DOI:
10.1039/D5RA00387C
(Review Article)
RSC Adv., 2025,
15, 7799-7825
Lipid-based nanoformulations in onychomycosis therapy: addressing challenges of current therapies and advancing treatment
Received
16th January 2025
, Accepted 5th March 2025
First published on 11th March 2025
Abstract
Onychomycosis significantly impacts approximately 20% of the global population. The physical barriers of the nail structure make fungal infections a persistent therapeutic challenge. Traditional approaches, including topical and oral antifungal agents, have limitations such as toxicities, low nail permeability, adverse effects, and high recurrence rates. Consequently, emerging lipid-based delivery systems have gained interest because of their potential to address these drawbacks. Nanostructured lipid carriers (NLCs), solid lipid nanoparticles (SLNs), liposomes, and transferosomes are innovative formulations that offer enhanced drug solubility, sustained release, and targeted delivery to the nail matrix. These lipid-mediated approaches have shown promise in overcoming the hurdles associated with conventional therapies, thereby improving treatment outcomes, patient compliance, and the overall quality of life. A comprehensive review focusing on the potential of lipid-based drug delivery systems in treating onychomycosis is lacking in the existing literature. This review explores various aspects of the clinical presentation of onychomycosis, available treatments, challenges associated with treatment, formulation science related to lipid-based vehicles and their applications, highlighted by the promising aspects of these novel formulations, and provides insights into clinical developments. In addition, the regulatory perspective is critical to such development, and approval is discussed, particularly in managing regulatory compliance complexities to ensure successful implementation. The holistic approach provides a comprehensive basis for determining lipid-based drug delivery systems' state-of-the-art and future scope.
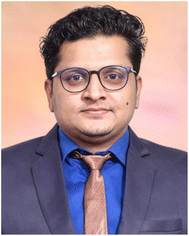 Shiv Kumar Prajapati | Shiv Kumar Prajapati is currently a PhD research scholar at the Institute of Pharmaceutical Research, GLA University, Mathura, India, under the supervision of Dr Meenakshi Bajpai and Dr Ankit Jain. He has five years of academic experience and previously worked as a Junior Research Fellow on a Madhya Pradesh Council of Science and Technology-sponsored project at Bhagyoday Tirth Pharmacy College, Sagar, India. He earned his MPharm degree from Adina Institute of Pharmaceutical Sciences, Sagar, India. He has co-authored more than 40 research and review papers, along with book chapters published in reputed international journals. His research work has received over 835 citations, with an h-index of 12 and an i10-index of 13. His research interests include drug delivery systems, formulation development, nanotechnology, biomedical applications of nanomaterials, targeted drug delivery, polymer-based drug carriers, and biopharmaceutical research. He has received several awards for his research presentations at national and international conferences, including the International Golden Globe Award 2019. |
 Ankit Jain | Dr Ankit Jain has been employed as an assistant professor in the Department of Pharmacy at BITS-Pilani since March 2024. He is a distinguished scientist renowned in the field of drug delivery, having secured a position among the Top 2% of scientists globally according to Stanford University. Over the past decade, his research has concentrated on the development of lipid/polymer hybrid nanostructures and advanced ionic liquids for targeted drug delivery applications in cancer and chronic diseases. Dr Jain possesses specialized knowledge in drug delivery systems for BCS-II/IV drugs, particularly in lipidic and polymeric carriers. He has authored over 100 publications in highly esteemed journals, garnering more than 4000 citations with an H-index of 37. During his postdoctoral training at Texas A&M University, he gained expertise in protein and peptide-based carriers aimed at addressing cerebral ischemic conditions. Previously, at the Indian Institute of Science, he worked as a CV Raman Postdoctoral Fellow with Prof. Ashok M. Raichur, researching pH-responsive lipid/polymer self-assembling systems designed for mitochondrial targeting in cancer therapy. His doctoral research focused on thermosensitive liposomal therapy, resulting in a granted patent (IN, 535581). In various leadership roles across both intramural and extramural projects, he has made substantial contributions to the stability and development of bioactive compounds and delivery vehicles, including liposomes, nanoparticles, and carbon nanotubes. |
 Meenakshi Bajpai | Dr Meenakshi Bajpai is currently working as Professor and Head of Institute of Pharmaceutical Research at GLA University, Mathura, India. She is a distinguished academician and researcher with over 45 years of experience in pharmaceutical sciences. She earned her MPharm in Pharmaceutics and PhD from Dr Hari Singh Gour Vishwavidyalaya, Sagar. Dr Bajpai has made significant contributions to research, teaching, and academic administration. She has authored more than 70 research papers in reputed national and international journals and holds multiple patents. As an active peer reviewer for various scientific journals, she has played a crucial role in maintaining research integrity. Additionally, she has published several books and review articles. Her research work has received over 1158 citations, with an h-index of 21 and an i10-index of 40. Her research interests focus on drug delivery systems, formulation development, and nanotechnology. |
1. Introduction
Onychomycosis is characterized by fungal colonization within the nail bed and manifests with various clinical presentations depending on the causative fungal species, disease severity, and individual factors. The therapeutic management of onychomycosis presents numerous challenges due to the complex nature of the ailment and the unique composition of the afflicted nail structure.1,2 The nail serves as a challenging barrier, impeding the effective diffusion of topical antifungal substances to the underlying infection site, thus compromising treatment efficacy.3 Furthermore, the chronic and refractory characteristics of the condition, sluggish growth of nails, and presence of fungal spores in the environment may cause non-compliance by patients, necessitating prolonged therapeutic interventions.4–6 This challenge is compounded by the diverse fungal species responsible for onychomycosis, each with distinct sensitivity to antifungal agents.7,8 Correct identification of the causative agent is critical to deciding on appropriate therapy. Onychomycosis has various clinical subtypes (Fig. 1A). Distal Lateral Subungual Onychomycosis (DSO), caused by T. rubrum and T. mentagrophytes, begins at hyponychium and invades the nail as it progresses, causing discoloration and thickening.9,10 White Superficial Onychomycosis (WSO), mainly from T. mentagrophytes, affects the upper nail plate, creating white patches and making the nail rough and crumbly.11 Proximal Subungual Onychomycosis (PSO), often seen in immunocompromised patients, begins at the proximal nail fold with T. rubrum or Fusarium species, leading to leukonychia.12,13 Endonyx onychomycosis involves only the nail plate, caused by Trichophyton soudanense and Trichophyton violaceum, causing milky patches without nail bed involvement.14,15 Total dystrophic onychomycosis (TDO) signifies the culminating phase of any subtype, leading to severe nail destruction.16,17 The prevalence of onychomycosis varies significantly among various parts of the world. For the United States, it has been estimated to be between 2 and 14%. European rates are much higher, at approximately 23% of the population affected. For example, the United Kingdom has about 2.7%, while Germany has between 15 and 20%. It is more prevalent in France, between 20% and 30%. Its incidence is substantially high in the Asia-Pacific region; about 10% of the population of Japan translates to approximately 11 million people. In Latin America, the prevalence is relatively significant, especially with the high prevalence of diabetes and HIV infections. Both infections heighten the risk of acquiring the infection with these fungi. In the Middle East and Africa, onychomycosis is increasingly on the rise due to the sharp increase in diabetes. Countries in the region, such as Saudi Arabia, are dominated by enormous fungal infections that affect most individuals diagnosed with diabetes.18 The development of drug-resistant strains has complicated treatment strategies. A patient's compliance with a topical or systemic regimen often becomes an ongoing challenge, at least in regular application or administration. This convergence of multifactorial challenges makes the issue of onychomycosis not only a diffuse concern but also one that is complex in nature, such that the treatment of onychomycosis involves a complete, patient-specific approach that encompasses not only clinical resolution but also aesthetic improvement considering the individual's health. Only a few classes of antifungals are currently available to treat toenail infections; Fig. 1B shows a timeline of their discovery and market launch. Scheme 1 shows the mechanism of various antifungal drugs and targeted delivery by lipid-based formulations to treat fungal infections in the nail bed.
 |
| Fig. 1 (A) Clinical subtypes of toenail fungal infection (this figure has been reproduced from ref. Lipner and Scher19 with permission from Elsevier, copyright, 2019). (B) Milestones in antifungal drug development for onychomycosis; (C) co-occurrence-based bibliometric analysis of available literature on the PUBMED database from 2000 to 2024 using the VOSviewer platform. | |
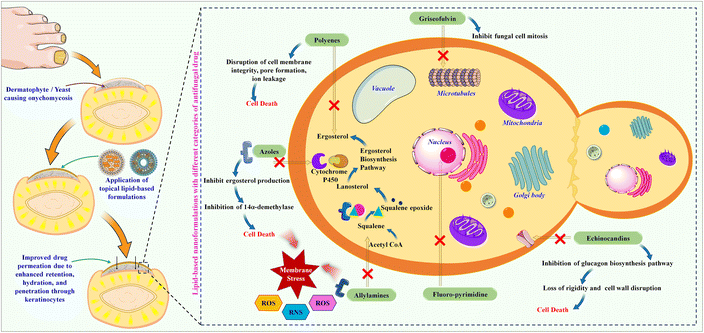 |
| Scheme 1 Schematic illustration of fungal nail growth and inhibition causative organism by ungual administration of lipid-based formulations. | |
There are conventional approaches, such as systemic and topical treatments, and physical treatments, such as laser therapy. However, these approaches often have disadvantages, such as poor drug penetration, long treatment times, and increased relapse rates, highlighting the urgent need for innovative solutions. Lipid-based nanocarriers have been developed as a cutting-edge delivery method to circumvent the limitations of traditional antifungal formulations in onychomycosis. A compact keratinized nail plate is an impermeable barrier, which limits the penetration of the drug and prevents the effectiveness of local therapy. At the same time, antifungals administered systemically also lose effectiveness due to reduced bioavailability and systemic toxicity.20 These nanocarriers, including SLNs, NLCs, liposomes nanoemulsions, etc., are molecularly optimized solutions for increasing solubility, stability, permeability, and drug retention.20 The lipid composition of these carriers is biocompatible. It interacts with the nail matrix through hydrophobic and van der Waal interactions, allowing drug permeation at deeper sites and long-term treatment. In addition, their ability to regulate the release pathways prevents the drug's initial degradation and systemic clearance, leading to prolonged drug release around the infection. Lipid nanotechnology as an antifungal treatment option has transformed the paradigms of drug delivery and provides precision-driven and targeted drug transport while circumventing the limitations of pharmacokinetics and drug resistance mechanisms. In addition to improved intracellular drug retention, these carriers, together with a lower dose regimen, prevent systemic toxicity improve therapeutic efficacy and patient compliance.21,22 Unlike conventional antifungals that act solely on fungal cells, lipid-based carriers can modify the nail structure, increasing hydration and softening the keratin barrier, thereby improving drug uptake and retention. By addressing the key problems of conventional antifungal treatments, lipid-based carriers propose a promising platform for the most effective treatment of onychomycosis and merit further translational and clinical research.
This review explores the pathophysiology and clinical attributes of onychomycosis, the status of available treatment, and discusses the challenges to effective treatment. Further, the review specifically focused on the advances and applications of lipid-based nanocarrier systems for treating onychomycosis, their ability to improve drug delivery, and achieving optimal therapeutic effects. These novelties are considered promising avenues to formulate more efficient and patient-oriented treatment approaches for onychomycosis.
2. Bibliometric analysis
VOSviewer, a popular software tool for generating bibliometric network maps and visualization, was used as the bibliometric analysis platform. Fig. 1C presents a thorough, co-occurrence-based bibliometric network analysis of terms related to onychomycosis or fungal infections of the toenails from the available literature in the PUBMED database from 2000 to 2024. It also provides a detailed account of the relationships between the clinical, diagnostic, and therapeutic areas within the onychomycosis research landscape. Key elements include “human,” “foot dermatoses,” “physical treatment approach,” “new treatment approach,” “transungual/topical administration,” and “treatment outcome,” with the links representing co-existing relationships between topics such as drug delivery systems, diagnostic techniques, and antifungal treatments. Also, prevalent research pathways and critical connections are highlighted that promote innovation and future directions in onychomycosis research and treatment (Fig. 1C).
3. Nail structure and clinical manifestations of onychomycosis
Anatomically, the nail includes the proximal nail fold (PNF), nail matrix, nail bed, and hyponychium, collectively making up the nail plate.23 The ungueal plate, i.e., nail plate, is characterized by a flat rectangular shape with a translucent appearance composed primarily of keratin protein.24 Its flat, adherent, interlocking cells are densely packed and oriented perpendicular to the nail plate.25 The keratogenous zone of the nail bed that extends beyond the PNF is the whitish lunula, seen clinically and proximally, and covers about 1/4th of the total nail length.26 The matrix of the nail is substantially proliferative. It produces a nail plate and consists of three bonded layers.26,27
The nail plate primarily consists of hard α-keratin, with the dorsal layer potentially acting as a barrier to permeation.28,29 The nail bed is a pink, non-keratinized epithelium that connects the matrix to the hyponychium and contains living cells in the nail plate. Granular layers and sebaceous glands are absent.30 The hyponychium lies beneath the free distal end of the nail and has a protective function comparable to that of the lateral folds, the PNF, and the cuticle (Fig. 1).31 Drug delivery through the transungual route is essential for treating nail diseases, such as onychomycosis, psoriasis, paronychia, and onycholysis. Topical drug delivery is preferred to avoid systemic side effects and to provide direct benefits. However, a significant challenge is the poor permeability of drugs through the nail plate, which is attributed to the dense network of cross-linked keratin proteins.32 The human nail contains 2–35% water and 0.1–1% lipids, resembling a hydrophilic gel membrane with a lipophilic route, 10.60% disulfide linkage with 50–1000 μ thickness, 25% Maximum swelling capacity and 1.94 mg cm−2 h−1 water loss rate.33 Variations in solute penetration are due to nail properties. Traditionally seen as a fiber matrix, nails may have residual lipids, lowering the permeability to specific solutes. Cellular membrane remnants may partially obstruct the transport of hydrophilic solutes.32,34,35 Topical drugs are typically favored because of their localized action, without any side effects, which helps patients tolerate the therapy inexpensively.
The following are the sophisticated clinical presentation of onychomycosis: (i) dystrophic alterations mark the early stages, encompassing nail plate discoloration, often displaying hues of yellow, white, brown, or even a dark greenish-black tint as the disease advances. (ii) Progressive infection leads to hypertrophy and malformation of the affected nail. Altered contouring, including convexity or irregular shaping, emerges, potentially causing discomfort during footwear use. (iii) Nail fragility becomes evident, resulting in onychorrhexis, in which nails are susceptible to splitting or breaking, contributing to further structural compromise. (iv) The nail texture undergoes a friable transformation and is prone to crumbling into minute fragments, indicative of advanced pathology. (v) Progressive fungal growth induces onycholysis, which causes nail separation from the underlying nail bed. This separation causes discomfort and creates an interstice susceptible to secondary infections. (vi) Subungual hyperkeratosis signifies keratin debris accumulation beneath the nail plate. This accumulation resulted in nail elevation and interstitial spacing. (vii) In advanced instances, fungal degradation products contribute to the malodor emanating from the infected nail site. (viii) As the infection escalates, nail hypertrophy and malformation provoke discomfort and pain, accentuated during ambulation or footwear application. (ix) The contiguous spread of onychomycosis from one nail to adjacent ones is characteristic, resulting in progressive poly dystrophy, affecting multiple nails. (x) Impaired nail defense mechanisms render nails susceptible to secondary pyogenic bacterial infections. Ensuing bacterial colonization accentuates inflammation and exacerbates patient discomfort.
4. Pathogenesis of fungal nail invasion
Onychomycosis usually begins upon contact of the nail with an agent of fungi such as dermatophytes, non-dermatophyte molds, and yeasts in settings that promote the growth of fungi. Following this specific contact, the fungus secretes particular enzymes capable of degrading keratin, allowing it to penetrate deeper into the nail. The formation of biofilms leads to a reduced immune response in the area of the nail processes, which aggravates the infection (Fig. 2). Thus, it becomes difficult to manage therapeutically. Onychomycosis is transmitted through direct contact between the nail and dermatophytes, non-dermatophyte molds, or yeasts.36 This contact can occur in various settings, such as damp environments, locker rooms, or swimming pools, where fungi thrive. When nails encounter these fungal agents, they can adhere to the nail surface and initiate infection. Fungi, especially dermatophytes, produce proteolytic, keratinolytic, and lipolytic activity enzymes. Proteolytic enzymes break down proteins, keratinolytic enzymes degrade keratin, and lipolytic enzymes digest lipids.36,37 In onychomycosis, these enzymes work together to degrade the tough keratin in the nail plate, enabling the fungi to access nutrients within the nail. This enzymatic action softens nail structure, allowing fungal penetration.38 Once keratin is degraded, fungi can easily invade the nail.39 They penetrate through small openings, injuries, or separations between the nail plate and bed. Softened keratin provides a pathway for fungal hyphae (thread-like structures) to infiltrate the nail layers, starting from the hyponychium.40–42 This reduced immune activity makes nails susceptible to fungal invasion. Unlike the skin or mucous membranes, immune cell access to the nail plate is restricted, allowing fungi to establish infections with less resistance.43,44
 |
| Fig. 2 Pathogenesis for fungal nail invasion represents keratin degradation, biofilm formation, and invasive patterns that cause different clinical subtypes of onychomycosis: (a) DSO; (b) endonyx onychomycosis; (c) WSO; (d) PSO; (e) TDO. | |
Fungal biofilms are microorganisms surrounded by a protective layer of proteins, sugars, and other substances.45 These biofilms form within the nails as a defense mechanism, making it difficult for antifungal agents and immune cells to reach and eliminate the infection. The biofilm creates a barrier that protects the fungi from the immune system and makes the treatment less effective.46,47 Clinical subtypes of onychomycosis can arise depending on the location and manner of the invasion within the nail. For instance, the invasion that starts in the hyponychium and moves distally is known as the distal lateral subungual subtype. The white superficial subtype comprises invasion within the upper nail plate surface. The proximal subungual subtype extends distally from the proximal nail fold. The unique invasion pattern of each subtype gives rise to its characteristic clinical features (Fig. 2).
5. Conventional antifungal therapies and associated challenges with transungual drug delivery
The main reason for the challenge in managing onychomycosis is the not-so-understood intrinsic pathophysiological mechanisms, and other factors that complicate the scenario include characteristics of fungal growth and particular susceptibility of the individual patient. Various approaches to improving drug delivery to the nail to treat nail infections and disorders effectively have already been developed. In any case, it should be noted that every method of pulsed lasers and ultrasound-mediated delivery to traditional topical and oral therapies has its specific mechanism, advantages, and challenges. This comparative analysis facilitates clinical practitioners in making well-informed decisions by suggesting a detailed understanding of the benefits and shortcomings of each technique. Each technique is discussed in Table 1.
Table 1 Different approaches for onychomycosis treatment with their key insights
Approach |
Mechanism |
Key considerations |
Benefits |
Challenges |
Ref. |
Pulsed lasers |
Pulsed laser disrupts the integrity of keratin chains in the nail, creating craters or holes to allow for deeper drug penetration |
Laser intensity and duration: choice between removing the nail or creating perforations |
Allows direct drug application to the site of infection; reduces relapse rates, especially with carbon dioxide lasers in onychomycosis |
Risks include thermal damage and uncertain outcomes in some cases |
29, 48 and 49 |
Ultrasound mediated delivery |
Low-intensity ultrasound is used to increase permeability by cavitation or formation of pits |
Acoustic power and exposure duration |
It enhances drug flux threefold, reduces treatment time, and minimizes side effects associated with systemic therapy |
Technology needs more testing for broader use |
50 and 51 |
Iontophoresis |
It uses an electric field to enhance transungual permeation by electrophoresis and electroosmosis |
pH, buffer ionic strength, current density (maximum 0.5 mA cm−2) |
Achieves higher drug concentrations within a shorter time, making it practical for fungal infections |
It requires a controlled electric field and limited penetration of larger molecules |
52–55 |
Hydration |
Water acts as a plasticizer, increasing pore size and facilitating transungual permeation |
Humidity level, water loss, ceramide concentration, and water-binding capacity |
Increased permeation threefold with higher relative humidity (100%) improves nail condition in diseases like onychomycosis |
Prolonged exposure to water may weaken nails; efficacy depends on hydration status |
41 and 56 |
Etching |
It uses chemicals like phosphoric acid to create microporosities that increase surface roughness, improve drug penetration and bonding, promote the reduction of contact angle, enhance wettability |
Roughness, wettability, and interpenetration of the delivery system |
Enhances permeability and interpenetration of antifungals |
It can lead to surface damage if overused and limited to localized treatment |
48, 57 and 58 |
Topical applications |
Involves direct application of antifungal or therapeutic agents over the nail plate for localized drug delivery |
Drug formulation, penetration enhancers, frequency of application |
Minimizes systemic side effects and allows direct treatment of fungal or bacterial infections |
Often limited by poor permeability of the nail plate, leading to insufficient drug concentrations at the target site |
59–61 |
Oral therapy |
Involves systemic absorption and subsequent transport of drugs through the bloodstream to the affected nail tissue |
Absorption rate, metabolism, bioavailability, and potential for drug interactions |
Provides a comprehensive treatment for infections, including those affecting the nail bed |
A higher risk of side effects, including liver toxicity and gastrointestinal disturbances, often requires prolonged treatment |
62 and 63 |
5.1 Challenges associated with transungual drug delivery
The treatment modalities for onychomycosis face several challenges that require developing novel therapeutic approaches.64 There are several challenges in effectively treating onychomycosis, a fungal infection of the nails. These challenges include the rigid structure of the nail, which makes it difficult for medications to penetrate, the high cost of treatment, the prolonged duration of therapy required, and the high probability of disease recurrence. These factors contribute to the barriers to successful outcomes in treating onychomycosis.65 Oral antifungals have a limited safety profile, often resulting in concerns regarding liver toxicity and drug interactions. Topically applied antifungal agents can penetrate the nail plate in vitro, but their penetration is insufficient to penetrate the deeper keratinized layers and matrix. This limitation limits its use to superficial infection only, but clinical studies are still inconclusive. This more extended presence of the active ingredient in the nail enables weekly dosing, a shortened treatment period, or pulse treatments. Combining topical and oral antifungal agents acting on the nail from multiple sides is an optimal pharmacokinetic approach. The high porosity, water-resistant, dense nail plate matrix is such a core limitation of drug penetration that it confines the efficacy of these agents against any deeper-lying infections. This poor penetration limits topical therapies to superficial mycoses, which have limited chances of a successful outcome; therefore, the infection is only limited to the outer layers of the nail. Antifungal molecules can reach the nail bed and the nail matrix via the blood circulatory system with the oral/systemic route of administration. Because onychomycosis is challenging to treat, it often takes more than 18 months to achieve a complete cure, and 20–25% of cases do not respond to treatment. Doctors become aware of rapid recurrence because either fungal spores or hyphae remain. The slow growth rate of toenails, the thickness of the nail keratin, and the persistence of the fungus in the environment (e.g., in shoes) influence its effectiveness. On the other hand, the immune response and the high keratin density in the closed nail bed are responsible for the complexity of complete healing.66 Onychomycosis usually persists for a long time, mainly if effective treatment is not provided. Biofilms, complex communities of microorganisms that adhere tightly to epithelial surfaces via an extracellular matrix, can be formed by fungi. The development of these biofilms represents a barrier to treatments, which is a significant factor in the high rate of recurrence, relapse, and treatment failure observed in onychomycosis. This defensive measure highlights the challenge of eliminating the infection and developing novel therapeutic approaches to combat biofilm resistance.67,68
5.1.1 Limited nail penetration. Penetration of the drug into the nail matrix is often insufficient, limiting the effectiveness of treatment. This is partly caused by the thickness of the keratin in the nails, the slow growth rate of toenails, and the ability of fungi to survive in conditions such as shoes. Because of these factors, it is difficult to eradicate fungal infections with topical treatments. The rugged, protective nail plate, the storage of pathogens between the plate and the nail bed, and the slow growth of the nail also cause problems. The barrier properties of the nail plate make it even more challenging to deliver antifungal drugs to the tissue in the concentrations required to kill fungi deeply embedded in the nail bed. When comparing drug penetration, weight-based measurements or highly concentrated solutions or suspensions have generally been used.69
5.1.2 Fungal resistance mechanisms. Antifungal resistance has significant implications for treating onychomycosis and other fungal infections. Resistance can lead to reduced treatment effectiveness, and problems such as low adherence and long treatment durations can further promote the development of resistance. Non-compliance with treatment can lead to frequent relapses, leading to the selection of resistant fungal strains.70 Longer disease duration and decreased antifungal efficacy allow the fungi that cause onychomycosis to spread and become more invasive. Antifungal resistance can be inherent. That is, it arises without prior drug exposure or is acquired using antifungal drugs.71,72 Microbiological resistance mechanisms include gene mutations or changes in gene expression, which can reduce drug absorption, induce structural changes, accelerate drug degradation, or increase drug efflux. Biofilms also play a role in mediating resistance by protecting the fungi from the immune system and drugs.73,74 Despite proper medication intake, clinical resistance persists, i.e., the interaction between patient, drug, and fungus. Correct diagnosis and ongoing treatment are among the basic measures for resistance prevention and treatment of onychomycosis.5
5.1.3 Host immunity variability and impact of comorbidities. Variabilities in the host immune response, such as those seen in immunocompromised individuals, can impact treatment outcomes and lead to chronic or recurrent infections. Nails pose a significant challenge for treatment due to their lack of intrinsic immune function and impenetrability. Factors such as a weakened immune system (standard in HIV patients), diabetes, and peripheral vascular disease can affect treatment success. 75–77 In addition, conditions such as tinea pedis, age, and obesity increase the risk, while comorbidities such as diabetes and psoriasis increase susceptibility to disease. Effective treatment is often hampered by slow nail growth, patient comorbidities, and the reluctance of prescribers and patients to take oral medications due to perceived toxicity concerns. The threat of developing onychomycosis is significantly increased by several underlying medical conditions, including nail trauma, nail psoriasis, tinea pedis (athlete's foot), and patient characteristics such as age and obesity. Comorbid medical conditions that increase susceptibility to this fungal infection include diabetes mellitus,78 various cancers,79 immunodeficiency disorders,80 and peripheral arterial disease.81 In addition, a genetic predisposition to onychomycosis has been identified, suggesting that certain people may be predisposed to the condition. A study conducted at Buea and Limbe Regional Hospitals found a prevalence of onychomycosis to be 50.7% in 152 diabetic patients. T. rubrum was the most common fungus (62%), followed by T. metagraphyte (22%) and T. tonsurans (16%). No significant sociodemographic or clinical predictors were identified. The fungi were most sensitive to miconazole (66%), with less sensitivity to amphotericin B (19%) and ketoconazole (14%).82 In a study of 38 patients with psoriasis and/or psoriatic arthritis, 57.89% were diagnosed with onychomycosis. Positive results were detected by direct mycological examination in 44.8%, culture in 42.1%, and histopathological examination in 31.6%. T. rubrum was the most common dermatophyte, and yeasts such as C. parapsilosis and C. albicans were also present. The study found a high rate of onychomycosis (92.8%) in patients taking methotrexate alone, suggesting a possible association between psoriatic disease, immunosuppressive therapy, and fungal infections.83
5.1.4 Safety profiles and side effects. Prolonged use of oral antifungals can lead to liver toxicity and drug interactions, especially in vulnerable groups such as the elderly and diabetics. Azole antifungals are responsible for serious drug reactions, including QT prolongation, torsade de pointes, heart failure, rhabdomyolysis, and even death. Topical antifungals used to treat onychomycosis can also cause local side effects, with efinaconazole potentially triggering allergic skin reactions.84 Both patients with and without a history of liver disease may experience increased liver enzymes after taking terbinafine.85 While terbinafine rarely causes side effects in patients with onychomycosis, those who suffer from onychomycosis often report changes in taste and smell. Although it is unclear exactly how terbinafine causes taste disorders, it is thought that taste receptor dysfunction may be due to the inhibition of cytochrome P-450-dependent enzymes.86
5.1.5 Environmental re-exposure and reinfection. The risk of recurrent onychomycosis is strongly influenced by environmental factors, including inappropriate footwear and shared public spaces. Repeated contact with contaminated textiles, shoes, and socks can lead to a new fungal infection. It is known that dermatophytes can colonize in shoes and remain there for long periods by feeding on trapped skin cells and sweat.73,87 In addition, blankets, sheets, and towels can serve as harbors for fungi. After antifungal treatment, wearing contaminated socks and shoes can facilitate reinfection. In addition, the risk of reinfection may be increased if textiles and shoes are stored together or washed in the same machine, as cross-contamination of sterile laundry can occur.88
5.1.6 Aging and nail growth impairment. Treating and monitoring onychomycosis in older people is problematic because nails grow more slowly and take longer to show signs of improvement. Since recurrences are common, older people should develop good hygiene practices and change their lifestyles accordingly. In the long term, topical antifungals one to three times per week for prevention can reduce recurrences. In addition, it is extremely successful in treating the primary infection and keeping the nail structure firm and in good condition.89 Amorolfine and ciclopirox are lacquer-based topical antifungal agents that pose a practical challenge for elderly patients due to frequency of use, effective nail removal, and prolonged treatment duration. However, this was not as practical for these older people as they could not carry out demanding protocols. Therefore, options for treating onychomycosis in this age group with a more straightforward application and maintenance schedule might be worthwhile.90 some nail characteristics make the treatment ineffective. These are onychogryphosis and onycholysis, slow nail growth, and greater nail matrix or plate involvement, which affect over 75 percent. These factors complicate treatment and reduce its effectiveness.91 In addition to the high prevalence of peripheral vascular disease, diabetes, immunosuppression, and physical trauma in the elderly, which are also responsible for poor treatment response or recurrence, there are other factors of interest. On the other hand, patients' lack of compliance, poor hygiene, and unsuitable footwear are mainly responsible for this. These are the critical points that should be addressed to improve the result.92
5.1.7 Cost and accessibility of advanced treatments. Main patient compliance and clinical outcome appear to be significantly compromised by costly treatment options for onychomycosis. In certain cases, the financial burden may result in inadequate or incomplete treatment of a disease, promoting the growth of numerous pathogens and increasing the risk of recurrence. Non-confirmation burdens healthcare systems as it leads to poor health and increased resistance to fungi. Therefore, accessible and affordable treatment options are required for effective treatment, and timely education about the importance and cost of treatments is necessary to increase patient adherence. According to Yousefian and colleagues, the cost of treating onychomycosis varies greatly depending on the treatment plan. Unlike oral therapies, which typically cost $100 or less per month, topical therapies can cost between $100 and $500. PDT, LLLT, and laser treatments can cost between $100 and $1500 per month for a maximum of two months. The average monthly cost for OTC medications and plasma therapy is between $100 and $500.93 When treating onychomycosis, it is critical to consider the patient's financial situation and insurance coverage due to the chronic nature of the disease and the possibility of recurrence.
5.2 Challenges specific to lipid-based nanoformulation
5.2.1 Stability. Lipid-based nanomaterials, such as liposomes, SLNs, and NLCs contend with stability challenges due to particle aggregation, lipid phase transitions, and drug leakage.94,95 Lipid composition, surfactant selection, storage conditions, and pH or ionic strength influence stability. Strategies to enhance stability include the incorporation of cryoprotectants (e.g., sucrose, trehalose) during freeze-drying, PEGylation to improve colloidal stability, optimization of lipid ratios, and adjustment of zeta potential to prevent aggregation. Liposomes are prone to drug leakage, while SLNs may undergo drug expulsion as a result of lipid recrystallization. In contrast, NLCs offer improved stability due to their imperfect lipid matrix. Maintaining stability necessitates careful formulation design, proper storage at low temperatures, and advanced stabilization techniques such as lyophilization.96,97
5.2.2 Scalability. Scaling up the manufacturing of lipid-based nanomaterials presents several challenges, including maintaining consistent particle size distribution, managing complex multi-step processes, ensuring sterility, and addressing batch-to-batch variability. Key issues include particle size control, thoroughly mixing lipid components and active ingredients, equipment limitations, and solvent removal.98 Ensuring sterility, robust process monitoring, and formulation optimization are also crucial. Potential solutions include using microfluidic technology, high-pressure homogenization, single-use systems, and advanced characterization techniques. Collaboration with experienced formulation scientists and process engineers is essential to address these challenges effectively.99,100
5.2.3 Toxicity concerns. Lipid-based nanomaterials hold promise for drug delivery but present notable toxicity concerns that must be carefully addressed. The composition of lipids is a critical factor, as certain lipids can trigger immune responses or induce toxicity. At the same time, oxidation and phase transitions may generate reactive oxygen species, leading to cellular damage.101,102 Particle size and surface charge influence biodistribution, with aggregation potentially causing organ accumulation, particularly in the liver and spleen, increasing toxicity risks.103 Additionally, interactions with plasma proteins can lead to protein corona formation, altering targeting efficiency and immune recognition.104,105 Immune responses, including inflammation and antibody development upon repeated administration, may further limit their clinical application.106,107
6. Designing of lipid-based carrier for transungual drug delivery
Designing a lipid-based formulation for transungual drug delivery presents challenges due to the dense keratinized structure, which significantly hinders drug permeation. This keratin matrix further restricts the diffusion of therapeutic agents by restricting lipid fluidity and hydration and acting as a physical barrier. To overcome this natural barrier, transungual formulations must be specifically developed. In the case of API, as the molecule size increases, drug penetration would decrease. Conversely, the penetration of the active ingredient decreases as the molecular size increases. Except for miconazole, which penetrates the nail plate regardless of pH, it should be noted that different antifungal drugs have different acid strengths. In addition, efinaconazole and tavaborole do not bind to keratin. The permeability coefficient initially decreases as the lipophilicity of the diffusing alcohol molecule increases. However, an additional increase in lipophilicity leads to a higher permeation rate once a particular threshold value is exceeded.108 Nail permeability was studied in terms of the drug's molecular weight, ionic nature, and lipophilicity. Permeability was shown to be unaffected by lipophilicity, but it was considerably lower for ionic drugs and decreased as molecular weight increased. According to a model based on these two variables, permeability is independent of the octanol/water partition coefficient but decreases with increasing molecular weight. Ion hydration increases the apparent molecular weight, which is likely why the dissociation of the drug further reduces permeability. Healthy nail plates and nail plates with fungal infection appear to have similar permeabilities.109 Keratins, the primary structural proteins in human nails, have an isoelectric point (pI) between 4.0 and 5.0. These keratins have a net positive charge at pH values below their pI and a net negative charge above their pI. The surface of the nail plate has an apparent pH of about 5.0, while the interior has an approximate pH of 4.1. Under normal physiological conditions, the nail plate is approximately neutral.35,110 The weakly acidic or basic ionization of API is modulated by the pH of aqueous formulations, altering the hydrophilicity or lipophilicity of these active ingredients. These manipulations directly and significantly affect the solubility of the materials in the formulation matrix, their solubility in the keratinized nail plate, and their interactions with their keratin matrix.111,112 The susceptibility of fungi to certain antifungals is influenced by pH. In particular, naftifine's in vitro antifungal activity against T. mentagrophytes decreases as the pH decreases from 7 to 4.113 Lipids are considered an essential component of topical drug delivery systems intended to act as systemic therapeutics across the skin. These improve the penetration/retention of the drug in the skin. Two primary lipid excipients commonly used in these formulations are fatty acids, triglycerides, phospholipids, and sterols such as cholesterol. They support the formation of efficient and malleable vesicles, the solubility of drugs, and the permeability of the skin barrier.114 Surfactants are added to the formulation to adjust the release profile and improve drug delivery. Surfactants with an HLB greater than or equal to 12 reduce the interfacial tension at the interface, which aids in formulating stabilized colloidal dispersions or vesicular carriers.115 Hydrolysed vegetable oils and polyethylene glycol are familiar sources of these materials, including ethoxylated alcohols and polysorbates. Polyoxyl-hydrogenated castor oil (Cremophor) is widely used for this purpose.116 Surfactants increasing drug solubility aggravate the bioavailability while also controlling the drug release characteristics and improving pharmacokinetic parameters, possibly by inhibiting efflux transporters (Fig. 3) (Table 2).132
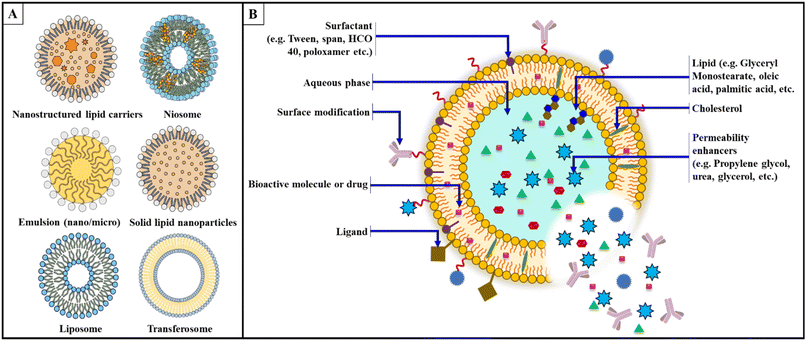 |
| Fig. 3 (A) Different types of lipid-based delivery vehicles; (B) designing of lipid-based drug delivery system. | |
Table 2 Examples of lipid-based excipients for transungual drug delivery
Chemical name |
Synonym |
Melting point (°C) |
Functionalities |
Ref. |
Glycerol behenate |
Compritol 888 ATO |
65–77 |
Viscosity enhancer, sustained-release, lipidic coating excipient |
117 |
Glyceryl caprylate |
Capmul |
30–39 |
Moisturizer, preservative, permeability enhancer, emulsion stabilizer, solubilizer |
118 |
Glyceryl monostearate |
Capmul GMS-50 |
55–60 |
Lubricant, emulsifier, sustained-release ingredient, emollient, stabilizing agent |
117 |
Glyceryl tristearate |
Tristearin |
72–75 |
Controlled release, thickening agent |
119 |
Glyceryl palmitostearate |
Precirol ATO 5 |
52–55 |
Sustained-release agent |
120 and 121 |
Hydrogenated castor wax |
Castorwax, Kolliwa® HCO |
85–88 |
Provide stiffness, thickener, emollient |
122 and 123 |
Cholesterol |
— |
147–150 |
Emollient, emulsifying agent, improves rigidity and stability |
124 |
Stearic acid |
Cetylacetic acid, Kortacid 1895 |
>54 |
Emulsifying agent, solubilizing agent |
125 and 126 |
Palmitic acid |
Cetylic acid, Emersol 140 |
63–64 |
Emulsifying agent, penetration enhancer |
127 |
Oleic acid |
Crodolene |
13–14 |
Emulsifying agent, penetration enhancer |
128 and 129 |
Medium-chain triglycerides |
Miglyol 812 |
|
Emulsifying agent, solvent |
129 |
Castor oil |
EmCon CO; Lipovol CO |
−12 |
Stabilize the emulsion, emollient, oleaginous vehicle, solvent |
130 |
Linoleic acid |
Emersol 310 |
−5 |
Emulsifying agent, penetration enhancer |
131 |
7. Leveraging lipid-based formulations for effective onychomycosis treatment
Novel approaches enhance topical antifungal therapy, addressing conventional treatment limits and the high relapse rate of onychomycosis. Nanotechnology-based approaches modulate drug stability, release kinetics, and residence time, transforming onychomycosis treatment. Target-specific drug delivery systems for administering pharmaceutical compounds enable drug localization to the diseased sites. These novelties support antifungal drug penetration using lipid-based formulations, NEs, MEs and heightened delivery depth. Targeted drug delivery via liposomes, NLCs, and nanoparticles directly direct antifungal agents to the infection site, enhancing efficacy, minimizing side effects, and optimizing outcomes. Sustained drug release is fundamental, allowing controlled and extended delivery, reducing frequent applications, improving convenience, and maintaining therapeutic levels for combatting persistent infection. These systems mitigate systemic exposure by delivering drugs directly to the infection site, reducing the risk of systemic side effects and thus enhancing safety.
Lipid-based formulations provide several advantages over conventional antifungal treatments, such as significantly enhancing hydrophobic antifungal drugs' solubility, stability, and transungual penetration by encapsulating the latter in amphiphilic lipids. Due to their nano size, lipid nanocarriers enable deep diffusion of drugs through microchannels in the nail plate, unlike traditional topical formulations that are unable to penetrate the compact keratinized nail matrix. With a solid lipid core, SLNs inhibit burst release and ensure persistent therapeutic activity by regulating drug release via matrix degradation and diffusion processes. Because of this prolonged drug retention, fewer doses are required, a major drawback of traditional creams requiring multiple daily dosages. Moreover, unlike conventional remedies, which are unstable, SLNs protect encapsulated antifungal drugs from oxidation and enzymatic degradation, extending their shelf life. The partly amorphous matrix of NLCs, a modified version of SLNs, provides better stability and a greater drug-loading capacity by combining liquid and solid lipids. This structural flexibility improves medication absorption by decreasing crystallinity and reducing drug expulsion. Liquid lipids, which are absent from traditional topicals, modulate lipid fluidity and enhance diffusion in NLCs, further improving penetration through the hard structure of the nail. Additionally, through hydrophobic and van der Waals forces, lipid-based systems have robust interactions with keratin, enhancing adhesion and extending drug retention at the infection site. Hydrophobic antifungals are well soluble in nanoemulsions composed of water, oil, and surfactants. This ensures that the antifungals are well distributed and penetrate the nail bed. Their exceptionally tiny droplet size (less than 100 nm) improves surface area and promotes the absorption of drugs in contrast to conventional emulsions or ointments, which have limited penetration and spreading. Compared to conventional topical treatments that mostly stay on the nail surface, adding surfactants in nanoemulsions increases drug dispersion by further aiding in the disruption of the dense keratin network. Additionally, lipid-based nanoformulations reduce systemic toxicity, a significant disadvantage of oral antifungal treatments, which frequently result in gastrointestinal adverse effects and liver damage because of the extensive drug distribution. While retaining high therapeutic concentrations at the infection site, SLNs, NLCs, and nanoemulsions minimize systemic exposure by facilitating localized drug delivery. Furthermore, by ensuring regulated and prolonged drug release, these systems lessen the need for frequent dosing that traditional formulations need. Their therapeutic effectiveness is further increased by their capacity to modify medication partitioning and retention at the molecular level.
7.1 Mechanism of lipid-based formulations penetrating the nail plate
Nanocarriers facilitate transungual delivery by leveraging their small size to cross the minute spaces interspersed among keratin fibers within the nail plate and periungual skin, releasing the drug into the nail bed.34,133,134 This enables the potential disruption of the tight junctions within the nail plate and may be further enhanced using penetration enhancers that disrupt the disulfide bonds in keratin, allowing for the deeper infiltration of encapsulated drug molecules. The nanoscale dimensions allow them to navigate inter-fiber gaps within the nail plate, which are otherwise inaccessible to larger molecular entities. Lipid-based nanocarrier formulations integrate penetration enhancers, such as thiols (e.g., cysteine, glutathione) or sulfites, that facilitate the breakdown of disulfide bonds in keratin, thereby loosening the protein structure and promoting drug penetration.65,135 Additionally, some nanocarriers possess hygroscopic properties, enabling them to absorb moisture from the surrounding environment, resulting in slight hydration of the nail plate and subsequent enhancement of drug diffusion.136,137 Furthermore, the surface properties of nanocarriers can be meticulously engineered to interact favorably with the nail plate, thereby promoting adhesion and optimizing drug delivery. Ultimately, these advancements enable personalized medicine and tailoring treatment based on factors such as infection severity and medical history, thereby improving outcomes and satisfaction (Fig. 3).
7.2 Applications of different lipid-based formulation in onychomycosis
7.2.1 Microemulsion. MEs are thermodynamically stable transparent colloidal carriers with globule sizes ranging from 10 nm to 100 nm, composed of a lipid phase, aqueous phase, emulsifier, and co-emulsifier.138,139 MEs composition can facilitate the permeation of therapeutic agents across the skin and nails. The low viscosity of MEs is a significant challenge because of their minimal retention when applied topically. However, the retention of ME-based formulations can be improved by utilizing viscosity modifiers or gel formation.140 Barot and colleagues developed and optimized MEs using a D-optimal design. MEs were formed in the gel using a carbopol polymer. Permeation of the developed formulations was evaluated using human cadaver skin. The permeation of terbinafine from the MEs and MEs gels was about 4.2 and 2.7-fold higher, respectively, than those of the marketed formulation after 12 h. The higher permeation was attributed to the smaller globule size of MEs, which provides a larger surface area. The antifungal activity of the MEs gel also revealed promising results against C. albicans and T. rubrum compared to marketed formulations, confirming the potential of formulations for treating onychomycosis.141 Another study by Thatai and Sapra reported the delivery of terbinafine hydrochloride utilizing MEs and optimized it via a D-optimal design. Two different permeation enhancers, N-acetyl-L-cysteine (NAC) and urea, are used alone or in combination to improve drug permeation. The permeation study was performed on an animal hoof membrane, where the receptor medium was PBS (pH 5.8) containing 1% Tween 80. The MEs gel comprising both permeation enhancers showed improved drug retention, that is, ∼6.6-and ∼9.2 folds higher in the hoof than in the MEs and marketed formulations, respectively. This difference is attributed to the short residence time on the nail plate owing to the low viscosity of the MEs. At the same time, the oily base of the marketed cream formulation might have prevented the drug from being transported across the nail plate. The morphological characterization by SEM showed that untreated hooves showed smooth surfaces and with very few striations; water-treated hooves displayed swelled, and layer loosened; hooves treated with 5% urea or 5% NAC showed uneven surfaces with irregular pores, and pores even more numerous in the case of urea treatment and treated with a 1
:
1 mixture of urea. NAC showed increased disruption, bigger pore size, and more pronounced surface alterations, which suggested synergistic action between the two enhancers.142 Agrawal and colleagues developed and optimized a MEs formulation of TVB for the targeted treatment of onychomycosis, employing a 32 factorial design (Fig. 4-I). The study demonstrated that the cumulative permeation of TVB was significantly enhanced in the TVB-loaded microemulsion (TLM), exhibiting 2.0 times greater permeation than the reference formulation and 1.4 times greater than the TLM gel. TEM photograph is presented in Fig. 4-II; the reduced globule size of TLM facilitates deeper penetration into the nail matrix, while its lamellar microstructure supports sustained drug release, highlighting its superior efficacy. Results from nail clipping studies aligned with ex vivo bovine hoof membrane permeation data, indicating that the TLM gel's elevated viscosity might hinder nail penetration and hydration. The investigation confirmed that TLM exhibited significantly enhanced antifungal activity compared to reference formulations, with approximately 2.67 times greater inhibition against T. rubrum, 2.72 times against T. mentagrophytes, and 1.64 times against C. albicans. Results from nail clipping studies aligned with ex vivo bovine hoof membrane permeation data, indicating that the TLM gel's elevated viscosity might hinder nail penetration and hydration. Additionally, the TLM gel displayed significant antifungal potency, with 2.23 times greater inhibition for T. rubrum, 2.30 times for T. mentagrophytes, and 1.48 times for C. albicans, confirming the potential of these formulations.143 Agarwal and coworkers developed efinaconazole-loaded MEs and optimized them using 32 factorial designs, and their potential for transungual delivery was evaluated. In ex vivo permeability performed on the bovine hoof membrane, the permeability was significantly higher for both MEs and ME gel over 24 hours. This is considerably higher than the permeability measured for the reference drug formulation. The poor permeability of the free drug might be due to binding with nail keratin. The globules appeared dark in ME-based formulations due to the smaller globule size. Fig. 4-III showed a more significant concentration gradient in the nail-affected area. Antifungal activity of T. rubrum, T. mentagrophytes, and C. albicans. The ME formulations showed significantly better antifungal activity than the reference formulations. Moreover, cytotoxicity data reported no toxic effects of excipients evaluated in the Vero cell line compared to free API and control (Fig. 4-IV).144
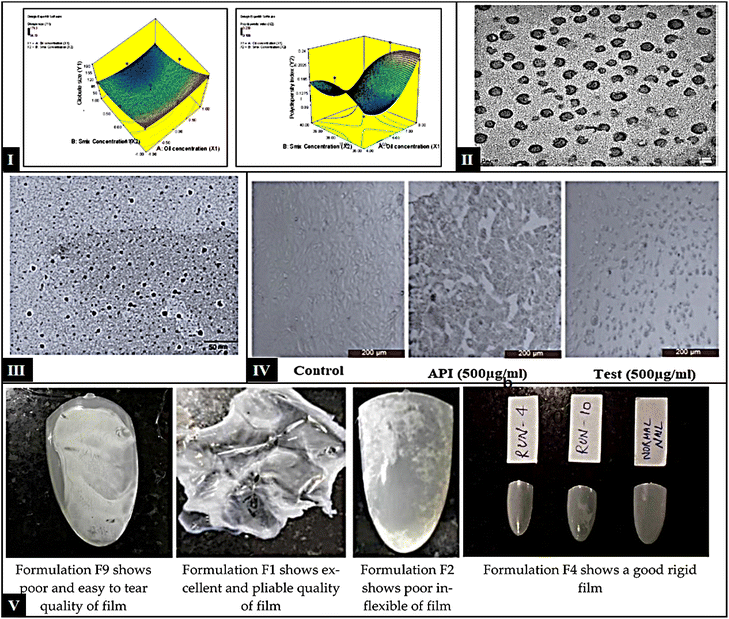 |
| Fig. 4 (I) 3D response–surface plots – globule size (left) and PDI (right); (II) TEM micrograph of TLM showing uniform and small size globules less than 50 nm (this figure has been reproduced from ref. Agrawal et al.143 with permission from Elsevier, copyright 2022); (III): TEM of developed efinaconazole MEs and (IV) cytotoxicity study of control, API, and test formulation (this figure has been reproduced from ref. Agrawal et al.144 with permission from Elsevier, copyright 2021). (V) TEM of Amorolfine HCl NEs(V) different films obtained during the optimization (the figure adapted from Fatima et al.145 under CC BY license). | |
7.2.2 Nanoemulsions and nanoemulgel. NEs serve as carriers to deliver therapeutic ingredients, characterized by droplet sizes ranging from 20 to 200 nm. Their excellent thermodynamic and kinetic stability makes them an ideal choice over other carrier systems.146–148 Such type of carrier requires a minimum concentration to stabilize and effective topical drug delivery attributed to their nano size, considerable surface area, and minimum surface tension, helping them to penetrate rapidly with enhanced efficacy and tolerability of antifungal drug even with the lower dose of therapeutic ingredient when applied topically.149–151 For improved topical drug delivery, NEs are suitable substitutes for lipidic vesicular carriers, including liposomes, noisomes, phytosomes, etc.NEs, as transungual delivery carriers, have come into view for the onychomycosis treatment, providing beneficial therapy without any side effects. A nanoemulgel can be formed by converting NEs into a gel. This provides added stability and prolongs the drug's contact time with the affected area. By incorporating penetration enhancers, such as thioglycolic acid, the permeation of the drug through the nail plate can be further enhanced. Mahtab and co-workers further developed 55 NEs for the delivery of ketoconazole and the optimized emulsion as nanoemulgel for the transungual delivery of the drug—the TEM images of NEs revealed the spherical shape of globules. The drug release from NEs (NE3), nanoemulgel (NEG1), and drug suspension was evaluated in phosphate buffer media using a dialysis membrane, showing a significant difference in drug release. The permeation was performed utilizing the membrane of the goat hoof. The NEG1 showed maximum permeation compared to NE3 and drug suspension. Comparative antifungal activity was performed on C. albicans and T. rubrum. After 48 hours of study, the ZOI was found to be approximately similar for both fungal strains in the case of NEG1, which is comparatively higher than the ZOI observed by applying drug suspension.152 A study reported by Fatima and coworkers elaborates on the development of NEs of amorolfine, and then nanoemulgel was prepared for optimized formulation utilizing thioglycolic acid as a permeation enhancer. The films developed (Fig. 4-V) for drug-free dual-component loaded nail lacquer contain HPC (5% w/v), Eudragit 100 RS (5% w/v), and ethanol
:
water (9
:
1) ml. The drug release from NEs-based nail liquor was observed in PBS and showed better sustained release than in the marketed formulation; the release kinetic study revealed formulations that followed the Higuchi model. The transungual permeation was evaluated on the human nails; the nanoemulgel showed improved permeation than the marketed formulation.145
7.2.3 Solid lipid nanoparticles (SLNs). SLNs became a viable alternative delivery vehicle because of their many advantages over liposomes, including integrating hydrophilic and lipophilic drugs, low skin irritation, improved stability for up to three years, controlled release, and lower costs.153–156 When they dry, SLNs form a film on the skin surface or nail, preventing water loss and enhancing delivery.157 This occlusion effect is not specific to SLNs but may be more pronounced because of their nanoscale size. The large surface area of SLNs allows for more contact between the drug and the SC and may also lead to prolonged retention of the drug in the skin layers, resulting in sustained release.158 NLCs have been developed due to some drawbacks of SLNs, like aggregation, relatively low drug loading, and storage stability. Tiwari and coworkers reported the development of a terbinafine hydrochloride gel formulation based on SLNs. SLNs were incorporated in chitosan gel and evaluated for antifungal activity and drug release. Terbinafine hydrochloride release from SLNs and their gel formulation confirmed sustained release. The SLN-based formulation enhanced antifungal efficacy against C. albicans compared to the marketed formulation.159 Abobakr et al. developed and characterized SLNs for the onychomycosis treatment where terbinafine hydrochloride was used as an antifungal agent. The SLN formulation was comparatively evaluated with the marketed cream Lamifen®. The in vitro antifungal activity of the SLN formulation showed a more significant zone of inhibition and significantly higher drug uptake than that of the marketed formulation. N2 showed 1.78 times while N8 showed 1.67 times ZOI, depicting significantly higher antifungal activity compared to marketed Lamifen cream. The SLN formulation also improved nail hydration, a critical requirement in onychomycosis treatment.160
7.2.4 Nanostructured lipid carriers. NLCs are second-generation lipid nanocarrier systems derived from o/w nanoemulsions. The NLCs comprise a blend of solid and liquid lipids that are biodegradable and compatible with each other and the drug.161 Using liquid lipids helps reduce the melting point of solid lipids and avoids the recrystallization of solid lipids. The liquid-to-solid lipid ratio can vary from 30
:
70 to 0.1
:
99.9. The NLCs have comparatively high drug loading and payload of lipophilic and hydrophilic drugs and prevent drug leakage from the NLCs matrix. The NLCs are stabilized in aqueous dispersion using an optimized surfactant concentration with or without co-surfactant.162,163 NLCs are categorized into three categories. NLCs are classified into three types based on their structure and composition. Type I, or imperfect crystal NLCs, are formed by mixing solid and liquid lipids, resulting in an imperfect lattice that allows higher drug loading by avoiding a highly ordered matrix. Type II, known as amorphous or structureless NLCs, are composed of medium-chain triglycerides with solid lipids, creating an amorphous core where drugs remain embedded, thus preventing crystallization and improving drug stability. Type III NLCs, or multiple types, are formulated by mixing solid lipids with oils, forming nano-compartments within the matrix, and utilizing the w/o/w emulsion concept to enhance drug loading capacity and stability.164–166 The investigation undertaken by Rocha et al. reported the development of NLCs utilizing urea as a permeation enhancer for delivering VOR. The in vitro permeation was tested on porcine hooves. The structural changes observed are represented in Fig. 5-I. Hooves without treatment show a smooth surface (Fig. 5-IA), whereas hydration (Fig. 5-IB) increased keratin expansion, leading to irregular surfaces and pores due to the weakening of van der Waals forces, hydrogen bonds, and ionic interactions. All these processes are further disrupted with VOR-NLC and VOR-NLC-Ur in Fig. 5-IC and ID, further increasing the roughness and porosity due to the destabilization of ionic interactions by cetylpyridinium chloride in keratin.167
 |
| Fig. 5 (I) SEM of surface of porcine hoof – (A) untreated; (B) water treated (C) with VOR-NLC treated; (D) VOR-NLC-Ur treated (this figure has been reproduced from ref. Rocha et al.167 with permission from Elsevier, copyright 2017); (II) TEM the NLC formulations (a) F 30 and (b) F 85 (the figure adapted from Pereira et al.168 under CC BY license). | |
Remarkably, successive extractions revealed a considerable increase in the concentration of VOR retained in deeper regions when VOR-NLC or VOR-NLC-Ur was used, suggesting the potential of NLCs for treating onychomycosis.167 Research conducted by Pereira et al., the authors reported optimizing and developing Ucuùba fat-based NLCs for delivering ketoconazole. The drug encapsulation was observed above 90%. Two optimized formulations with particle sizes 30 nm and 85 nm were preferred and characterized up to 30 days of storage at room temperature; the TEM photographs are depicted in Fig. 5-II, showing the spherical shape. Formulations remained stable over time despite initial drug loss during pre-emulsification and homogenization. The drug release of free drug solution and F 30 and F 85 were evaluated up to 24 h. NLC confirmed the controlled release of ketoconazole, with F 30 and F 80 releasing 81.3% and 45.0% of the drug after 24 hours, respectively. The lipid phase composition influenced particle size, PDI, drug entrapment, and drug release rate. The variation in the release was due to the variation in the composition of both formulations.168
7.2.5 Liposomes. Liposomes, as vesicular vehicles composed of phospholipids, play a considerable role in enhancing the drug(s) pervasion through the upper layer of the stratum corneum. Despite their efficacy in topical drug delivery, liposomes' limited ability to penetrate the skin's deeper layers constrains their use as transdermal delivery systems.169,170 Specifically, nanosized liposomes are employed as topical drug delivery systems, where their efficacy relies on several factors, including size, lipid and cholesterol content, ingredient percentage, lamellarity, and surface charge. Such characteristics allow liposomes to tailor their functions in topical drug delivery. Liposomes in the topical drug delivery system are attributed to their amphiphilic nature, differentiating them as excellent permeation enhancers for topical administration compared to conventional formulations.124,171,172 Due to their physicochemical properties and safety profiles, liposomes have been widely used to treat microbial infections. Liposomes comprise one or more phospholipid layers that encapsulate the aqueous phase. They can be surface engineered using polymer, targeting ligands, etc., to make them suitable for desired antifungal drug delivery applications. Liposomes can treat infections related to dermatophytes, including T. rubrum and C. albicans, and provide numerous benefits over conventional treatment by overcoming the emergence of resistant microorganisms and concerns associated with the drug's efficacy. They can be surface engineered using polymer, targeting ligands, etc., to make them suitable for desired antifungal drug delivery applications. Liposomes can treat infections related to dermatophytes, including T. rubrum and C. albicans, and provide numerous benefits over conventional treatment by overcoming the emergence of resistant microorganisms and concerns associated with the drug's efficacy. Treatments for nail disorders may become more effective when liposomes, which are lipophilic in nature, utilize the lipophilic pathways in the nail plate.173 Several antifungal therapeutic agents have previously been delivered using liposomes, including itraconazole, amphotericin, ketoconazole, etc. The empirical work conducted by Tuncay and colleagues documented their findings on the ability of liposome-loaded Eudragit films (LI-E) and pullulan films (LI-P) formulations to deliver terbinafine hydrochloride. It was characterized by antifungal activity in onychomycosis. Liposome film (LI-P), a thinner film formulation, showed comparatively high and faster drug release than other formulations. Ex vivo nail permeations were evaluated using the TOWL study. The TOWL of the nail was found to be increased due to swelling in each case. The lowest value was noted because the LI-P formulation had less impact on the nail. The day after the experiment, the nails dried, and the TOWL values returned to baseline. Nail permeation revealed drug accumulation in the nail, and after ten days, the drug was not found in the receptor compartment, confirming the potential of formulations for the first time; an in vivo antifungal experiment was executed to study the therapeutic efficacy of these preparations against T. mentagrophytes infected nails. After 5 weeks of infection, the fungal hyphae and spores were visible within the plate. After 6 weeks of treatment, all formulations showed considerable therapeutic effects; rarely, hyphae and spores were seen after treatment. Residual hyphae persisted with ethosome and ethosome chitosan gel formulations, but lesser spores persisted in liposome and liposome poloxamer formulations. The best result came with the formulation (LI-P), in which neither hyphae nor spores were detected.174 Shah and collaborator prepared liposomal formulation of nail lacquer of terbinafine HCl by optimizing them with the response surface method using BBD. Transungual permeability flux across human nail plate was notably higher in the case of novel liposomal formulation than nail lacquer comprising permeation enhancer. At the same time, similar results were observed in the case of cattle hoof, improving therapeutic efficiency. The higher permeation rate of the liposomal formulation was attributed to small vesicle size, hydration forces, and osmotic gradient. Antifungal activity confirmed that the formulation's efficacy was comparable to that of the pure drug solution.175 A comprehensive study by Tuncay, 2018 reported their observations on transungual drug delivery using liposomes and ethosomes to evaluate their permeation capabilities. The particle size of liposomes was found to be relatively higher than that of the ethosomes. The ex vivo permeation studies performed on human cadaver nails revealed the potential of ethosomes, which showed comparatively more permeation through nails than liposomes. The permeation results confirmed the potential of both formulations to enhance the drug permeation.173 Chouhan et al. (2021) developed nail liquor loaded with liposomes to deliver antifungal luliconazole. The liposomal suspension (LF) was prepared using a modified film hydration technique. The liposomal suspension showed maximum drug release compared to nail liquor. The antifungal activity against C. albicans showed a maximum of 47 mm ZOI compared to plain nail liquor of luliconazole, which showed 36 mm of ZOI.176
7.2.6 Invasomes. Invasomes represent a class of modified liposome carriers intended to improve drug penetration significantly. Liposomes have a rigid membrane, consist mainly of cholesterol and phospholipids, and have limited penetration into the skin. Structurally, invasomes consist of a bilayer membrane composed of soy phosphatidylcholine, lysophosphatidylcholine, terpenes, and ethanol.177–179 Each component specifically optimizes delivery efficiency: phosphatidylcholine acts as the primary bilayer-forming lipid, while lysophosphatidylcholine confers the membrane flexibility critical for adaptation to the skin microenvironment. Ethanol and terpenes act as powerful permeation enhancers, increasing the fluidity and elasticity of the bilayer, parameters directly linked to improved intradermal penetration capacity. Ethanol further contributes to the physicochemical stability of invasomes by inducing a strongly negative surface charge, effectively reducing vesicle aggregation via electrostatic repulsion.180,181 This stabilization minimizes vesicle fusion during storage, maintaining vesicle integrity and optimizing shelf-life. The unique compositional framework of invasomes enables efficient and sustained drug release and improves transdermal bioavailability, underscoring their utility in therapeutic interventions requiring enhanced ungual and dermal absorption.181 Hoda and collaborators developed valencene-modified invasomes to potentiate the transungual delivery of itraconazole (ITZ), with optimization conducted via the CCD approach. The ITZ-loaded invasome gel demonstrated exceptional homogeneity, with a viscosity of 7.33 ± 0.67 Pa s, and drug EE of 94.13 ± 1.13%. Spreadability and extrudability measurements yielded values of 7.85 ± 0.24 g cm s−1 and 162 ± 2.74 g, respectively, indicative of the gel's optimal rheological and application properties. Permeation studies using goat hoof membranes exhibited a cumulative permeation of 71.11 ± 3.65%, signifying superior penetration efficiency of the ITZ-loaded invasome gel across keratinized substrates. In vitro antifungal activity assays against T. rubrum revealed a significantly larger zone of inhibition for the invasome formulation (21.42 mm) compared to a commercial itraconazole gel (10.64 mm), underscoring its potent fungicidal activity. The enhanced efficacy is likely attributed to the invasome's ability to facilitate ITZ vesicular diffusion through fungal cell walls, consequently inhibiting ergosterol biosynthesis more effectively.182 To explore the potential of invasomes in transungual delivery, Gupta and colleagues developed linalool-containing invasomes for terbinafine (TBF-IN) delivery and optimized the formulation using the BBD approach. The optimized invasome formulation (TBF-INopt) showed a spherical sealed vesicle and EE of 74.23%. CLSM showed that TBF-IN penetrated the keratin layer of goat hooves to a depth of 56 μm, significantly more profound than the control TBF solution, which only reached 15 μm. Furthermore, the TBF-INopt formulation showed higher fluorescence intensity than the TBF suspension, indicating a more uniform distribution of TBF in deeper hoof layers, confirming improved drug permeation (Fig. 6-I). The TBF-INopt gel demonstrated significantly increased antifungal activity compared to the marketed terbinafine gel, as evidenced by a greater ZOI against both T. rubrum and C. albicans. In particular, the TBF-INopt gel demonstrated a 1.6-fold larger zone of inhibition against T. rubrum than the marketed gel. Against C. albicans, the TBF-INopt gel demonstrated a 2.3-fold increase in the zone of inhibition compared to the marketed gel. The control vehicle showed no inhibitory effect against any of the microorganisms. This suggests that the TBF-INopt gel formulation provides significantly superior antifungal efficacy.183
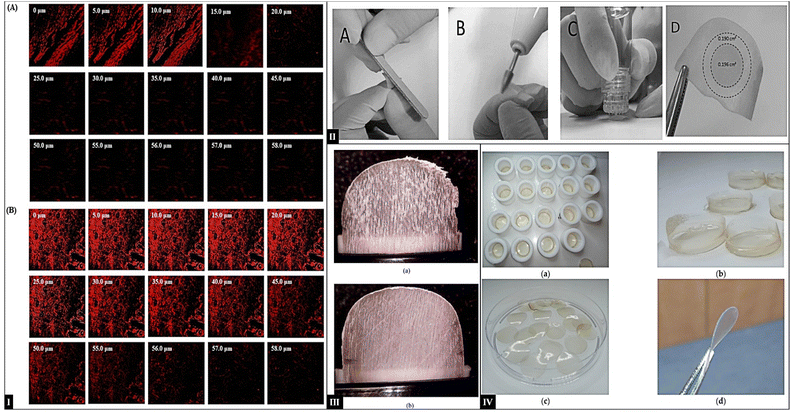 |
| Fig. 6 (I) CLSM images of goat hooves (A) TBF suspension treated and (B) TBF-INopt treated; (the figure adapted from Gupta et al. 183 under CC BY license); (II-A) manually sanding of nails; (II-B) microporation by electric filing; (II-C) poration using Hydra needle; (II-D) after IVPT nail sections to quantify CPO in the diffusional area and the outer ring (the figure adapted from Kishishita et al.184 under CC BY license); (III) comparison of membrane formed: (A) flaky membrane; (B) smooth membrane (this figure has been reproduced from ref. Bonetti et al. 185 with permission from Elsevier, copyright 2021); (IV) keratin films: (a) intermediate soft KF; (b) KF-40 before punching; (c) KF-40 after punching; and (d) KF-110 after curing (the figure adapted from Valkov et al.186 under CC BY license). | |
7.2.7 Transferosome. Transferosomes are deformable and stress-responsive lipid-based vesicles comprising phospholipids and edge activators. Edge activator is a membrane softening agent that helps to enable the ultra-deformable attributes and increases the lipid bilayer flexibility and permeability of the transferosome.187,188 Such properties allow transferosomes to dehydrate and deform to permeate even across the tiny pores and then recover their shape through the rehydration process.189 Driven by the transcutaneous water gradient, transferosomes can penetrate the permeability barrier of skin and nails.190 A study reported the potential of nanovesicles for trans-ungual drug delivery. The ethanol injection approach was used in the development of the formulation, optimized using response surface methodology, and evaluated for ex vivo nail permeation study. The permeation and retainment were assessed on a cadaver nail plate for 03 days, and the nanovesicle permeation was higher than the marketed formulation.191 Another study reported the liquid spray-based transfer formulation for terbinafine (TDT 067) delivery. The formulations were evaluated for their antifungal efficacy against 25 strains. The MI50 value of TDT 067 was 60-fold lower than terbinafine spray and eight-fold lower than free terbinafine solution. In vivo study of the morphological effects of TDT 067 in patients with onychomycosis, assessed through subungual samples following 28 days of topical administration. TEM analysis demonstrated significant morphological alterations, including the formation of large lipid droplets within fungal cells as early as 7 days, supporting in vitro observations. TEM revealed that TDT 067 induced rapid disruption of intracellular structures, with detectable changes evident within 5 minutes of exposure. Moreover, the investigation showed that terbinafine concentrations in nail debris were markedly lower post-treatment than those retained with oral terbinafine, indicating reduced binding affinity within the nail matrix. The findings emphasize TDT 067 capacity to effectively penetrate nail tissue and exert rapid antifungal activity, which may account for the elevated mycological cure rates observed in Phase-II open-label efficacy trials.190
7.2.8 Niosomes. Niosomes are a multifaceted vesicular vehicle composed of cholesterol and nonionic surfactants. Structurally, these are bilayered carriers wherein the hydrophilic head is exterior and interior-centric, whereas the hydrophobic tail resides between the hydrophilic heads. Therefore, the arrangements of components facilitate niosomes to deliver lipophilic drugs via lipid material and hydrophilic drugs via an aqueous core.169 Niosomes contribute several advantages, covering high drug loading ability, better stability, scalability, and lower formulation cost than liposomes.192 However, such properties depend on various conditions, including the formulation method, lipids concentration, and other ingredients used.192,193 Additionally, niosomes are osmotically active, non-toxic, non-immunogenic, biocompatible, and biodegradable. The functionality can be improved by overcoming biological complexity and therapeutic challenges associated with the niosomes. The niosomes can be formed multifunctionally by modification with targeting ligands, stimuli-responsive moiety, polyethylene glycol, etc. The multifunctional niosomes show improved therapeutic ability, which is attributed to the synergic benefits of linking several targeting mechanisms.194 A study reported the delivery of efinaconazole utilizing niosomes-based nail liquor. The developed niosomes were smaller in size, ranging between 95 nm to 135 nm. The niosomes-based liquor showed comparatively significantly more drug release than other formulations.195 Another study reported the antimycotic activity of itraconazole-loaded niosomes. The niosomes were tested for size, drug release, and antimycotic activity. The niosomes showed good permeation through the skin and were found to show comparatively improved antimycotic activity against C. albicans than that of the marketed formulation.196
8. Nail models for evaluating drug penetration
The permeability of drugs through the nail plate is assessed using various in vitro, and ex vivo models. To evaluate drug transport under controlled circumstances, ex vivo models use human or animal nails mounted in diffusion cells, whereas in vitro models usually use artificial membranes that mimic nail characteristics. Lipid-based nanocarriers, due to their ability to hydrate and disrupt intercellular lipid domains, facilitate deeper penetration, which is particularly useful in cadaver nail models where drug transport is inherently slow. These nanocarriers promote prolonged drug retention within the nail matrix, improving therapeutic outcomes in antifungal and antimicrobial treatments. Their adaptability to alternative models, such as bovine hoof membranes, compensates for differences in keratin density and disulfide bond content, ensuring comparable permeation kinetics. In human nail studies, inter-donor variability in thickness and composition can lead to inconsistent results, but lipid nanocarriers provide controlled and reproducible drug release, reducing variability. The slow drug diffusion across the nail necessitates highly sensitive analytical tools, and lipid nanocarriers improve drug solubilization, aiding in precise detection and quantification. Additionally, they enhance the bioavailability of poorly soluble drugs, ensuring higher therapeutic concentrations at the site of action. Their biocompatibility makes them ideal candidates for long-term drug delivery applications with minimal toxicity concerns. These advantages collectively improve the accuracy and reliability of drug permeation studies across different nail models, making lipid-based nanocarriers a valuable tool in transungual drug delivery research.
8.1 Human nail plate models
Acquiring human plates can be challenging, as nails are hardly available after nail loss or surgeries. If obtained, nail plates from different donors can vary in composition, thickness, and penetration kinetics, leading to less credible data. The tiny size and curved shape make it difficult to mount them on standard diffusion cells. Besides, drug transport through nails is typically slow and limited, necessitating compassionate, analytical tools to detect and quantify the minute amounts of drug permeating. While human nail plates offer valuable insight into transungual drug delivery, their limitations necessitate exploring alternative models and analytical techniques to enhance the accuracy and efficiency of drug permeation studies.
8.2 Cadaver nail
The cadaver nail provides the closest anatomical structure of a human nail and the actual dynamics of therapeutic agent permeation through the nail plate. The benefit of using this model is its ability to replicate the different nail conditions (like structural traits, thickness, and keratin content) of the patient with onychomycosis. They can be directly infected with fungi like T. rubrum, enabling research on antifungal agent efficacy within the actual disease context. However, cadaver nails necessitate ethical considerations, may require specific regulatory approvals, and can cause difficulties. Moreover, individual variations in donor health and age impact nail properties, leading to potential inconsistencies in data.197 Fingernails from corpses can be purchased from hospitals or tissue banks. They had to be stored at −80 °C.198 Tuncay (2018) developed liposomal and ethosomes formulations for transungual drug delivery. Human cadaver nails were used as a model in the ex vivo investigations to assess their penetration. The nail plates were allowed to acclimate to room temperature overnight. The nail plates were weighed, and their thickness was measured before the permeation experiments commenced.173 Kishishita and a coworker procured the human fingernail clippings with ethical approval, which were 8 mm long or more. The clippings were kept for further preparation for in vitro permeation IVPT and microbiological tests at −20 °C. Before performing the tests, two treatments were employed: non-prorated nails were soaked in water for 2 hours, and slight sanding was done, whereas prorated pores nails were also soaked in water, were sanded using an electric file, and microporated using a device with 0.60 mm titanium needles creating approximately 20 pores for testing (Fig. 6-IIA–C). In attempts to measure nail plate permeability, nails were swabbed with isopropyl alcohol. The central circular area of the diffusion region (Fig. 6-IID) was cut off, and the cut portions were used for the investigations. The cut samples were transferred into centrifuge tubes containing seventy percent ethanol and left in a shaking water bath for seven days. After that, the liquor was separated, and the samples were examined using the HPLC-UV method. To investigate the lateral transport of active substances, a ring of the surrounding nail cut off the diffusion region was also evaluated this area of diffusion.184
8.3 Bovine hoof membrane
Nails can be replaced by hooves in the studies that deal with permeation characteristics. Hooves, however, are, on average, lower than human nails in terms of periungual penetration enhancers. They are more permeable than human nails because of their relatively lower number of disulfide bonds.135 Bovine hoof membranes are models in permeability studies as these mimic natural nail conditions. While using Bovine membranes as a model, it is crucial to consider the differences between the bovine hoof membrane and the human nail plate.110 The primary source of hoof membranes is freshly slaughtered cattle. The hoof is typically soaked in water after the surrounding tissue has been removed, and membranes are then cut with a microtome to achieve the appropriate thickness. Since they are byproducts of slaughterhouses, bovine hooves are usually available and typically inexpensive or free, but human nails are difficult to source and costly. The potential of this model for applications other than permeability studies remains unexplored. The bovine hoof membranes have a less dense/porous keratin network, leading to less resistance to drug permeation. Incubation in water causes hoof membranes to swell up 36%, compared to 27% for human nail clippings.185,199 Furthermore, compared to human nails, hoof proteins probably contain fewer disulfide bonds and significantly fewer cysteine residues. Therefore, enhancers such as breaking keratin disulfide bonds on bovine hooves and nail plates can exhibit varied effects.199 Bonetti and their coworker worked on hoof membranes' production protocol and characterization. Hoof membranes from freshly slaughtered 3 years cattle were obtained, immersed in liquid nitrogen, and then coring with a 16 mm plug cutter, followed by slicing on a precision lathe, were cleaned with a 70% v/v ethanol solution and mix of benzalkonium chloride and isopropyl alcohol followed under controlled conditions for 3 days. The membranes (Fig. 6-III) were further classified as flaky, smooth, or borderline based on their morphological features, surface desquamation, and thickness.185 Temporiti and coworkers reported that membranes were acquired from freshly slaughtered cattle. The resulting hooves were soaked in liquid nitrogen to avoid deformation during core drilling and cutting. They were then cored with a plug cutter and cut into 800 μm long slices using a precision lathe. In their experiment, 28 membranes were chosen and sanitized using ethanol 70% v/v and a mixture of benzalkonium chloride, isopropyl alcohol, and water (0.4 g, 70, and 100 g distilled water, respectively). Membranes were kept at 25 °C and 40% RH. After that, the prepared membrane's mechanical characteristics and contact angle were assessed. Since the developed membrane proved successful, membranes with the appropriate mechanical properties, consistent morphology, and optimal thickness could be produced.200
8.4 Keratin biomembranes
These are artificial films produced from keratin, the critical constituent of human nails.201 They are usually prepared by extracting keratin from human hair and then reconstituting it under specific conditions. This results in membranes with structural and compositional similarities to natural nail plates.202,203 Compared to actual human nails, keratin bio-membranes are ethically obtained and available in consistent sizes, providing a controlled environment for assessing drug permeation. The composition and thickness of the keratin film can be adjusted to mimic different nail types and conditions, allowing researchers to conduct reproducible and reliable measurements and enhancing the model's accuracy.204 Valkov and colleagues reported a method for producing keratin films from human hair that can be used as a model for nail permeability study. The technique involves extracting keratin from human hair using a 1,4-dithiothreitol solution. The extracted keratin was filtered, dialyzed, and centrifuged to remove aggregates. The resulting solution was then dispensed into Teflon rings, and water was evaporated to form soft keratin films. The films were then punched and cured at 110 °C to form rigid keratin films Fig. 6-IV. The width of the films formed was approximately 67 μm. In FTIR investigations of human hair, nails, KF-110, and bovine hoof samples, characteristic absorption bands are amide A, I, II, and III. Samples of nails invariably have sensitive peaks of amide A centered at 3295–3390 cm−1 shifted due to hydrogen bonds with humidity in the samples. The films of human hair and KF-110 have identical spectra. Films of human hair and KF-110 miss one or two bands from nail-specific ones. Therefore, keratin films could be considered a good model for nails.186 While promising, keratin biomembranes still have limitations. They may not fully capture the complexity of the natural nail environment, and scaling up production for large-scale studies can be challenging.
9. Onychopharmacokinetics of antifungals
Onychopharmacokinetics focuses on understanding the dynamics of drug permeation through the nail and the factors influencing drug retention and efficacy in treating nail disorders (such as onychomycosis). Key factors influencing onychopharmacokinetics include the drug's molecular size, lipophilicity, formulation vehicle, and the condition of the nail itself, as any damage or alteration in the nail structure can affect drug penetration and distribution. Since the treatment efficacy is a function of the capability of topically applied antifungal agents to penetrate the strong keratin matrix of the nail and directly reach the infection site, different research studies have established a good correlation between the ability of the drug to permeate the nail and clinical efficacy in infections such as onychomycosis. PK/PD metrics include the fAUC/MIC ratio, fAUC for the unbound, free drug, and the efficacy coefficient proposed as a marker of clinical effectiveness during antifungal treatment. On this basis, through relationships established between PK/PD measures and clinical results, professionals and researchers can predict the success of therapy more accurately and improve dosing schedules with better patient outcomes in addressing fungal infections.205,206 Hui et al. examined the onychopharmacokinetics of the novel antifungal drug “ME1111” using a finite dose in vitro model. Compared to the MIC90 and MFC90 for major dermatophytes, ME1111 concentrations were higher in the deeper nail and cotton pad/nail bed. At 3 days after a single dose and 14 days of multiple doses of 10% ME1111, concentrations reached 253 and 7991 μg g−1 in nails, respectively, exceeding the values of 8% ciclopirox. ME1111 concentrations in the cotton pad increased linearly throughout the 336 hours experiment and were much higher than ciclopirox. With a flux rate of 50.9 μg per cm3 per day, ME1111 demonstrated superior penetration into the nail plate, significantly exceeding ciclopirox levels.207 Jarratt and coworkers examined the pharmacokinetics of a 10% efinaconazole solution and assessed potential DDI when applied topically to patients with onychomycosis and healthy volunteers. The mean Cmax of efinaconazole was 0.54 ng ml−1 in healthy volunteers and 0.67 ng ml−1 in patients. Its metabolite H3 reached 1.63 ng ml−1 and 2.36 ng ml−1, respectively. Both accumulated after repeated dosing, reaching a steady state by day 14. Efinaconazole showed very low systemic exposure and was well tolerated without adverse events. Despite being a CYP inhibitor, the Cmax/ki ratio of 0.007 suggests that efinaconazole 10% solution has a low potential for clinically significant DDIs.208 Finlay studied terbinafine permeation in 12 patients over 48 days with a 250 mg per day dose. Terbinafine levels of 0.25–0.55 ng mg−1 were quickly reached and remained stable, with similar concentrations in affected and unaffected nails. While these levels fall within the fungicidal range, the anatomy of infected nails may remain protected, leading to persistent or recurrent infections.209 In another study, Kubota-Ishida et al. studied the PK and PD of “ME1111”. In a 14 days in vitro model, ME1111 showed considerably higher concentrations in nails and cotton pads, unlike efinaconazole, ciclopirox, and amorolfine, similar to TVB. Deep nail layers and pads had free drug levels that were noticeably higher than T. rubrum MIC90. In contrast to other antifungals, ME1111 activity was not impacted by mild acidity (pH 5.0) or 5% human keratin. The antidermatophytic efficacy coefficient of ME1111 was substantially higher than that of other antifungals, both at pH 5.0 and pH 7.0.210 Krishna et al. explored the PK of POS in 146 adults with toenail onychomycosis. Participants received 100 mg, 200 mg, or 400 mg of POS once daily for 24 weeks or 400 mg daily for 12 weeks. POS concentrations in the toenails increased dose-dependently starting from week 2, with a toenail-to-plasma concentration ratio of roughly 3
:
1 by the end of the 24 weeks treatment at 400 mg. The study included all participants with baseline PK data and at least one posttreatment assessment. POS plasma concentrations increased with dose and remained steady once they reached a flat profile in the toenails. The drug was detectable from week 2 in the 200 mg and 400 mg groups, and concentrations continued to rise even after treatment ended, while plasma concentrations, as expected, declined after treatment cessation. The maximum toenail concentration (Tmax) was reached between 36 and 42 weeks.211
10. Clinical progress
Clinical trials for onychomycosis have improved knowledge of the safety and antifungal effectiveness of different formulations and have produced encouraging outcomes in patient populations specifically targeted, such as children and people with comorbid conditions. When treating fungal infections of the toenails, topical medications like efinaconazole and TVB have shown long-term effectiveness and excellent tolerability, meeting the need for safer substitutes for systemic treatments. Longer treatment times, frequently necessary for complete nail restoration, showed that such therapies could be optimized for extended use without posing serious safety risks and offered crucial information on recurrence rates.
Pannu et al. (2009) in their clinical trial demonstrated that NB-002, an oil-in-water nanoemulsion-based antifungal, exhibits broad-spectrum fungicidal activity against major dermatophytes (T. rubrum, T. mentagrophytes, Epidermophyton floccosum, and Microsporum spp.), several filamentous fungi, and both azole-susceptible and azole-resistant C. albicans. Unlike conventional antifungal drugs such as ciclopirox, terbinafine, and itraconazole, which were largely fungistatic, NB-002 displayed rapid fungicidal activity, effectively killing both mycelia and microconidia, including dormant fungal forms. The study also found low spontaneous resistance development, with minimal MIC variation in resistant mutants, suggesting a lower possibility of resistance emergence than conventional therapies. Electron microscopy revealed severe structural disruption in fungal cells exposed to NB-002, supporting its kill-on-contact mechanism. These findings underline NB-002 as a capable topical antifungal agent with superior efficacy and resistance-limiting potential.212 Firooz and colleagues (2023) conducted a pilot clinical study in 15 subjects (18 to 60 years of age) to assess the efficacy and safety of topical onychomycosis-resistant micrograms of amphotericin B 0.4 mg gel. Patients applied the gel twice daily for up to 36 weeks and were monitored at 12, 24, and 36 weeks. By week 24, 91.66% of patients had achieved complete and mycotic recovery, with only 8.33% not responding to treatment. The most common adverse reaction was temporary discoloration of the nails, while no systemic adverse reactions were reported. One patient had a nail fall in week 2, but was fully cured. These findings highlight that nanoliposomal amphotericin B is a highly effective and well tolerated alternative to systemic antifungal therapy and justify further extensive studies.213 In another trial, Moazeni et al. (2024) conducted a randomized clinical trial to evaluate the efficacy of a Zataria multiflora nanostructured lipid carrier (Zt-NLC) gel for Candida-associated onychomycosis. The study included 40 volunteers, aged 30 to 60 years, who were randomly assigned to receive either Zt-NLC gel or placebo for four weeks. The results showed a 97.5% mycological cure rate in the Zt-NLC group compared with 75% in the placebo group. Clinical assessments indicated a cure rate of 87.5%, which was significantly higher than that of placebo (67.5%). Patients treated with Zt-NLC gel experienced faster symptom relief, reduced pain, and higher satisfaction (75%). Additionally, the formulation exhibited enhanced drug permeation, sustained antifungal activity, and no cytotoxic effects, thereby confirming its safety and tolerability. These findings indicate that the Zt-NLC gel is a promising and patient-friendly alternative for the treatment of onychomycosis.214
Table 3 summarizes different clinical trial studies of the formulations for onychomycosis.
Table 3 Summary of phase 3 and 4 clinical trials for onychomycosis treatments
NCT number |
Formulation type |
Interventions |
Brief summary |
Enrolment |
Sponsor |
Drug(s) in clinical trial for onychomycosis completed phase 4 |
NCT03110029 |
Solution |
Efinaconazole 10% topical application solution, application of nail polish |
This trial was evaluated using efinaconazole solution (Jublia) to treat toenail fungal infections in patients with and without nail polish |
13 |
University of Alabama at Birmingham |
NCT03280927 |
Solution |
Jublia® |
Jublia® topical solution was tested for 48 weeks on patients with mild or moderate dermatophyte-induced onychomycosis to assess its antifungal effectiveness and safety |
97 |
Dong-A ST Co., Ltd |
NCT02464826 |
Solution |
Nailprotex |
The study was designed to demonstrate this product's efficacy in treating non-dermatophyte onychomycosis and chronic paronychia in Thai patients |
19 |
Mahidol University |
NCT03405818 |
Solution |
TVB 5% topical solution |
An open-label study tested TVB 5% topical solution on children and adolescents (ages 6 to 16) with toenail fungal infections. The solution was applied daily for 48 weeks after confirming eligibility. Safety and infection extent were monitored throughout, with additional assessments at 52 weeks |
55 |
Pfizer |
NCT03814343 |
Microemulsion |
Amphotericin B in 30% DMSO, 30% DMSO |
The purpose was to compare the safety and efficacy of amphotericin B in a 30% DMSO solution with that of a 30% DMSO solution alone in treating onychomycosis triggered by NDMs |
19 |
Mahidol University |
NCT03098615 |
Solution |
Jublia (efinaconazole 10% topical solution) |
The study examined the effects of Jublia on dermatophytomas, which were challenging to treat with other options |
19 |
University of Alabama at Birmingham |
NCT02812771 |
Solution |
Efinaconazole |
The study assessed the safety and PK of daily topical efinaconazole for treating pediatric toenail onychomycosis |
62 |
Bausch Health Americas, Inc. |
NCT01419847 |
Nail lacquer |
Ciclopirox, placebo |
A five-month course of therapy effectively addressed onychomycosis in children. Penlac nail lacquer's topical treatment proved as effective as systemic therapy, offering superior cost-effectiveness and safety |
40 |
Rady Children's Hospital, San Diego |
![[thin space (1/6-em)]](https://www.rsc.org/images/entities/char_2009.gif) |
Drug(s) in clinical trial for onychomycosis completed phase 3 |
NCT02547701 |
Solution |
P-3058 |
Pediatric patients with mild-to-moderate DSO or WSO caused by dermatophytes were treated topically with P-3058 nail solution according to the treatment schedule |
20 |
Polichem S.A. |
NCT02549001 |
Solution |
P-3058 10%, vehicle of P-3058 10%, amorolfine 5% |
The study tested whether the P-3058 nail solution safely and effectively treated onychomycosis |
953 |
Polichem S.A. |
NCT00781820 |
Cream |
Bifonazole cream 1%, placebo cream |
The study focused on demonstrating the superiority of 1% bifonazole cream over a placebo cream following non-surgical nail ablation using a 40% urea paste |
693 |
Bayer |
NCT00443820 |
Solution |
Terbinafine, placebo, terbinafine, placebo |
This study tested a daily topical terbinafine solution for toenail fungus, comparing 24 and 48 weeks treatments for mild to moderate cases on the big toe |
526 |
Novartis Pharmaceuticals |
NCT02859519 |
Solution |
MOB015B, MOB015B vehicle |
The study aimed to assess topical MOB015B's effectiveness and safety in patients with mild to moderate DSO |
365 |
Moberg Pharma AB |
NCT01007708 |
Solution |
IDP-108, vehicle |
The study aimed to compare IDP-108 and a vehicle in treating onychomycosis, evaluating both safety and efficacy |
780 |
Dow Pharmaceutical Sciences |
NCT03168841 |
Solution |
Efinaconazole |
Topical efinaconazole (10%) was tested for treating toenail onychomycosis in diabetic patients, and its efficacy and safety were assessed |
40 |
Western University of Health Sciences |
NCT01302119 |
Solution |
AN2690 topical solution, 5%, solution vehicle |
The study evaluated the safety and efficacy of AN2690 topical solution for treating toenail onychomycosis |
604 |
Pfizer |
NCT00459537 |
Nail lacquer |
Terbinafine hydrogen chloride, amorolfine nail lacquer |
The study compared 10% terbinafine applied daily to 5% amorolfine applied twice weekly for 48 weeks in patients with mild toenail onychomycosis |
1029 |
Novartis |
NCT02866032 |
Solution |
MOB015B, Ciclopirox 80 mg g−1 |
The study aimed to assess topical MOB015B's effectiveness and safety in mild to moderate DSO patients |
452 |
Moberg Pharma AB |
NCT00443898 |
Solution |
Terbinafine, placebo, terbinafine, placebo |
The study evaluated a daily topical terbinafine solution with mild to moderate toenail fungus, comparing two treatment durations: 24 or 48 weeks |
518 |
Novartis Pharmaceuticals |
NCT01008033 |
Solution |
IDP-108, vehicle |
The trial aimed to assess the efficacy of IDP-108, which was compared to a vehicle when applied topically to treat patients with onychomycosis |
870 |
Dow Pharmaceutical Sciences |
NCT01270971 |
Solution |
AN2690 topical solution, 5%, solution vehicle |
The trial aimed to assess if the AN2690 topical solution safely and effectively treats toenail onychomycosis |
594 |
Pfizer |
11. Regulatory considerations
Nanomaterials are controlled similarly to any other regulated medicinal product, which implies that similar standards and regulations are followed to ensure their quality, safety, and efficacy. Nanomaterials play various roles in drug formulations, such as active ingredients, excipients, drug carriers, or complexes. Liposomes are the most common nanomaterial used, followed by nanocrystals, emulsions, iron–polymer complexes, micelles, and other materials like drug–protein and polymeric nanoparticles.215 nanoparticles may serve in roles such as drug carriers or combination products that integrate both drug and device and/or theranostic functionalities. The FDA has outlined specific guidelines to accurately classify whether a product falls under a drug or a device category. Section 201(g) of the FD&C Act (21 USC 321(g)) describes “drug” and section 201(h) of the FD&C Act (21 USC 321(h)) describes “device”. These guidelines are based on evaluating both the inherent properties of the product and its intended application.216 Despite their distinct nanoscale characteristics, which may result in unique biological interactions, regulatory authorities often adopt existing frameworks used for conventional drug products. This approach involves rigorous preclinical and clinical evaluation, toxicity testing, manufacturing controls, and post-market surveillance to monitor potential risks. Special consideration is given to the nanoscale size and surface properties, as these can influence absorption, distribution, metabolism, excretion, and potential environmental impacts. GMP regulations present significant concerns regarding developing and producing nano drug delivery systems to ensure quality, safety, and efficacy due to the unique nature of nanoparticles. Important concerns are maintaining sterility, which is difficult with such tiny particles, and accurately characterizing the size, shape, and drug encapsulation. Strict control over raw materials and process validation is needed to ensure consistency.217,218 Technology transfer presents notable challenges, mainly when the manufacturers are not the original developers of the process. In such cases, there can be a frequent lack of understanding regarding process performance, highlighting gaps that can emerge during the transfer process.219 To this end, the FDA guidance on drug products containing nanomaterials suggests the basis for assessing the safety, efficacy, and quality of such drug and biological products in which a nanomaterial plays an active role as an ingredient or carrier. The FDA further suggests monitoring nanomaterials' interaction with the biological system, particularly pharmacokinetics, biodistribution, and toxicity. Control over the manufacturing process will be essential to ensuring a quality product, whereas nonclinical and clinical studies should demonstrate how nanomaterials affect ADME. The FDA encourages a total case-by-case assessment to mitigate risks and ensure the safety and efficacy of nanomaterial-containing drug products.220–222
12. Conclusion and foresightful opportunities
Lipid-based drug delivery systems signify a growing approach to the treatment of onychomycosis, a disease that has proven to be persistent and difficult to treat effectively. Advanced lipid-based formulations have shown considerable potential to tackle significant treatment barriers, including MEs, NEs, SLNs, NLCs, liposomes, transfersomes, and niosomes. These enhance drug penetration, prolong the drug retention in the infected nail bed, and enable sustained delivery of antifungal agents directly to the site of infection. Clinical data shows that they can increase both nail permeation and therapeutic efficiency compared to conventional approaches, representing a promising option for treating this disease. Despite these advances, numerous challenges must be resolved to maximize the therapeutic potential of lipid-based systems. Optimization of formulation components, including the selection of lipids, co-solvents, and surfactants, remains critical to attaining desired physicochemical properties and compatibility with specific drugs. Scalability of manufacturing processes is also essential to confirm reproducibility and maintain product quality for large-scale production. Regulatory requirements for complex formulations add further complications and require robust safety, efficacy, and quality data to support clinical use. While the high penetration ability of these nanocarriers is beneficial for targeted drug delivery, it also poses the risk of systemic exposure if not adequately controlled. This concern is particularly relevant for antifungal therapies, where inadvertent systemic exposure could lead to side effects or drug resistance. Preclinical and clinical evaluations are essential to address these safety issues and ensure that formulations are practical and patient-friendly. In addition, surface modifications can be explored to increase stability, regulate drug release and optimize permeation rates, thus enabling a controlled and targeted therapeutic approach. By eliminating the limitations of current treatments, lipid-based drug delivery systems offer several advantages, including shorter treatment durations, improved patient adherence, and lower recurrence rates. Their nanoscale size allows them to penetrate nails and surrounding skin via mechanisms such as intercellular pathways, hydration-induced pathways and fusion with lipid bilayers, ensuring precise drug delivery to infected sites while minimizing systemic side effects. These innovative systems improve patient outcomes and reduce the overall burden on healthcare resources. Manufacturing and cost remain critical factors in the widespread adoption of these advanced systems. While their initial development costs may be higher, recent cost-effectiveness analyzes suggest that lipid-based therapies may reduce long-term costs.
Abbreviation
BBD | Box–Behnken design |
bioAgNPs | Biogenic silver nanoparticles |
C. albicans | Candida albicans |
CLSM | Confocal laser scanning microscopy |
DDI | Drug–drug interactions |
EE | Encapsulation efficiency |
fAUC | Area under the concentration–time curve |
FD&C | Food drug and cosmetics |
FDA | Food drug and administration |
GMP | Good manufacturing practices |
ICH | International Council for Harmonisation of Technical Requirements for Pharmaceuticals for Human Use |
IVPT | In vitro permeation test |
KF | Keratin films |
LLLT | Low-level laser therapy |
MEs | Microemulsion |
MIC | Minimum inhibitory concentration |
NDMs | Nondermatophyte moulds |
NEs | Nanoemulsion |
NLCs | Solid lipid nanocarriers |
OEO | Oregano essential oil |
OTC | Over-the-counter |
PD | Pharmacodynamics |
PDI | Polydispersity Index |
PDT | Photodynamic therapy |
PK | Pharmacokinetics |
POS | Posaconazole |
REO | Rosemary essential oil |
RH | Relative humidity |
SCIO | Scoring clinical index for onychomycosis |
SEM | Scanning electron microscopy |
SLNs | Nanostructured lipid carriers |
T. mentagrophytes | Trichophyton mentagrophytes |
T. rubrum | Trichophyton rubrum |
TEM | Transmission electron microscopy |
TOWL | Transbronchial water loss |
TVB | Tavaborole |
VOR | Voriconazole |
ZOI | Zone of inhibition |
Data availability
No primary research results, no new data were generated or analysed as part of this review.
Author contribution
Shiv Kumar Prajapati: conceptualization, data curation, methodology, investigation, formal analysis, writing – original draft. Meenakshi Bajpai: supervision, writing – original draft, writing – review and editing. Ankit Jain: data curation, writing – original draft, writing – review and editing, supervision.
Conflicts of interest
The authors disclose no financial or non-financial interests that may affect the publication process for this manuscript.
Acknowledgements
We would like to thank the Birla Institute of Technology and Science, Pilani, for providing generous financial support, which made it possible to publish this article as open access.
References
- J. M. Falotico and S. R. Lipner, Clin., Cosmet. Invest. Dermatol., 2022, 15, 1933–1957 CrossRef PubMed.
- F. Farheen, H. K. Yadav, A. Raizaday and K. Dhakad, J. Pharm. Negat. Results, 2022, 3669–3690 CAS.
- S. Chakraborty, Sanshita and I. Singh, Expert Opin. Ther. Pat., 2023, 33, 613–630 Search PubMed.
- E. Axler and S. R. Lipner, Infect. Drug Resist., 2024, 17, 819–843 CrossRef PubMed.
- A. K. Gupta, B. Elewski, W. S. Joseph, S. R. Lipner, C. R. Daniel, A. Tosti, E. Guenin and M. Ghannoum, Mycoses, 2024, 67, e13683 Search PubMed.
- C. Petrokilidou, G. Gaitanis, I. D. Bassukas, A. Velegraki, E. Guevara, M. Z. Vardaki and N. Kourkoumelis, Appl. Sci., 2020, 10, 2340 CrossRef CAS.
- N. Ferara, S. Špoljar, L. Lugović-Mihić, A. Gverić Grginić, V. Rezo Vranješ, I. Bešlić, J. Perović and T. Regović Džombeta, Life, 2024, 14, 154 CrossRef CAS PubMed.
- S. Rather, A. Keen, F. Y. Shah, A. Yaseen, S. Farooq and A. Bakhshi, Indian J. Dermatol., 2021, 66, 132–137 CrossRef PubMed.
- N. Maskan Bermudez, G. Rodríguez-Tamez, S. Perez and A. Tosti, J. Fungi, 2023, 9(5), 559 CrossRef CAS PubMed.
- N. Mendoza, A. Arora, C. A. Arias, C. A. Hernandez, V. Madkam and S. K. Tyring, in Clinical Mycology, Elsevier, 2009, pp. 509–523 Search PubMed.
- S. Campos and A. Lencastre, in Imaging in Dermatology, ed. M. R. Hamblin, P. Avci and G. K. Gupta, Academic Press, Boston, 2016, pp. 43–58, DOI:10.1016/B978-0-12-802838-4.00005-4.
- P. Chang, Z. M. Q. Ucelo and H. M. Pilar Garzaro Chávez, Curr. Fungal Infect. Rep., 2017, 11, 252–257 CrossRef.
- H. S. Maji, R. Chatterjee, D. Das and S. Maji, in Viral, Parasitic, Bacterial, and Fungal Infections, ed. D. Bagchi, A. Das and B. W. Downs, Academic Press, 2023, pp. 625–644, DOI:10.1016/B978-0-323-85730-7.00059-X.
- A. M. Ibrahim, H. M. Morsi, N. S. I. Elsayed and E. S. Elgharabawy, J. Pharm. Negat. Results, 2023, 320–325 CAS.
- M. Pal, P. Dave, K. Dave, K. P. Gutama, L. Thangavelu, C. R. Paula and D. P. Jr Leite, Am. J. Microbiol. Res., 2023, 11, 19–24 CrossRef CAS.
- W. T. Frazier, Z. M. Santiago-Delgado and K. C. Stupka 2nd, Am. Fam. Physician, 2021, 104, 359–367 Search PubMed.
- S. Nikitha, N. Kondraganti and V. Kandi, Cureus, 2022, 14, e29765 Search PubMed.
- G. V. Research, Onychomycosis Market Size, Share & Trends Analysis Report by type (Distal subungual, white superficial, proximal subungual onychomycosis), by treatment (Oral, Topical), by region, and segment Forecasts, 2024 - 2030, https://www.grandviewresearch.com/industry-analysis/onychomycosis-market-report, accessed 12/10/2024.
- S. R. Lipner and R. K. Scher, J. Am. Acad. Dermatol., 2019, 80(4), 835–851 CrossRef PubMed.
- Y. Liu, Y. Liang, J. Yuhong, P. Xin, J. L. Han, Y. Du, X. Yu, R. Zhu, M. Zhang, W. Chen and Y. Ma, Drug Des., Dev. Ther., 2024, 18, 1469–1495 CrossRef PubMed.
- S. Nene, S. Shah, N. Rangaraj, N. K. Mehra, P. K. Singh and S. Srivastava, J. Drug Delivery Sci. Technol., 2021, 62, 102397 CrossRef CAS.
- G. D. Nagasa and A. Belete, J. Multidiscip. Healthc., 2022, 15, 1819–1840 Search PubMed.
- J. P. Sundberg, L. E. King Jr and R. V. Kuiper, in Pathology of Genetically Engineered and Other Mutant Mice, 2021, pp. 159–212, DOI:10.1002/9781119624608.ch10.
- L. Farren, S. Shayler and A. R. Ennos, J. Exp. Biol., 2004, 207, 735–741 CrossRef CAS PubMed.
- B. Richert, N. Di Chiacchio, M. Caucanas and N. G. Di Chiacchio, in Management of Ingrowing Nails: Treatment Scenarios and Practical Tips, ed. B. Richert, N. Di Chiacchio, M. Caucanas and N. G. Di Chiacchio, Springer International Publishing, Cham, 2016, pp. 1–34, DOI:10.1007/978-3-319-30555-4_1.
- N. Zaias, 2014.
- A. Hosseini-Tabatabaei, in Encyclopedia of Toxicology, ed. P. Wexler, Academic Press, Oxford, 3rd edn, 2014, pp. 429–431, DOI:10.1016/B978-0-12-386454-3.00633-3.
- O. S. Malallah, L. Coleman, S. M. Nasereddin, M. Lockhat, T. Chen and S. A. Jones, Drug Discov. Today, 2024, 29, 103844 CrossRef CAS PubMed.
- T. Kreutz, S. P. de Matos and L. S. Koester, Recent Pat. Drug Delivery Formulation, 2019, 13, 203–218 CrossRef CAS PubMed.
- H. H. Bragulla and D. G. Homberger, J. Anat., 2009, 214, 516–559 CrossRef CAS PubMed.
- A. Brahs and S. Bolla, Histology, Nail, StatPearls Publishing, Treasure Island (FL), 2023 Search PubMed.
- A. Chauhan, N. Nainwal, M. Butola and V. Jakhmola, Philipp. J. Sci., 2023, 152, 1895–1909 Search PubMed.
- M. V. Saner, A. D. Kulkarni and C. V. Pardeshi, J. Drug Targeting, 2014, 22, 769–789 CrossRef CAS PubMed.
- V. B. Rajendra, A. Baro, A. Kumari, D. L. Dhamecha, S. R. Lahoti and S. D. Shelke, J. Appl. Pharm. Sci., 2012, 203–209 Search PubMed.
- S. Baswan, G. B. Kasting, S. K. Li, R. Wickett, B. Adams, S. Eurich and R. Schamper, Mycoses, 2017, 60, 284–295 CrossRef PubMed.
- A. K. C. Leung, J. M. Lam, K. F. Leong, K. L. Hon, B. Barankin, A. A. M. Leung and A. H. C. Wong, Recent Pat. Inflammation Allergy Drug Discovery, 2020, 14, 32–45 CAS.
- K. Pakshir, T. Mohamadi, H. Khodadadi, M. Motamedifar, K. Zomorodian, S. Alipour and M. Motamedi, Curr. Med. Mycol., 2016, 2, 9–14 CrossRef CAS PubMed.
- B. Bhattacharjee, N. Dey, D. Barman, A. Karmakar and N. Ahmed, J. Drug Deliv. Therapeut., 2021, 11, 116–131 CrossRef.
- D. K. Mercer and C. S. Stewart, Med. Mycol., 2018, 57, 13–22 CrossRef PubMed.
- K. Adamczyk, A. Garncarczyk and D. Wcisło-Dziadecka, J. Cosmet. Dermatol., 2023, 22, 2774–2779 CrossRef PubMed.
- A. Singal and D. Khanna, Indian J. Dermatol., Venereol. Leprol., 2011, 77, 659 CrossRef PubMed.
- HealthlinkBC, Fungal nail infections, https://www.healthlinkbc.ca/illnesses-conditions/infectious-diseases/fungal-nail-infections, accessed 10-03-2024.
- A. B. Macura, A. Macura-Biegun and B. Pawlik, Comp. Immunol. Microbiol. Infect. Dis., 2003, 26, 223–232 CrossRef PubMed.
- S. Wu, W. Guo, B. Li, H. Zhou, H. Meng, J. Sun, R. Li, D. Guo, X. Zhang, R. Li and W. Qu, Front. Cell. Infect. Microbiol., 2023, 13, 1142029 CrossRef CAS PubMed.
- C. B. Costa-Orlandi, J. C. O. Sardi, N. S. Pitangui, H. C. De Oliveira, L. Scorzoni, M. C. Galeane, K. P. Medina-Alarcón, W. C. M. A. Melo, M. Y. Marcelino, J. D. Braz, A. M. Fusco-Almeida and M. J. S. Mendes-Giannini, J. Fungi, 2017, 3, 22 Search PubMed.
- A. K. Gupta, D. Daigle and J. L. Carviel, J. Am. Acad. Dermatol., 2016, 74, 1241–1246 CrossRef CAS PubMed.
- A. K. Gupta and K. A. Foley, G. Ital. Dermatol. Venereol., 2019, 154, 50–55 Search PubMed.
- R. Elkeeb, A. AliKhan, L. Elkeeb, X. Hui and H. I. Maibach, Int. J. Pharm., 2010, 384, 1–8 Search PubMed.
- E. Ranjan, S. Arora and N. Sharma, Indian J. Dermatol., Venereol. Leprol., 2023, 89, 47–53 CrossRef PubMed.
- D. Abadi and V. Zderic, J. Ultrasound Med., 2011, 30, 1723–1730 CrossRef PubMed.
- S. M. Z. Uddin, D. E. Komatsu, T. Motyka and S. Petterson, J. Clin. Med., 2021, 10, 2698 CrossRef CAS PubMed.
- K. Chen, V. Puri and B. Michniak-Kohn, AAPS J., 2021, 23, 25 CrossRef PubMed.
- J. Hao and S. Kevin Li, J. Pharm. Sci., 2008, 97, 893–905 CrossRef CAS PubMed.
- A. Kushwaha, H. Shivakumar and S. N. Murthy, Drug Dev. Ind. Pharm., 2016, 42, 1678–1682 CrossRef CAS PubMed.
- P. C. Pandey, S. Shukla, S. A. Skoog, R. D. Boehm and R. J. Narayan, Sensors, 2019, 19, 1028 CrossRef CAS PubMed.
- T. Angelo, L. N. Borgheti-Cardoso, G. M. Gelfuso, S. F. Taveira and T. Gratieri, Med. Mycol., 2016, 55, 461–475 Search PubMed.
- M. A. Repka, P. K. Mididoddi and S. P. Stodghill, Int. J. Pharm., 2004, 282, 95–106 CrossRef CAS PubMed.
- W. H. Abd-Elsalam and S. M. Abouelatta, AAPS PharmSciTech, 2023, 24, 150 CrossRef PubMed.
- J. K. Behera, S. Kumar, R. Sharma, A. Jain, N. K. Garg, A. Khopade, K. K. Sawant, R. Singh and P. Nirbhavane, Adv. Drug Deliv. Rev., 2023, 115174 Search PubMed.
- S. Kaur, M. Kumar, A. Bhatia and S. Chopra, J. Dispersion Sci. Technol., 2024, 1–20 Search PubMed.
- B. A. Witika, L. L. Mweetwa, K. O. Tshiamo, K. Edler, S. K. Matafwali, P. V. Ntemi, M. T. R. Chikukwa and P. A. Makoni, J. Pharm. Pharmacol., 2021, 73, 1427–1441 CrossRef PubMed.
- S. Kreijkamp-Kaspers, K. Hawke, L. Guo, G. Kerin, S. E. Bell-Syer, P. Magin, S. V. Bell-Syer and M. L. van Driel, Cochrane Database Syst. Rev., 2017, 7, Cd010031 Search PubMed.
- F. Crawford, P. Young, C. Godfrey, S. E. M. Bell-Syer, R. Hart, E. Brunt and I. Russell, Arch. Dermatol., 2002, 138, 811–816 CAS.
- R.
K. Dhamoon, H. Popli and M. Gupta, Pharm. Nanotechnol., 2019, 7, 24–38 CrossRef CAS PubMed.
- R. Aggarwal, M. Targhotra, P. K. Sahoo and M. K. Chauhan, J. Drug Delivery Sci. Technol., 2020, 57, 101774 CrossRef CAS.
- P. Halteh, R. K. Scher and S. R. Lipner, Cutis, 2016, 98, E16–e25 Search PubMed.
- M. Mustafa, M. El-Samahy, H. Diab and S. Bendary, Mycobact. Dis., 2017, 7, 2161 Search PubMed.
- P. d. S. Costa, V. Mendes, F. F. Veiga, M. Negri and T. I. E. Svidzinski, Microb. Pathog., 2022, 169, 105640 CrossRef CAS PubMed.
- H. Davies-Strickleton, J. Cook, S. Hannam, R. Bennett, A. Gibbs, D. Edwards, C. Ridden, J. Ridden and D. Cook, PLoS One, 2020, 15, e0229414 CrossRef CAS PubMed.
- N. M. Martinez-Rossi, N. T. Peres, T. A. Bitencourt, M. P. Martins and A. Rossi, J. Fungi, 2021, 7, 629 CrossRef CAS PubMed.
- N. A. R. Gow, C. Johnson, J. Berman, A. T. Coste, C. A. Cuomo, D. S. Perlin, T. Bicanic, T. S. Harrison, N. Wiederhold, M. Bromley, T. Chiller and K. Edgar, Nat. Commun., 2022, 13, 5352 CrossRef CAS PubMed.
- C. M. Hossain, L. K. Ryan, M. Gera, S. Choudhuri, N. Lyle, K. A. Ali and G. Diamond, Encyclopedia, 2022, 2, 1722–1737 CrossRef.
- S. R. Jartarkar, A. Patil, Y. Goldust, C. J. Cockerell, R. A. Schwartz, S. Grabbe and M. Goldust, J. Fungi, 2021, 8, 39 Search PubMed.
- N. M. Martinez-Rossi, T. A. Bitencourt, N. T. A. Peres, E. A. S. Lang, E. V. Gomes, N. R. Quaresemin, M. P. Martins, L. Lopes and A. Rossi, Front. Microbiol., 2018, 9, 1108 CrossRef PubMed.
- J. M. Q. B. Bersano, M. G. Cordeiro and F. A. L. Marson, Front. Med., 2023, 10, 1268324 CrossRef PubMed.
- J. K. Christenson, G. M. Peterson, M. Naunton, M. Bushell, S. Kosari, K. E. Baby and J. Thomas, J. Fungi, 2018, 4, 87 CrossRef CAS PubMed.
- D. K. Mercer, J. C. Robertson, L. Miller, C. S. Stewart and D. A. O'Neil, Med. Mycol., 2020, 58, 1064–1072 CrossRef CAS PubMed.
- J. A. Winston and J. L. Miller, Clin. Diabetes, 2006, 24, 160–166 CrossRef.
- Y. Grigorov, S. Philipov, J. Patterson, G. Tchernev, S. Gianfaldoni, T. Lotti and U. Wollina, Open Access Maced. J. Med. Sci., 2017, 5, 480–482 CrossRef PubMed.
- P. Tuchinda, W. Boonchai, P. Prukpaisarn, C. Maungprasat and P. Suthipinittharm, J. Med. Assoc. Thai., 2006, 89, 1249–1252 Search PubMed.
- A. Fukunaga, K. Washio, K. Ogura, K. Taguchi, K. Chiyomaru, Y. Ohno, T. Masaki, H. Nagai, T. Nagano and M. Oka, Acta Derm. Venereol., 2013, 93, 747–748 CrossRef PubMed.
- M. Eba, A. L. Njunda, R. N. Mouliom, E. T. Kwenti, A. N. Fuh, G. T. Nchanji and J. Atashili, BMC Res. Notes, 2016, 9, 494 CrossRef PubMed.
- N. C. P. d. O. P. Alves, T. d. A. Moreira, L. D. S. Malvino, J. J. Rodrigues, R. Ranza, L. B. d. Araújo and R. d. S. Pedroso, Dermatol. Res. Pract., 2020, 2020, 7209518 Search PubMed.
- J. W. Ricardo and S. R. Lipner, Expet Opin. Drug Saf., 2020, 19, 1395–1408 CrossRef CAS PubMed.
- F. Etgü, Cureus, 2023, 15, e44914 Search PubMed.
- R. I. Henkin, Drug Saf., 1994, 11, 318–377 Search PubMed.
- C. Adams, E. Athanasoula, W. Lee, N. Mahmudova and T. C. Vlahovic, J. Fungi, 2015, 1, 211–216 CrossRef PubMed.
- A. K. Gupta, M. Venkataraman, H. J. Renaud, R. Summerbell, N. H. Shear and V. Piguet, Skin Appendage Disord., 2021, 7, 351–358 CrossRef PubMed.
- A. K. Gupta, M. Venkataraman and M. Talukder, Drugs Aging, 2022, 39, 191–198 CrossRef CAS PubMed.
- D. S. Loo, Drugs Aging, 2007, 24, 293–302 CrossRef CAS PubMed.
- G. Singh, N. S. Haneef and A. Uday, Indian J. Dermatol., Venereol. Leprol., 2005, 71, 386–392 CrossRef PubMed.
- R. K. Scher and R. Baran, Br. J. Dermatol., 2003, 149, 5–9 Search PubMed.
- F. Yousefian, C. Smythe, H. Han, B. E. Elewski and M. Nestor, J. Clin. Aesthet. Dermatol., 2024, 17, 24–33 Search PubMed.
- C. Viegas, A. B. Patrício, J. M. Prata, A. Nadhman, P. K. Chintamaneni and P. Fonte, Pharmaceutics, 2023, 15, 1593 CrossRef CAS PubMed.
- R. L. Ball, P. Bajaj and K. A. Whitehead, Int. J. Nanomedicine, 2017, 12, 305–315 CrossRef CAS PubMed.
- G. F. Boafo, K. T. Magar, M. D. Ekpo, W. Qian, S. Tan and C. Chen, Int. J. Mol. Sci., 2022, 23, 12487 CrossRef CAS PubMed.
- S. Javed, B. Mangla, Y. Almoshari, M. H. Sultan and W. Ahsan, Nanotechnol. Rev., 2022, 11, 1744–1777 CrossRef CAS.
- B. Moloney, Lipid nanoparticle (LNP) manufacturing: Challenges & Solutions, https://www.susupport.com/knowledge/biopharmaceutical-products/drug-delivery/lipid-nanoparticle-manufacturing-challenges-solutions#:%7E:text=Scalinguptheproductionof,foron%2Dsitecleaningprocesses, accessed 01/03, 2025.
- J. Zhang, The Unique Challenges of Lipid Nanoparticle Development and Manufacturing, https://cellculturedish.com/the-unique-challenges-of-lipid-nanoparticle-development-and-manufacturing/, accessed 01/03, 2025.
- K. Osouli-Bostanabad, S. Puliga, D. R. Serrano, A. Bucchi, G. Halbert and A. Lalatsa, Pharmaceutics, 2022, 14, 1940 CrossRef CAS PubMed.
- H. E. Thu, M. Haider, S. Khan, M. Sohail and Z. Hussain, OpenNano, 2023, 14, 100190 CrossRef CAS.
- S. M. Moghimi and D. Simberg, Mol. Ther., 2022, 30, 2109–2110 CrossRef CAS PubMed.
- U. Havelikar, K. B. Ghorpade, A. Kumar, A. Patel, M. Singh, N. Banjare and P. N. Gupta, Discover Nano, 2024, 19, 165 CrossRef CAS PubMed.
- A.-H. Olfati, A. Safary, M. Akbarzadeh-Khiavi and K. Adibkia, J. Drug Delivery Sci. Technol., 2023, 87, 104844 CrossRef CAS.
- V. Francia, R. M. Schiffelers, P. R. Cullis and D. Witzigmann, Bioconjugate Chem., 2020, 31, 2046–2059 CrossRef CAS PubMed.
- T. Korzun, A. S. Moses, P. Diba, A. L. Sattler, O. R. Taratula, G. Sahay, O. Taratula and D. L. Marks, Pharmaceuticals, 2023, 16, 1088 CrossRef CAS PubMed.
- Y. Zhou, Q. Ge, X. Wang, Y. Wang, Q. Sun, J. Wang, T. Yang and C. Wang, ChemMedChem, 2025, e202400938 CrossRef PubMed.
- K. A. Walters, G. L. Flynn and J. R. Marvel, J. Pharm. Pharmacol., 1983, 35, 28–33 Search PubMed.
- Y. Kobayashi, T. Komatsu, M. Sumi, S. Numajiri, M. Miyamoto, D. Kobayashi, K. Sugibayashi and Y. Morimoto, Eur. J. Pharmaceut. Sci., 2004, 21, 471–477 CrossRef CAS PubMed.
- M. M. A. Elsayed, J. Controlled Release, 2015, 199, 132–144 CrossRef CAS PubMed.
- K. A. Smith, J. Hao and S. K. Li, J. Pharm. Sci., 2010, 99, 1955–1967 CrossRef CAS PubMed.
- S. Murdan, Int. J. Pharm., 2002, 236, 1–26 CrossRef CAS PubMed.
- A. Georgopoulos, G. Petranyi, H. Mieth and J. Drews, Antimicrob. Agents Chemother., 1981, 19, 386–389 CrossRef CAS PubMed.
- H. Nsairat, D. Khater, U. Sayed, F. Odeh, A. Al Bawab and W. Alshaer, Heliyon, 2022, 8, e09394 CrossRef CAS PubMed.
- V. Jhawat, M. Gulia and A. K. Sharma, in Chemoinformatics and Bioinformatics in the Pharmaceutical Sciences, ed. N. Sharma, H. Ojha, P. K. Raghav and R. k. Goyal, Academic Press, 2021, pp. 455–481, DOI:10.1016/B978-0-12-821748-1.00011-7.
- J. Y. Yamaguchi, Y. Nishimura, A. Kanada, M. Kobayashi, K. Mishima, T. Tatsuishi, K. Iwase and Y. Oyama, Toxicology, 2005, 211, 179–186 CrossRef CAS PubMed.
- H. Harde, A. K. Agrawal, M. Katariya, D. Kale and S. Jain, RSC Adv., 2015, 5, 43917–43929 Search PubMed.
- P. A. Cornwell, J. Tubek, H. A. H. P. van Gompel, C. J. Little and J. W. Wiechers, Int. J. Pharm., 1998, 171, 243–255 CrossRef CAS.
- F. Luisa Lüdtke, M. Aparecida Stahl, R. Grimaldi, M. Bruno Soares Forte, M. Lúcia Gigante and A. Paula Badan Ribeiro, Food Res. Int., 2022, 160, 111746 Search PubMed.
- J.-Y. Fang, C.-L. Fang, C.-H. Liu and Y.-H. Su, Eur. J. Pharm. Biopharm., 2008, 70, 633–640 CrossRef CAS PubMed.
- J. Liu, W. Hu, H. Chen, Q. Ni, H. Xu and X. Yang, Int. J. Pharm., 2007, 328, 191–195 Search PubMed.
- W. C. Evans and D. Evans, in Trease and Evans' Pharmacognosy, ed. W. C. Evans and D. Evans, W.B. Saunders, 16th edn, 2009, pp. 173–193, DOI:10.1016/B978-0-7020-2933-2.00019-8.
- A. Jentzsch and P. Aikens, in Delivery System Handbook for Personal Care and Cosmetic Products, ed. M. R. Rosen, William Andrew Publishing, Norwich, NY, 2005, pp. 861–871, DOI:10.1016/B978-081551504-3.50046-8.
- H. Nsairat, D. Khater, U. Sayed, F. Odeh, A. Al Bawab and W. Alshaer, Heliyon, 2022, 8, e09394 CrossRef CAS PubMed.
- C. Pereira-Leite, M. Bom, A. Ribeiro, C. Almeida and C. Rosado, Cosmetics, 2023, 10, 99 CrossRef CAS.
- J. Park, U. Scheler and R. J. Messinger, Langmuir, 2024, 40, 21814–21823 CrossRef CAS PubMed.
- S. B. Han, S. S. Kwon, Y. M. Jeong, E. R. Yu and S. N. Park, Int. J. Cosmet. Sci., 2014, 36, 588–597 CrossRef CAS PubMed.
- Y. Agrawal, K. C. Petkar and K. K. Sawant, Int. J. Pharm., 2010, 401, 93–102 CrossRef CAS PubMed.
- R. A. Sanad, N. S. AbdelMalak, T. S. Elbayoomy and A. A. Badawi, AAPS PharmSciTech, 2010, 11, 1684–1694 CrossRef CAS PubMed.
- V. R. Patel, G. G. Dumancas, L. C. Kasi Viswanath, R. Maples and B. J. Subong, Lipid Insights, 2016, 9, 1–12 CrossRef PubMed.
- S. A. Nasrollahi, A. Ayatollahi, T. Yazdanparast, A. Samadi, H. Hosseini, M. Shamsipour, A. A. Akhlaghi, S. Yadangi, C. Abels and A. Firooz, Clin., Cosmet. Invest. Dermatol., 2018, 11, 21–28 CrossRef CAS.
- P. Mohite, S. Singh, A. Pawar, A. Sangale and B. G. Prajapati, Frontiers Drug Deliv., 2023, 3, 1232012 CrossRef.
- K. Sugiura, N. Sugimoto, S. Hosaka, M. Katafuchi-Nagashima, Y. Arakawa, Y. Tatsumi, W. Jo Siu and R. Pillai, Antimicrob. Agents Chemother., 2014, 58, 3837–3842 CrossRef.
- F. C. Flores, R. S. Rosso, L. Cruz, R. C. Beck and C. B. Silva, Eur. J. Pharm. Sci., 2017, 100, 56–63 CrossRef CAS PubMed.
- P. P. Shanbhag and U. Jani, New Horizons in Translational Medicine, 2017, vol. 3, pp. 252–263 Search PubMed.
- D. Schoon, Do nails absorb everything or nothing?, https://nailknowledge.org/blog/do-nails-absorb-everything-or-nothing#:%7E:text=Thenailplate'supper,easilythroughthenailplate, accessed 01/03, 2025.
- E. Cutrín-Gómez, S. Anguiano-Igea, M. B. Delgado-Charro, J. L. Gómez-Amoza and F. J. Otero-Espinar, Pharmaceutics, 2018, 10, 273 Search PubMed.
- P. Mohan, J. Rajeswari and K. Kesavan, Ther. Deliv., 2024, 15, 23–39 CrossRef CAS PubMed.
- D. Mitra, Mater. Today: Proc., 2023, 83, 75–82 Search PubMed.
- D. Y. Tayah and A. M. Eid, Saudi Pharm. J., 2023, 31, 526–534 CrossRef CAS PubMed.
- B. S. Barot, P. B. Parejiya, H. K. Patel, M. C. Gohel and P. K. Shelat, AAPS PharmSciTech, 2012, 13, 184–192 CrossRef CAS PubMed.
- P. Thatai and B. Sapra, AAPS PharmSciTech, 2017, 18, 2316–2328 CrossRef CAS.
- V. Agrawal, R. Patel and M. Patel, J. Drug Delivery Sci. Technol., 2022, 74, 103494 CrossRef CAS.
- V. Agrawal, R. Patel, M. Patel, K. Thanki and S. Mishra, Colloids Surf., B, 2021, 201, 111652 Search PubMed.
- M. Fatima, S. Monawwar, S. Mohapatra, T. S. Alex, A. Ahmed, M. Taleuzzaman, A. Ali, M. J. Ansari, M. A. Mirza and Z. Iqbal, Gels, 2021, 7, 221 CrossRef CAS.
- P. M. Campos, G. D. A. Camargo, A. B. Klosowski and P. C. Ferrari, in Topical and Transdermal Drug Delivery Systems, Apple Academic Press, 2023, pp. 169–204 Search PubMed.
- G. Patel, B. Prajapati and H. Hinglajia, in Lipid-Based Drug Delivery Systems, Jenny Stanford Publishing, 2024, pp. 83–124 Search PubMed.
- Preeti, S. Sambhakar, R. Malik, S. Bhatia, A. Al Harrasi, C. Rani, R. Saharan, S. Kumar, Geeta and R. Sehrawat, Scientifica, 2023, 2023, 6640103 CrossRef CAS PubMed.
- Y. Singh, J. G. Meher, K. Raval, F. A. Khan, M. Chaurasia, N. K. Jain and M. K. Chourasia, J. Contr. Release, 2017, 252, 28–49 CrossRef CAS PubMed.
- K. B. Sutradhar and M. L. Amin, Eur. J. Nanomed., 2013, 5, 97–110 Search PubMed.
- N. Salim, N. Ahmad, S. H. Musa, R. Hashim, T. F. Tadros and M. Basri, RSC Adv., 2016, 6, 6234–6250 Search PubMed.
- A. Mahtab, M. Anwar, N. Mallick, Z. Naz, G. K. Jain and F. J. Ahmad, AAPS PharmSciTech, 2016, 17, 1477–1490 CrossRef CAS.
- S. Almawash, Saudi Pharm. J., 2023, 31, 1167–1180 CrossRef CAS PubMed.
- S. J. Aljurbui, A. Hussain, M. Yusuf, M. Ramzan, O. Afzal, B. Almohaywi, S. Yasmin and A. S. A. Altamimi, ACS Omega, 2022, 7, 22406–22420 CrossRef CAS PubMed.
- I. Arduino, Z. Liu, A. Rahikkala, P. Figueiredo, A. Correia, A. Cutrignelli, N. Denora and H. A. Santos, Acta Biomater., 2021, 121, 566–578 CrossRef CAS PubMed.
- R. Gaikwad, P. Dange, P. Nayakal and P. Ramugade, Asian J. Pharmaceut. Res., 2023, 13, 18–24 Search PubMed.
- S. A. Wissing and R. H. Müller, Int. J. Pharm., 2003, 254, 65–68 CrossRef CAS PubMed.
- H. Mu and R. Holm, Expet Opin. Drug Deliv., 2018, 15, 771–785 CrossRef CAS PubMed.
- S. Tiwari, P. Mistry and V. Patel, J. Pharm. Drug Dev., 2014, 1, 604 Search PubMed.
- F. E. Abobakr, S. M. Fayez, V. S. Elwazzan and W. Sakran, AAPS PharmSciTech, 2021, 22, 33 CrossRef CAS.
- K. Rajpoot, S. K. Prajapati, A. Malaiya, R. Jain and A. Jain, AAPS PharmSciTech, 2022, 23, 241 CrossRef CAS PubMed.
- S. Priya, V. M. Desai and G. Singhvi, ACS Omega, 2023, 8, 74–86 Search PubMed.
- E. B. Souto, I. Baldim, W. P. Oliveira, R. Rao, N. Yadav, F. M. Gama and S. Mahant, Expet Opin. Drug Deliv., 2020, 17, 357–377 CrossRef CAS.
- I. Chauhan, M. Yasir, M. Verma and A. P. Singh, Adv. Pharmaceut. Bull., 2020, 10, 150–165 CrossRef CAS PubMed.
- V. R. Salvi and P. Pawar, J. Drug Delivery Sci. Technol., 2019, 51, 255–267 CrossRef CAS.
- C. Viegas, A. B. Patrício, J. M. Prata, A. Nadhman, P. K. Chintamaneni and P. Fonte, Pharmaceutics, 2023, 15, 1593 Search PubMed.
- K. A. D. Rocha, A. P. Krawczyk-Santos, L. M. Andrade, L. C. Souza, R. N. Marreto, T. Gratieri and S. F. Taveira, Int. J. Pharm., 2017, 531, 292–298 CrossRef CAS.
- R. R. Pereira, M. Testi, F. Rossi, J. O. C. Silva Junior, R. M. Ribeiro-Costa, R. Bettini, P. Santi, C. Padula and F. Sonvico, Pharmaceutics, 2019, 11 Search PubMed.
- R. Kesharwani, P. Jaiswal, D. K. Patel and P. K. Yadav, Biomed. Mater. Dev., 2023, 1, 648–663 CAS.
- M. Rahman, J. G. Singh, O. Afzal, A. S. A. Altamimi, M. Alrobaian, J. Haneef, M. A. Barkat, W. H. Almalki, M. Handa, R. Shukla, S. Nasar Mir Najib Ullah, V. Kumar and S. Beg, ACS Omega, 2022, 7, 43499–43509 CrossRef CAS PubMed.
- E. D. Castañeda-Reyes, M. J. Perea-Flores, G. Davila-Ortiz, Y. Lee and E. Gonzalez de Mejia, Int. J. Nanomedicine, 2020, 15, 7627–7650 CrossRef PubMed.
- S. A. Ayatollahi Mousavi, A. Mokhtari, M. Barani, A. Izadi, A. Amirbeigi, N. Ajalli, A. Amanizadeh and S. Hadizadeh, Heliyon, 2023, 9, e18960 CrossRef CAS PubMed.
- T. S. Tuncay, Turk. J. Pharm. Sci., 2018, 15, 178–183 CrossRef PubMed.
- T. S. Tuncay, S. Hilmioğlu Polat, D. Yeşim Metin, G. Kandiloğlu and Ö. Özer, J. Liposome Res., 2016, 26, 163–173 Search PubMed.
- V. H. Shah and A. Jobanputra, AAPS PharmSciTech, 2018, 19, 213–224 CrossRef CAS PubMed.
- S. S. Chouhan, N. Farooqui and D. K. Mishra, Asian J. Pharm. Pharmacol., 2021, 7, 131–137 CrossRef CAS.
- M. G. Nava-Arzaluz, E. Piñón-Segundo and A. Ganem-Rondero, in Nanoparticles in Pharmacotherapy, Elsevier, 2019, pp. 311–390 Search PubMed.
- B. Kumar, M. Pandey, R. Aggarwal and P. K. Sahoo, Futur. J. Pharm. Sci., 2022, 8, 50 CrossRef.
- S. Jain, S. Tripathi and P. K. Tripathi, J. Drug Delivery Sci. Technol., 2021, 61, 102166 CrossRef CAS.
- S. Babaie, A. R. D. Bakhshayesh, J. W. Ha, H. Hamishehkar and K. H. Kim, Nanomaterials, 2020, 10, 341 CrossRef CAS PubMed.
- S. Nangare and S. Dugam, Futur. J. Pharm. Sci., 2020, 6, 1–21 CrossRef.
- Q. Hoda, M. Aqil, A. Ahad, S. S. Imam, A. Praveen, A. Qadir and Z. Iqbal, 3 Biotech, 2021, 11, 137 CrossRef.
- I. Gupta, S. N. Adin, M. A. Rashid, Y. Alhamhoom, M. Aqil and M. Mujeeb, Materials, 2023, 16, 4424 CrossRef CAS PubMed.
- J. Kishishita, C. de Almeida Perez Pimenta, D. P. Cerqueira Macedo, M. B. Delgado-Charro and L. Bastos Leal, Pharmaceutics, 2024, 16, 72 CrossRef CAS PubMed.
- M. Bonetti, M. Bracchi, G. Musitelli and P. Perugini, Int. J. Pharm., 2021, 600, 120409 CrossRef CAS PubMed.
- A. Valkov, M. Zinigrad, A. Sobolev and M. Nisnevitch, Int. J. Mol. Sci., 2020, 21, 3512 CrossRef CAS PubMed.
- S. A. T. Opatha, V. Titapiwatanakun and R. Chutoprapat, Pharmaceutics, 2020, 12, 855 CrossRef CAS PubMed.
- R. Rajan, S. Jose, V. P. Mukund and D. T. Vasudevan, J. Adv. Pharm. Technol Res., 2011, 2, 138–143 CrossRef CAS PubMed.
- R. Fernández-García, A. Lalatsa, L. Statts, F. Bolás-Fernández, M. P. Ballesteros and D. R. Serrano, Int. J. Pharm., 2020, 573, 118817 CrossRef PubMed.
- B. Sigurgeirsson and M. Ghannoum, Expet Opin. Invest. Drugs, 2012, 21, 1549–1562 CrossRef CAS PubMed.
- N. I. Elsherif, R. N. Shamma and G. Abdelbary, AAPS PharmSciTech, 2017, 18, 551–562 CrossRef CAS PubMed.
- A. Ritwiset, S. Maensiri and S. Krongsuk, RSC Adv., 2024, 14, 1697–1709 RSC.
- X. Ge, M. Wei, S. He and W. E. Yuan, Pharmaceutics, 2019, 11, 55 CrossRef CAS PubMed.
- D. B. Momekova, V. E. Gugleva and P. D. Petrov, ACS Omega, 2021, 6, 33265–33273 CrossRef CAS PubMed.
- V. Chatur, S. Dhole and N. Kulkarni, Int. J. Drug Deliv. Technol., 2024, 14, 895–901 CrossRef.
- V. D. Wagh and O. J. Deshmukh, ISRN Pharm., 2012, 2012, 653465 Search PubMed.
- S. Kappes, T. Faber, L. Nelleßen, T. Yesilkaya, U. Bock and A. Lamprecht, Pharmaceutics, 2021, 13, 2098 CrossRef CAS PubMed.
- R. P. Narayan, Indian J. Plast. Surg., 2012, 45, 396–402 CrossRef CAS PubMed.
- L. Nogueiras-Nieto, J. L. Gómez-Amoza, M. B. Delgado-Charro and F. J. Otero-Espinar, J. Controlled Release, 2011, 156, 337–344 CrossRef CAS PubMed.
- M. E. E. Temporiti, M. Guerini, R. M. Baiguera, S. Buratti, A. Desiderio, L. Goppa, P. Perugini and E. Savino, J. Fungi, 2022, 8, 1133 CrossRef CAS PubMed.
- S. Reichl and C. C. Müller-Goymann, Eur. J. Pharm. Biopharm., 2011, 78, 432–440 CrossRef PubMed.
- P. Hill, H. Brantley and M. Van Dyke, Biomaterials, 2010, 31, 585–593 CrossRef CAS PubMed.
- I. A. Larabi and J.-C. Alvarez, in Perspectives and Challenges of Hair Analysis, ed. P. Kintz, A. Salomone and M. Vincenti, Royal Society of Chemistry, 2023, vol. 47 Search PubMed.
- F. Laubé, A. Poupon, P. Zinck, C. Müller-Goymann, S. Reichl and V. Nardello-Rataj, Eur. J. Pharm. Sci., 2019, 131, 208–217 CrossRef PubMed.
- D. Monti, U. Herranz, L. Dal Bo and A. Subissi, J. Eur. Acad. Dermatol. Venereol., 2013, 27, e153–e158 CrossRef CAS PubMed.
- A. Louie, G. L. Drusano, P. Banerjee, Q.-F. Liu, W. Liu, P. Kaw, M. Shayegani, H. Taber and M. H. Miller, Antimicrob. Agents Chemother., 1998, 42, 1105–1109 Search PubMed.
- X. Hui, E. C. Jung, H. Zhu and H. I. Maibach, Drug Dev. Ind. Pharm., 2017, 43, 22–29 CrossRef CAS PubMed.
- M. Jarratt, W. J. Siu, E. Yamakawa, N. Kodera, R. Pillai and K. Smith, J. Drugs Dermatol., 2013, 12, 1010–1016 CAS.
- A. Y. Fnlay, Br. J. Dermatol., 1992, 126, 28–32 Search PubMed.
- N. Kubota-Ishida, N. Takei-Masuda, K. Kaneda, Y. Nagira, T. Chikada, M. Nomoto, Y. Tabata, S. Takahata, K. Maebashi and X. Hui, Antimicrob. Agents Chemother., 2018, 62, e00779 CrossRef PubMed.
- G. Krishna, L. Ma, M. Martinho, P. Prasad, J. Wahl and A. Tavakkol, Antimicrob. Agents Chemother., 2011, 55, 4424–4426 CrossRef CAS PubMed.
- J. Pannu, A. McCarthy, A. Martin, T. Hamouda, S. Ciotti, A. Fothergill and J. Sutcliffe, Antimicrob. Agents Chemother., 2009, 53, 3273–3279 CrossRef CAS PubMed.
- A. Firooz, S. Zamani, A. Ghadrei, A. Ayatollahi, P. Tamimi, A. Khamesipour, M. Jafari and M. Fattahi, Dermatol. Ther., 2023, 2023, 9955124 CrossRef.
- M. Moazeni, H. Kelidari, Y. Nasirzadehfard, T. Shokohi, B. Roohi, Z. Hajheidari, A. Kazeminejad, S. Parsay, K. Asare-Addo and A. Nokhodchi, Med. Drug Discov., 2024, 22, 100187 CrossRef CAS.
- S. R. D'Mello, C. N. Cruz, M.-L. Chen, M. Kapoor, S. L. Lee and K. M. Tyner, Nat. Nanotechnol., 2017, 12, 523–529 CrossRef PubMed.
- FDA, Classification of Products as Drugs and Devices and Additional Product Classification Issues, https://www.fda.gov/regulatory-information/search-fda-guidance-documents/classification-products-drugs-and-devices-and-additional-product-classification-issues, accessed 10/10/2024.
- S. J. Oldenburg, W. N. Boehm, K. Sauerova and T. K. Darlington, in Nanomaterials for Magnetic and Optical Hyperthermia Applications, ed. R. M. Fratila and J. M. De La Fuente, Elsevier, 2019, pp. 339–353, DOI:10.1016/B978-0-12-813928-8.00013-2.
- ICH, Good Manufacturing Practice Guide For Active Pharmaceutical Ingredients Q7, https://database.ich.org/sites/default/files/Q7Guideline.pdf, accessed 05/10/2024.
- ICH, Pharmaceutical Quality System, Q10, https://database.ich.org/sites/default/files/Q10Guideline.pdf, accessed 05/10/2024.
- FDA, Nanotechnology Fact Sheet, https://www.fda.gov/science-research/nanotechnology-programs-fda/nanotechnology-fact-sheet, accessed 10/10/2024.
- FDA, Considerations for Drug Products that Contain Nanomaterials, CDER Small Business and Industry Assistance Chronicles, 2024, https://www.fda.gov/drugs/cder-small-business-industry-assistance-sbia/considerations-drug-products-contain-nanomaterials, accessed 8/10/2024 Search PubMed.
- FDA, Drug Products, Including Biological Products, that Contain Nanomaterials - Guidance for Industry, https://www.fda.gov/regulatory-information/search-fda-guidance-documents/drug-products-including-biological-products-contain-nanomaterials-guidance-industry, accessed 08/10/2024.
|
This journal is © The Royal Society of Chemistry 2025 |
Click here to see how this site uses Cookies. View our privacy policy here.