the counterion of Cu(I) was then screened. When CuCl, CuBr and CuI were used, the ee value was further improved (Table 1, entries 3–5) though the yield decreased a little, and CuI gave the highest yield and ee (89% yield and 90% ee). Encouraged by these results, the efficiency of CuI with other N,N′-dioxide ligands was explored (Fig. 1). It was showed that the reactivity and enantioselectivity were closely dependent on both the substituent R of the amide moiety and the chiral backbone of the ligand. Increasing the steric hindrance of the R group from methyl to isopropyl (Table 1, entries 5–7) led to an obvious increase in the yield and ee value. But the more hindered ligand L-PiPr3 derived from 2,4,6-triisopropylaniline didn't give better results (Table 1, entry 8). Besides, L-pipecolic acid derived L-PiPr2 was superior to L-proline-derived L-PrPr2 and L-ramipril-derived L-RaPr2 in enantioselectivity (Table 1, entry 7 vs. entries 9 and 10).
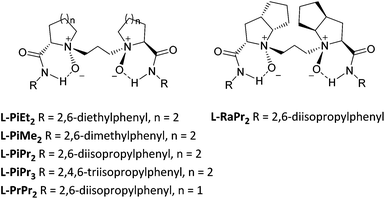 |
| Fig. 1 Ligands screened in this study. | |
Table 1 Screen of Lewis acids and N,N′-dioxide ligandsa
With the optimized conditions in hand, the substrate scope of the reaction was evaluated, and the results are summarized in Table 2. When 1-tetralone-derived β-keto esters were investigated, the ee values of the products increased gradually with the increase of the steric hindrance of the R2 group located on the ester side and the yields were maintained (Table 2, entries 1–3). When the R2 group was varied from methyl, ethyl to adamantyl, the ee value increased from 80%, 86% to 95%. The 1-tetralone-derived β-keto esters, bearing electron-donating groups at different positions on the phenyl ring (Table 2, entries 4–6), could transform into the corresponding products in excellent yield and high ee values (91–98% yield and 90–94% ee). Next, the scope of azodicarboxylates 2 was tested, with the increase of the steric hindrance of the R3 group located on the ester side (Table 2, entry 3 vs. entries 7 and 8), both the yield and the ee value decreased obviously. When the R3 group was changed from ethyl, isopropyl to tert-butyl, the yield decreased from 96%, 95% to 76% and the ee value decreased from 95%, 90% to 82%. Dibenzyl azodicarboxylate 2d was also a suitable substrate, which was converted to the corresponding product 3cd in 98% yield and 90% ee value (Table 2, entry 9).
Table 2 Substrate scope of the catalytic asymmetric α-amination of β-keto estersa
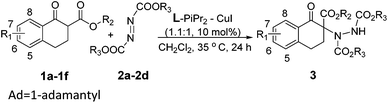
|
Entry |
R1, R2, R3 |
Product |
Yieldb (%) |
eec (%) |
Unless otherwise noted, all reactions were performed with L-PiPr2/CuI (1.1 : 1, 10 mol%), 1 (0.1 mmol), and 2 (0.12 mmol) in CH2Cl2 (0.5 mL) at 35 °C for 24 hours.
Isolated yield.
Determined by chiral HPLC analysis.
|
1 |
H, Me, Et |
3aa
|
94 |
80 |
2 |
H, Et, Et |
3ba
|
92 |
86 |
3 |
H, Ad, Et |
3ca
|
96 |
95 |
4 |
5-MeO, Ad, Et |
3da
|
91 |
90 |
5 |
6-MeO, Ad, Et |
3ea
|
98 |
94 |
6 |
7-MeO, Ad, Et |
3fa
|
95 |
94 |
7 |
H, Ad, i-Pr |
3cb
|
95 |
90 |
8 |
H, Ad, t-Bu |
3cc
|
76 |
82 |
9 |
H, Ad, Bn |
3cd
|
98 |
90 |
After investigation of the β-keto esters, we then turn our attention to the more challenging 1-tetralone-derived β-keto amides (Table 3). The amides with a more hindered 1-adamantyl or tert-butyl substituent on the nitrogen atom could give better results (90–96% yield, 87–92% ee; Table 3, entries 1–6). In terms of azodicarboxylates, generally, compared to diethyl azodicarboxylate 2a and dibenzyl azodicarboxylate 2d, the diisopropyl azodicarboxylate 2b afforded the products in lower yields with lower enantioselectivities. Next, the influence of substituents on 1-tetralone-derived β-ketone amides was tested. The amides, bearing electron-donating groups at different positions on the phenyl ring, could transform into the corresponding products in high yields and high ee values (89–93% yield and 90–94% ee, Table 3, entries 7–10). Besides, the 1-indanone-derived β-keto amide 4g and 1-benzosuberanone-derived β-keto amide 4h were also examined with the same catalytic system. However, the enantioselectivities were very poor since only racemic 5ga and 5ha with 0% and 25% ee, respectively, were obtained (Table 3, entries 11 and 12).
Table 3 Substrate scope of the catalytic asymmetric α-amination of β-keto amidesa
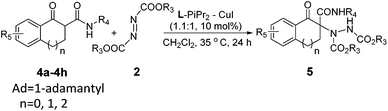
|
Entry |
n, R3, R4, R5 |
Product |
Yieldb (%) |
eec (%) |
Unless otherwise noted, all reactions were performed with L-PiPr2/CuI (1.1 : 1, 10 mol%), 4 (0.1 mmol), 2 (0.12 mmol) in CH2Cl2 (0.5 mL) at 35 °C for 24 hours.
Isolated yield.
Determined by chiral HPLC analysis.
The reaction time was 48 hours.
|
1 |
1, Et, Ad, H |
5aa
|
95 |
90 |
2 |
1, i-Pr, Ad, H |
5ab
|
90 |
87 |
3 |
1, Bn, Ad, H |
5ad
|
96 |
90 |
4 |
1, Et, t-Bu, H |
5ba
|
96 |
92 |
5 |
1, i-Pr, t-Bu, H |
5bb
|
90 |
88 |
6 |
1, Bn, t-Bu, H |
5bd
|
94 |
90 |
7d |
1, Et, t-Bu, 5-MeO |
5ca
|
90 |
90 |
8d |
1, Et, t-Bu, 6-MeO |
5da
|
93 |
92 |
9d |
1, Et, t-Bu, 7-MeO |
5ea
|
92 |
92 |
10d |
1, Et, t-Bu, 5,7-Me2 |
5fa
|
89 |
94 |
11d |
0, Et, t-Bu, H |
5ga
|
82 |
0 |
12d |
2, Et, t-Bu, H |
5ha
|
87 |
25 |
For the purpose of examining the synthetic potential of the catalytic system, a gram-scaled synthesis of 3cd was performed (Scheme 1). As shown in Scheme 1, under the optimized reaction conditions (Table 2, entry 9), 2 mmol 1c reacted with 1.2 equivalents of 2d smoothly, giving 1.20 g (95% yield) of the desired product 3cd with 90% ee.
 |
| Scheme 1 Scaled-up version of the catalytic asymmetric amination reaction. | |
Conclusions
In summary, we have developed a highly efficient N,N′-dioxide/Cu(I) complex catalytic system for the asymmetric α-amination of β-keto esters and β-keto amides by using dialkyl azodicarboxylates as amination reagents. A series of corresponding chiral α-amino dicarbonyl compounds were obtained in excellent yields (up to 98%) and excellent enantioselectivities (up to 95% ee) under mild reaction conditions. The synthetic potential of this methodology was also demonstrated by the excellent results obtained on a gram scale.
Acknowledgements
We appreciate the National Natural Science Foundation of China (no. 21321061 and 21372162) for financial support.
Notes and references
-
(a)
R. M. Williams, in Synthesis of Optically Active a-Amino Acids, ed. J. E. Baldwin, Pergamon Press, Oxford, 1989 Search PubMed
;
(b) H. Heimgartner, Angew. Chem., Int. Ed. Engl., 1991, 30, 238 CrossRef
;
(c) R. M. Williams and J. A. Hendrix, Chem. Rev., 1992, 92, 889 CrossRef CAS
;
(d) M. Arend, Angew. Chem., Int. Ed., 1999, 38, 2873 CrossRef CAS
;
(e) S. C. Bergmeier, Tetrahedron, 2000, 56, 2561 CrossRef CAS
;
(f) L. Yet, Angew. Chem., Int. Ed., 2001, 40, 875 CrossRef CAS
;
(g) T. Ooi, M. Takahashi, K. Doda and K. Maruoka, J. Am. Chem. Soc., 2002, 124, 7640 CrossRef CAS PubMed
;
(h) J. T. Suri, D. D. Steiner and C. F. Barbas III, Org. Lett., 2005, 7, 3885 CrossRef CAS PubMed
;
(i) G. Li, L. Dai and S. You, Chem. Commun., 2009, 1807 Search PubMed
.
- For representative reviews on asymmetric α-amination reaction, see:
(a) R. O. Duthaler, Angew. Chem., Int. Ed., 2003, 42, 975 CrossRef CAS PubMed
;
(b) C. Greck, B. Drouillat and C. Thomassigny, Eur. J. Org. Chem., 2004, 1377 CrossRef CAS
;
(c) E. Erdik, Tetrahedron, 2004, 60, 8747 CrossRef CAS
;
(d) J. M. Janey, Angew. Chem., Int. Ed., 2005, 44, 4292 CrossRef CAS PubMed
;
(e) G. Guillena and D. J. Ramón, Tetrahedron: Asymmetry, 2006, 17, 1465 CrossRef CAS
;
(f) N. J. M. Sansano, Chem. Rev., 2007, 107, 4584 CrossRef PubMed
;
(g) V. Nair, A. T. Biju, S. C. Mathew and B. P. Babu, Chem. – Asian J., 2008, 3, 810 CrossRef CAS PubMed
;
(h) L.-W. Xu, J. Luo and Y. Lu, Chem. Commun., 2009, 1807 RSC
;
(i) T. Vilaivan and W. Bhanthumnavin, Molecules, 2010, 15, 917 CrossRef CAS PubMed
;
(j) A. Vallribera, R. M. Sebastian and A. Shafir, Curr. Org. Chem., 2011, 15, 1539 CrossRef CAS
;
(k) A. Russo, C. De Fusco and A. Lattanzi, RSC Adv., 2012, 2, 385 RSC
;
(l) F. Zhou, F.-M. Liao, J.-S. Yu and J. Zhou, Synthesis, 2014, 2983 CrossRef CAS
.
- For selected examples of metal-catalyzed electrophilic amination of 1,3-dicarbonyl compounds with diazocarboxylates, see:
(a) Y. K. Kang and D. Y. Kim, Tetrahedron Lett., 2006, 47, 4565 CrossRef CAS
;
(b) T. Mashiko, N. Kumagai and M. Shibasaki, J. Am. Chem. Soc., 2009, 131, 14990 CrossRef CAS PubMed
;
(c) J. Y. Mang, D. G. Kwon and D. Y. Kim, Bull. Korean Chem. Soc., 2009, 30, 249 CrossRef CAS
;
(d) A. Pericas, A. Shafir and A. Vallribera, Org. Lett., 2013, 15, 1448 CrossRef CAS PubMed
;
(e) M. Torres, A. Maisse-Francois and S. Bellemin-Laponaz, ChemCatChem, 2013, 5, 3078 CrossRef CAS
.
- For selected examples of organocatalyst-catalyzed electrophilic amination of 1,3-dicarbonyl compounds with diazocarboxylates, see:
(a) S. Saaby, M. Bella and K. A. Jørgensen, J. Am. Chem. Soc., 2004, 126, 8120 CrossRef CAS PubMed
;
(b) P. M. Pihko and A. Pohjakallio, Synlett, 2004, 2115 CrossRef CAS
;
(c) X. Liu, H. Li and L. Deng, Org. Lett., 2005, 7, 169 Search PubMed
;
(d) L. Santacruz, S. Niembro, A. Santillana, A. Shafir and A. Vallribera, New J. Chem., 2014, 38, 636 RSC
;
(e) X. Xu, T. Yabuta, P. Yuan and Y. Takemoto, Synlett, 2006, 137 Search PubMed
;
(f) M. Terada, M. Nakano and H. Ube, J. Am. Chem. Soc., 2006, 128, 16044 CrossRef CAS PubMed
;
(g) H. Konishi, T. Y. Lam, J. P. Malerich and V. H. Rawal, Org. Lett., 2010, 12, 2028 CrossRef CAS PubMed
;
(h) X. Han, F. Zhong and Y. Lu, Adv. Synth. Catal., 2010, 352, 2778 CrossRef CAS
;
(i) P. Trillo, M. G. Martinez, A. A. Diego and A. Baeza, Synlett, 2015, 95 CAS
.
- For selected examples of phase-transfer-catalyzed electrophilic amination of 1,3-dicarbonyl compounds with diazocarboxylates, see:
(a) R. He, X. Wang, T. Hashimoto and K. Maruoka, Angew. Chem., Int. Ed., 2008, 47, 9466 CrossRef CAS PubMed
;
(b) Q. Lan, X. Wang, R. He, C. Ding and K. Maruoka, Tetrahedron Lett., 2009, 50, 3280 CrossRef CAS
;
(c) R. He and K. Maruoka, Synthesis, 2009, 2289 CAS
.
- For selected examples of copper-catalyzed electrophilic amination, see:
(a) S. Ghosh, M. V. Nandakumar, H. Krautscheid and C. Schneider, Tetrahedron Lett., 2010, 51, 1860 CrossRef CAS
;
(b) M. Marigo, K. Juhl and K. A. Jørgensen, Angew. Chem., Int. Ed., 2003, 42, 1367–1369 CrossRef CAS PubMed
;
(c) C. Foltz, B. Stecker, G. Marconi, S. Bellemin-Laponaz, H. Wadepohl and L. H. Gade, Chem. Commun., 2005, 5115 RSC
;
(d) M. V. Nandakumar, S. Ghosh and C. Schneider, Eur. J. Org. Chem., 2009, 6393 CrossRef CAS
;
(e) S. Ghosh, M. V. Nandakumar, H. Krautscheid and C. Schneider, Tetrahedron Lett., 2010, 51, 1860 CrossRef CAS
;
(f) D. Sandoval, P. F. Charles, A. Bugarin and J. Read de Alaniz, J. Am. Chem. Soc., 2012, 134, 18948 CrossRef CAS PubMed
;
(g) S. Ma, N. Jiao, Z. Zheng, Z. Ma, Z. Lu, L. Ye, Y. Deng and G. Chen, Org. Lett., 2004, 6, 2193 CrossRef CAS PubMed
;
(h) D. P. Huber, K. Stanek and A. Togni, Tetrahedron: Asymmetry, 2006, 17, 658 CrossRef CAS
.
- For selected examples of electrophilic amination of keto amide compounds with diazocarboxylates, see:
(a) D. P. Huber, K. Stanek and A. Togni, Tetrahedron: Asymmetry, 2006, 17, 658 CrossRef CAS
;
(b) F. Berhal, S. Takechi, N. Kumagai and M. Shibasaki, Chem. – Eur. J., 2011, 17, 1915 CrossRef CAS PubMed
;
(c) T. Mashiko, N. Kumagai and M. Shibasaki, J. Am. Chem. Soc., 2009, 131, 14990 CrossRef CAS PubMed
.
-
(a) A. W. Pilling, J. Boehmer and D. J. Dixon, Angew. Chem., Int. Ed., 2007, 46, 5428 CrossRef CAS PubMed
;
(b) A. W. Pilling, J. Böhmer and D. Dixon, Chem. Commun., 2008, 832 RSC
;
(c) J. Franzén and A. Fisher, Angew. Chem., Int. Ed., 2009, 48, 787 CrossRef PubMed
;
(d) Z. Jin, X. Wang, H. Huang, X. Liang and J. Ye, Org. Lett., 2011, 13, 564 CrossRef CAS PubMed
;
(e) C. Pan, X. Zeng, Y. Guan, X. Jiang, L. Li and H. Zhang, Synlett, 2011, 425 CAS
.
-
(a) P. Y. Toullec, C. Bonaccorsi, A. Mezzetti and A. Togni, Proc. Natl. Acad. Sci. U. S. A., 2004, 101, 5810 CrossRef CAS PubMed
;
(b) J. Yu, J. Cui and C. Zhang, Eur. J. Org. Chem., 2010, 7020 CrossRef CAS
.
-
Copper-Catalyzed Asymmetric Synthesis, ed. A. Alexakis, N. Krause and S. Woodward, Wiley, 2014, pp. 1–472 Search PubMed
.
- For reviews on chiral N-oxides in asymmetric catalysis, see:
(a)
X. M. Feng and X. H. Liu, in Scandium: Compounds, Productions and Applications, ed. V. A. Greene, Nova Science, New York, 2011, pp. 1–48 Search PubMed
;
(b) X. H. Liu, L. L. Lin and X. M. Feng, Acc. Chem. Res., 2011, 44, 574 CrossRef CAS PubMed
;
(c) K. Shen, X. H. Liu, L. L. Lin and X. M. Feng, Chem. Sci., 2012, 3, 327 RSC
;
(d) X. H. Liu, L. L. Lin and X. M. Feng, Org. Chem. Front., 2014, 1, 298 RSC
. For recent examples see:
(e) Z. G. Yang, Z. Wang, S. Bai, K. Shen, D. H. Chen, X. H. Liu, L. L. Lin and X. M. Feng, Chem. – Eur. J., 2010, 16, 6632 CrossRef CAS PubMed
;
(f) L. Chang, Y. L. Kuang, B. Qin, X. Zhou, X. H. Liu, L. L. Lin and X. M. Feng, Org. Lett., 2010, 12, 2214 CrossRef CAS PubMed
;
(g) Z. G. Yang, Z. Wang, S. Bai, X. H. Liu, L. L. Lin and X. M. Feng, Org. Lett., 2011, 13, 596 CrossRef CAS PubMed
;
(h) Z. Wang, Z. L. Chen, S. Bai, W. Li, X. H. Liu, L. L. Lin and X. M. Feng, Angew. Chem., Int. Ed., 2012, 51, 2776 CrossRef CAS PubMed
;
(i) J. N. Zhao, X. H. Liu, W. W. Luo, M. S. Xie, L. L. Lin and X. M. Feng, Angew. Chem., Int. Ed., 2013, 52, 3473 CrossRef CAS PubMed
;
(j) M. S. Xie, X. H. Liu, X. X. Wu, Y. F. Cai, L. L. Lin and X. M. Feng, Angew. Chem., Int. Ed., 2013, 52, 5604 CrossRef CAS PubMed
;
(k) W. Li, X. H. Liu, X. Y. Hao, Y. F. Cai, L. L. Lin and X. M. Feng, Angew. Chem., Int. Ed., 2012, 51, 8644 CrossRef CAS PubMed
;
(l) J. Guo, S. X. Dong, Y. L. Zhang, Y. L. Kuang, X. H. Liu, L. L. Lin and X. M. Feng, Angew. Chem., Int. Ed., 2013, 52, 10245 CrossRef CAS PubMed
;
(m) W. Li, X. H. Liu, F. Tan, X. Y. Hao, J. F. Zheng, L. L. Lin and X. M. Feng, Angew. Chem., Int. Ed., 2013, 52, 10883 CrossRef CAS PubMed
;
(n) L. Zhou, X. H. Liu, J. Ji, Y. H. Zhang, L. L. Lin and X. M. Feng, J. Am. Chem. Soc., 2012, 134, 17023 CrossRef CAS PubMed
;
(o) H. F. Zheng, X. H. Liu, C. R. Xu, Y. Xia, L. L. Lin and X. M. Feng, Angew. Chem., Int. Ed., 2015, 54, 10958 CrossRef CAS PubMed
;
(p) X. H. Zhao, X. H. Liu, H. J. Mei, J. Guo, L. L. Lin and X. M. Feng, Angew. Chem., Int. Ed., 2015, 54, 4032 CrossRef CAS PubMed
.
Footnote |
† Electronic supplementary information (ESI) available. See DOI: 10.1039/c6qo00095a |
|
This journal is © the Partner Organisations 2016 |