DOI:
10.1039/C4QI00100A
(Research Article)
Inorg. Chem. Front., 2014,
1, 673-681
In situ synthesis and structural characterization of a series of acylhydrazidate-extended Ln3+ and Zn2+ coordination polymers†
Received
22nd July 2014
, Accepted 20th August 2014
First published on 20th August 2014
Abstract
By utilizing the in situ hydrothermal acylation of N2H4 with aromatic polycarboxylic acids, a series of new acylhydrazidate-extended two-dimensional (2-D) or three-dimensional (3-D) coordination polymers, [Ln(epdh)3(H2O)] (Ln3+ = Dy3+1, Eu3+2, Tb3+3, Gd3+4, Y3+5, Lu3+6; epdh = 5-ethylpyridine-2,3-dicarboxylhydrazidate), [Dy(ox)(pdh)(H2O)] (ox = oxalate, pdh = pyridine-2,3-dicarboxylhydrazidate) 7, [Zn2(mpth)(atez)2] (mpth = 4-methylphthalhydrazidate, atez = 5-aminotetrazolate) 8 and [Zn3(OH)2(Hpth)2(atrz)2] (pth = phthalhydrazidate, atrz = 3-amino-1,2,4-triazolate) 9 were isolated. X-ray single-crystal diffraction revealed that (i) the isomorphic compounds 1–6 posses 2-D layer structures with (4,4) topologies. Two types of epdh molecules act as the rod-like connectors; (ii) compound 7 also possesses a 2-D layer structure, but shows a (6,3) topology. The pdh molecules first link the Dy3+ ions into a dimer. The ox molecules further extend the dimers into this 2-D layer network; (iii) compound 8 has a 3-D network structure (symbol: (4·64·8)2(42·62·82)), which is constructed from the one-dimensional (1-D) Zn2(mpth)2+ chains by the atez molecules; (iv) the 2-D layer network of compound 9 also shows a (6,3) topology, but the geometrical form is different from that of compound 7. Only the atrz molecule serves as the linker in compound 9; (v) via the N–H⋯O and/or N–H⋯N interactions, compounds 1–6, 7 and 9 self-assemble into the different 3-D supramolecular networks. Photoluminescence analysis revealed that (i) compounds 2 and 3 exhibit the characteristic transitions of the Eu3+ or Tb3+ ion; (ii) compounds 1, 4, 5 and 6 emit green light, suggesting their behaviors are dominated by the epdh molecules; (iii) compound 8 emits violet light, whereas compound 9 does not emit light.
Introduction
Over the last two decades, considerable attention has been paid to the design and synthesis of novel coordination polymers (CPs) or metal organic frameworks (MOFs) due to their structural diversity1 and potential applications in such fields as adsorption,2 optics,3 magnetism4 and catalysis.5 For example, the porous MOFs can selectively adsorb gases such as H2, N2, CO2, H2O, CH4, C2H6 and C3H8. The pore size can be tuned through changing the lengths and shapes of the ligands.6 Ligand-sensitized Ln-CPs or Ln-MOFs emit light with different colors. The organic ligands act as the chromophores7 and some CPs or MOFs can serve as luminescence probes to sense organic molecules, especially toxic substances or explosives.8 N-heterocyclic molecules and polycarboxylic acid molecules have been widely employed in the construction of novel CPs, especially the porous MOFs.9 Although our group has reported a series of acylhydrazidate-extended CPs in the last three years,10 the related studies are still limited.11 As shown in Scheme 1, the acylhydrazide molecule is derived from the in situ hydrothermal acylation of N2H4 with an aromatic polycarboxylic acid. The two carboxyl groups in each phthalic acid moiety adopt a trans arrangement, but after being acylated by N2H4, the four donor atoms in each phthalhydrazidate moiety are nearly coplanar. Therefore, the coordination behavior of the phthalhydrazidate moiety in a complex is completely different from that of phthalic acid. The acylhydrazidate molecules can exhibit multiple coordination modes upon interaction with metal centers. In particular, when two acylamino groups or two acylamino O atoms act as the donors, the acylhydrazidate molecule can be regarded as a rod-like connector. Rod-like molecules have the potential to link the metal centers into an extended network. Recently, our group has reported some acylhydrazidate-extended transition metal (Mn2+, Co2+, Cu+, Zn2+, Cd2+) and main-group metal (Pb2+, Ba2+) CPs. However, the acylhydrazidate-propagated rare-earth metal CPs and their properties have been less well investigated. These studies indicate that the monoacylhydrazidate molecule is prone to forming oligomers with metal ions. In order to construct acylhydrazidate-extended MOFs, we have attempted to introduce a second linker into the metal–monoacylhydrazidate system. The generation of three-dimensional (3-D) MOFs [Zn2(pdh)2(ox)]·H2O (pdh = pyridine-2,3-dicarboxylhydrazidate, ox = oxalate),10b [Zn2(pth)(atez)2] (pth = phthalhydrazidate, atez = 5-aminotetrazolate),10d [Zn2(3-apth)(atrz)2] (3-apth = 3-aminophthalhydrazidate; atrz = 3-amino-1,2,4-triazole) and [Zn2(4-apth)(atez)2] (4-atez = 4-aminophthalhydrazidate)10c suggests this strategy is feasible. Small organic molecules can be used as the second connector. In this article, rare-earth metal salts were selected as the metal precursors. Meanwhile, we continued trying to incorporate a second linker into the metal–monoacylhydrazidate system. A series of new acylhydrazidate-bridged coordination polymers, [Ln(epdh)3(H2O)] (Ln3+ = Dy3+1, Eu3+2, Tb3+3, Gd3+4, Y3+5, Lu3+6; epdh = 5-ethylpyridine-2,3-dicarboxylhydrazidate), [Dy(ox)(pdh)(H2O)] 7, [Zn2(mpth)(atez)2] (mpth = 4-methylphthalhydrazidate) 8 and [Zn3(OH)2(Hpth)2(atrz)2] 9 were obtained. Their thermal stabilities and photoluminescence and adsorption properties were investigated (Scheme 2).
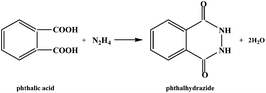 |
| Scheme 1 Acylation of N2H4 with phthalic acid. | |
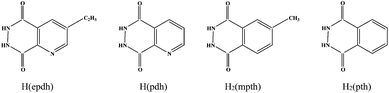 |
| Scheme 2 Structures of acylhydrazide molecules in title compounds. | |
Experimental
Materials and physical measurements
All chemicals were of reagent grade quality, obtained from commercial sources without further purification. Elemental analysis was performed on a Perkin-Elmer 2400LS II elemental analyzer. Infrared (IR) spectra were recorded on a Perkin Elmer Spectrum 1 spectrophotometer in the 4000–400 cm−1 range using a powdered sample in a KBr plate. Powder X-ray diffraction (XRD) data were collected on a Rigaku/max-2550 diffractometer with Cu-Kα radiation (λ = 1.5418 Å). Thermogravimetric (TG) behavior was investigated on a Perkin-Elmer TGA-7 instrument with a heating rate of 10 °C min−1 in air. Fluorescence spectra were obtained on a LS 55 fluorescence/phosphorescence spectrophotometer at room temperature. The measurement of N2 adsorption was carried out on an ASAP-2010 apparatus.
Synthesis of the title compounds
[Ln(epdh)3(H2O)] (Ln3+ = Dy3+1, Eu3+2, Tb3+3, Gd3+4, Y3+5, Lu3+6).
The yellow block crystals of 1 were obtained from a simple hydrothermal self-assembly of Dy(NO3)3 (114 mg, 0.25 mmol), 5-ethylpyridine-2,3-dicarboxylic acid (epdca) (98 mg, 0.5 mmol) and N2H4·H2O (0.14 mL) in a 10 mL aqueous solution (pH = 7, adjusted by N2H4) at 160 °C for 4 days. Yield: ca. 30% based on Dy(III). Anal. calcd (%) C27H26N9O7Dy 1: C 43.17, H 3.49, N 16.79. Found: C 43.18, H 3.49, N 17.36. IR (cm−1): 1655 s, 1580 s, 1538 s, 1489 s, 1383 m, 1262 m, 1188 s, 1099 w, 922 m, 812 s, 665 m, 538 w. The crystals of 2–6 were obtained from a similar process to that of 1.
[Dy(ox)(pdh)(H2O)] 7.
The yellow columnar crystals of 7 were obtained from a simple hydrothermal self-assembly of Dy(NO3)3 (114 mg, 0.25 mmol), pyridine-2,3-dicarboxylic acid (pdca) (84 mg, 0.5 mmol), H2(ox) (32 mg, 0.25 mmol) and N2H4·H2O (0.14 mL) in a 10 mL aqueous solution (pH = 6, adjusted by H2(ox)) at 160 °C for 4 days. Yield: ca. 15% based on Dy(III). Anal. calcd (%) C9H6N3O7Dy 7: C 25.10, H 1.40, N 9.76. Found: C 24.91, H 1.44, N 10.39. IR (cm−1): 1663 w, 1639 s, 1583 w, 1481 s, 1390 m, 1351 m, 1310 m, 1218 m, 1114 s, 834 w, 802 s, 647 w, 489 w.
[Zn2(mpth)(atez)2] 8.
The yellow block crystals of 8 were obtained from a simple hydrothermal self-assembly of Zn(CH3COO)2·2H2O (110 mg, 0.5 mmol), 4-methylphthalic acid (mpha) (90 mg, 0.5 mmol), H(atez) (51 mg, 0.25 mmol) and N2H4·H2O (0.2 mL) in a 15 mL aqueous solution (pH = 8, adjusted by N2H4) at 170 °C for 4 days. Yield: ca. 20% based on Zn(II). Anal. calcd (%) C11H9N12O2Zn28: C 27.99, H 1.92, N 35.62. Found: C 28.14, H 2.05, N 36.18. IR (cm−1): 1644 s, 1559 s, 1448 s, 1385 w, 1320 w, 1229 w, 1094 m, 1084 s, 999 m, 872 m, 742 m, 502 w.
[Zn3(OH)2(pth)2(atrz)2] 9.
The yellow block crystals of 9 were obtained from a simple hydrothermal self-assembly of Zn(CH3COO)2·2H2O (110 mg, 0.5 mmol), phthalic acid (pha) (83 mg, 0.5 mmol), H(atrz) (42 mg, 0.5 mmol) and N2H4·H2O (0.2 mL) in a 15 mL aqueous solution (pH = 8, adjusted by N2H4) at 170 °C for 4 days. Yield: ca. 20% based on Zn(II). Anal. calcd (%) C20H16N12O6Zn39: C 33.52, H 2.25, N 23.46. Found: C 33.42, H 2.47, N 23.38. IR (cm−1): 1659 s, 1620 s, 1586 s, 1515 s, 1396 s, 1384 s, 1215 m, 1162 m, 1058 m, 867 w, 786 w, 697 m.
X-ray crystallography
The data were collected with Mo-Kα radiation (λ = 0.71073 Å) on a Siemens SMART CCD diffractometer for compounds 1, 7 and 8, and on a Rigaku R-AXIS RAPID IP diffractometer for compound 9. With the SHELXTL program, the structures of compounds 1 and 7–9 were solved using direct methods.12 The non-hydrogen atoms were assigned anisotropic displacement parameters in the refinement. The H atoms on the water molecule in compound 7, and on C3 in compound 8 were obtained from the difference Fourier maps. The H atoms on the water molecule in compound 1 were not located. The other H atoms were treated using a riding model. The structures were then refined on F2 using SHELXL-97.12 CCDC numbers are 1015188–1015191 for 1 and 7–9, respectively. The crystallographic data for the title compounds are summarized in Table 1.
Table 1 Crystallographic data for 1 and 7–9
|
1
|
7
|
8
|
9
|
Formula |
C27H26N9O7Dy |
C9H6N3O7Dy |
C11H9N12O2Zn2 |
C20H16N12O6Zn3 |
M
|
751.07 |
430.67 |
472.04 |
716.56 |
T (K) |
293(2) |
293(2) |
293(2) |
293(2) |
Crystal system |
Monoclinic |
Triclinic |
Monoclinic |
Monoclinic |
Space group |
C2/c |
P![[1 with combining macron]](https://www.rsc.org/images/entities/char_0031_0304.gif) |
C2/c |
C2/c |
a (Å) |
39.586(3) |
7.1376(4) |
12.3935(6) |
14.175(3) |
b (Å) |
9.0348(5) |
7.8130(4) |
13.3918(7) |
8.9672(18) |
c (Å) |
18.2097(11) |
10.3661(7) |
10.3258(6) |
20.104(4) |
α (°) |
|
90.681(4) |
|
|
β (°) |
116.851(4) |
103.088(4) |
117.390(3) |
93.01(3) |
γ (°) |
|
101.710(3) |
|
|
V (Å3) |
5810.5(6) |
550.33(6) |
1970.8(2) |
2552.0(9) |
Z
|
8 |
2 |
4 |
4 |
D
c (g cm−3) |
1.717 |
2.599 |
2.060 |
1.865 |
μ (mm−1) |
2.634 |
6.830 |
3.194 |
2.861 |
Reflections collected |
15 947 |
3129 |
4199 |
12 106 |
Unique reflections |
5117 |
1949 |
1352 |
2907 |
R
int
|
0.0429 |
0.0247 |
0.0276 |
0.0850 |
Gof |
1.186 |
1.178 |
1.047 |
1.044 |
R
1, I > 2σ(I) |
0.0386 |
0.0211 |
0.0214 |
0.0499 |
wR2, all data |
0.1068 |
0.0584 |
0.0565 |
0.1372 |
Results and discussion
Synthetic analysis
All of the title compounds were obtained under hydrothermal conditions. The acylhydrazide molecules appearing in the title compounds originated from the in situ acylation of N2H4 with aromatic polycarboxylic acids. The reaction of Dy3+, epdca and N2H4 yielded compound 1. Using the other Ln3+ ions Eu3+, Tb3+, Gd3+, Y3+, or Lu3+ instead of Dy3+, compounds 2–6 were isolated. The IR spectra revealed that compounds 2–6 are isostructural to that of 1. On incorporation of ox into the reaction system mentioned above, the target product was not obtained. Changing epdca to pdca, the reaction of Dy3+, pdca and N2H4 produced a mononuclear compound [Dy(pdh)3(H2O)3]·H2O, which has been reported.11a Mixing ox into this reaction afforded compound 7. A series of reactions of Ln3+, mpdca (6-methylpyridine-2,3-dicarboxylic acid) and N2H4 with or without ox was also carried out, but no crystalline solids were obtained. Moreover, we have successfully introduced trz, datrz and atez into the Zn2+-pth system, giving compounds [Zn5(OH)2(Hpth)2(pth)2(trz)2] (trz = 1,2,4-triazole; 2-D), [Zn3(OH)2(Hpth)2(datrz)2] (datrz = 3,5-diamino-1,2,4-triazolate; 2-D) and [Zn2(pth)(atez)2] (3-D).11d In this article, atez was incorporated into the Zn2+-mpth system to give compound 8 and atrz was mixed into the Zn2+-pth system to produce compound 9. In the reactions, N2H4 was largely in excess in order to ensure the carboxyl groups were thoroughly acylated. The single-crystal growth for compounds 1–9 was strictly controlled by adjusting the pH levels of the systems: pH 7 for 1–6, pH 6 for 7 and pH 8 for 8 and 9. The dilute N2H4 and H2(ox) solutions were used to adjust the pH levels of the reactions.
Structural description
[Ln(epdh)3(H2O)] (Ln3+ = Dy3+1, Eu3+2, Tb3+3, Gd3+4, Y3+5, Lu3+6).
Compounds 1–6 are isomorphic, so only compound 1, as the representative, was structurally characterized. X-ray single-crystal diffraction revealed that compound 1 is an epdh-propagated 2-D layered Dy3+ coordination polymer. It crystallizes in the space group C2/c, and the asymmetric unit is found to be composed of one Dy3+ ion (Dy1), three types of epdh molecules (epdh I, epdh II, epdh III) and one coordinated water molecule (Ow1). One C–O distance is obviously longer than the other, indicating that the three epdh molecules in compound 1 all exist in the keto-hydroxyl form. Each has a −1 charge. As shown in Scheme 3, epdh I adopts a simple coordination mode: the pyridyl N atom together with the adjacent hydroxylimino O atom chelates to one Dy3+ center. Epdh II and epdh III are involved in similar double-bridged coordination modes. Besides the chelation of the pyridyl N atom and the hydroxylimino/acylamino O atom, the remaining hydroxylimino/acylamino O atom further bonds to one Dy3+ center. Bridged by epdh molecules, compound 1 exhibits a 2-D layer structure (see Fig. 1a). Edph I has no contribution to the formation of the layer structure. Epdh II and epdh III link the Dy3+ ions into this 2-D layer. Along the direction of the c-axis, the epdh II molecules link the Dy3+ ions into an infinite 1-D chain. Then along the b-axis the epdh III molecules extend the chains further into the 2-D layer network of compound 1. Based on the topological viewpoint,13 both epdh II and epdh III can be viewed as the linkers, and compound 1 has a (4,4) topology. Fig. 1b is the projection of compound 1 in the (010) direction. Two types of hydrogen-bonded synthons are observed between the layers. Via N–H⋯N interactions (N4⋯N5c = 2.929(8) Å), two neighboring hydrazino groups from epdh II molecules form a hydrogen-bonded dimer (R22(4)), while via N–H⋯O interactions (N2⋯O2d = 2.866(9) Å), two adjacent acylamino groups from epdh I molecules form another kind of hydrogen-bonded dimer (R22(8)). Via these hydrogen-bonding interactions, compound 1 self-assembles into a 3-D supramolecular network. Dy1 is in an eight-coordinate site, surrounded by four hydroxylimino/acylamino O atoms (O3, O5, O4a, O6b), three pyridyl N atoms (N3, N6, N9b) and one water molecule (Ow1) (see Fig. 1a, right). The Dy–O and Dy–N bond lengths range from 2.286(4)–2.455(4) Å and 2.610(5)–2.712(5) Å, respectively.
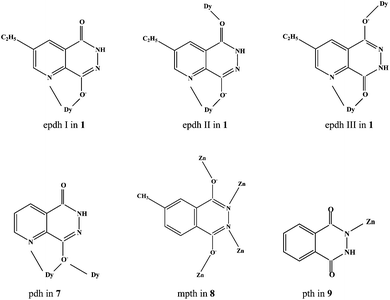 |
| Scheme 3 Coordination modes of acylhydrazidate molecules in title compounds. | |
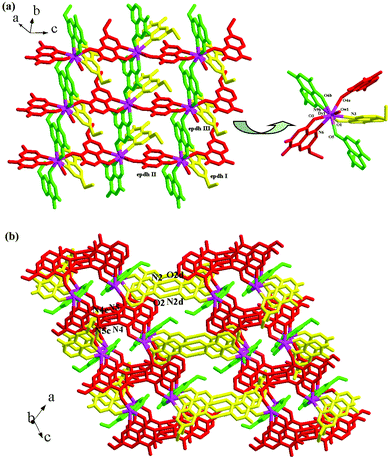 |
| Fig. 1 (a) 2-D layer and (b) 3-D supramolecular network of 1 (a: x, −y + 2, z − 1/2; b: x, y − 1, z; c: −x + 1, y, −z + 3/2; d: −x + 1/2, −y + 5/2, −z). | |
[Dy(ox)(pdh)(H2O)] 7.
Compound 7 is another 2-D layered Dy3+ coordination polymer bridged by pdh and ox ligands. It crystallizes in the space group P
, and the asymmetric unit is found to be composed of one Dy3+ ion (Dy1), one pdh molecule, one ox molecule and one coordinated water molecule (Ow1). Pdh in compound 7 exists in the keto-hydroxyl form, which is revealed by the different C–O distances (1.309(5) Å for C1–O6, 1.260(5) Å for C2–O7). It has a −1 charge. As shown in Scheme 3, pdh adopts a μ2 coordination mode. Chelation of the pyridyl N atom and the hydroxylimino O atom to the Dy3+ ion is also observed. The difference in this compound is that the hydroxylimino O atom further interacts with another Dy3+ center. Extended by ox and pdh, compound 7 exhibits a 2-D layer network (see Fig. 2a). Two pdh molecules alternately link two Dy3+ ions with their hydroxylimino O atoms to form a dimer (see Fig. 2a, top right). The Dy2O2 unit is planar, and the Dy⋯Dy contact is 3.9372(4) Å. Then the ox linkers propagate these dimers to form the 2-D layer network of compound 7. The bridging mode of the ox molecule is common. Based on the topological method, this 2-D layer network possesses a (6,3) topology (see Fig. 2a, bottom right).13 The acylamino group of the pdh molecule is non-coordinate. Similar to the situation observed in compound 1, the neighboring acylamino groups form the hydrogen-bonded dimer via N–H⋯O interactions (N3⋯O2d = 2.851(5) Å). Via this kind of hydrogen-bonded synthon, compound 7 self-assembles into a 3-D supramolecular network (see Fig. 2b). The 8-coordinate Dy1 center bonds to two hydroxylimino O atoms (O1, O1b), one pyridyl N atom (N1), four carboxyl O atoms (O3, O5, O4a, O6c) and one water molecule (Ow1). The Dy–O bond length range of 2.334(3)–2.430(4) Å and the Dy–N distance of 2.575(3) Å are basically in agreement with those found in compound 1.
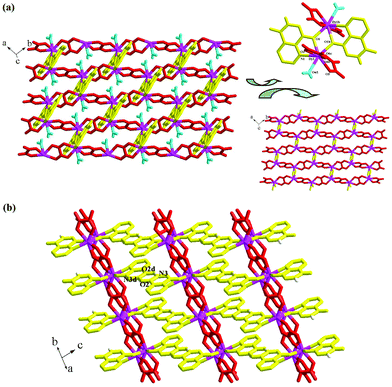 |
| Fig. 2 (a) 2-D layer and (b) 3-D supramolecular network for 7 (a: −x − 2, −y − 2, −z + 2; b: −x − 1, −y − 2, −z + 2; c: −x − 1, −y − 3, −z + 2; d: −x − 1, −y − 1, −z + 1). | |
[Zn2(mpth)(atez)2] 8.
Compound 8 is a new 3-D Zn(II) MOF propagated by mpth and atez ligands. It crystallizes in the space group C2/c, and the asymmetric unit is found to be composed of one Zn2+ ion (Zn1), 0.5 mpth molecules and one atez molecule. The C–O distance of 1.301(3) Å indicates that mpth exists in the dihydroxyl form. Mpth in compound 8 has a −2 charge. As shown in Scheme 3, mpth is involved in a tetra-bridged coordination mode: each donor atom monodentately coordinates to one Zn2+ ion. As displayed in Fig. 3a (right), the μ4 mpth molecules, with two hydroxylimino groups as the donors, link the Zn2+ ions into an infinite 1-D chain, running along the c-axis. The Zn1 center in the chain is in a tetrahedral site. Two positions are occupied by the hydroxylimino N and O atoms (N1, O1a), while the remaining two positions are occupied by the atez N atoms (N5, N2b). The atez molecule adopts a double-bridged mode, utilizing the 1- and 4-position N atoms to act as the donors. As shown in Fig. 3 (left), via the linkage of atez, each Zn2(mpth)2+ chain interacts with the adjacent four Zn2(mpth)2+ chains. In this way, the atez molecules extend the Zn2(mpth)2+ chains into the 3-D network of compound 8. A 1-D channel of ca. 7 × 3.5 Å2 in size is observed. Based on the topological method, the Zn2+ center can be regarded as a 4-connected node with a tetrahedral geometry, the mpth molecule can be considered as a planar 4-connected node, and the double-bridged atez molecule acts as the linker. Compound 8 exhibits a 3-D (4,4)-connected net with a symbol (4·64·8)2(42·62·82). The Zn–O and Zn–N bond lengths are 1.9828(17) Å and 1.979(3)–2.003(3) Å, respectively. The shortest Zn⋯Zn contact in compound 8 is Zn1⋯Zn1a = 3.765 Å.
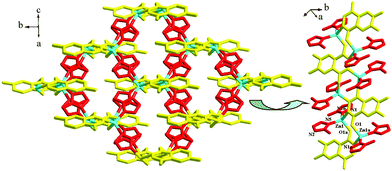 |
| Fig. 3 3-D network of 8 (a: −x + 1/2, −y + 1/2, −z + 2; b: x, −y + 1, z − 1/2). | |
[Zn3(OH)2(Hpth)2(atrz)2] 9.
Compound 9 is an atrz-extended 2-D layered Zn2+ coordination polymer. It crystallizes in the space group C2/c and the asymmetric unit is found to be composed of two types of Zn2+ ions (Zn1, Zn2; occupancy: 1 for Zn1, 0.5 for Zn2), one hydroxyl group, one pth molecule and one atrz molecule. Two C–O distances of 1.250(5) Å and 1.244(6) Å are smaller than 1.27 Å, indicating that pth exists in the diketo form. As shown in Scheme 3, pth adopts a terminal coordination mode, only using one acylimino N atom to act as the donor. Before coordination, the H atom on this N atom is lost. So pth in compound 9 has a −1 charge. Fig. 4a illustrates the 2-D layer structure of compound 9. OH and pth are not involved in the formation of this layer network. The triple-bridged atrz molecules extend the Zn2+ ions into the 2-D layers of compound 9 (see Fig. 4a, bottom right). The layer is based on 22-membered rings. Each ring consists of six Zn(II) ions and six atrz molecules, arranged in an alternating manner. The 2-D layer network of compound 9 also has a (6,3) topology, but the geometrical form is different from that of compound 7.13Fig. 4b displays the projection diagram of compound 9 in the (010) direction. The pth molecules are distributed on both sides of the layer, and protrude from the layer. Via the π⋯π interactions between the adjacent pth molecules (contact: ca. 3.5 Å), the 2-D layers self-assemble into a 3-D supramolecular network. The neighboring non-coordinated acylamino groups also form hydrogen-bonded dimers (N2⋯O2c = 2.771(5) Å), stabilizing the 3-D supramolecular network of compound 9. Both Zn(II) ions are in tetrahedral sites, but the detailed environments are different (see Fig. 4a, top right). Zn1 is coordinated to one hydroxylimino N atom (N1), two atrz N atoms (N3, N5a) and one hydroxyl group (OH1), whereas Zn2 lies at an inversion center, surrounded by two hydroxyl groups (OH1, OH1b) and two atrz N atoms (N4, N4b). The Zn–N bond lengths of 1.980(3)–2.027(3) Å and the Zn–O bond lengths of 1.956(3)–1.974(3) Å are comparable with those observed in compound 8. The shortest Zn⋯Zn separation is Zn1⋯Zn2 = 3.257 Å.
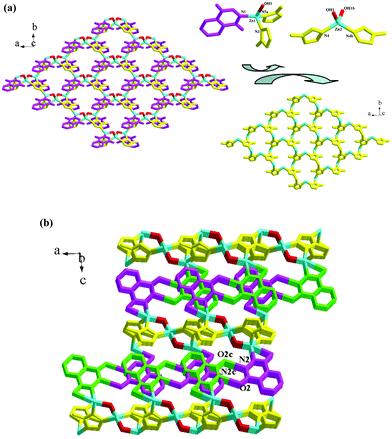 |
| Fig. 4 (a) 2-D layer and (b) 3-D supramolecular network of 9 (a: −x + 1/2, y + 1/2, −z + 1/2; b: −x + 1, y, −z + 1/2; c: −x + 1, −y, −z + 1). | |
Based on the structural information and the relevant reports, we know that (i) the acylhydrazidate molecules have three forms in the complexes. The majority of acylhydrazidate molecules exist in the keto-hydroxyl form. This implies that one acylamino group has isomerized into a hydroxylimino group after acylation. The other acylamino group generally does not isomerize. The keto-hydroxyl form has been theoretically confirmed to be the most stable existing form for the acylhydrazidate molecule.14 In only limited examples, the acylhydrazidate molecules exist in an unstable dihydroxyl or diketo form, as observed in compounds 8 and 9; (ii) the monoacylhydrazidate molecule has a −1 or −2 charge in a complex. Upon interaction with the metal ion, the hydroxyl group deprotonates to balance the metal charge. So the monoacylhydrazidate molecule having the keto-hydroxyl form exhibits a −1 charge, whereas the monoacylhydrazidate molecule having a dihydroxyl form exhibits a −2 charge. The unique exception is the reported compound [Cd2(ox)0.5(Hpth)(pth)(bpy)2] (bpy = 2,2′-bipyridine), in which pth exists in the keto-hydroxyl form, but has a −2 charge.10g In compound 9 the monoacylhydrazidate molecule having the diketo form exhibits a −1 charge; (iii) four donor atoms can all coordinate to the metal center. As shown in Scheme 3, the acylhydrazidate molecules generally utilize the hydroxylimino group and acylamino O atoms to interact with a metal center. No examples show that the acylamino N atom could have the activity to donate to the metal center. Once this acylamino group also isomerizes, this N atom can be activated, as observed in compound 8. The coordination ability of the pyridyl N atom should be greater than that of the hydroxylimino N atom; (iv) the acylhydrazidate molecules can form multiple types of hydrogen-bonded synthons, for example, R22(4) between two hydrozino groups and R22(8) between two acylamino groups. The adjacent hydroxylimino groups can also aggregate to form a hydrogen-bonded synthon, which has been found in the reported compound [bpth]·0.5H2O (bpth = 3,3′-biphthalhydrazide).10e These synthons play a key role in the formation of supramolecular networks; (v) the side group on the organic molecule impacts the resulting framework structure. For instance, the pdh-coordinated Ln3+ compound [Ln(pdh)3(H2O)3]·H2O possesses a mononuclear structure,11a while the epdh-coordinated Ln3+ compound [Ln(epdh)3(H2O)] possesses a 2-D layer structure. The Zn2+ compound bridged by 3-apth and atrz [Zn2(3-apth)(atrz)2] exhibits a 3-D network structure,11c whereas the Zn2+ compound extended by pth and atrz [Zn3(OH)2(Hpth)2(atrz)2] 9 shows a 2-D layer structure.
Characterization
Fig. 5a presents the experimental and simulated powder XRD patterns of compounds 1 and 7–9. The experimental powder XRD pattern for each compound is in accord with the simulated one generated on the basis of structural data, confirming that the as-synthesized product is phase pure. Fig. S1† also gives the IR spectra of compounds 1 and 7–9. The appearance of strong peaks at 1655 cm−1 for 1, 1639 cm−1 for 7, 1644 cm−1 for 8 and 1659 cm−1 for 9 indicate that the acylation of N2H4 with the aromatic polycarboxylic acid has occurred, because the ν(COO) peaks are generally either larger than 1680 cm−1 or smaller than 1610 cm−1, whereas the ν(CONH) peaks appear in the range of 1620–1680 cm−1.15 The TG behaviors of compounds 1, 8 and 9 were investigated. Fig. 5b presents the temperature vs. weight loss curves. Compound 1 underwent two steps of weight loss. The first step of ca. 3.0% should be assigned to the removal of the coordinated water molecule (calcd: 2.4%). In the second step, the epdh molecules decomposed, and the Dy3+ ion synchronously combined with O2. So the resulting residue is Dy2O3 (calcd: 24.8%; found: ca. 25.0%). Compound 8 possesses good thermal stability, and is thermally stable up to ca. 430 °C. Compound 8 also underwent two steps of weight loss. It is difficult to assign these weight loss steps, but the final residue is proven to be ZnO (calcd: 34.5%; found: ca. 34.0%). Compound 9 underwent three steps of weight loss. Ca. 5.0% weight loss for the first step may be assigned to the removal of the substituted –NH2 group on the atrz molecule (calcd: 4.5%). In the second and third steps, the sublimation of atrz and H(pth) occurred. The final remaining compound was ZnO (calcd: 34.1%; found: ca. 34.0%).
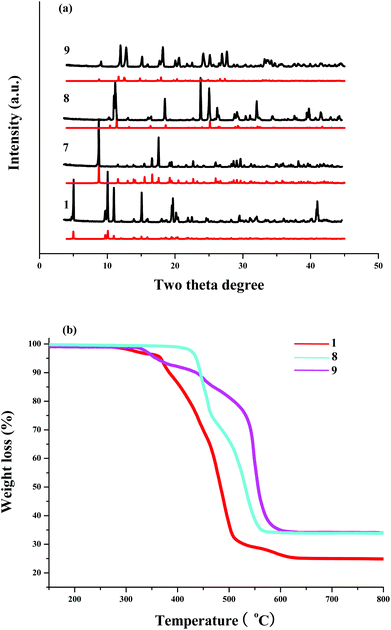 |
| Fig. 5 Powder XRD patterns (a) and TG curves (b) for title compounds. | |
Properties
To assess the porosities of the as-synthesized MOFs, the N2 adsorption isotherm of compound 8 at 77 K was measured. Fig. S2† illustrates the corresponding adsorption–desorption isotherms. The capacity to adsorb N2 at 77 K and 1 bar is only ca. 25.0 cm3 g−1, suggesting that compound 8 is non-porous. A structural change may have occurred during the pre-treatment process. The BET surface area is estimated to be 2.179 m2 g−1, and the measured pore volume is ca. 0.0499 cm3 g−1 for compound 8. The photoluminescence properties of the title compounds were investigated. Fig. 6 illustrates the relevant excitation and emission spectra. As shown in Fig. 6a and 6b, the emissions of compounds 2 and 3 exhibit the characteristic transitions of the rare earth metal ions. No emission resulting from the epdh molecule is observed. As shown in Fig. 6a, the hypersensitive peak at 618 nm is assigned to the 5D0→7F2 transition of the Eu3+ ion, which determines the red light of compound 2. Two medium intensity peaks at 593 nm and 698 nm are attributed to the 5D0→7F1 and 5D0→7F4 transitions of the Eu3+ ion, respectively. The intensity of the electric-dipole-induced 5D0→7F2 transition is obviously higher than that of the magnetic-dipole-induced 5D0→7F1 transition, indicating the absence of inversion symmetry of the Eu3+ ion in compound 2. The peak corresponding to the 5D0→7F3 (653 nm) transition is rather weak, and difficult to observe. As shown in Fig. 6b, the peaks at 491, 542, 584 and 622 nm should be assigned to the transitions from the Tb3+-centered 5D4 excited state to the 7FJ (J = 3, 4, 5 and 6) ground state. The green light of the Tb3+ compound is dominated by the most intense peak at 542 nm. Different from the emissions of the Eu3+ and Tb3+ compounds, the characteristic transitions of 4F9/2→6HJ (J = 11/2, 13/2, 15/2) of the blue-light Dy3+ ion do not appear. A strong and broad green-light emission band centered at 523 nm is observed (see Fig. 6c). The emission of the Dy3+ compound should result from the epdh molecule, because several reported epdh-containing compounds [Pb2(epdh)4(H2O)],10j [Cd(epdh)2(H2O)]10k and [Zn(epdh)2(H2O)2]16 exhibit similar emissions. The related density functional theory (DFT) calculations indicate that the green-light emissions of these compounds originate from charge transfer from the π* orbital of the pyridine ring of the epdh molecule to the π orbital of its acylhydrazidate ring.10f,g,h,j,k The Gd3+, Lu3+ and Y3+ compounds show similar emission behaviors to that of the Dy3+ compound. Fig. 7 shows the emissions of the Dy3+, Gd3+, Lu3+, and Y3+ compounds under the ultraviolet (UV) lamp. The emission intensity of the Dy3+ and Gd3+ compounds are weaker, whereas the Lu3+ and Y3+ compounds emit extremely strong green light (yellow-green for the Y3+ compound; blue-green for the Lu3+ compound). Upon excitation (λex = 343 nm), compound 8 emits violet light with the maximum at 387 nm (see Fig. 6d). Based on our latest report, the violet-light emission of compound 8 should be assigned to a combination of two charge transfer paths: the charge transfer from the benzene ring moiety to the acylhydrazidate ring moiety (path I) and the charge transfer from the tetrazole molecule to the acylhydrazidate ring moiety (path II).10c Compound 9 does not emit light. The acidic protons in the pth molecule (NH proton) may be participating in excited-state proton transfer (ESPT), which would quench any emission processes.17 The photoluminescence properties of the title compounds demonstrate that (i) the epdh molecule can serve as the so-called antenna, effectively transferring the adsorbed energy to the emitting levels of the Eu3+ and Tb3+ ions; (ii) the Ln3+ ion impacts the emission intensity of the Ln3+-epdh compounds; (iii) ESPT maybe leads to luminescence quenching.
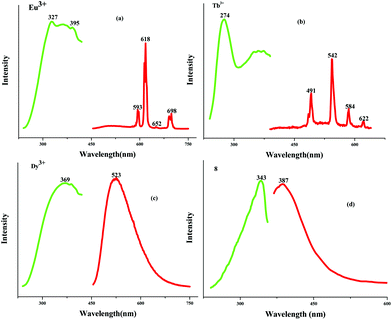 |
| Fig. 6 Photoluminescence spectra of title compounds. | |
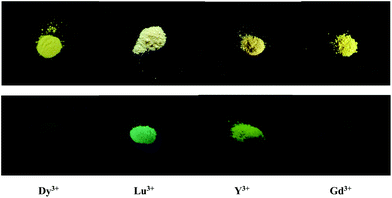 |
| Fig. 7 Bottom images show emissions of Dy3+, Lu3+, Y3+ and Gd3+ compounds under UV lamp (top images are samples for comparison). | |
Conclusion
In summary, we reported the synthesis, structural characterization, and photoluminescence and adsorption properties of several epdh/pdh-extended Ln3+ coordination polymers, and two Zn2+ coordination polymers propagated by mpth/pth and atez/atrz ligands. The acylhydrazidate molecules epdh, pdh, mpth and pth were derived from the in situ hydrothermal acylation of N2H4 with the corresponding polycarboxylic acid molecules. The acylhydrazidate molecules are a new class of bridging ligands. These ligands generally exist in the keto-hydroxyl form in a complex. Occasionally they also take the dihydroxyl or diketo forms, as found in compounds 8 and 9. The hydroxylimino N and O atoms, as well as the acylamino O atom, can all coordinate to the metal center. Especially when two hydroxylimino O atoms act as the donors, the acylhydrazidate molecule can serve as a rod-like linker, as observed in compounds 1 and 8. The acylhydrazidate molecules can link the metal centers into an extended network. The incorporation of bridging organic small molecules is helpful for obtaining multi-dimensional networks. Compound 8 is just one example of a new MOF bridged by mpth and atez. The acylamino N atom does not coordinate to the metal center, but two neighboring hydrazino groups or two neighboring acylamino groups can form hydrogen-bonded synthons, which play a crucial role in the formation of supramolecular networks in compounds 1, 7 and 9. Photoluminescence analysis revealed that the epdh molecule in compounds 2 and 3 acts as the chromophore, making the Eu3+ or Tb3+ compound emit characteristic red or green light, whereas the epdh-extended Dy3+, Gd3+, Lu3+ and Y3+ compounds emit green light, which is dominated by the epdh molecule. Some acylhydrazidate-propagated Zn2+ compounds emit light, while some do not. ESPT possibly quenches any emission in those that do not.
Acknowledgements
This research was supported by the National Natural Science Foundation of China (no. 21271083).
References
-
(a) H. Furukawa, F. Gándara, Y. B. Zhang, J. Jiang, W. L. Queen, M. R. Hudson and O. M. Yaghi, J. Am. Chem. Soc., 2014, 136, 4369 CrossRef CAS PubMed;
(b) K. Hindelang, A. Kronast, S. I. Vagin and B. Rieger, Chem. – Eur. J., 2013, 19, 8244 CrossRef CAS PubMed;
(c) O. Karagiaridi, W. Bury, J. E. Mondloch, J. T. Hupp and O. K. Farha, Angew. Chem., Int. Ed., 2014, 53, 4530 CrossRef CAS PubMed;
(d) Y. Liu, J. R. Li, W. M. Verdegaal, T. F. Liu and H. C. Zhou, Chem. – Eur. J., 2013, 19, 5637 CrossRef CAS PubMed;
(e) P. Cubillas, M. W. Anderson and M. P. Attfield, Chem. – Eur. J., 2013, 19, 8236 CrossRef CAS PubMed;
(f) Q. R. Fang, D. Q. Yuan, J. Sculley, W. G. Lu and H. C. Zhou, Chem. Commun., 2012, 48, 254 RSC;
(g) C. Falaise, C. Volkringer, J. F. Vigier, N. Henry, A. Beaurain and T. Loiseau, Chem. – Eur. J., 2013, 19, 5324 CrossRef CAS PubMed.
-
(a) J. Pang, F. Jiang, M. Wu, D. Yauan, K. Zhou, J. Qian, K. Su and M. Hong, Chem. Commun., 2014, 50, 2834 RSC;
(b) H. Wang, K. Yao, Z. Zhang, J. Jagiello, Q. Gong, Y. Han and J. Li, Chem. Sci., 2014, 5, 620 RSC;
(c) R. J. Li, M. Li, X. P. Zhou, D. Li and M. O'Keeffe, Chem. Commun., 2014, 50, 4047 RSC;
(d) J. Park, D. Yuan, K. T. Pham, J. R. Li, A. Yakovenko and H. C. Zhou, J. Am. Chem. Soc., 2012, 134, 99 CrossRef CAS PubMed;
(e) F. Gándara, H. Furukawa, S. Lee and O. M. Yaghi, J. Am. Chem. Soc., 2014, 136, 5271 CrossRef PubMed;
(f) P. Kanoo, S. K. Reddy, G. Kumari, R. Haldar, C. Narayana, S. Balasubramanian and T. K. Maji, Chem. Commun., 2012, 48, 8487 RSC;
(g) A. Santra, I. Senkovska, S. Kaskel and P. K. Bharadwaj, Inorg. Chem., 2013, 52, 7358 CrossRef CAS PubMed;
(h) P. Shen, W. W. He, D. Y. Du, H. L. Jiang, S. L. Li, Z. L. Lang, Z. M. Su, Q. Fu and Y. Q. Lan, Chem. Sci., 2014, 5, 1368 RSC.
-
(a) A. M. Katzenmeyer, J. Canivet, G. Holland, D. Farrusseng and A. Centrone, Angew. Chem., Int. Ed., 2014, 53, 2852 CrossRef CAS PubMed;
(b) M. E. Foster, J. D. Azoulay, B. M. Wong and M. D. Allendorf, Chem. Sci., 2014, 5, 2081 RSC;
(c) D. Fischer, L. V. Meyer, M. Jansen and K. M. Buschbaum, Angew. Chem., Int. Ed., 2014, 53, 706 CrossRef CAS PubMed;
(d) Q. B. Bo, H. T. Zhang, H. Y. Wang, J. L. Miao and Z. W. Zhang, Chem. – Eur. J., 2014, 20, 3712 CrossRef CAS PubMed;
(e) Q. Zheng, F. Yang, M. Deng, Y. Ling, X. Liu, Z. Chen, Y. Wang, L. Weng and Y. Zhou, Inorg. Chem., 2013, 52, 10368 CrossRef CAS PubMed;
(f) Y. Liu, M. Pan, Q. Y. Yang, L. Fu, K. Li, S. C. Wei and C. Y. Su, Chem. Mater., 2012, 24, 1954 CrossRef CAS;
(g) G. Liu, Y. Qin, L. Jing, G. Wei and H. Li, Chem. Commun., 2013, 49, 1699 RSC;
(h) J. H. Wang, M. Li and D. Li, Chem. Sci., 2013, 4, 1793 RSC.
-
(a) S. Chen, R. Shang, K. L. Hu, Z. M. Wang and S. Gao, Inorg. Chem. Front., 2014, 1, 83 RSC;
(b) W. B. Sun, B. Yan, Y. Q. Zhang, B. W. Wang, Z. M. Wang, J. H. Jia and S. Gao, Inorg. Chem. Front., 2014, 1, 503 RSC;
(c) C. B. Murgui, L. Salmon, A. Akou, N. A. O. Villar, H. J. Shepherd, M. C. Muñoz, G. Molnár, J. A. Real and A. Bousseksou, Chem. – Eur. J., 2012, 18, 507 CrossRef PubMed;
(d) A. F. Cozzolino, C. K. Brozek, R. D. Palmer, J. Yano, M. Li and M. Dincǎ, J. Am. Chem. Soc., 2014, 136, 3334 CrossRef CAS PubMed;
(e) C. K. Brozek and M. Dincǎ, Chem. Sci., 2012, 3, 2110 RSC;
(f) R. J. Holmberg, M. Kay, L. Korobkov, E. Kadantsev, P. G. Boyd, T. Aharen, S. Desgreniers, T. K. Woo and M. Murugesu, Chem. Commun., 2014, 50, 5333 RSC;
(g) E. C. Yang, Y. Y. Zhang, Z. Y. Liu and X. J. Zhao, Inorg. Chem., 2014, 53, 327 CrossRef CAS PubMed;
(h) L. F. Ma, M. L. Han, J. H. Qin, L. Y. Wang and M. Du, Inorg. Chem., 2012, 51, 9431 CrossRef CAS PubMed.
-
(a) Q. Zhang, L. Cao, B. Li and L. Chen, Chem. Sci., 2012, 3, 2708 RSC;
(b) M. J. Beier, W. Kleist, M. T. Wharmby, R. Kissner, B. Kimmerle, P. A. Wright, J. D. Grunwaldt and A. Baiker, Chem. – Eur. J., 2012, 18, 887 CrossRef CAS PubMed;
(c) F. Carson, S. Agrawal, M. Gustafsson, A. Bartoszewicz, F. Moraga, X. Zou and B. M. Matute, Chem. – Eur. J., 2012, 18, 15337 CrossRef CAS PubMed;
(d) G. Q. Kong, S. Ou, C. Zou and C. D. Wu, J. Am. Chem. Soc., 2012, 134, 19851 CrossRef CAS PubMed;
(e) L. Bromberg, Y. Diao, H. Wu, S. A. Speakman and T. A. Hatton, Chem. Mater., 2012, 24, 1664 CrossRef CAS;
(f) L. Meng, Q. Cheng, C. Kim, W. Y. Gao, L. Wojtas, Y. S. Chen, M. J. Zaworotko, X. P. Zhang and S. Ma, Angew. Chem., Int. Ed., 2012, 51, 10082 CrossRef CAS PubMed;
(g) S. Takaishi, E. J. DeMarco, M. J. Pellin, O. K. Farha and J. T. Hupp, Chem. Sci., 2013, 4, 1509 RSC;
(h) R. Saha, B. Joarder, A. S. Roy, S. M. Islam and S. Kumar, Chem. – Eur. J., 2013, 19, 16607 CrossRef CAS PubMed;
(i) Y. X. Tan, Y. P. He and J. Zhang, Chem. Mater., 2012, 24, 4711 CrossRef CAS.
-
(a) D. X. Xue, A. J. Cairns, Y. Belmabkhout, L. Wojtas, Y. Liu, M. H. Alkordi and M. Eddaoudi, J. Am. Chem. Soc., 2013, 135, 7660 CrossRef CAS PubMed;
(b) J. Jia, F. Sun, H. Ma, L. Wang, K. Cai, Z. Bian, L. Gao and G. Zhu, J. Mater. Chem. A, 2013, 1, 10112 RSC;
(c) D. J. Tranchemontagne, K. S. Park, H. Furukawa, J. Eckert, C. B. Knobler and O. M. Yaghi, J. Phys. Chem. C, 2012, 116, 13143 CrossRef CAS;
(d) X. Kong, H. Deng, F. Yan, J. Kim, J. A. Swisher, B. Smit, O. M. Yaghi and J. A. Reimer, Science, 2013, 341, 882 CrossRef CAS PubMed;
(e) H. Deng, S. Grunder, K. E. Cordova, C. Valente, H. Furukawa, M. Hmadeh, F. Gándara, A. C. Whalley, Z. Liu, S. Asahina, H. Kazumori, M. O'Keeffe, O. Terasaki, J. F. Stoddart and O. M. Yaghi, Science, 2012, 336, 1018 CrossRef CAS PubMed;
(f) P. V. Dau, M. Kim and S. M. Cohen, Chem. Sci., 2013, 4, 601 RSC;
(g) J. B. Liu and G. K. H. Shimizu, Inorg. Chem. Front., 2014, 1, 302 RSC;
(h) O. K. Farha, C. E. Wilmer, I. Eryazici, B. G. Hauser, P. A. Parilla, K. O'Neill, A. A. Sarjeant, S. T. Nguyen, R. Q. Snurr and J. T. Hupp, J. Am. Chem. Soc., 2012, 134, 9860 CrossRef CAS PubMed;
(i) S. Henke, A. Schneemann, A. Wütscher and R. A. Fischer, J. Am. Chem. Soc., 2012, 134, 9464 CrossRef CAS PubMed.
-
(a) L. R. Guo, X. L. Tang, Z. H. Ju, K. M. Zhang, H. E. Jiang and W. S. Liu, CrystEngComm, 2013, 15, 9020 RSC;
(b) Y. Gao, J. Cao, Y. Song, G. Zhang, Y. Wang and Z. Liu, CrystEngComm, 2013, 15, 8522 RSC;
(c) Y. B. Shu, X. L. Tang and W. S. Liu, Inorg. Chem. Front., 2014, 1, 226 RSC;
(d) J. M. Zhou, W. Shi, N. Xu and P. Cheng, Inorg. Chem., 2013, 52, 8082 CrossRef CAS PubMed;
(e) J. S. Qin, S. R. Zhang, D. Y. Du, P. Shen, S. J. Bao, Y. Q. Lan and Z. M. Su, Chem. – Eur. J., 2014, 20, 5625 CrossRef CAS PubMed;
(f) Y. Wang, J. Yang, Y. Y. Liu and J. F. Ma, Chem. – Eur. J., 2013, 19, 14591 CrossRef CAS PubMed;
(g) Y. P. He, Y. X. Tan and J. Zhang, Inorg. Chem., 2013, 52, 12758 CrossRef CAS PubMed;
(h) S. Y. Liao, W. Gu, L. Y. Yang, T. H. Li, J. L. Tian, L. Wang, M. Zhang and X. Liu, Cryst. Growth Des., 2012, 12, 3927 CrossRef CAS;
(i) Y. L. Cai, F. L. Jiang, L. Chen, Y. Bu, K. Z. Su, S. A. Al-Thabaiti and M. C. Hong, Inorg. Chem., 2013, 52, 7658 CrossRef PubMed;
(j) Q. Tang, S. Liu, Y. Liu, D. He, J. Miao, X. Wang, Y. Ji and Z. Zheng, Inorg. Chem., 2014, 53, 289 CrossRef CAS PubMed;
(k) C. Gao, A. M. Kirillov, W. Dou, X. Tang, L. Liu, X. Yan, Y. Xie, P. Zang, W. Liu and Y. Tang, Inorg. Chem., 2014, 53, 935 CrossRef CAS PubMed;
(l) Y. Liu, M. Pan, Q. Y. Yang, L. Fu, K. Li, S. C. Wei and C. Y. Su, Chem. Mater., 2012, 24, 1954 CrossRef CAS.
-
(a) D. Tian, Y. Li, R. Y. Chen, Z. Chang, G. Y. Wang and X. H. Bu, J. Mater. Chem. A, 2014, 2, 1465 RSC;
(b) M. Guo and Z. M. Sun, J. Mater. Chem., 2012, 22, 15939 RSC;
(c) S. Dang, X. Min, W. Yang, F. Y. Yi, H. You and Z. M. Sun, Chem. – Eur. J., 2013, 19, 17172 CrossRef CAS PubMed;
(d) H. Wang, W. Yang and Z. M. Sun, Chem. – Asian J., 2013, 8, 982 CrossRef CAS PubMed;
(e) L. Zhou, J. Zhang, Y. Z. Li and H. B. Du, CrystEngComm, 2013, 15, 8989 RSC;
(f) D. Banerjee, Z. Hu and J. Li, Dalton Trans., 2014, 43, 10668 RSC;
(g) G. Liu, Y. Qin, L. Jing, G. Wei and H. Li, Chem. Commun., 2013, 49, 1699 RSC;
(h) M. J. Dong, M. Zhao, S. Ou, C. Zou and C. D. Wu, Angew. Chem., Int. Ed., 2014, 53, 1575 CrossRef CAS PubMed;
(i) S. Pramanik, Z. Hu, X. Zhang, C. Zheng, S. Kelly and J. Li, Chem. – Eur. J., 2013, 19, 15964 CrossRef CAS PubMed;
(j) B. Gole, A. K. Bar and S. Mukherjee, Chem. – Eur. J., 2014, 20, 2276 CrossRef CAS PubMed;
(k) G. Y. Wang, C. Song, D. M. Kong, W. J. Ruan, Z. Chang and Y. Li, J. Mater. Chem. A, 2014, 2, 2213 RSC.
-
(a) D. Cunha, M. B. Yahia, S. Hall, S. R. Miller, H. Chevreau, E. Elkaïm, G. Maurin, P. Horcajada and C. Serre, Chem. Mater., 2013, 25, 2767 CrossRef CAS;
(b) O. K. Farha, I. Eryazici, N. C. Jeong, B. G. Hauser, C. E. Wilmer, A. A. Sarjeant, R. Q. Snurr, S. T. Nguyen, A. Ö. Yazaydin and J. T. Hupp, J. Am. Chem. Soc., 2012, 134, 15016 CrossRef CAS PubMed;
(c) J. H. Wang, M. Li and D. Li, Chem. Sci., 2013, 4, 1793 RSC;
(d) M. Zhang, W. Lu, J. R. Li, M. Bosch, Y. P. Chen, T. F. Liu, Y. Liu and H. C. Zhou, Inorg. Chem. Front., 2014, 1, 159 RSC;
(e) T. Wan, D. X. Zhang, Q. R. Ding, H. B. Zhang and J. Zhang, Inorg. Chem. Front., 2014, 1, 389 RSC;
(f) A. J. Calahorro, A. P. Sanjuan, M. Melguizo, D. F. Jimenez, G. Zaragoza, B. Fernández, A. S. Castillo and A. R. Diéguez, Inorg. Chem., 2013, 52, 546 CrossRef CAS PubMed;
(g) Z. Zhang, Y. Chen, X. Xu, J. Zhang, G. Xiang, W. He and X. Wang, Angew. Chem., Int. Ed., 2014, 53, 429 CrossRef CAS PubMed;
(h) W. Y. Gao, W. Yan, R. Cai, K. Williams, A. Salas, L. Wojtas, X. Shi and S. Ma, Chem. Commun., 2012, 48, 8898 RSC;
(i) T. K. Prasad and M. P. Suh, Chem. – Eur. J., 2012, 18, 8673 CrossRef CAS PubMed.
-
(a) Y. N. Wang, J. H. Yu and J. Q. Xu, Polyhedron, 2014 DOI:10.1016/j.poly.2014.06.028;
(b) Y. N. Wang, Q. F. Yang, G. H. Li, P. Zhang, J. H. Yu and J. Q. Xu, Dalton Trans., 2014, 43, 11646 RSC;
(c) Y. N. Wang, G. H. Li, F. Q. Bai, J. H. Yu and J. Q. Xu, Dalton Trans. 10.1039/c4dt01877j;
(d) Y. N. Wang, G. H. Li, Q. F. Yang, J. H. Yu and J. Q. Xu, CrystEngComm, 2014, 16, 2692 RSC;
(e) Y. N. Wang, F. Q. Bai, J. H. Yu and J. Q. Xu, Dalton Trans., 2013, 42, 16547 RSC;
(f) J. Jin, F. Q. Bai, M. J. Jia, Y. N. Wang, H. L. Jia, J. H. Yu and J. Q. Xu, Dalton Trans., 2013, 42, 8771 RSC;
(g) J. Jin, F. Q. Bai, M. J. Jia, J. H. Yu and J. Q. Xu, CrystEngComm, 2013, 15, 5919 RSC;
(h) J. Jin, F. Q. Bai, G. H. Li, M. J. Jia, J. J. Zhao, H. L. Jia, J. H. Yu and J. Q. Xu, CrystEngComm, 2012, 14, 8162 RSC;
(i) J. Jin, M. J. Jia, G. H. Li, G. Zeng, J. H. Yu and J. Q. Xu, Dalton Trans., 2012, 41, 10267 RSC;
(j) J. Jin, F. Q. Bai, M. J. Jia, J. H. Yu and J. Q. Xu, Dalton Trans., 2012, 41, 6137 RSC;
(k) J. Jin, F. Q. Bai, M. J. Jia, Y. Peng, J. H. Yu and J. Q. Xu, Dalton Trans., 2012, 41, 2382 RSC.
-
(a) W. Ahmad, L. Zhang and Y. Zhou, Photochem. Photobiol. Sci., 2014, 13, 660 RSC;
(b) W. Ahmad, L. Zhang and Y. Zhou, CrystEngComm, 2014, 16, 3521 RSC;
(c) X. X. Hu, J. Q. Xu, P. Cheng, X. Y. Chen, X. B. Cui, J. F. Song, G. D. Yang and T. G. Wang, Inorg. Chem., 2004, 43, 2261 CrossRef CAS PubMed;
(d) X. X. Hu, C. L. Pan, J. Q. Xu, X. B. Cui, G. D. Yang and T. G. Wang, Eur. J. Inorg. Chem., 2004, 1566 CrossRef CAS PubMed;
(e) X. Y. Yu, L. Ye, X. Zhang, X. B. Cui, J. B. Zhang, J. Q. Xu, Q. Hou and T. G. Wang, Dalton Trans., 2010, 39, 10617 RSC;
(f) X. Y. Yu, X. B. Cui, J. J. Yang, J. P. Zhang, Y. H. Luo, H. Zhang and W. P. Gao, CrystEngComm, 2012, 14, 4719 RSC.
- G. M. Sheldrick, Acta Crystallogr., Sect. A: Found. Crystallogr., 2008, 64, 112 CrossRef CAS PubMed.
- S. R. Battern and R. Robson, Angew. Chem., Int. Ed., 1998, 37, 1460 CrossRef.
-
(a) B. Morzyk-Ociepa, J. Mol. Struct., 2007, 846, 74 CrossRef CAS PubMed;
(b) B. Morzyk-Ociepa, J. Mol. Struct., 2007, 833, 121 CrossRef CAS PubMed.
- J. Jin, D. Wu, M. J. Jia, Y. Peng, J. H. Yu, Y. C. Wang and J. Q. Xu, J. Solid State Chem., 2011, 184, 667 CrossRef CAS PubMed.
- J. Jin, W. Xu, M. J. Jia, J. J. Zhao, J. H. Yu and J. Q. Xu, Inorg. Chim. Acta, 2011, 378, 72 CrossRef CAS PubMed.
-
(a) K. Kanagaraj and K. Pitchumani, Chem. – Asian J., 2014, 9, 146 CrossRef CAS PubMed;
(b) K. Gavvala, A. Sengupta, R. K. Koninti and P. Hazra, ChemPhysChem, 2013, 14, 3375 CrossRef CAS PubMed;
(c) W. Yang and X. Chen, Phys. Chem. Chem. Phys., 2014, 16, 4242 RSC.
Footnote |
† Electronic supplementary information (ESI) available. CCDC 1015188–1015191. For ESI and crystallographic data in CIF or other electronic format see DOI: 10.1039/c4qi00100a |
|
This journal is © the Partner Organisations 2014 |
Click here to see how this site uses Cookies. View our privacy policy here.