DOI:
10.1039/C6QO00134C
(Research Article)
Org. Chem. Front., 2016,
3, 813-816
Cobalt(III)-catalyzed efficient synthesis of indenones through carboannulation of benzoates and alkynes†
Received
31st March 2016
, Accepted 27th April 2016
First published on 28th April 2016
Abstract
Cobalt(III)-catalyzed C–H activation of simple benzoate esters has been achieved, and redox-neutral annulative coupling with internal alkynes allowed efficient synthesis of indenones. The employment of the weak and simple ester directing group makes it an attractive protocol in carbocycle synthesis.
In the past few decades, transition metal-catalyzed C–H functionalization of arenes has been widely employed as an advantageous strategy to streamline chemical synthesis without prefunctionalization of arenes.1 Among the transition metals, cobalt(III) has attracted increasing attention owing to its earth-abundance, low cost, low toxicity, and unique catalytic reactivity compared with heavy metal congeners such as Rh(III) and Ir(III).2 Indeed, various types of C–C and C-heteroatom bond-forming reactions have been developed via cobalt-catalyzed C–H activation recently, as in the reports by Kanai/Matsunaga,3 Glorius,4 Ackermann,5 Ellman,6 Daugulis,7 Chang,8 and others.9 However, compared to the well established Rh(III) catalysis,10 only a handful examples of C–H activation systems have been realized via cobalt(III) catalysis that lead to construction of heterocycles,11 and the construction of carbocycles is even unprecedented.
Indenones are important structural motifs that have found broad applications in synthetic chemistry, medicinal chemistry, and materials science.12 Thus, the development of synthetic methods to access the indenone skeleton via an efficient C–H activation pathway has attracted increasing attention over the past few decades (Scheme 1).13 For example, under rhodium catalysis the annulative couplings of aroyl chlorides,14 benzaldehydes,15 and benzamides16 with internal alkynes delivered indenones as a result of ortho-functionalization. Recently, we reported mild indenone synthesis via Rh(III)-catalyzed carbocyclization between arylnitrones and alkynes under redox-neutral conditions.17 In spite of the progress, these coupling systems mostly relied heavily on precious late metal catalysts and/or tediously synthesized substrates. Thus, the development of a more readily available substrate for the construction of the indenone framework in an efficient and atom-economical fashion using an inexpensive cobalt catalyst is highly desirable. We identified benzoate esters as a suitable candidate owing to their low cost and ready availability. While Shi and coworkers briefly demonstrated their application in Rh(III)-catalyzed indenone synthesis in one example, the efficiency was low.16b We reasoned that the weak directing effect of the ester group called for a more Lewis acidic metal. Therefore, Co(III) catalysts likely make efficient catalysts in that the stronger ligation of the ester directing group should favor the C–H activation. In addition, the stronger nucleophilicity of the cobalt alkenyl species should lead to more favorable interactions with the weakly electrophilic ester group. We now report cobalt(III) catalyzed C–H activation of benzoates with subsequent annulative coupling with internal alkynes, leading to efficient synthesis of indenones.
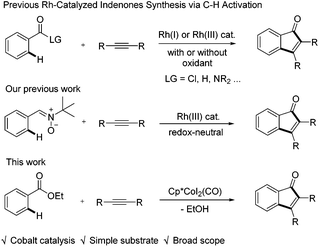 |
| Scheme 1 Transition metal-catalyzed indenone synthesis. | |
We commenced our studies by examining the reaction parameters of the coupling between ethyl benzoate (1a) and diphenylacetylene (2a) in the presence of a cobalt(III) catalyst (Table 1). While traces of the product were detected using [Cp*CoI2]2/AgNTf2 as a catalyst with or without a NaOAc additive (Table 1, entries 1 and 2), switching the additive to Cu(OAc)2 improved the efficiency and the desired indenone 3aa was isolated in 21% yield using HFIP as a solvent (entry 3). No desired product was observed when AgOAc was used as an additive (entry 4). Various cobalt catalysts were then screened (entries 5 and 6) and Cp*Co(CO)I2 proved to be the optimal catalyst, affording product 3aa in 86% isolated yield (entry 7). In contrast, a lower yield was obtained when AgSbF6 was used as a halide scavenger (entry 8). Screening of the solvents revealed that other solvents tended to give inferior results (entries 9 and 10).
Table 1 Screening of reaction conditionsa
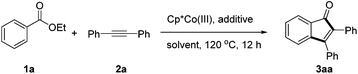
|
Entry |
Cat. (mol%/20 mol%) |
Additive |
Solvent |
Yieldb (%) |
Reaction conditions: ethyl benzoate (1a, 0.2 mmol), diphenylacetylene (2a, 0.24 mmol), catalyst/silver salt, additive (2.0 equiv.), solvent (2 mL),120 °C, 12 h, pressure tube under N2.
Isolated yield after column chromatography.
HFIP = 1,1,1,3,3,3-hexafluoro-iso-propanol.
|
1 |
[Cp*CoI2]2(5)/AgNTf2 |
— |
HFIPc |
<5 |
2 |
[Cp*CoI2]2(5)/AgNTf2 |
NaOAc |
HFIP |
<5 |
3 |
[Cp*CoI2]2(5)/AgNTf2 |
Cu(OAc)2 |
HFIP |
21 |
4 |
[Cp*CoI2]2(5)/AgOAc |
Cu(OAc)2 |
HFIP |
<5 |
5 |
[Cp*CoI2]2(5)/AgNTf2 |
Zn(OAc)2 |
HFIP |
46 |
6 |
[Cp*CoCl2]2(5)/AgNTf2 |
Zn(OAc)2 |
HFIP |
55 |
7 |
Cp*Co(CO)I2(10)/AgNTf2 |
Zn(OAc)2 |
HFIP |
86 |
8 |
Cp*Co(CO)I2(10)/AgSbF6 |
Zn(OAc)2 |
HFIP |
72 |
9 |
Cp*Co(CO)I2(10)/AgNTf2 |
Zn(OAc)2 |
TFE |
76 |
10 |
Cp*Co(CO)I2(10)/AgNTf2 |
Zn(OAc)2 |
DCE |
69 |
The effects of the ester group were further examined by following the optimized conditions (Scheme 2). It turned out that other esters such as methyl, n-propyl, and i-propyl esters were all applicable, affording the indenone product in 58–80% yield. In contrast, essentially no desired product was obtained when t-butyl or phenyl benzoate was used as an arene substrate, indicating that both steric and electronic effects of the leaving group played an important role.
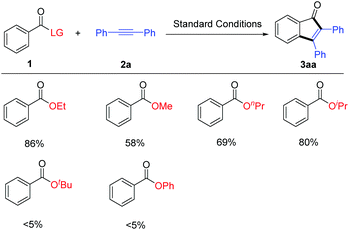 |
| Scheme 2 Effects of the leaving group. Reaction conditions: benzoate esters (1, 0.2 mmol), diphenylacetylene (2a, 0.24 mmol), HFIP (2 mL), Zn(OAc)2 (2.0 equiv.), 120 °C, 12 h, sealed tube under N2. Isolated yield after column chromatography. | |
With the optimized reaction conditions in hand, we first examined the scope and generality of the reaction (Scheme 3). A wide variety of ethyl benzoates bearing various electron-donating and halogen groups at the para position were compatible, giving the coupled products 3aa–3ga in 51–91% yields. It was observed that introduction of an electron-withdrawing group caused a slight decrease of the reaction efficiency. Various meta-substituted substrates also proceeded efficiently at both the ortho positions with low to good regioselectivities (1.3–15
:
1). In contrast to the reactions of 3ha and 3ja, ethyl 3-fluorobenzoate reacted in high yield (90%) and excellent regioselectivity (15
:
1) at the more hindered ortho position (3ia) with the assistance of the ligating effect of the F atom and its reduced steric bulk.18 Moreover, ortho-substituted ethyl benzoates were also tolerated (3ka–3na). The benzoates could also be extended to ethyl 1-naphthoate in good yield (3oa).
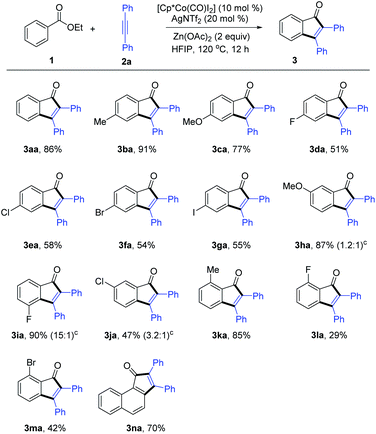 |
| Scheme 3 Scope of ethyl esters. Reaction conditions: ethyl esters (1, 0.2 mmol), diphenylacetylene (2a, 0.24 mmol), HFIP (2 mL), Zn(OAc)2 (2.0 equiv.), 120 °C, 12 h, sealed tube under N2. Isolated yield after column chromatography. Regioselectivity ratio (determined by 1H NMR spectroscopy). | |
The scope of diarylacetylenes was next investigated (Scheme 4). The substituent in the diarylacetylene exhibited profound electronic effects, with a lower efficiency being observed for an EDG-functionalized diarylacetylene (3ab and 3ac). Various diarylacetylenes with electron-withdrawing groups at the para position of the phenyl ring all reacted smoothly to give moderate to high yields (3ad–3af). In addition, diarylacetylenes with substituents such as methyl and halide groups at the meta position were also tolerated. In contrast, aryl-, alkyl- alkynes or internal aliphatic alkynes only reacted with poor efficiency under the standard conditions.
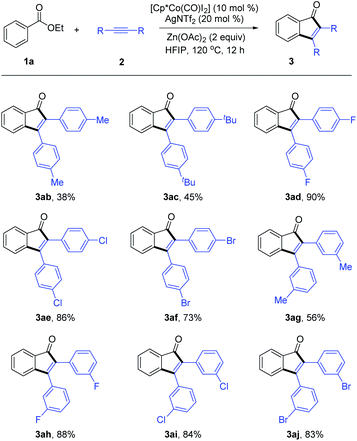 |
| Scheme 4 Scope of the alkyne substrates. Reaction conditions: ethyl benzoate (1a, 0.2 mmol), diarylacetylenes (2, 0.24 mmol), HFIP (2 mL), Zn(OAc)2 (2.0 equiv.), 120 °C, 12 h, sealed tube under N2. Isolated yield after chromatography. | |
To gain some insights into the reaction mechanism, several experiments were conducted (Scheme 5). A kinetic isotope effect (KIE) value of 4.5 was obtained using 1a and 1a-d5 by two side-by-side reactions in the coupling with 2a at a low conversion. This result indicated that the C–H bond cleavage is likely involved in the turnover-limiting step. When an equimolar mixture of 1b and 1e was allowed to compete under the standard conditions in the coupling with diphenylacetylene 2a, two indenones were obtained in 2.3
:
1 ratio, where an electron-rich benzoate reacted preferentially.
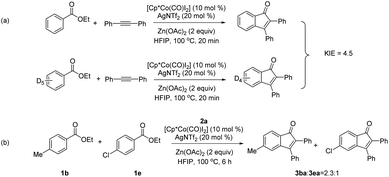 |
| Scheme 5 Mechanistic studies. | |
On the basis of our mechanistic experiments and previous reports,11k a plausible catalytic cycle is given in Scheme 6. An active catalyst [Cp*Co(OAc)2] was generated from the ligand substitution between the cobalt catalyst precursor and AgNTf2 and Zn(OAc)2. C–H activation of ethyl benzoate gives a five-membered metallacyclic intermediate A. Alkyne insertion gives a seven-membered Co(III) alkenyl species B, which undergoes migratory insertion of the alkenyl group into the carbonyl group, affording a Co(III) alkoxide species C. Protonolysis of C by HOAc regenerates the active catalyst and releases the final product. The beneficial effect of Zn(OAc)2 may originate from its role in enhancing the electrophilicity of the ester moiety though coordination with zinc(II), which facilitates insertion of the vinyl-Co bond into the carbonyl group.
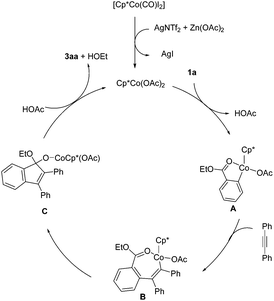 |
| Scheme 6 Proposed mechanism. | |
Conclusions
In summary, a Cp*Co(III)-catalyzed annulation between benzoates and alkynes has been developed under redox-neutral conditions, which expands the applications of high valent cobalt(III) catalysts in the synthesis of diverse compounds. Starting from commercially available benzoates and their derivatives, a range of functionalized indenones were constructed with this protocol. Moreover, this catalytic system is highly efficient over a broad range of substrates with good functional group tolerance.
Acknowledgements
Financial support from the NSFC (no. 21525208) is gratefully acknowledged.
Notes and references
- For a recent review on transition-metal-catalyzed direct C–H functionalization, see:
(a) K. M. Engle, T.-S. Mei, M. Wasa and J.-Q. Yu, Acc. Chem. Res., 2012, 45, 788 CrossRef CAS PubMed;
(b) S. H. Cho, J. Y. Kim, J. Kwak and S. Chang, Chem. Soc. Rev., 2011, 40, 5068 RSC;
(c) T. Satoh and M. Miura, Chem. – Eur. J., 2010, 16, 11212 CrossRef CAS PubMed;
(d) L. Ackermann, Acc. Chem. Res., 2014, 47, 281 CrossRef CAS PubMed;
(e) X.-S. Zhang, K. Chen and Z.-J. Shi, Chem. Sci., 2014, 5, 2146 RSC;
(f) L. McMurray, F. O'Hara and M. J. Gaunt, Chem. Soc. Rev., 2011, 40, 1885 RSC;
(g) C. S. Yeung and V. M. Dong, Chem. Rev., 2011, 111, 1215 CrossRef CAS PubMed;
(h) Z. Chen, B. Wang, J. Zhang, W. Yu, Z. Liu and Y. Zhang, Org. Chem. Front., 2015, 2, 1107 RSC;
(i) J. Yamaguchi, A. D. Yamaguchi and K. Itami, Angew. Chem., Int. Ed., 2012, 51, 8960 CrossRef CAS PubMed;
(j) J. F. Hartwig, Acc. Chem. Res., 2012, 45, 864 CrossRef CAS PubMed;
(k) S. R. Neufeldt and M. S. Sanford, Acc. Chem. Res., 2012, 45, 936 CrossRef CAS PubMed;
(l) T. Brückl, R. D. Baxter, Y. Ishihara and P. S. Baran, Acc. Chem. Res., 2012, 45, 826 CrossRef PubMed.
- M. Moselage, J. Li and L. Ackermann, ACS Catal., 2016, 6, 498 CrossRef CAS.
-
(a) T. Yoshino, H. Ikemoto, S. Matsunaga and M. Kanai, Angew. Chem., Int. Ed., 2013, 52, 2207 CrossRef CAS PubMed;
(b) B. Sun, T. Yoshino, S. Matsunaga and M. Kanai, Adv. Synth. Catal., 2014, 356, 1491 CrossRef CAS;
(c) Y. Suzuki, B. Sun, K. Sakata, T. Yoshino, S. Matsunaga and M. Kanai, Angew. Chem., Int. Ed., 2015, 54, 9944 CrossRef CAS PubMed;
(d) B. Sun, T. Yoshino, S. Matsunaga and M. Kanai, Chem. Commun., 2015, 51, 4659 RSC.
-
(a) T. Gensch, S. Vásquez-Céspedes, D.-G. Yu and F. Glorius, Org. Lett., 2015, 17, 3714 CrossRef CAS PubMed;
(b)
J. H. Kim, S. Greßies and F. Glorius, Angew. Chem., Int. Ed., 2016, 55, 5577 Search PubMed;
(c) D.-G. Yu, T. Gensch, F. de Azambuja, S. Vásquez-Céspedes and F. Glorius, J. Am. Chem. Soc., 2014, 136, 17722 CrossRef CAS PubMed;
(d) D. Zhao, J. H. Kim, L. Stegemann, C. A. Strassert and F. Glorius, Angew. Chem., Int. Ed., 2015, 54, 4508 CrossRef CAS PubMed.
-
(a) N. Sauermann, M. J. González and L. Ackermann, Org. Lett., 2015, 17, 5316 CrossRef CAS PubMed;
(b) J. Li and L. Ackermann, Angew. Chem., Int. Ed., 2015, 54, 8551 CrossRef CAS PubMed;
(c) J. Li and L. Ackermann, Angew. Chem., Int. Ed., 2015, 54, 3635 CrossRef CAS PubMed.
-
(a) J. R. Hummel and J. A. Ellman, Org. Lett., 2015, 17, 2400 CrossRef CAS PubMed.
-
(a) L. Grigorjeva and O. Daugulis, Angew. Chem., Int. Ed., 2014, 53, 10209 CrossRef CAS PubMed;
(b) L. Grigorjeva and O. Daugulis, Org. Lett., 2015, 17, 1204 CrossRef CAS PubMed.
-
(a) A. B. Pawar and S. Chang, Org. Lett., 2015, 17, 660 CrossRef CAS PubMed;
(b) J. Park and S. Chang, Angew. Chem., Int. Ed., 2015, 54, 14103 CrossRef CAS PubMed;
(c) P. Patel and S. Chang, ACS Catal., 2015, 5, 853 CrossRef CAS.
-
(a) Z.-Z. Zhang, B. Liu, C.-Y. Wang and B.-F. Shi, Org. Lett., 2015, 17, 4094 CrossRef CAS PubMed;
(b) X.-G. Liu, S.-S. Zhang, J.-Q. Wu, Q. Li and H. Wang, Tetrahedron Lett., 2015, 56, 4093 CrossRef CAS;
(c) X.-G. Liu, S.-S. Zhang, C.-Y. Jiang, J.-Q. Wu, Q. Li and H. Wang, Org. Lett., 2015, 17, 5404 CrossRef CAS PubMed;
(d) S.-S. Zhang, X.-G. Liu, S.-Y. Chen, D.-H. Tan, C.-Y. Jiang, J.-Q. Wu, Q. Li and H. Wang, Adv. Synth. Catal. DOI:10.1002/adsc.201600025.
- Reviews on Rh-catalyzed C–H activation:
(a) N. Kuhl, N. Schröder and F. Glorius, Adv. Synth. Catal., 2014, 356, 1443 CrossRef CAS;
(b) G. Song, F. Wang and X. Li, Chem. Soc. Rev., 2012, 41, 3651 RSC;
(c) G. Song and X. Li, Acc. Chem. Res., 2015, 48, 1007 CrossRef CAS PubMed;
(d) D. A. Colby, A. S. Tsai, R. G. Bergman and J. A. Ellman, Acc. Chem. Res., 2012, 45, 814 CrossRef CAS PubMed;
(e) D. A. Colby, R. G. Bergman and J. A. Ellman, Chem. Rev., 2010, 110, 624 CrossRef CAS PubMed.
- For selected examples, see:
(a) H. Ikemoto, T. Yoshino, K. Sakata, S. Matsunaga and M. Kanai, J. Am. Chem. Soc., 2014, 136, 5424 CrossRef CAS PubMed;
(b) B. Sun, T. Yoshino, M. Kanai and S. Matsunaga, Angew. Chem., Int. Ed., 2015, 54, 12968 CrossRef CAS PubMed;
(c) A. Lerchen, S. Vásquez-Céspedes and F. Glorius, Angew. Chem., Int. Ed., 2016, 55, 3208 CrossRef CAS PubMed;
(d) Q. Lu, S. Vásquez-Céspedes, T. Gensch and F. Glorius, ACS Catal., 2016, 6, 2352 CrossRef CAS;
(e) L. Kong, S. Yu, X. Zhou and X. Li, Org. Lett., 2016, 18, 588 CrossRef CAS PubMed;
(f) F. Wang, H. Wang, Q. Wang, S. Yu and X. Li, Org. Lett., 2016, 18, 1306 CrossRef CAS PubMed;
(g) H. Wang, J. Koeller, W. Liu and L. Ackermann, Chem. – Eur. J., 2015, 21, 15525 CrossRef CAS PubMed;
(h) H. Wang, M. Moselage, M. J. González and L. Ackermann, ACS Catal., 2016, 6, 2705 CrossRef CAS;
(i) J. R. Hummel and J. A. Ellman, J. Am. Chem. Soc., 2015, 137, 490 CrossRef CAS PubMed;
(j) Y. Liang, Y.-F. Liang, C. Tang, Y. Yuan and N. Jiao, Chem. – Eur. J., 2015, 21, 16395 CrossRef CAS PubMed;
(k) Y. Liang and N. Jiao, Angew. Chem., Int. Ed., 2016, 55, 4035 CrossRef CAS PubMed;
(l) M. Sen, D. Kalsi and B. Sundararaju, Chem. – Eur. J., 2015, 21, 15529 CrossRef CAS PubMed.
-
(a) J. L. Jeffrey and R. Sarpong, Org. Lett., 2009, 11, 5450 CrossRef CAS PubMed;
(b) R. E. McDevitt, M. S. Malamas, E. S. Manas, R. J. Unwalla, Z. B. Xu, C. P. Miller and H. A. Harris, Bioorg. Med. Chem. Lett., 2005, 15, 3137 CrossRef CAS PubMed;
(c) W. M. Clark, A. M. Tickner-Eldridge, G. K. Huang, L. N. Pridgen, M. A. Olsen, R. J. Mills, I. Lantos and N. H. Baine, J. Am. Chem. Soc., 1998, 120, 45 Search PubMed;
(d) E. V. Banide, C. O'Connor, N. Fortune, Y. Ortin, S. Milosevic, H. Muller-Bunz and M. J. McGlinchey, Org. Biomol. Chem., 2010, 8, 3997 RSC.
-
(a) Y. Harada, J. Nakanishi, H. Fujihara, M. Tobisu, Y. Fukumoto and N. Chatani, J. Am. Chem. Soc., 2007, 129, 5766 CrossRef CAS PubMed;
(b) H. Tsukamoto and Y. Kondo, Org. Lett., 2007, 9, 4227 CrossRef CAS PubMed;
(c) R. C. Larock, M. J. Doty and S. Cacchi, J. Org. Chem., 1993, 58, 4579 CrossRef CAS.
- K. Kokubo, K. Matsumasa, M. Miura and M. Nomura, J. Org. Chem., 1996, 61, 6941 CrossRef CAS PubMed.
- S. Chen, J. Yu, Y. Jiang, F. Chen and J. Cheng, Org. Lett., 2013, 15, 4754 CrossRef CAS PubMed.
-
(a) P. Zhao, F. Wang, K. Han and X. Li, Org. Lett., 2012, 14, 5506 CrossRef CAS PubMed;
(b) B.-J. Li, H.-Y. Wang, Q.-L. Zhu and Z.-J. Shi, Angew. Chem., Int. Ed., 2012, 51, 3948 CrossRef CAS PubMed.
- Z. Qi, M. Wang and X. Li, Org. Lett., 2013, 15, 5440 CrossRef CAS PubMed.
-
(a) E. Clot, C. Mégret, O. Eisenstein and R. N. Perutz, J. Am. Chem. Soc., 2009, 131, 7817 CrossRef CAS PubMed;
(b) B.-H. Tan, J. Dong and N. Yoshikai, Angew. Chem., Int. Ed., 2012, 51, 9610 CrossRef CAS PubMed.
Footnote |
† Electronic supplementary information (ESI) available: Experimental procedures and characterization data for new compounds. See DOI: 10.1039/c6qo00134c |
|
This journal is © the Partner Organisations 2016 |
Click here to see how this site uses Cookies. View our privacy policy here.