Nutritional composition and bioactive potential of watermelon seeds: a pathway to sustainable food and health innovation
Received
12th November 2024
, Accepted 6th January 2025
First published on 29th January 2025
Abstract
Watermelon (Citrullus lanatus), belonging to the Cucurbitaceae family, is a globally cultivated fruit with significant commercial value. While the fleshy part is eaten for its taste and nutritional benefits, the seeds are generally thrown away as waste, although they harbour high nutritional and bioactive contents. Watermelon seeds are rich in proteins, fats, vitamins, and minerals, and they also contain bioactive compounds such as phenolic acids, flavonoids, and carotenoids. This review seeks to investigate the proximate composition of watermelon seeds, novel techniques employed in the extraction of bioactive compounds and the various facets of therapeutic applications of these seed bioactivities, such as antioxidant, antimicrobial, antimutagenic, hypoglycaemic and anti-inflammatory properties. The effects of these seeds are assessed based on the pharmacological mechanisms underlying their therapeutic actions. Also, this review discusses the potential of using watermelon seeds and their components in the food industry as valuable functional foods and in the cosmeceuticals and nutraceuticals industries. These include health-enhancing food supplements, cosmetics, and newly developed edible products for human consumption. However, there are some issues related to its composition, yield, and safety profile that impede its optimum use. In particular, this paper highlights the sustainability problems, suggesting that converting agricultural waste into such by-products could lead to a circular economy and improve human welfare.
Sustainability spotlight
The study of watermelon seeds unveils their potential as a valuable sustainable resource that contributes to world food security as well as health improvement. With drastic growing concerns related to food waste and the increasing demand for alternate nutrient-dense food products, watermelon seeds offer an invaluable substitution. The seeds are protein-rich, loaded with healthy fats, vitamins and various bioactive compounds. Thus, in addition to being a sustainable nutritional source, they also contribute to the circular economy by agricultural waste reduction. Furthermore, their bioactive potential includes antioxidant, antimicrobial and anti-inflammatory properties, positioning watermelon seeds as an effective ingredient in natural and functional food products. By promoting and highlighting the potential of watermelon seeds, this paper provides an effective pathway aligned with the principles of sustainability in food technology. This can be further elaborated by mentioning the role of watermelon seed utilization in food waste reduction, enhancing resource efficiency as well as supporting the innovative creation and health-promoting solutions. Therefore, watermelon seeds present both environmental as well as effective health benefits, serving as an effective and key constituent in advancing sustainable food systems and fostering human nutrition globally.
|
1. Introduction
Watermelon, scientifically named Citrullus lanatus (CL), is a fruit with potential nutritional and pharmacological value. Watermelon belongs to the Cucurbitaceae family and the Citrullus genus.1 This fruit is among the most extensively grown fruits in the world and holds significant commercial value. With reference to the area, watermelons are commonly cultivated in Asia (accounting for 79.5%), Africa (7.5%), and America (6.9%), with approximately 3.1 million hectares dedicated to its cultivation and a production capacity of about 104 million tons every year. China is the major producer of the crop, comprising 67% of the global production.2,3 According to the FAO, the duration that it takes for a watermelon to attain ripeness differs with climate, typically taking between 80 and 110 days.
Consumption of watermelons (Fig. 1) has been increasing because of their taste, nutritional value, and attractive color, which ranges from red and yellow to pink. The flesh color of watermelon depends predominately on carotenoids and the degree of their deposition in chromoplasts during the ripening stage.4 For example, red-fleshed watermelons contain lycopene and β-carotene; yellow-fleshed watermelons have neoxanthin, violaxanthin, and luteoxanthin; and orange-fleshed watermelons contain β-carotene, prolycopene, phytoene and ζ-carotene. On the other hand, white-fleshed watermelons contain low levels of carotenoids, as previously shown.5
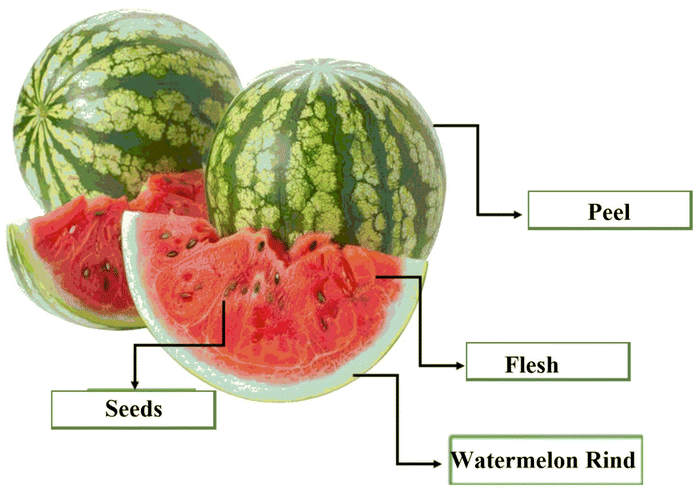 |
| Fig. 1 Parts of the watermelon fruit. | |
Watermelon is widely consumed by a large population worldwide owing to its incredibly nutrient-dense, low-calorie, and thirst-quenching properties.6,7 The water content of watermelon is approximately 92%. Watermelon is also cholesterol-free and high in vitamins, especially B1, B6, and C, as well as lycopene, a carotenoid, and minerals such as magnesium and potassium. These components have positive health effects, such as better cardiovascular health, management of hypertension in hypertensive patients, lower LDL oxidation, and prevention of age-related diseases and some forms of cancer.8–10
However, the rising consumption of watermelon has led to the massive production of by-products, most of which are generated majorly by food processing industries, including huge amounts of peels and seeds.11–13 The accumulation of these agri-food by-products presents economical and environmental challenges, conditions that are counterproductive to food security.14 Organically, watermelon processing involves stripping the edible portion that makes up approximately 57–68% of the fruit, the rind forms between 30–41% of the whole fruit and the seeds form 2% of the entire fruit. Out of all by-products, watermelon rinds contribute the largest portion of waste but can be reused in jam manufacturing.11 By-products, mostly watermelon seeds, have high concentrations of bioactive compounds, which offer prevention against various human ailments. The watermelon seeds have been proven to exhibit antimutagenic,15 antioxidant,16,17 antimicrobial,18 hypoglycaemic4 and anti-inflammatory activities.19 In addition, the watermelon pulp contains numerous vitamins, antioxidants, minerals, and bioactive compounds that are beneficial to human health.20
This article comprehensively reviews the nutritional composition, bioactive compound extraction, bioactive potential, mechanisms of action, and therapeutic properties of watermelon seeds. It also explores the potential applications in food processing and discusses the challenges and future perspectives in utilizing watermelon byproducts for novel functional ingredients.
Nonetheless, seeds and peels of watermelon are usually thrown away, although they are sources of nutrients. These by-products have bioactive prospects, which make them an affordable and untapped resource in the food and drug sectors.21 The consumption of watermelon by-products provides health benefits to the human body.22
This is where extensive prospects for revalorization can be viewed as highly possible for turning watermelon seed by-products into higher value, functional food ingredients, nutraceuticals, supplements, drugs or cosmetics.
This article systematically summarises all details of the nutritional composition, bioactive compound extraction, bioactivities, modes of action, and therapeutic uses of watermelon seeds.
2. Nutritional composition of watermelon seeds
Watermelon seeds are not only edible but also highly nutritious, containing ingredients including macronutrients, micronutrients and phytochemical compounds. Several researchers aimed at the proximate composition of watermelon seeds, stating that they contain crude fat (48–50%), protein (18–30%), carbohydrates (10–18%), moisture (4–8%), total dietary fiber (2.5–49%) and ash content (2.3–3.8%).23,24 The percentage yield of oil from the watermelon seeds outweighs the yields of other more conventional oil-bearing seeds such as cotton seeds, soybeans and olive seeds,25 as shown in Table 1.
Table 1 Nutritional and Bioactive Components of Watermelon Seeds
Component |
Concentration/Composition |
Bioactive potential |
References |
Protein |
25.2–35.6% |
Source of essential amino acids, promotes muscle growth, aids in tissue repair, and supports the immune system |
25
|
Fats (lipids) |
20.0–50.0% |
High in unsaturated fatty acids, particularly omega-6 and omega-9, and beneficial for cardiovascular health |
26–28
|
Carbohydrates |
8.0–20.0% |
Provides energy and dietary fiber, aiding digestion and promoting gut health |
29 and 30 |
Minerals |
Magnesium: 500–532 mg/100 g |
Crucial for muscle function, nerve regulation, and bone health |
30 and 31 |
Iron: 7.08–7.28 mg/100 g |
Vital for oxygen transport and prevention of iron deficiency anemia |
32 and 33 |
Zinc: 10.13–10.24 mg/100 g |
Supports immune function, cell growth, and wound healing |
32 and 33 |
Calcium: 54 mg/100 g |
Essential for maintaining bone health |
29 and 30 |
Phosphorus: 755 mg/100 g |
Important for energy metabolism, strong bones, and cellular functions, including DNA synthesis |
Vitamins |
Vitamin A (retinol, beta-carotene): 69.12 mg kg−1 |
Essential for vision, immune function, and skin health |
27, 28 and 34 |
Vitamin C (ascorbic acid): 19.45 mg kg−1 |
Antioxidant, supports skin health, and enhances immune response |
Vitamin D (cholecalciferol): 8.76 mg kg−1 |
Important for calcium absorption and bone health |
Vitamin B9 (folate): 1.84 mg kg−1 |
Crucial for DNA synthesis, cell division, and growth, especially during pregnancy |
Vitamin E (tocopherol): 3.53 mg kg−1 |
Antioxidant, protects cells from oxidative damage |
Vitamin K (phylloquinone): 1.44 mg kg−1 |
Essential for blood clotting and bone metabolism |
Amino acids |
Phenylalanine: 5.15 g/100 g |
Most abundant; essential for protein synthesis and neurotransmitter production |
27, 28 and 35 |
Arginine: 4.98 g/100 g |
Vital for protein metabolism and cardiovascular health |
Valine: 4.56 g/100 g |
Essential for muscle growth, tissue repair, and energy production |
Total phenols |
1494–5416 mg GAE/100 g |
Antioxidant properties, may reduce inflammation, support heart health, and lower cancer risk |
36
|
DPPH scavenging ability |
56.93–94.46% |
Defends against oxidative damage |
37
|
Free radical scavenging activity |
82.59–130.29 μM trolox/g |
Potential to lower the risk of chronic diseases |
36
|
In a study assessing the fatty acid composition of fifteen cultivars of watermelon, most of the cultivars contained higher levels of unsaturated fatty acids,38 with linoleic acid accounting for 45% to 55%, followed by oleic acid accounting for 10% to 20%, palmitic acid accounting for 10% to 15%, and the stearic acid accounts for 10.23%. Also, alpha-linolenic acid at 0.35%, palmitoleic acid at 0.29%, and myristic acid at 0.11% were detected in the seed oil.39 In addition to fatty acids, WSO has other lipophilic compounds such as sterols, diglycerides, monoglycerides and phospholipids.39,40 Consequently, watermelon seeds contain monounsaturated and polyunsaturated fatty acids, which help to avert heart and vascular diseases. The second biggest constituent found in watermelon seeds is protein. The seeds contain glutamic, aspartic, arginine and leucine amino acid distributions.41 However, among all the phytochemical compounds, L-citrulline is specifically notable, present not only in fresh pulp but also in seeds. As a simple, non-essential amino acid, it serves as a substrate for the production of L-arginine in the urea cycle. L-Arginine, in turn, generates nitric oxide, a crucial molecule required for maintaining endothelial function and regulating vascular resistance. L-Citrulline, primarily derived from watermelon, significantly contributes to cardiovascular health by facilitating nitric oxide production, which helps regulate blood pressure and prevent conditions such as atherosclerosis and hypertension.42 Notably, the citrulline concentration is higher in yellow and orange-fleshed watermelons compared to red-fleshed varieties.43
Watermelon seeds contain four major protein fractions: 46% globulin, 39% glutelin, 23% albumin and 22% prolamin. These proteins have satisfactory solubility and digestibility and show antimicrobial and antioxidant effects and have the potential to be natural food preservatives. However, due to their antioxidant activities, they can be subjected to variation depending on the pretreatment and extraction of the used methods.44 Research has demonstrated that watermelon seed proteins exhibit antioxidant activity by upregulating the Nrf2/HO-1 pathway, an antioxidant defense system implied in combating oxidative stress.45 These proteins generally have molecular weights between 25 kDa and 250 kDa, but with the hydrolysis process, bioactive peptides are obtained. This stability under various conditions also improves its application in food and health-related applications.44
It also means that watermelon seeds contain secondary metabolites that have positive effects on human health. These include alkaloids, polyphenols, phenolic acids, flavonoids, tannins, phytates and oxalates, with the alkaloids being present in the largest quantities.46 On the other hand, saponins are found in the watermelon in every part but seeds. The seeds are especially concentrated in triterpenoids, which are known to possess antibacterial effects. Studies on the relative antioxidant and antimicrobial potential of different parts of the watermelon revealed that the peel exhibited the highest total phenol content and antioxidant potential, followed sequentially by the seed, rind, and pulp. These results confirmed the high phytochemical level and antioxidant activity of the watermelon seeds, which can act as a health-beneficial ingredient.
The watermelon seeds also contain several phenolic acids such as gallic, p-coumaric, caffeic, sinapic, hydroxycinnamic and cinnamic; among these, the content of sinapic is the highest.4 Other phenolic compounds present at this concentration include naringenin-7-O-glycoside, apigenin, amentoflavone, rutin, quercitrin, quercetin, luteolin, and kaempferol.39,41 Among the phenolic glycosides isolated from watermelon seeds, lanatusosides C and D have shown potential antitumor activity.47 The watermelon seeds also contain a variety of vitamins, especially vitamin A, vitamin C, B complex, vitamin E and vitamin K. They also contain micro-elements, such as magnesium, phosphorus, iron, zinc, calcium, manganese, and copper, with potassium being the most concentrated element. Potassium is vital for maintaining electrolyte balance and supporting heart function.4
Watermelon seeds also contain a good proportion of carbohydrates such as glucose, sucrose, fructose and carotenoids of beta-carotene.46 Furthermore, the arginine content of the seeds is high and has health benefits, including anti-ulcerative colitis and improved antioxidant activity.47 Because of these nutritional components, watermelon seeds find their applications in different food products.48 Several previous studies have quantified the phytochemicals present in watermelon seed methanol extracts, which also displayed antibacterial activities. Watermelon seeds have been reported to stimulate appetite and serve as an effective remedy for constipation.37
In addition to the nutritional and therapeutic effects, watermelon seeds also contain some anti-nutritional factors, including oxalates, phytates and tannins, compared to the rinds. These compounds can hinder nutrient uptake. However, these compounds can be modified by different processes like roasting, boiling and oven drying, making the antinutritional factors relatively ineffective.49
3. Molecular mechanisms of bioactive compounds in watermelon and its seeds: a biochemical perspective
The bioactive compounds in watermelon by-products primarily include various subclasses of cucurbitacins, glycosides, polyphenols and alkaloids, each exhibiting distinct molecular mechanisms that contribute to their health-promoting properties (Fig. 2).
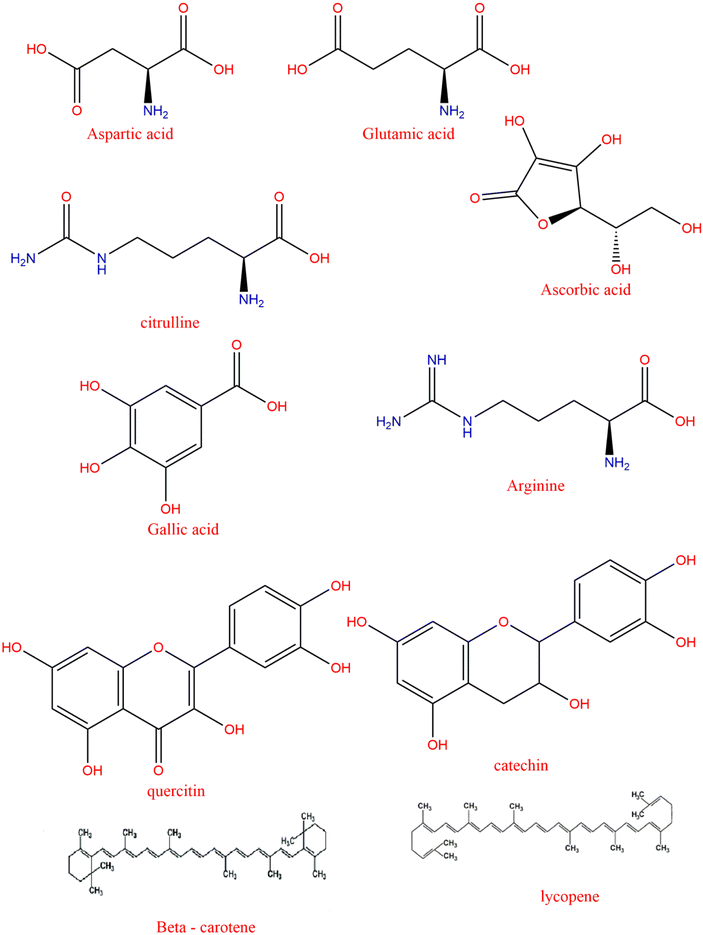 |
| Fig. 2 Chemical structures of watermelon seed bioactive compounds. | |
3.1 Cucurbitacins
Cucurbitacins are tetracyclic triterpenoids found in several plants belonging to the Cucurbitaceae family, particularly in watermelon.20 Terpenoid compounds are synthesized from terpene, mainly isopentenyl pyrophosphate five-carbon isoprene unit and dimethylallyl pyrophosphate and its isomer. Terpenoids also interact with the most extreme elements of regulatory proteins and exhibit antioxidant activity. For the mitigation of inflammatory diseases and cancer, plant extracts are used along with traditional and other forms of intervention medicine.50 Present-day medicine employs terpenes as NF-κB inhibitors as described previously.51 Under the category of cytoplasmic sensors, the NF-κB system reacts to the disturbance in the immune system and many other signals and internal signals such as genotoxic stress and hypoxia. Cellular resistance enlargement stands against anti-apoptotic signaling as well as the apoptosis of NF-κB also has a remarkable contribution.
Cucurbitacin E is distinguished by the fact that it is an anti-inflammatory agent that is also cataloged as acting as a triterpenoid. The reactive nitrogen-containing molecules have been neutralized and the cyclo-oxygenase enzymes have been inhibited by cucurbitacin E. This agent is used for the reduction of RNS through an anti-inflammatory agent but fails to eliminate at a cellular level ROS.52 For example, when cucurbitacin E was used in combination with doxorubicin, an increased cytotoxicity effect towards tumor cells both in the culture and experimental animals and a decrease of tumor size and mass were observed.53,54 Likewise, when cucurbitacin B was mixed with docetaxel, there was an improvement in the anticancer effect on human laryngeal cancer Hep-2 cells.55,56
3.2 Glycosides
The glycoside phytochemicals in watermelon seeds were identified as having anti-diarrheal benefits, amongst others.57 These compounds can normally exist concurrently with other bioactive agents, such as polysaccharides and thiol derivatives. Glycosides are chemical compounds that involve a carbohydrate pair with another non-carbohydrate component known as the aglycone or genin linked to the carbohydrate through alcohol, glycerol, or phenolic bond. The soluble material of the watermelon seed glycosides has various structures, such as a crystalline carbon–oxygen–hydrogen structure, a few carbon–oxygen–hydrogen–sulfur structure, or a carbon–oxygen–hydrogen–nitrogen structure.
Glycosides are readily susceptible to hydrolysis by acids, which makes such molecules extremely reactive in many physiological activities. These compounds are grouped according to the chemical nature of their aglycone or their pharmacological activity. For example, bitter principles like glycosides andrographolide, a diterpene lactone and amarogentin, and a triterpenoid lactone acting on gustatory nerves activate saliva as well as gastric juice release. Others, which include anthracene glycosides, are used in the treatment of the skin and to control bowel movement. Cyanogenic glycosides, in contrast, decompose to liberate hydrogen cyanide (HCN) through enzymatic splitting and although useful in controlled quantity for use such as in cough rectification (for instance, amygdalin), they are lethal and cause major gastrointestinal discomfort if ingested in large volumes.58
Therefore, proper processing and regulation are crucial to reduce the toxicity of cyanogenic glycosides in consumer food products, thus ensuring the health of the consumer as well as making the best use of the potential therapeutic benefits of the products that contain the glycosides.
3.3 Polyphenols
Water soluble, non-volatile organic compounds known as polyphenols are also bioactive substances present in watermelon and they are secondary metabolites of plants (Fig. 3). Its main distribution is in mesophyll cells, nucleus and areas where reactive oxygen species (ROS) are produced.59,60 These compounds, produced by the phenylpropanoid pathway, conduct numerous health-promoting actions.61,62
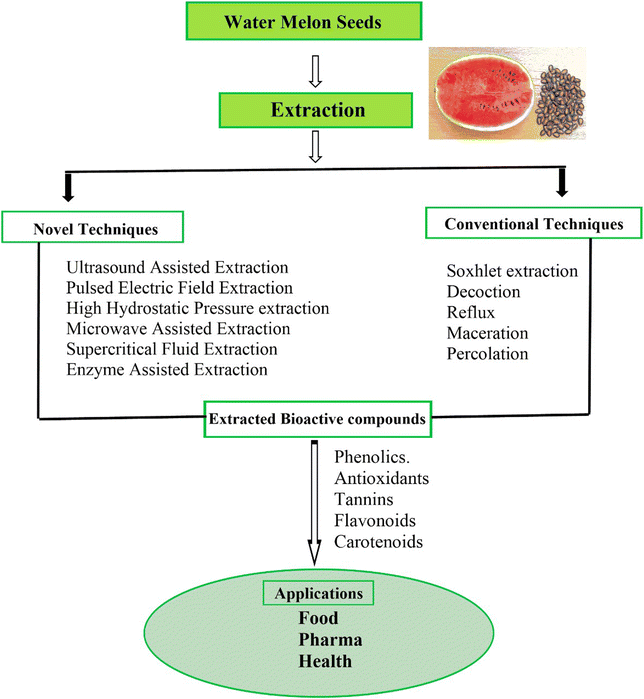 |
| Fig. 3 Extraction techniques for watermelon seed bioactive compounds. | |
Polyphenols, especially polar phenolic compounds with functional hydroxyl groups, remove free radicals, chelate metal ions, and trigger protective enzyme pathways; they serve as anticancer agents, antioxidants, and inhibitors of cardiovascular diseases and bacterial and viral infections.63 Their antioxidant action causes a decrease in ROS formation, inhibition of enzymes contributing to free radical formation, and reactive nitrogen species (RNS) elimination.64 Phenolic compounds such as anthraquinones are present in moderate concentrations and are known for their effectiveness in alleviating stomach aches and constipation. Anthraquinones, derived from anthracene, produce specific compounds like anthranols and anthrone derivatives. These compounds often exhibit unique structural features, such as double hydroxylation at the C-8 and C-1 positions, resulting in derivatives like chrysophanol, luteolin, emodin, and rhein. The presence of anthraquinones in plant samples can be visually identified by a violet or pink coloration in the base layer during analysis.58
The structure of flavonoids impacts their antioxidant capacity, with aglycones exhibiting higher degrees of activity in comparison to their glycosides.52 Polyphenols can suppress the activities of enzymes that produce ROS and potentiate the oxidation of lipids and other compounds through glutathione and monooxygenase inhibitors and other pathways.64,65 For instance, epicatechin and rutin reduce lipid peroxidation in vitro successfully.66 Furthermore, quercetin also has a chelating and stabilizing effect on iron, through which ethanol extracts containing epicatechin and quercetin originating from winery byproducts show a potent antiplatelet activity.67
Such molecular interactions highlight the great prospect of cucurbitacins and polyphenols from watermelon and by-products in the enhancement of functional food, nutraceuticals, and therapeutic agents for overall human health.
3.4 Alkaloids
Alkaloids, found in high concentrations in watermelon seeds compared to other components, are important plant secondary metabolites with significant therapeutic bioactivities. They are responsible for antispasmodic, analgesic and antibacterial actions and hence they are popular worldwide.68 Chemically, alkaloids are composed of ammonia derivatives that form the principal group of secondary chemical compounds synthesized from amino acid building blocks. These nitrogenous bases become incorporated in peptide rings. Alkaloids can be classified as primary, secondary or tertiary, depending on the level of basicity present in the compounds, their molecular structure, their functional groups, and the position of the nitrogen atom.58
Alkaloids combine with acids to render crystalline salts, and they are in different physical conditions. Some, such as atropine, are solids, while others are liquids formed from carbon, hydrogen and nitrogen. These are soluble in water and most of the salts, although exhibit higher solubility in alcohol. The solutions containing alkaloids are particularly bitter and this aspect forms the part of the sensory properties. In human and medical perception, pharmacologically, alkaloids are employed as painkillers and for stimulating the central nervous system. Around one-fifth of plant species synthesise pharmaceutical alkaloids and over twelve thousand different alkaloids are known. Some of the commonly known alkaloids are nicotine, cocaine, caffeine, ergotamine, codeine morphine, ephedrine, and atropine, of which many are known stimulants or therapeutic agents.
4. Extraction methods for watermelon seeds: focus on bioactive compounds
The process of obtaining bioactive compounds from plant matrices is a key process in making optimal use of the existing data about their availability and potential usage in different fields: food industry, pharmacy and production of chemical products. Phenolics, flavonoids, terpenoids, and alkaloids act as potential bioactive compounds that deliver enormous health benefits to human beings. However, the effectiveness and quality of the extracted compounds largely depend on the extraction methodology employed. Thus, improving standardized and effective extraction techniques is crucial when capturing the maximum volume of these compounds and their bioavailability. The selection of extraction techniques plays a significant role in determining the cost, time, recovery and purity of the bioactive compounds; therefore, the assessment of the conventional and innovative extraction techniques in terms of strength and weakness is crucial. Various pre-extraction unit operations are required to increase the extraction rate. Some of them are washing, cutting, size reduction and drying as postharvest handling activities.69
4.1 Conventional extraction methods for bioactive compounds
Most solvent-based extraction techniques are considered traditional because they employ factors such as polarity, ionic strength, heat, and mechanical force to extract bioactive compounds from plant samples. Among these methods are maceration, percolation, decoction, reflux extraction, and Soxhlet extraction; these methods have been used for many years in the food and pharmaceutical industry due to their simplicity and efficiency in different fields (Fig. 3).
4.1.1 Cold-pressing.
Cold pressing is a method for extracting oil from watermelon seeds by applying pressure to ground seeds to release the oil. This is followed by centrifugation to separate the oil from the remaining plant material, and the process concludes with filtration to obtain purified oil.70
4.1.2 Maceration.
Maceration is among the simplest and earliest extraction methodologies used for obtaining bioactive compounds and is also a very common method in phytochemical research. It involves reducing the size of the plant material in order to create a large surface area of the material, enhancing its ability to mix and interact with the solvent, either water or organic solvents. The mixture is then stirred for several hours and the liquid is filtered to eliminate unwanted material. Although maceration is a simple and inexpensive process, it still has some drawbacks, such as long extraction time and low extraction rate. It is most suitable when extracting thermolabile compounds, which tend to decompose during higher-temperature extraction conditions.47 The efficiency of extraction is generally based on factors like the solvent type, extraction period, sample particle size, extraction temperature, and the amount of solid matter and solution.71 Samples with small particles are easier for permeation by the solvent, and higher temperatures always favor the extraction process. However, excessive temperatures can cause the loss of the volatile oil constituents in question. If extraction time is increased, little difference to the extraction yield is observed since the concentration process starts reaching what can be considered equilibrium. However, the solid/solvent ratio should also be kept constant because, with a higher ratio of the solid phase, extraction time increases.72 The use of maceration at room temperature offered an option to extract phenolic compounds from an oil mixture pH and solvent extractant levels of watermelon seed extracts through Soxhlet extraction and maceration with methanol inhibited DPPH radicals, as established previously.73,74 The IC50 belonged between 1.41 and 2.60 mg mL−1, possessing considerable antioxidant properties.
4.1.3 Percolation.
Percolation is a more efficient procedure than maceration and includes concurrent filtration of the solvent through plant matter within a percolator. A powdered plant sample is normally steeped in boiling water and allowed to macerate for a few hours before the solvent is continuously replaced with fresh solvent. This approach enables better extraction outcomes and shortens the required period for processing thickened sludge. Following extraction, evaporation is employed to reduce the volume of extracted products proportionally. While percolation has benefits, the technique needs a special apparatus called a percolator and may not be suitable for some kinds of bioactive compounds.75
4.1.4 Decoction.
Another extraction method is decoction and is normally used for heat-stable products mainly due to its applicability. It involves treating the plant material with water or aqueous solution at a particular temperature and duration, after which the liquid is cooled, filtered and strained. Decoction is particularly good for extracting compounds soluble in water but is unsuitable when extracting thermolabile and volatile substances, which tend to be destroyed by heat. Moreover, it may be less productive due to kinetic and mass transfer limitations, which can influence the yield and quality of the extracted compounds.
4.1.5 Reflux extraction.
Reflux extraction, also known as solid–liquid extraction, is said to be more effective than percolation and maceration since it uses lower solvent volume and less time to extract. In this technique, the plant material is blended in a heated and stirred container with a solvent to recover the oil. The vapors formed are cooled and are permitted to drop back into the flask, thus increasing the overall mass transfer and contact between the solvent and the plant matrix. However, this method is quite good for the extraction of heat-stable compounds but not ideal for thermolabile compounds that tend to be degraded during extraction.47
4.1.6 Soxhlet extraction.
Soxhlet extraction is one of the oldest and most popular traditional methods for extracting bioactive compounds from plant materials. This method actually borrows features from the reflux and the percolation technique because it constantly pumps solvent through the sample, and as such, fresh solvent is constantly in contact with the sample. This results in high extraction capabilities, lesser solvent utilization and a relatively shorter time compared to conventional procedures such as maceration or percolation. However, Soxhlet extraction involves lengthy heating, which may lead to the thermal decomposition of some of the bioactive compounds and thus restricts its application to heat-stable compounds.76
Traditional extraction techniques like maceration, percolation, decoction, reflux extraction, and Soxhlet extraction are still considered important for the extraction of bioactive compounds due to their simplicity and wide applicability. Among the conventional methods of extraction, maceration and Soxhlet extraction are particularly significant. Conventional and non-conventional methods have been used for the extraction of different phenolic compounds from grape skin.77Vernonia cinerea leaf and feijoa peel extraction gave a total phenolic content of 48.6 to 71 mg GAE/g.78 However, considering factors such as the choice of solvent, temperature, or extraction time provided the basis for the yield and quality of extracted bioactive compounds. Selecting the appropriate method depends on the specific properties of the compounds being extracted and the desired end-use of the extracts.
4.2 Novel extraction methods for bioactive compounds
Conventional extraction methods like Soxhlet and maceration are commonly used but may present drawbacks such as long extraction times, high solvent consumption, and potential degradation of sensitive compounds. To overcome these challenges, novel extraction methods have emerged, offering enhanced efficiency, lower solvent use, and better preservation of bioactive compounds. Below is a detailed review of these innovative techniques in the context of watermelon seeds (Fig. 3).
4.2.1 Supercritical fluid extraction (SFE) for watermelon seed lipids.
Supercritical fluid extraction (SFE) uses CO2 as the extraction solvent, making it effective for extracting nonpolar and moderately polar compounds.79 CO2 is non-toxic and non-polar, has a high solute capacity and is suitable for extracting thermosensitive compounds. Watermelon seeds have very high amounts of unsaturated fats, and these fats respond poorly to high temperatures of conventional extraction techniques. SFE makes it possible to extract oil from nuts at relatively low temperatures and thus prevents the alteration of the nutritional properties of lipids. Furthermore, the method provides a high yield of bioactive lipids per unit of raw material with minimal solvent left behind, which makes it appropriate for commercial food-grade oil extraction from watermelon seeds.80 This method has been widely used in many types of seeds, including coca, linseeds and sesame seeds.81 A study found that high-density gases or supercritical fluids enhanced the pressing process since the required mechanical pressure was about 10 MPa, while the yield increased in the range of 10–20%.82 The current filtration technologies, in combination with the SC-CO2, have thus been tested for the purification of compounds of relatively low molecular weights of about 1500 g mol−1. This method has been successfully applied in the purification of beta-carotene extracted from carrot oil and triglyceride fraction from fish oil.
4.2.2 Microwave-assisted extraction (MAE) on antioxidants.
The MAE method can be applied to extract antioxidants from watermelon seeds. The seed matrix also consists of phenolic compounds, flavonoids, and tocopherol, and all of them have antioxidant values. MAE enhances the extraction process via the use of microwave radiation that heats both the solvent and seed matrix simultaneously. This leads to a faster extraction rate of these bioactive compounds with a small amount of solvent required. It has also been established that the method is most useful in the recovery of phenolic compounds with a high antioxidant potential. Microwave-assisted extraction (MAE) is widely used as a more efficient method for the extraction of bioactive compounds from plant and animal origins. Many benefits can be achieved with the help of this method, including increased extraction rate as well as a many-fold decrease in time for further processing. The technique increases the efficiency of cell disruption, thereby improving solvent penetration due to the effects of microwave energy and facilitating the extraction of target compounds in a shorter time than conventional methods.83 Moreover, in contrast to most organic solvents, MAE tends to use water or other Earth-friendly solvents, so it can be considered more environmentally friendly. Due to these benefits, MAE is used in this work and generally in the separation of various biologically active substances from natural matrices.84
4.2.3 Instant controlled pressure drop (DIC) technology.
DIC is a hydro-thermo-mechanical process that applies auto-vaporization and pressure variation to improve the qualities of organic products, including food products, cosmetics, and pharmaceutical biopolymers. The DIC system consists of four main components of the mill: (a) a pressure drop valve that provides fast and regulated release of steam pressure; (b) a vacuum chamber, fifty percent larger than the extraction vessel; (c) an extraction vessel fitted with a heating jacket autoclave where the sample is treated; (d) an extract collection chamber used for condensate collection with the tank pressure kept at roughly 5 kPa by a water ring pump. DIC is a potential substitute for handling temperature-sensitive food powders such as apples and onions.85 Instant controlled pressure drop (DIC) pretreatment has been recognized as a superior alternative to traditional drying methods, particularly for the extraction and texturization of vegetable materials. This technique involves a rapid transition from high pressure to vacuum, causing structural modifications in the plant matrix that enhance the release of target compounds. DIC pretreatment is especially effective for delicate fruits like strawberries, preserving their quality while improving extraction efficiency. Its ability to combine gentle processing with enhanced functionality makes DIC a valuable tool in the food and nutraceutical industries.85 They also concluded that the strawberries dried using DIC contained higher phenolic compounds, total anthocyanins, and flavonoids than other treatments.
4.2.4 Enzyme-assisted extraction for the recovery of proteins and polyphenols.
Proteins are present in watermelon seeds, and the extraction of these proteins can be improved by enzyme-assisted extraction (EAE). Cellulases and pectinase can be used to disrupt the seed cell walls so that bound proteins and polyphenols become accessible for extraction. EAE also improves the yield of these compounds by lowering the surface barrier to allow easier extraction of the compounds by the solvent. Each of the factors, such as the particle size and the enzyme-to-sample ratio, determines the polyphenol yield in enzymatic hydrolysis extraction. In this method, the sample is made up of enzymes, and the solvent is maintained at a low temperature in the range of 35–50 °C and the pH of the particular enzyme is controlled. Further, the hydrolysis process is stopped by inactivating the enzymes at 80–90 °C, which does not consume high energy as seen in the high-temperature extraction, which creates degradation. Knowledge-based process of extraction using enzymes is highly appreciated for its eco-friendliness since the enzyme works under controlled pH conditions and uses water instead of hazardous chemical solvents. However, the primary downside of EAE is the prolonged extraction time, which ranges from 3–48 hours.85 This method is especially helpful for isolating bioactive peptides and polyphenols that have operational uses in nutraceuticals.86
4.2.5 Pulsed electric field extraction (PEFE) for polyphenols and proteins.
Pulsed Electric Field Extraction (PEFE) is another emerging technique suitable for extracting proteins and polyphenols from watermelon seeds. PEFE weakens the cell membranes, facilitating the diffusion of the matrix and an enhanced solvent entry. This technique also reduces extraction time significantly and increases the amount of biologically active compounds like polyphenols, which are responsible for the antioxidant properties of the seed. PEFE is also cost-effective and has the advantage of energy efficiency; it is suitable for the extraction of sensitive compounds such as fatty acid profiles from watermelon seeds. Niu et al. (2021) revealed that the pulsed electric field (PEF) technology has proven to be a valuable tool in agro-industries, enhancing the selectivity and energy efficiency of extraction processes.87 By applying short bursts of high voltage to plant materials, PEF disrupts cell membranes, facilitating the release of intracellular components. This method has shown promise as a complement or alternative to conventional thermal treatments, particularly in sugar extraction from sugar beets. Its ability to maintain product quality while reducing energy consumption positions PEF as a sustainable and innovative solution for modern extraction technologies. The yields of compounds from plant cells as colourants (carotenoids and chlorophyll), carbohydrates such as sucrose and polyphenols, and other secondary metabolites can be enhanced when plant cells undergo PEF treatment in combination with other methods.
According to the findings of a previous study,88 PEF pre-treatment assisted extraction has the potential to enhance sucrose concentration, enhance the filterability of the juice, and reduce the colloidal impurities as well as color of the produced juice. In the production of wine, PEF has been used before the maceration and fermentation step to control the polyphenol extraction and to give wines with specific organoleptic characteristics. As with prior treatment used in conventional vinification waste, the selectivity of anthocyanin extraction is extended, and colorant yields are optimized. Furthermore, mechanical expressions after moderate PEF treatments have been associated with enhanced yields in fruit juices and vegetable oils.
Experiments showed that PEF treatment does not have a detrimental effect on the taste or flavor of the extracted oil while obtaining slightly cloudy but highly aromatic apple juice with increased polyphenol concentration.
4.2.6 High-pressure extraction (HPE) for lipids and phenolic compounds.
High-Pressure Extraction (HPE) is ideal for extracting lipids and phenolic compounds from watermelon seeds. By maintaining solvents in their liquid state at high pressure, HPE enhances solvent penetration into the seed matrix, improving the extraction efficiency. This method is known for reducing both solvent use and extraction time while preserving the quality of sensitive bioactive compounds. HPE has shown promise in extracting high-quality oil rich in essential fatty acids and phenolic compounds with antioxidant properties from watermelon seeds.89
4.2.7 Ultrasound-assisted extraction (UAE) for oils and antioxidants.
Ultrasound-Assisted Extraction (UAE) is highly effective for extracting oils and antioxidants from watermelon seeds. Ultrasound waves create cavitation, which causes cell breakage, and the mass transfer increases.90 UAE has been demonstrated as an efficient technique for extracting valuable compounds from watermelon seeds. This method significantly reduces the extraction time and the quantity of solvent required, making it a sustainable and cost-effective approach. UAE is particularly effective in recovering oils rich in unsaturated fatty acids, antioxidants, tocopherols, and phenolic compounds from watermelon seeds. This shows its potential in producing high-quality extracts for food, nutraceutical, and cosmeceutical applications. UAE is especially advantageous in recovering heat and oxidation-sensitive bioactives from foods.91
If done alongside other extraction approaches, ultrasound has been found to be very viable. It enhances heat and mass transfer rates, hence appropriate in extraction and complimenting other technologies.92 A previous study showed that ultrasound with the assistance of pressurized liquid extraction (PLE) is efficient for the extraction of phenolic compounds from pomegranate peels to promote the extraction of bioactive compounds.93 Yields increased under ultrasound treatments particularly if particle sizes were large with temperatures of 70–80 °C and ultrasound power of 480–640 W. Combined with PLE, UAE made the utilization of water as a solvent possible and shortened the extraction time. The most studied techniques included supercritical fluid extraction (SFE) and ultrasonic extraction. The influence of ultrasound on the antioxidant extraction from agave bagasse by SFE enhanced the antioxidant extraction yield when applying more than one transducer.91 Co-acoustic extraction using ultrasound and SFE yielded 70% more antioxidant and 200% more saponin extraction than the control. They also discovered that the arrangement of the ultrasound transducers heightened the intensification effect of SFE processes in the geometry of the transducers.91
In the case of high-quality product processing, particularly when focusing on phenolic compounds and essential oils, it is more advantageous to use multiple extraction methods. These integrated approaches not only enhance the extraction of phenolic compounds but also offer novel alternatives for obtaining bioactive compounds from natural sources. For example, bioactive compound extraction from peppers has been reported.94 They first used SFE to remove nonpolar fractions, followed by recovering phenolic compounds from Biquinho peppers through PLE.
5 Therapeutic potential of watermelon seed bioactive compounds
Watermelon seeds have garnered attention for their diverse bioactive potential, contributing to various health benefits (Table 2). Here is an overview of their key bioactive properties.
Table 2 Therapeutic potential of watermelon seed bioactives
Component |
Characteristics |
Therapeutic applications |
Mechanism of action |
Reference |
Triterpenoid |
Cucurbitacin E, anti-inflammatory effects |
Alleviates inflammation; manages conditions linked to reactive nitrogen species |
Inhibits cyclooxygenase enzymes; neutralizes reactive nitrogen species (RNS) |
95
|
Glycosides |
Non-sugar (aglycone) and sugar component |
Exhibits anti-diarrheal properties; used as flavoring agents |
Stimulates saliva and gastric fluid secretion; astringent effects from tannins |
30 and 96 |
Saponins |
High-molecular-weight, surface-active properties |
Treats inflammation, menopausal symptoms, and cardiovascular issues |
Induces hemolysis; toxic in excessive doses; soluble in water |
30
|
Alkaloids |
Nitrogen-containing compounds with notable bioactivity |
Antispasmodics, analgesics, and antibacterial effects |
Act as stimulants for the CNS and provide analgesic properties |
97
|
Coumarins |
Exhibit anticancer properties |
Effective against abnormal cell growth in cancers |
Induce apoptosis and inhibit cancer cell proliferation |
98
|
Watermelon seed extracts |
Extracts from inner (KI) and outer (KD) layers |
Investigated for lung and breast cancer treatment |
Dose-dependent cytotoxic effects, inhibiting tumor growth |
99
|
Amino acids |
Rich in essential amino acids (e.g., phenylalanine, arginine, valine) |
Dietary supplements for protein synthesis and tissue repair |
Contribute to protein synthesis and metabolic processes |
27
|
Phytochemicals and cations |
Bioactive compounds and essential cations |
Management of hypertension and cardiovascular health |
Induces vasodilation, inhibit ACE, and enhance nitric oxide synthesis |
31
|
Proteins, lipids, and antioxidants |
High in proteins, lipids, vitamins, and minerals |
Anti-diabetic, cardioprotective, anti-ulcerogenic, anti-obesity |
Enhance insulin sensitivity, modulates lipid profiles, reduce oxidative stress |
100
|
5.1 Antioxidant activity
Watermelon seeds have demonstrated excellent antioxidant capacity (Table 2) and are significantly capable of reducing free radicals. Various studies have reported the antioxidant effects of watermelon seeds and specific focus has been placed on different seed types, including Charleston gray, Crimson sweet, and Black diamond seeds. These varieties showed rich scavenging capability by evaluating the DPPH radical scavenger activity, which was found to be 59.88%, 94.46%, and 70.06%, respectively. A higher concentration of watermelon seeds has a linear relationship with an enhancement of the scavenging activity of up to 90% at 1000 μg mL−1 of concentration.7,101 Moreover, the flour produced from watermelon seed undergoing a blending process exhibited strong antioxidant activity, adopting the ABTS and DPPH tests.102 These in vivo studies have also shown that watermelon pulp and seed extracts increase the SOD and catalase, which are useful in unfolding oxidative stress.102 In addition, studies have shown that the watermelon seed proteins release antioxidant peptides for the protection of cells subjected to oxidative stress.103 Several research studies underscore the abundance of citrulline in watermelon flesh, which is a nonessential amino acid with antioxidant capacity and is metabolized to arginine, which is anti-inflammatory in nature.104–106 Employing this same concept with watermelon, a source that contains high amounts of vitamin C, a hydrophilic antioxidant, hinders the oxidation of iron and clears free radicals simultaneously.107,108 It also suggests that its antioxidant ability outperforms strawberries, tomatoes, and guava, which means the levels of protection against inflammation and cancer.10,109 Different fractions of watermelon seeds, especially those extracted by methanol, have been proven to possess high antioxidant effects and can be exploited in future nutraceutical markets.109,110
5.2 Hypoglycemic and hypolipidemic properties
Watermelon seed extracts have been studied for their potential to manage glucose levels and lipid profiles. These extracts have been found to have the potential to suppress the alpha-amylase and alpha-glucosidase effects, which in turn help to assist insulin release from the pancreatic beta cells.4 Studies on diabetic rats have shown an enhanced flow of serum glucose and improved lipid profiles with the addition of watermelon seed kernels, which suggests the kernels could be used as a hypoglycemic and hypolipidemic substance. Additional studies corroborate these findings, and data shows that using seed extracts positively influences plasma glucose levels in diabetic rats, thereby enhancing general lipid pmettabolism.111
5.3 Anti-inflammatory properties
Watermelon seeds exhibit remarkable anti-inflammatory properties, as demonstrated in studies assessing their ability to stabilize human red blood cell membranes and prevent tissue damage. Methanolic extracts of watermelon seeds have shown a significant protection percentage of 74.8%, while aqueous extracts results are not very different from methanolic extracts, albeit to a lesser extent. Watermelon seed extracts have almost similar anti-inflammatory effects to aspirin, and extracts have a tendency to inhibit albumin denaturation. The carotenoid pigment, lycopene present in watermelon seeds and its juice, is a lipid-soluble antioxidant that can significantly decrease inflammation and cholesterol and can also protect against cancer.30 Watermelon seed extracts, particularly those obtained using methanol as a solvent, have demonstrated significant antioxidant potential. These extracts contain bioactive compounds capable of neutralizing free radicals and preventing oxidative stress. Such properties make them promising candidates for applications in the nutraceutical industry, where they can contribute to the development of functional foods and supplements aimed at promoting health and preventing diseases linked to oxidative damage.109 The use of watermelon rind as a source of antioxidants and anti-inflammatory has also been supported in various research studies, where rind extracts improved lipid profiles and exhibited anti-inflammatory effects in animal models.112
5.4 Antibacterial and antifungal activity
It is well established that watermelon seeds possess strong antimicrobial activity and can be used as replacements for normal antimicrobial substances. Consequently, the inhibition of various bacterial and fungal strains is attributed to secondary metabolisms such as flavonoids, tannins, and saponins.88,113 It has also been observed that methanolic and aqueous extracts of watermelon seed inhibit the growth of Staphylococcus species; this explains the antimicrobial activity of the seeds whereby flavonoids saponin and tannin bioactive compounds have been established to inhibit bacterial as well as fungi.114
Also, the aqueous partition of watermelon seed showed the presence of bioactive compounds using cold and hot water, ethanol, and methanol, which exhibited antimicrobial activities against bacteria and fungi.4,115 Antimicrobial activities in chloroform extracts were accelerated due to the presence of watermelon antioxidants, type of phenols, flavonoids, tannins, and saponins. It has been considered that lycopene has antioxidant activity,4,113 but it has also been established that it has antimicrobial activity.116,117 Ethyl acetate-treated watermelon seed extracts showed remarkable activity against S. aureus, P. aeruginosa and Klebsiella species.118 It is also pointed out that melanin has antibacterial effects.119
5.5 Anticancer properties
Research reveals that watermelon seeds can act as anticancer agents, particularly for gastric and breast cancers. Research has further shown that watermelon seed extract possesses cytotoxic effects on gastric cancer cells with the IC50 value of 453.76 μg mL−1 after 24 h.120 Studies performed on MCF-7 breast cancer cells and vaginal melanoma cancer cells also underline these findings, arguing that both watermelon pulp and seed extracts reduce cancer cell proliferation while simultaneously exhibiting high selectivity towards cancer cells.102 One of the benefits of consuming seeds is that they contain antioxidants that can destroy cancer cells. Lycopene found in watermelon has been researched widely for its ability to slow down tumor progression and inhibit cancer in various organs.121 This compound inhibits cancer cell growth and carcinogenic activity, and it has been found that the intake of the lycopene substance can also mitigate the negative impacts of cancer treatments like radiotherapy.122,123 Citrullus violaceus seeds are also abundant in β-carotene and other phenolic contents that enhance this fruit's anticancer agent.124
5.6 Anti-ulcer properties
Watermelon seeds also exhibit anti-ulcer properties, as demonstrated in studies involving albino Wistar rats. Methanolic extracts of watermelon seeds significantly reduced ulcer indices in both pyloric ligated and water immersion stress-induced rats and were almost at par with standard drugs like ranitidine and omeprazole.125
5.7 Cardio-protective effects
The nutrients, phytochemicals, vitamins, minerals, and water in the watermelon enhance cardiovascular health due to the presence of lycopene, beta-carotene and unsaturated fatty acids, acting as both antioxidants and anti-inflammatory compounds. Some of these compounds aid in decreasing the chances of CVD by bringing down inflammation and stressful oxidation.30,126 The cardio-protective effects of watermelon seeds are credited to the presence of citrulline, arginine, and lycopene in watermelon juice, which enhances vasodilator to relax and widen blood vessels, hence reducing hypertension and high blood pressure.127 Watermelon extract helps obese adults lower their blood pressure, thus lowering the risk of hypertension. Also, potassium found in watermelon has been acknowledged to regulate heartbeat and healthy blood pressure levels due to research done previously.128 In the same vein, the watermelon seed oil's antioxidants help prevent chronic diseases like CVDs; more in vivo studies show that watermelon seed extract can prevent heart diseases by lowering specific heart disease indices.70,95
5.8 Hepatoprotective properties
Non-alcoholic fatty liver disease (NAFLD) can originate from simple steatosis, and if left untreated, it may progress to non-alcoholic steatohepatitis (NASH). It is indicated by smooth hepatocellular damage and may result in cirrhosis.129,130 Chronic liver injury is characterised by inflammation and lobular ballooning; it progresses to cirrhosis, the most serious and irreversible stage of the disease, whereas NAFLD and NASH are fully reversible if diagnosed early.131 To date, only lifestyle interventions have been identified to reverse NAFLD and these include a change in diet and use of supplements.132–134 It was observed that watermelon seed extract has the potential to prevent hepatic oxidative stress and protect the liver. Studies have shown that treatment with these extracts helps to lower the levels of such biomarkers of oxidative stress as aspartate amino-transferase (AST), alanine amino-transferase (ALT) and alkaline phosphates (ALP) in models of ethanol-induced hepatotoxicity. Further, the extracts have been shown to effectively scavenge the H2O2-induced oxidative injury of HepG2 cells and reduce inflammation and hepatocyte damage in acetaminophen administration models.103 Furthermore, watermelon seed oil has the potential to prevent the liver from oxidative stress and improve hepatic enzymes in animal models.
5.9 Nephropathy
Chronic kidney malfunctions frequently indicate kidney diseases, usually termed chronic kidney disease (CKD) or end-stage renal disease (ESRD). These conditions are inexorable and cumulative and subsequently will build up gradually and persist. The main factors are hypertension, diabetes mellitus, interstitial nephritis and chronic pyelonephritis, secondary glomerulonephritis, congenital conditions including cystic diseases, and vasculitis. Chronic renal failure (CRF) is the stage of chronic kidney disease in which kidney dysfunction is irreversible and progresses to a low glomerular filtration rate accompanied by a steady increase in plasma creatinine concentration.134
The progression of CRF is marked by three main stages: decreasing kidney performance, ESRD and kidney failure. Some of the significant factors that dictate the progression are the degree of proteinuria, hypertension, and compromised renal function. It has been shown that patients developing proteinuria or hypertension have a shorter duration of the disease.31 Research carried out reveals that lycopene possessing biochemical and histopathological effects prevents the occurrence of kidney disease.27,135 However, it is also beneficial to have other compounds combined with lycopene, including arginine and citrulline, found in watermelon. Arginine-packed nutritional products are especially beneficial to patients diagnosed with kidney failure. Arginine normalizes the vasoconstrictor effect of dimethylarginine, a non-NO substrate implicated in the pathogenesis of renal diseases. The explicit regulation of these biochemical pathways suggests that arginine supplementation may benefit kidney-related diseases.136,137
Watermelon seeds have therapeutic potential against hyperuricemia, as evidenced by studies on potassium oxonate-induced hyperuricemic albino rats. It has been found that the watermelon seeds have the potential to decrease uric acid, creatinine, and urea however globulin, albumin and total level of serum proteins reduce the activity of xanthine oxidase enzyme.138 This means that in addition to reducing kidney inflammation, the diuretic nature of watermelon seeds may prevent the formation of kidney stones as well as promote regular bowel movement, and hence the name diuretic.
5.10 Improvement in reproductive health
Watermelon seeds have been found to improve reproductive health, especially male fertility. The number of sperms in a sample and its motility and viability in Wistar rats increased by the administration of hydroalcoholic extracts of watermelon seeds, as revealed by Khaki and his team in their study. Also, these extracts have effectiveness against toxicity-mediated reproductive health complications, sexual viability, and benign prostatic hyperplasia, and experimental models have demonstrated shrinkage in size and weight of the prostates.139 The seeds contain arginine and phenolic compounds, which may contribute to their potential effectiveness in addressing erectile dysfunction.96
5.11 Neurological disorders
Lycopene and its metabolites have been identified in the brain, where they are expected to have neuroprotective effects. Several studies have endorsed the potential of lycopene in treating psychiatric and neurodegenerative diseases.140 Lycopene also reduced Aβ-induced cellular toxicity in cultured neurons in tau transgenic mice expressing the P301L mutation.140 This finding is based on a study that showed lycopene possesses the potential to mitigate motor dysfunction and learning disability, which are features of HD in animal subjects caused by 3-NP, which inhibits succinic acid dehydrogenase. Similarly, a study conducted on a PD model demonstrated that lycopene has the potential to suppress motor dysfunction resulting from MPTP, a compound that produces PD-like manifestations. It was also established that lycopene has neuroprotective effects against CNS disorders by decreasing oxidative stress and bioactive molecules influencing signal transduction cascades, including activation of protein kinase B, phosphatidylinositol-4,5-bisphosphate 3-kinase, adenosine 5′ monophosphate-activated protein kinase and peroxisome proliferator-activated receptor-γ.68
5.11 Analgesic properties
The vibrant red color of watermelon is attributed to substantive doses of lycopene present in it, which accounts for its analgesic attributes. The antinociceptive effect of watermelon seed extracts has also been investigated through in vivo approaches, suggesting their potential as functional ingredients in the formulation of pain-relieving products.30,112
6. Applications of water melon seeds
Watermelon seeds are rich in phytochemical compounds and high unsaturated fatty acids, demonstrating significant biological activity, particularly in combating free radicals.15 Due to these properties, watermelon seeds are considered valuable economic ingredients with potential applications across various industries, including food, cosmeceuticals, and nutraceuticals. The seeds have the highest oil content among their components, followed by protein, making them particularly useful in food products where these nutrients are desirable. In food applications, the protein and phytochemical contents are often emphasized, while the high unsaturated fatty acids and phytochemicals make them attractive for cosmeceutical uses. By harnessing these beneficial properties, watermelon seeds can be effectively utilized in commercial products, offering a range of health and nutritional benefits.
6.1 Food applications of water melon seeds
Watermelon seeds are widely used in food applications due to their rich protein (14–30%) as well as oil (40–50%) content. After the extraction of oil from watermelon seeds, the rest of the de-fatted watermelon seed meal, which is highly rich in proteins, can be used. In several areas of Africa, Arabia, India and Asia, salted and roasted watermelon seeds are consumed as snacks and are used in confectionery.70 The seeds provide high-quality proteins and can be used as protein supplements in food manufacturing (Table 3). For example, watermelon seed kernel flours have been used in the production of biscuits for people with gluten intolerance as well as low-carb consumers. Not only does the addition of toasted watermelon seed flour increase the protein quantity of these biscuits, but it also gives unique flavours and structures.148 According to a previous study,149 there is a possibility of using WSF at 5% as a functional ingredient that can boost the nutritive value of low-carbohydrate, high-protein bread for the elderly and active people.
Table 3 Application of watermelon seeds in the food industry
Food products |
Processing |
Results |
References |
Fortified bread |
0, 15, and 25% watermelon seed flour in wheat flour |
Nutrient content (protein, iron, calcium, potassium, phosphorus) increased significantly; sensory attributes unchanged |
141
|
Brown rice-watermelon seeds snacks |
90% brown rice flour, 10% watermelon seed powder, 120–140 °C, 300–420 rpm |
Protein content increased; phenolic content enhanced; intermediate glycemic index |
142
|
Watermelon seed extruded snacks |
Rice-corn flour blend with 10%, 15%, and 20% seed flour |
Maintained quality over 90 days; favourable proximate composition and sensory attributes |
143
|
Toasted watermelon seed meal biscuits |
Composite flour with toasted seed meal |
Increased protein, fat, crude fiber, and ash content; desirable sensory attributes |
144
|
Cookies with watermelon seed flour |
Substituting wheat flour with seed flour at 0%, 10%, 20%, 30%, 40% |
Higher protein, fat, and fiber; favourable sensory evaluations across formulations |
145
|
Bread from wheat-watermelon seed flour |
Blends of wheat and watermelon seed flour |
Bulk density, water absorption, oil absorption, moisture, and protein content varied; preferred blend noted |
146
|
Ice cream |
Milk fat and solid substitution with seed flour |
Increased fat, protein, total solids; best sensory quality at 10% substitution |
147
|
Apart from biscuits, watermelon seed flour has also been used in bread, fortification leading to a drastic increase in bread proteins, iron, calcium, potassium and phosphorus content without negative effect on bread sensory attributes as noted previously.150 With some applications, the complete watermelon seed matter, including the oil fraction, is employed. Spray drying is commonly used to change watermelon seeds into a powdery form to enhance their applicability in various foods. The dehulled watermelon seed flour has also been used as a margarine substitute in the cookies, improving the functional properties such as water and oil absorption.24
The oil extracted from watermelon seeds contains essential and unsaturated fatty acids, such as linoleic and oleic acids, which are conspicuous and beneficial for frying and cooking processes across Africa and some parts of the Middle East due to their unique flavor.70 The seeds are eaten in Nigeria, such as Ootanga, and the extracted oil is known as Kalahari oil.151 Another new product is watermelon seed milk; the work indicates this product's potential to be used as a partial replacement for cow's milk in yogurt production. The biochemical compositions of yogurt made from watermelon seed milk and those of the control cow's milk yogurt show that the milk yogurt contains 2.72% more total solids, 2.25% more ash, 1.78% more fiber content and 1.5 times more bioactive compounds than control yogurt. It also possesses a high antioxidant potential and is a better option.123
Watermelon seeds have also been used in ice cream as fat replacers because they have a high fat content, which gives a creamy texture to the final product. Watermelon seed flour-added ice creams contain higher fat and protein compared with cow's milk ice cream but this product may experience lower viscosity since the seeds contain unsaturated fatty acids. In traditional Nigerian snack foods – “robo,” watermelon seeds add up to amino acid contents, vitamin, and minerals content.152 Also, fermented condiments with watermelon seed pulp contain higher fat and protein compared to its seed flour and improved as an additive for soup thickeners, like increasing water and oil absorption capacity as well as calcium and thiamine content as postulated.151,153
6.2 Nutraceutical applications of watermelon seeds
Watermelon seeds are rich in essential fatty acids, proteins, minerals, and bioactive compounds, and they are becoming a promising source for nutraceutical supplementation. Unsaturated fatty acids, especially oleic and linoleic acids, which are good for the heart, are present in large amounts in these seeds. Watermelon seeds have a high concentration of antioxidants, including flavonoids and phenolic compounds, which help prevent oxidative stress and free radical damage linked to chronic illnesses like diabetes, cancer, and cardiovascular disease. The Na/K ratio of watermelon seeds varies from 0.06 to 0.29, depending on whether the values are for the whole, peeled, or skinned seed. Because of this lower Na/K ratio, watermelon seeds may help lower blood pressure and other cardiovascular diseases such as hypertension.154 Furthermore, a previous study 70 identified the presence of phytosterols in watermelon seed oil, including β-sitosterol, camp sterol, and stigmasterol.
Watermelon seeds have a particularly noteworthy protein content when it comes to nutraceuticals. They are an important source of protein, particularly for plant-based diets, since they offer the full spectrum of essential amino acids. The minerals magnesium, potassium, and iron, which are abundant in the seeds, support a number of body processes, including the health of the bones, muscles, and oxygen transport. Watermelon seed protein has been shown to contain antioxidant peptides with pharmaceutical properties. After the purification of five peptides, it was found that peptide RDPEER protected HepG2 cells against H2O2-induced oxidative damage by decreasing ROS, raising antioxidant enzyme levels, and decreasing the amount of malondialdehyde (MDA).23,155 Watermelon seed meal was utilized to make a defatted flour and a protein isolate.52 They concluded that watermelon seeds are a good source of protein, although they have moderate quality, with methionine and cysteine identified as the limiting amino acids. The bioactive compounds present in watermelon seeds, including cucurbitacin, have shown anti-inflammatory, antioxidant and anti-cancer properties in preliminary studies. These properties make watermelon seeds a powerful nutraceutical ingredient with the potential to support overall health and prevent various chronic conditions.30
7. Conclusion
Watermelon seeds, often regarded as agricultural waste, hold immense potential as a source of essential nutrients and bioactive compounds. The present review highlights the pharmacological prospects of watermelon seeds, such as their antioxidant, antimicrobial, antimutagenic, hypoglycemic and anti-inflammatory effects and importance to human health. This review discusses various extraction methods that have not been utilized fully in the current healthcare practices, reviews their working mechanisms, and highlights the huge research space involved in the medical research of these by-products. The proposed revalorization of watermelon seeds shows potential for generating innovation in food processing and nutraceutical and cosmeceutical sectors and can contribute towards sustainability and a circular economy. However, the limitations, including variation in the composition of seeds, technology for extraction, and safety of bioactive extracts, remain problematic in order to popularize bioactive compounds.
Further studies should also emphasise how to improve extraction methods to become consistent, confirm the safety and effectiveness of the products from watermelon seeds and search for other opportunities for their usage to come into full effect. Therefore the changing the perspectives of these by products from waste to potential resources, watermelon seeds have a great significance to positively influence human health and act as a push for paving way for sustainable food processing.
Consent form
No animal and human studies were conducted as this is a review article.
Data availability
Data sharing is not applicable to this article as no datasets were generated or analysed during the current study.
Conflicts of interest
There is no conflict of interest between the authors.
References
- C. O. Karikpo, E. S. Bartimaeus and B. Holy, Evaluation of the cardioprotective effect of Citrullus lanatus (watermelon) seeds in streptozotocin induced diabetic albino rats, Asian J. Biochem. Genet. Mol. Biol, 2019, 1(4), 1–6 Search PubMed.
- S. Zia, M. R. Khan, M. A. Shabbir and R. M. Aadil, An update on functional, nutraceutical and industrial applications of watermelon by-products: A comprehensive review, Trends Food Sci. Technol., 2021, 114, 275–291 CrossRef CAS.
-
FAO. Watermelon | Land & Water | Food and Agriculture Organization of the United Nations. 2023. https://www.fao.org/land-water/databases-and-software/crop-information/watermelon/en/ Search PubMed.
- D. Neglo, C. O. Tettey, E. K. Essuman, N. K. Kortei, A. A. Boakye, G. Hunkpe, F. Amarh, P. Kwashie and W. S. Devi, Comparative antioxidant and antimicrobial activities of the peels, rind, pulp and seeds of watermelon (Citrullus lanatus) fruit, Sci. Afr., 2021, 11, e00582 CAS.
- S. Zamuz, P. E. Munekata, B. Gullón, G. Rocchetti, D. Montesano and J. M. Lorenzo,
Citrullus lanatus as source of bioactive components: An up-to-date review, Trends in Food Science & Technology, 2021, 111, 208–222 Search PubMed.
- Z. Nishat, M. Y. Quddoos, N. Shahzadi, K. Ameer, I. A. Ahmed, S. Yaqub, S. Mukhtar, S. Mahmood, A. Rafique, M. S. Raza and A. Shahzad, Probing the physicochemical impact of musk melon seed oil on mayonnaise, Ital. J. Food Sci., 2024, 36(2), 181–194 Search PubMed.
- L. R. Yadav, D. Kumar, C. Kavitha, H. Rajanaika, B. D. Prasad, H. Nagabhushana and G. Nagaraju, Antibacterial and photocatalytic activities of ZnO nanoparticles: synthesized using water melon juice as fuel, Int. J. Nanosci., 2016, 15(01n02), 1650006 CrossRef CAS.
- G. Bianchi, L. Provenzi and A. Rizzolo, Evolution of volatile compounds in ‘Cuoredolce®’and ‘Rugby’mini-watermelons (Citrullus lanatus (Thunb.) Matsumura and Nakai) in relation to ripening at harvest, J. Sci. Food Agric., 2020, 100(3), 945–952 CrossRef CAS PubMed.
- M. I. Dammak, Y. B. Salem, A. Belaid, H. B. Mansour, S. Hammami, D. Le Cerf and H. Majdoub, Partial characterization and antitumor activity of a polysaccharide isolated from watermelon rinds, Int. J. Biol. Macromol., 2019, 136, 632–641 CrossRef CAS PubMed.
- I. Tlili, R. Ilahy, L. Romdhane, T. R’him, H. Ben Mohamed, H. Zgallai, Z. Rached, M. Azam, I. Henane, M. N. Saïdi and Z. Pék, Functional quality and radical scavenging activity of selected watermelon (Citrullus lanatus (Thunb.) Mansfeld) genotypes as affected by early and full cropping seasons, Plants, 2023, 12(9), 1805 CrossRef CAS PubMed.
- X. Rico, B. Gullón, J. L. Alonso and R. Yáñez, Recovery of high value-added compounds from pineapple, melon, watermelon and pumpkin processing by-products: An overview, Food Res. Int., 2020, 132, 109086 CrossRef CAS PubMed.
- F. Boukid, J. Pera, J. Parladé and M. Castellari, Fungal bioconversion of brewery by-products: Assessment of fatty acids and sterols profiles, Qual. Assur. Saf. Crops Foods, 2022, 14(4), 202–211 CrossRef CAS.
- M. K. Samota, M. Kaur, M. Sharma, V. Krishnan, J. Thakur, M. Rawat, B. Phogat and P. N. Guru, Hesperidin from citrus peel waste: extraction and its health implications, Qual. Assur. Saf. Crops Foods, 2023, 15(2), 71–99 CrossRef CAS.
- N. A. Sagar, S. Pareek, S. Sharma, E. M. Yahia and M. G. Lobo, Fruit and vegetable waste: Bioactive compounds, their extraction, and possible utilization, Compr. Rev. Food Sci. Food Saf., 2018, 17(3), 512–531 CrossRef CAS PubMed.
- A. Petchsomrit, M. I. McDermott, S. Chanroj and W. Choksawangkarn, Watermelon seeds and peels: fatty acid composition and cosmeceutical potential, OCL:Oilseeds Fats, Crops Lipids, 2020, 27, 54 CrossRef CAS.
- P. Dash and G. Ghosh, Fractionation, amino acid profiles, antimicrobial and free radical scavenging activities of Citrullus lanatus seed protein, Nat. Prod. Res., 2017, 31(24), 2945–2947 CrossRef CAS PubMed.
- C. Ukwubile, U. Idriss and A. Isah, Phytochemical evaluation, in vitro-in vivo antioxidant and cytotoxicity activities of various layers of watermelon fruit Citrullus lanatus (Cucurbitaceae) Matsum. & Nakai, Prog. Chem. Biochem. Res., 2022, 5, 97 CAS.
- A. D. Osinubi, O. O. Banjoko, O. H. Anselm, O. M. Akinrinola and A. Osofodunrin, Comparative effects of drying methods on phytochemical contents and anti-microbial activities of watermelon (Citrullus lanatus) seed and rind, J. Chem. Soc. Niger., 2020, 45(1), 70–78 Search PubMed.
- S. Iswariya and T. S. Uma, Evaluation of in vitro anti-inflammatory and antimicrobial activity of aqueous and methanolic seed extracts of Citrullus lanatus, Int J Pharm Pharm Sci., 2017, 9(5), 29–33 CrossRef CAS.
- A. S. Vinhas, C. S. Silva, C. Matos, C. Moutinho and A. Ferreira da Vinha, Valorization of watermelon fruit (Citrullus lanatus) byproducts: phytochemical and biofunctional properties with emphasis on recent trends and advances, World J. Adv. Healthc. Res., 2021, 5(1), 302–309 Search PubMed.
- M. S. Hasanin and A. H. Hashem, Eco-friendly, economic fungal universal medium from watermelon peel waste, J. Microbiol. Methods, 2020, 168, 105802 CrossRef CAS PubMed.
- D. K. Nkoana, J. Mashilo, H. Shimelis and R. M. Ngwepe, Nutritional, phytochemical compositions and natural therapeutic values of citron watermelon (Citrullus lanatus var. citroides): a review, S. Afr. J. Bot., 2022, 145, 65–77 CrossRef CAS.
- E. Franca, Chemical properties of watermelon seed and the utilization of dehulled seed in cookies production, Carpathian J. Food Sci. Technol., 2017, 9(1), 126–135 Search PubMed.
- T. Kausar, M. T. Hassan and G. M. ud Din, 21. Utilization of watermelon seed flour as protein supplement in cookies, Pure Appl. Biol., 2020, 9(1), 202–206 CAS.
-
B. Tabiri, J. K. Agbenorhevi, F. D. Wireko-Manu and E. I. Ompouma. Watermelon seeds as food: Nutrient composition, phytochemicals and antioxidant activity.
- F. D. Benmeziane, Composition, bioactive potential and food applications of watermelon (citrullus lanatus) seeds–a review, J. Food Meas. Charact., 2023, 17(5), 5045–5061 CrossRef.
- V. Enemor, C. Oguazu, A. Odiakosa and S. Okafor, Research Article Evaluation of the Medicinal Properties and Possible Nutrient Composition of Citrullus lanatus (Watermelon) Seeds, Res J Med Plant, 2019, 13, 129–135 CrossRef CAS.
- G. Alka, S. Anamika and P. Ranu, A review on watermelon (Citrullus lanatus) medicinal seeds, J. Pharmacogn. Phytochem., 2018, 7(3), 2222–2225 CAS.
- H. B. Zainab, U. I. Aminu, I. Mustapha and S. T. Adam, Proximate Analysis and Antinutritional Factors of Water Melon Seeds (Citrullus lenatus), Int. J. Biochem. Res. Rev., 2021, 30(8), 41–47 CrossRef.
- M. Nadeem, M. Navida, K. Ameer, A. Iqbal, F. Malik, M. A. Nadeem, H. Fatima, A. Ahmed and A. Din, A comprehensive review on the watermelon phytochemical profile and their bioactive and therapeutic effects, Food Sci. Preserv., 2022, 29(4), 546–576 Search PubMed.
- S. Sajjad, B. Israr, F. Ali and I. Pasha, Investigating the effect of phytochemicals rich watermelon seeds against hypertension, Pak. J. Agric. Sci., 2020, 57(4), 1157–1164 Search PubMed.
- S. A. Omoniyi, Nutrient and anti-nutritional composition of Watermelon (Citrullus lanatus) seed: A review, FTSTJ, 2020, 5, 048–051 Search PubMed.
- A. C. Egbuonu and C. Anthony Cemaluk, Assessment of some antinutrient properties of watermelon (Citrullus lanatus) rind and seed, Res. J. Environ. Sci., 2015, 9(5), 225–232 CrossRef CAS.
- G. Rekha and A. L. Rose, Proximate nutritional analysis of dried watermelon seed, Int J Eng Res Gen Sci., 2016, 4(6), 44–46 Search PubMed.
- E. A. Mogotlane, P. W. Mokwala and P. Mangena, Comparative analysis of the chemical compositions of indigenous watermelon (Citrullus lanatus) seeds from two districts in Limpopo Province, South Africa, Afr. J. Biotechnol., 2018, 17(32), 1001–1006 CrossRef CAS.
- B. Tabiri, J. K. Agbenorhevi, F. D. Wireko-Manu and E. I. Ompouma, Watermelon seeds as food: Nutrient composition, phytochemicals and antioxidant activity, Int. J. Food Sci. Nutr., 2016, 5(2), 139–144 CrossRef CAS.
- M. Islam, M. S. Islam, S. Zannah, G. Sadik and M. Rashid, Momordica charantia (Bitter melon) in combination with metformin potentiates hypoglycemic and hypolipidemic effects in alloxan-induced diabetic rats, Bangladesh Pharm. J., 2018, 21(2), 109–117 CrossRef.
- H. R. Mahla, S. S. Rathore, K. Venkatesan and R. Sharma, Analysis of fatty acid methyl esters and oxidative stability of seed purpose watermelon (Citrullus lanatus) genotypes for edible oil, J. Food Sci. Technol., 2018, 55, 1552–1561 CrossRef CAS PubMed.
-
B. Sultana and R. Ashraf, Watermelon (Citrullus lanatus) Oil, in Fruit OilsChemistry and Functionality, 2019, 741–756 Search PubMed.
- R. Ekanem, Detection and quantification of potential bioactive compounds in the acetone extract of Citrullus lanatus (Melon) seed using GC-MS and FTIR 4 spectrometer, Asian J. Chem. Sci., 2018, 5, 1–5 CrossRef.
- T. O. Jimoh, A. O. Ademiluyi, G. Oboh and A. A. Boligon, Phenolic extracts and amino acids content from Cucumeropsis mannii naudin and Citrullus lanatus inhibit relevant enzymes of erectile dysfunction in rat's penile tissue, Biochem. Biophys. Rep., 2017, 12, 5–11 Search PubMed.
- J. Fan, E. Park, L. Zhang, I. Edirisinghe, B. Burton-Freeman and A. K. Sandhu, Pharmacokinetic parameters of watermelon (rind, flesh, and seeds) bioactive components in human plasma: a pilot study to investigate the relationship to endothelial function, J. Agric. Food Chem., 2020, 68(28), 7393–7403 CrossRef CAS PubMed.
- A. D. Assefa, O. S. Hur, N. Y. Ro, J. E. Lee, A. J. Hwang, B. S. Kim, J. H. Rhee, J. Y. Yi, J. H. Kim, H. S. Lee and J. S. Sung, Fruit morphology, citrulline, and arginine levels in diverse watermelon (Citrullus lanatus) germplasm collections, Plants, 2020, 9(9), 1054 CrossRef CAS PubMed.
- C. Wen, J. Zhang, H. Zhang, Y. Duan and H. Ma, Effects of divergent ultrasound pretreatment on the structure of watermelon seed protein and the antioxidant activity of its hydrolysates, Food Chem., 2019, 299, 125165 CrossRef CAS PubMed.
- C. Wen, J. Zhang, Y. Feng, Y. Duan, H. Ma and H. Zhang, Purification and identification of novel antioxidant peptides from watermelon seed protein hydrolysates and their cytoprotective effects on H2O2-induced oxidative stress, Food Chem., 2020, 327, 127059 CrossRef CAS PubMed.
- M. A. Khan, R. M. Amir, K. Ameer, A. Rakha, F. Faiz, I. Hayat, M. Nadeem, Z. Ahmed, A. Riaz and I. Ashraf, Characterization of oat bran β-glucan with special reference to efficacy study to elucidate its health claims for diabetic patients, Food Sci. Technol., 2020, 41(1), 105–112 CrossRef.
- Q. W. Zhang, L. G. Lin and W. C. Ye, Techniques for extraction and isolation of natural products: A comprehensive review, Chin. Med., 2018, 13, 1–26 CrossRef CAS PubMed.
- Q. Xu, M. Hu, M. Li, J. Hou, X. Zhang, Y. Gao, B. Chachar and X. Li, Dietary bioactive peptide alanyl-glutamine attenuates dextran sodium sulfate-induced colitis by modulating gut microbiota, Oxid. Med. Cell. Longevity, 2021, 2021(1), 5543003 CrossRef PubMed.
- P. W. Addo, J. K. Agbenorhevi and D. Adu-Poku, Antinutrient contents of watermelon seeds, MOJ Food Process. Technol., 2018, 6(2), 237–239 Search PubMed.
- P. K. Mukherjee, S. Singha, A. Kar, J. Chanda, S. Banerjee, B. Dasgupta, P. K. Haldar and N. Sharma, Therapeutic importance of Cucurbitaceae: A medicinally important family, J. Ethnopharmacol., 2022, 282, 114599 CrossRef CAS PubMed.
-
L. Nahar and S. D. Sarker, in Chemistry for Pharmacy Students: General, Organic and Natural Product Chemistry, John Wiley & Sons, 2019 Search PubMed.
- M. Akshaya, S. Sasikala, V. Nithyalakshmi, N. Meenakshi, K. Dhivya Kiruthika and M. S. Pavithra, Effect of pre-treatments on the phytochemical composition of watermelon (Citrullus lanatus) rind, Int. Food Res. J., 2018, 25(5), 1081 Search PubMed.
- B. Salehi, C. Quispe, J. Sharifi-Rad, L. Giri, R. Suyal, A. K. Jugran, P. Zucca, A. Rescigno, S. Peddio, O. Bobiş and A. R. Moise, Antioxidant potential of family Cucurbitaceae with special emphasis on Cucurbita genus: A key to alleviate oxidative stress-mediated disorders, Phytother. Res., 2021, 35(7), 3533–3557 CrossRef PubMed.
-
G. Wu, C. J. Meininger, C. J. McNeal, F. W. Bazer and J. M. Rhoads, Role of L-arginine in nitric oxide synthesis and health in humans, in Amino acids in nutrition and health: Amino acids in gene expression, metabolic regulation, and exercising performance, Advances in Experimental Medicine and Biology, ed. G. Wu, Springer, Cham, 2021, ch. 10, vol. 1332, pp. 167–187 Search PubMed.
- B. Zieniuk and M. Pawełkowicz, Recent Advances in the Application of Cucurbitacins as Anticancer Agents, Metabolites, 2023, 13(10), 1081 CrossRef CAS PubMed.
- H. As’ ari, Influence of administering watermelon rind water extracts (citrullus vulgaris schard) on glucose level of male white rats (Rattus norvegicus) induced with streptozotosin, Folia Med. Indones (2355-8393), 2020, 56(3), 661–680 Search PubMed.
- B. Hameed, Q. Ali, M. M. Hafeez and A. Malik, Antibacterial and antifungal activity of fruit, seed and root extracts of Citrullus colocynthis plant, BCSRJ, 2020, 33, 1–5 Search PubMed.
- S. Alseekh, L. P. de Souza, M. Benina and A. R. Fernie, The style and substance of plant flavonoid decoration; towards defining both structure and function, Phytochemistry, 2020, 174, 112347 CrossRef CAS PubMed.
- P. Mostashari and A. Mousavi Khaneghah, Sesame Seeds: A Nutrient-Rich Superfood, Foods, 2024, 13(8), 1153 CrossRef CAS PubMed.
- L. Jurasova, T. Jurikova, M. Baron and J. Sochor, Content of selected polyphenolic substances in parts of grapevine, Italian Journal of Food Science, 2023, 35(3), 17–43 CrossRef CAS.
- S. Khatri, A. Paramanya and A. Ali, Phenolic acids and their health-promoting activity. Plant and Human Health, Phytochemistry and Molecular Aspects, 2019, 2, 661–680 Search PubMed.
- M. Parcheta, R. Świsłocka, S. Orzechowska, M. Akimowicz, R. Choińska and W. Lewandowski, Recent developments in effective antioxidants: The structure and antioxidant properties, Materials, 2021, 14(8), 1984 CrossRef CAS PubMed.
- R. Apak, A. Calokerinos, S. Gorinstein, M. A. Segundo, D. B. Hibbert, İ. Gülçin, S. Demirci Çekiç, K. Güçlü, M. Özyürek, S. E. Çelik and L. M. Magalhães, Methods to evaluate the scavenging activity of antioxidants toward reactive oxygen and nitrogen species (IUPAC Technical Report), Pure Appl. Chem., 2022, 94(1), 87–144 CrossRef CAS.
- B. Ahmad, A. A. Khan, M. U. Rehman, M. Siraj, A. Ali, A. Sarwar, J. Naseeb and A. F. Alasmari, Evaluation of analgesic, antioxidant, and anti-inflammatory potential of Dianthus crinitus using mice as a research animal, Ital. J. Food Sci., 2024, 36(2), 38–47 Search PubMed.
- B. Akbari, N. Baghaei-Yazdi, M. Bahmaie and F. Mahdavi Abhari, The role of plant-derived natural
antioxidants in reduction of oxidative stress, BioFactors, 2022, 48(3), 611–633 CrossRef CAS PubMed.
- A. Keshavarzi, A. Ranjbar, N. Kheiripour, A. Ghaleiha, A. Soltaniyan and S. M. Hashemi, Comparing the Effect of Grape Fermentative Product and Fresh Red Grape Juice on Antioxidant Biomarkers of Liver Mitochondria Isolated From Rats in Vitro, Res. Mol. Med., 2021, 9(1), 21–28 CrossRef CAS.
- M. Choleva, V. Boulougouri, A. Panara, E. Panagopoulou, A. Chiou, N. S. Thomaidis, S. Antonopoulou and E. Fragopoulou, Evaluation of anti-platelet activity of grape pomace extracts, Food Funct., 2019, 10(12), 8069–8080 RSC.
- L. Jian, C. J. Du, A. H. Lee and C. W. Binns, Do dietary lycopene and other carotenoids protect against prostate cancer?, Int. J. Cancer, 2005, 113(6), 1010–1014 CrossRef PubMed.
- P. Gong, S. Wang, M. Liu, F. Chen, W. Yang, X. Chang, N. Liu, Y. Zhao, J. Wang and X. Chen, Extraction methods, chemical characterizations and biological activities of mushroom polysaccharides: A mini-review, Carbohydr. Res., 2020, 494, 108037 CrossRef CAS PubMed.
- L. Rezig, M. Chouaibi, W. Meddeb, K. Msaada and S. Hamdi, Chemical composition and bioactive compounds of Cucurbitaceae seeds: Potential sources for new trends of plant oils, Process Saf. Environ. Prot., 2019, 127, 73–81 CrossRef CAS.
- F. Garavand, S. Rahaee, N. Vahedikia and S. M. Jafari, Different techniques for extraction and micro/nanoencapsulation of saffron bioactive ingredients, Trends Food Sci. Technol., 2019, 89, 26–44 CrossRef CAS.
- M. Zwingelstein, M. Draye, J. L. Besombes, C. Piot and G. Chatel, Viticultural wood waste as a source of polyphenols of interest: Opportunities and perspectives through conventional and emerging extraction methods, Waste Manage., 2020, 102, 782–794 CrossRef CAS PubMed.
- Y. Ji, Y. Hou, S. Ren, C. Yao and W. Wu, Highly efficient extraction of phenolic compounds from oil mixtures by trimethylamine-based dicationic ionic liquids via forming deep eutectic solvents, Fuel Process. Technol., 2018, 171, 183–191 CrossRef CAS.
- Azwanida, A review on the extraction methods use in medicinal plants, principle, strength, and limitation, Med. Aromat. Plants, 2015, 4, 196 Search PubMed.
- M. Salihović, M. Pazalja and A. Ajanović, Antioxidant activity of watermelon seeds determined by DPPH assay, KUI, 2022, 71(5–6), 295–300 Search PubMed.
- G. Kasiramar, Significant role of soxhlet extraction process in phytochemical research, Mintage J. Pharm. & Med. Sci., 2019, 7, 43–47 Search PubMed.
- T. W. Caldas, K. E. Mazza, A. S. Teles, G. N. Mattos, A. I. Brígida, C. A. Conte-Junior, R. G. Borguini, R. L. Godoy, L. M. Cabral and R. V. Tonon, Phenolic compounds recovery from grape skin using conventional and non-conventional extraction methods, Ind. Crops Prod., 2018, 111, 86–91 CrossRef CAS.
- O. R. Alara, N. H. Abdurahman and C. I. Ukaegbu, Soxhlet extraction of phenolic compounds from Vernonia cinerea leaves and its antioxidant activity, J. Appl. Res. Med. Aromat. Plants, 2018, 11, 12–17 Search PubMed.
- A. Molino, S. Mehariya, G. Di Sanzo, V. Larocca, M. Martino, G. P. Leone, T. Marino, S. Chianese, R. Balducchi and D. Musmarra, Recent developments in supercritical fluid extraction of bioactive compounds from microalgae: Role of key parameters, technological achievements and challenges, J. CO2 Util., 2020, 36, 196–209 CrossRef CAS.
- M. Maza, I. Álvarez and J. Raso, Thermal and non-thermal physical methods for improving polyphenol extraction in red winemaking, Beverages, 2019, 5(3), 47 CrossRef CAS.
- F. Chemat, M. A. Vian, A. S. Fabiano-Tixier, M. Nutrizio, A. R. Jambrak, P. E. Munekata, J. M. Lorenzo, F. J. Barba, A. Binello and G. Cravotto, A review of sustainable and intensified techniques for extraction of food and natural products, Green Chem., 2020, 22(8), 2325–2353 RSC.
-
W. Arthur, P. T. Akonor, T. Najah and C. Oduro-Yeboah, Report on training of processors and agric extension officers on handling postharvest losses of watermelons, mangoes and pineapples held on 11th and 12th May 2021, at MOFA, Shai Osudoku District Assembly, Accra, Ghana, 2021, p. 11063 Search PubMed.
- A. Martínez-Sánchez and E. Aguayo, Effects of ozonated water irrigation on the quality of grafted watermelon seedlings, Sci. Hortic., 2020, 261, 109047 CrossRef.
- R. K. Saini and Y. S. Keum, Carotenoid extraction methods: A review of recent developments, Food Chem., 2018, 240, 90–103 CrossRef CAS PubMed.
- M. Alonzo-Macías, A. Cardador-Martínez, S. Mounir, G. Montejano-Gaitán and K. Allaf, Comparative study of the effects of drying methods on antioxidant activity of dried strawberry (Fragaria Var. Camarosa), J. Food Res., 2013, 2(2), 92 CrossRef.
- M. M. Cavalluzzi, A. Lamonaca, N. P. Rotondo, D. V. Miniero, M. Muraglia, P. Gabriele, F. Corbo, A. De Palma, R. Budriesi, E. De Angelis and L. Monaci, Microwave-assisted extraction of bioactive compounds from lentil wastes: Antioxidant activity evaluation and Metabolomic characterization, Molecules, 2022, 27(21), 7471 CrossRef CAS PubMed.
- D. Niu, E. F. Ren, J. Li, X. A. Zeng and S. L. Li, Effects of pulsed electric field-assisted treatment on the extraction, antioxidant activity and structure of naringin, Sep. Purif. Technol., 2021, 265, 118480 CrossRef CAS.
- V. Athanasiadis, T. Chatzimitakos, D. Kalompatsios, K. Kotsou, M. Mantiniotou, E. Bozinou and S. I. Lalas, Recent Advances in the Antibacterial Activities of Citrullus lanatus (Watermelon) By-Products, Appl. Sci., 2023, 13(19), 11063 CrossRef CAS.
- A. M. Awad, P. Kumar, M. R. Ismail-Fitry, S. Jusoh, M. F. Ab Aziz and A. Q. Sazili, Green extraction of bioactive compounds from plant biomass and their application in meat as natural antioxidant, Antioxidants, 2021, 10(9), 1465 CrossRef CAS PubMed.
- G. J. Fadimu, K. Ghafoor, E. E. Babiker, F. Al-Juhaimi, R. A. Abdulraheem and M. K. Adenekan, Ultrasound-assisted process for optimal recovery of phenolic compounds from watermelon (Citrullus lanatus) seed and peel, J. Food Meas. Charact., 2020, 14, 1784–1793 CrossRef.
- S. Zamuz, P. E. Munekata, B. Gullón, G. Rocchetti, D. Montesano and J. M. Lorenzo,
Citrullus lanatus as source of bioactive components: An up-to-date review, Trends Food Sci. Technol., 2021, 111, 208–222 CrossRef CAS.
- D. Panda and S. Manickam, Cavitation technology—The future of greener extraction method: A review on the extraction of natural products and process intensification mechanism and perspectives, Appl. Sci., 2019, 9(4), 766 CrossRef CAS.
- B. R. Sumere, M. C. de Souza, M. P. Dos Santos, R. M. Bezerra, D. T. da Cunha, J. Martinez and M. A. Rostagno, Combining pressurized liquids with ultrasound to improve the extraction of phenolic compounds from pomegranate peel (Punica granatum L.), Ultrason. Sonochem., 2018, 48, 151–162 CrossRef CAS PubMed.
- B. R. Sumere, M. C. de Souza, M. P. Dos Santos, R. M. Bezerra, D. T. da Cunha, J. Martinez and M. A. Rostagno, Combining pressurized liquids with ultrasound to improve the extraction of phenolic compounds from pomegranate peel (Punica granatum L.), Ultrason. Sonochem., 2018, 48, 151–162 CrossRef CAS PubMed.
- D. E. Rotimi and R. M. Asaleye, Impact of Watermelon (Citrullus lanatus) on Male Fertility, JBRA Assist. Reprod., 2023, 27(4), 702 Search PubMed.
- Y. F. Mustafa, R. N. Ismael and R. M. Jebir, Natural coumarins from two cultivars of watermelon seeds as biosafe anticancer agents, an algorithm for their isolation and evaluation, J. Mol. Struct., 2024, 1295, 136644 CrossRef CAS.
- F. Yesilyurt, H. Özbek, M. E. Hacımüftüoğlu, A. N. Coşkun, A. Dumlu and O. Coşkun, Anticancer Potential of Watermelon Seed Extracts Against Lung and Breast Cancer Cell Lines, Trends Pharmacol. Sci, 2024, 2(1), 21–26 Search PubMed.
- I. Galit, A. C. Grosu, N. Băbeanu and O. Popa, Efficient utilization of watermelon wastes, Scientific Bulletin Series F. Biotechnologies., 2019, 23, 100–106 Search PubMed.
- A. I. Peter-Ikechukwu, C. G. Ogazi, A. E. Uzoukwu, N. O. Kabuo and M. N. Chukwu, Proximate and functional properties of composite flour produced with date fruit pulp, toasted watermelon seed and wheat, J. Food Chem. Nanotechnol., 2020, 6(3), 159–166 Search PubMed.
- M. Wójcik, A. Bieńczak, P. Woźniak and R. Różyło, Impact of Watermelon Seed Flour on the Physical, Chemical, and Sensory Properties of Low-Carbohydrate, High-Protein Bread, Processes, 2023, 11(12), 3282 CrossRef.
- K. T. Seidu and O. L. Otutu, Phytochemical composition and radical scavenging activities of watermelon (Citrullus lanatus) seed constituents, Croat. J. Food Sci. Technol., 2016, 8(2), 83–89 CrossRef.
- E. Aguayo, A. Martínez-Sánchez, B. Fernández-Lobato and F. Alacid, L-Citrulline: a non-essential amino acid with important roles in human health, Appl. Sci., 2021, 11(7), 3293 CrossRef CAS.
- H. Dawoud and T. Malinski, Vitamin D3, L-Arginine, L-Citrulline, and antioxidant supplementation enhances nitric oxide bioavailability and reduces oxidative stress in the vascular endothelium–Clinical implications for cardiovascular system, Pharmacogn. Res., 2020, 12(1), 112347 Search PubMed.
- Z. Su, Y. Han and J. Du, Effects of acidified apple juice before fermentation on ethyl carbamate and volatile components of apple distillate, Ital. J. Food Sci., 2023, 35(2), 1–12 CAS.
- R. Ilahy, I. Tlili, M. W. Siddiqui, C. Hdider and M. S. Lenucci, Inside and beyond color: Comparative overview of functional quality of tomato and watermelon fruits, Front. Plant Sci., 2019, 10, 769 CrossRef PubMed.
- A. Manivannan, E. S. Lee, K. Han, H. E. Lee and D. S. Kim, Versatile nutraceutical potentials of watermelon—A modest fruit loaded with pharmaceutically valuable phytochemicals, Molecules, 2020, 25(22), 5258 CrossRef CAS PubMed.
- B. T. Priya, V. Ajithkumar, S. M. Kathija Beevi, J. H. Husain and K. T. Poovarasan, Antioxidant assays of watermelon (Citrullus lanatus) seeds, Biochem. Cell. Arch., 2023, 23(1), 111546 Search PubMed.
- A. O. Adebayo, I. Alozie and C. O. somtochi Olivia, Evaluating the influence of Citrullus lanatus seed extracts on electrolytes, urea and creatinine in Streptozotocin induced diabetic albino rats, Am. J. Life Sci., 2018,(1), 87–94 Search PubMed.
- S. Messaoudi, S. Tebibel, A. K. Beladjila, F. K. Touhami and Z. Kabouche, Anti-hyperlipidemic, Anti-inflammatory and Antioxidant Activities of Citrullus lanatus, World J. Environ. Biosci., 2019, 8(1–2019), 100–106 Search PubMed.
- S. Wahid, R. A. Khan, Z. Feroz and R. Ikram, Analgesic, anti-inflammatory and toxic effects of ethanol extracts of Cucumis melo and Citrullus lanatus seeds, Pak. J. Pharm. Sci., 2020, 33(3), 4199284 Search PubMed.
- V. Athanasiadis, T. Chatzimitakos, K. Kotsou, D. Kalompatsios, E. Bozinou and S. I. Lalas, Polyphenol extraction from food (by) products by pulsed electric field: A review, Int. J. Mol. Sci., 2023, 24(21), 15914 CrossRef CAS PubMed.
- G. E. Irabor, J. E. Ebhoaye and A. Odia, Qualitative and quantitative screening of some phytochemical compounds in watermelon (Citrullus lanatus) seeds cultivated in esan west local, Int J Eng Sci Technol., 2020, 5, 268–273 Search PubMed.
- S. Alseekh, L. P. de Souza, M. Benina and A. R. Fernie, The style and substance of plant flavonoid decoration; towards defining both structure and function, Phytochemistry, 2020, 174, 112347 CrossRef CAS PubMed.
- A. Hillenbrand, B. Kiebler, C. Schwab, L. Scheja, P. Xu, D. Henne-Bruns, A. M. Wolf and U. Knippschild, Prevalence of non-alcoholic fatty liver disease in four different weight related patient groups: association with small bowel length and risk factors, BMC Res. Notes, 2015, 8, 1–9 CrossRef CAS PubMed.
- V. Divyadharsini, T. U. Maheswari and S. Rajeshkumar, Assessment of Antimicrobial Activity of Lycopene, Vitamin E, and Lycopene-Vitamin E Combination Against Staphylococcus aureus, Streptococcus mutans, Enterococcus faecalis, and Candida albicans: An In Vitro Study, Cureus, 2023, 15(7), 702 Search PubMed.
- Ł. Łopusiewicz, E. Drozłowska, P. Trocer, M. Kostek, M. Śliwiński, M. H. Henriques, A. Bartkowiak and P. Sobolewski, Whey protein concentrate/isolate biofunctional films modified with melanin from watermelon (Citrullus lanatus) seeds, Materials, 2020, 13(17), 3876 CrossRef PubMed.
- A. Petchsomrit, M. I. McDermott, S. Chanroj and W. Choksawangkarn, Watermelon seeds and peels: fatty acid composition and cosmeceutical potential, OCL, 2020, 27, 54 CrossRef CAS.
- Z. M. Younossi, A. B. Koenig, D. Abdelatif, Y. Fazel, L. Henry and M. Wymer, Global epidemiology of nonalcoholic fatty liver disease—meta-analytic assessment of prevalence, incidence, and outcomes, Hepatology, 2016, 64(1), 73–84 CrossRef PubMed.
- X. Song, Y. Luo, L. Ma, X. Hu, J. Simal-Gandara, L. S. Wang, V. K. Bajpai, J. Xiao and F. Chen, Recent trends and advances in the epidemiology, synergism, and delivery system of lycopene as an anti-cancer agent, Semin. Cancer Biol, 2021, 73, 331–346 CrossRef CAS PubMed.
- W. J. Qi, W. S. Sheng, C. Peng, M. Xiaodong and T. Z. Yao, Investigating into anti-cancer potential of lycopene: Molecular targets, Biomed. Pharmacother., 2021, 138, 111546 CrossRef CAS PubMed.
- B. P. Puah, J. Jalil, A. Attiq and Y. Kamisah, New insights into molecular mechanism behind anti-cancer activities of lycopene, Molecules, 2021, 26(13), 3888 CrossRef CAS PubMed.
- G. J. Fadimu, K. Ghafoor, E. E. Babiker, F. Al-Juhaimi, R. A. Abdulraheem and M. K. Adenekan, Ultrasound-assisted process for optimal recovery of phenolic compounds from watermelon (Citrullus lanatus) seed and peel, J. Food Meas. Charact, 2020, 14, 1784–1793 CrossRef.
- Y. Andargie, W. Sisay, M. Molla, A. Norahun and P. Singh, Evaluation of the antiulcer activity of methanolic extract and solvent fractions of the leaves of Calpurnia aurea, (Ait.) Benth.(Fabaceae) in rats. Evidence-Based Complementary and Alternative Medicine, 2022, 2022(1), 4199284 Search PubMed.
- A. Hussain, H. Pu and D. W. Sun, Measurements of lycopene contents in fruit: A review of recent developments in conventional and novel techniques, Crit. Rev. Food Sci. Nutr., 2019, 59(5), 758–769 CrossRef CAS PubMed.
- T. Lum, M. Connolly, A. Marx, J. Beidler, S. Hooshmand, M. Kern, C. Liu and M. Y. Hong, Effects of fresh watermelon consumption on the acute satiety response and cardiometabolic risk factors in overweight and obese adults, Nutrients, 2019, 11(3), 595 CrossRef CAS PubMed.
- C. O. Karikpo, E. S. Bartimaeus and B. Holy, Evaluation of the cardioprotective effect of Citrullus lanatus (watermelon) seeds in streptozotocin induced diabetic albino rats, Asian J. Biochem. Genet. Mol. Biol, 2019, 1(4), 1–6 Search PubMed.
- M. R. Shahein, E. S. Atwaa, K. M. El-Zahar, A. A. Elmaadawy, H. H. Hijazy, M. Z. Sitohy, A. Albrakati and E. K. Elmahallawy, Remedial action of yoghurt enriched with watermelon seed milk on renal injured hyperuricemic rats, Fermentation, 2022, 8(2), 41 CrossRef CAS.
- Y. M. Rayyan and R. F. Tayyem, Non-alcoholic fatty liver disease and associated dietary and lifestyle risk factors, Diabetes Metab. Syndr., 2018, 12(4), 569–575 CrossRef PubMed.
- A. Hekmatdoost, A. Shamsipour, M. Meibodi, N. Gheibizadeh, T. Eslamparast and H. Poustchi, Adherence to the dietary approaches to stop hypertension (DASH) and risk of nonalcoholic fatty liver disease, Int. J. Food Sci. Nutr., 2016, 67(8), 1024–1029 CrossRef CAS PubMed.
- Z. Yari, M. Rahimlou, T. Eslamparast, N. Ebrahimi-Daryani, H. Poustchi and A. Hekmatdoost, Flaxseed supplementation in non-alcoholic fatty liver disease: a pilot randomized, open labeled, controlled study, Int. J. Food Sci. Nutr., 2016, 67(4), 461–469 CrossRef CAS PubMed.
- Z. Mokhtari, D. L. Gibson and A. Hekmatdoost, Nonalcoholic fatty liver disease, the gut microbiome, and diet, Adv. Nutr., 2017, 8(2), 240–252 CrossRef CAS PubMed.
- M. Amiri and H. Nasri, Secondary Hyperparathyroidism in chronic kidney disease patients; current knowledge, J Parathyr Dis., 2014, 2(1), 1–2 Search PubMed.
- K. Kachhawa, M. Varma, P. Kachhawa, D. Agrawal, M. K. Shaikh and S. Kumar, Study of dyslipidemia and antioxidant status in chronic kidney disease patients at a hospital in South East Asia, J Health Res. Rev. Dev. Ctries., 2016, 3(1), 28–30 Search PubMed.
- A. M. Rimando and P. M. Perkins-Veazie, Determination of citrulline in watermelon rind, J. Chromatogr. A, 2005, 1078(1–2), 196–200 CrossRef CAS PubMed.
- L. Yu, W. Wang, W. Pang, Z. Xiao, Y. Jiang and Y. Hong, Dietary lycopene supplementation improves cognitive performances in tau transgenic mice expressing P301L mutation via inhibiting oxidative stress and tau hyperphosphorylation, J. Alzheimer's Dis., 2017, 57(2), 475–482 CAS.
- A. Popolo, S. Adesso, A. Pinto, G. Autore and S. Marzocco, L-Arginine and its metabolites in kidney and cardiovascular disease, Amino Acids, 2014, 46, 2271–2286 CrossRef CAS PubMed.
- J. J. DuPont, W. B. Farquhar, R. R. Townsend and D. G. Edwards, Ascorbic acid or L-arginine improves cutaneous microvascular function in chronic kidney disease, J. Appl. Physiol., 2011, 111(6), 1561–1567 CrossRef CAS PubMed.
- M. Nadeem, M. Navida, K. Ameer, A. Iqbal, F. Malik, M. A. Nadeem, H. Fatima, A. Ahmed and A. Din, A comprehensive review on the watermelon phytochemical profile and their bioactive and therapeutic effects, Food Preserv. Sci., 2022, 29(4), 546–576 Search PubMed.
- T. M. Alnour, E. H. Ahmed-Abakur, E. H. Elssaig, F. M. Abuduhier and M. F. Ullah, Antimicrobial synergistic effects of dietary flavonoids rutin and quercetin in combination with antibiotics gentamicin and ceftriaxone against E. coli (MDR) and P. mirabilis (XDR) strains isolated from human infections: Implications for food–medicine interactions, Ital. J. Food Sci., 2022, 34(2), 34–42 CAS.
- R. Paul, M. K. Mazumder, J. Nath, S. Deb, S. Paul, P. Bhattacharya and A. Borah, Lycopene-a pleiotropic neuroprotective nutraceutical: deciphering its therapeutic potentials in broad spectrum neurological disorders, Neurochem. Int., 2020, 140, 104823 CrossRef CAS PubMed.
- M. S. Farid, R. Anjum, Y. Yang, M. Tu, T. Zhang, D. Pan, Y. Sun and Z. Wu, Recent Trends in Fermented Plant-Based Analogues and Products, Bioactive Peptides, and Novel Technologies-Assisted Fermentation, Trends Food Sci. Technol., 2024, 10, 104529 CrossRef.
- A. I. Peter-Ikechukwu, G. C. Omeire, N. O. Kabuo, C. N. Eluchie, C. Amandikwa and G. I. Odoemenam, Production and evaluation of biscuits made from wheat flour and toasted watermelon seed meal as fat substitute, J. Food Res., 2024, 7(5), 112–112 CrossRef.
- O. T. Bolaji, S. A. Adeyeye and D. Ogunmuyiwa, Quality evaluation of bread produced from whole wheat flour blended
with watermelon seed flour, J. Culin. Sci. Technol, 2024, 22(4), 607–630 CrossRef.
- A. Qayyum, N. Huma, A. Sameen, A. Siddiq and M. Munir, Impact of watermelon seed flour on the physico-chemical and sensory characteristics of ice cream, J. Food Process. Preserv., 2017, 41(6), e13297 CrossRef.
- A. Prema, U. Janakiraman, T. Manivasagam and A. J. Thenmozhi, Neuroprotective effect of lycopene against MPTP induced experimental Parkinson's disease in mice, Neurosci. Lett., 2015, 599, 12–19 CrossRef CAS PubMed.
- S. I. Abdelwahab, L. E. Hassan, H. M. Sirat, S. M. Yagi, W. S. Koko, S. Mohan, M. M. Taha, S. Ahmad, C. S. Chuen, P. Narrima and M. M. Rais, Anti-inflammatory activities of cucurbitacin E isolated from Citrullus lanatus var. citroides: role of reactive nitrogen species and cyclooxygenase enzyme inhibition, Fitoterapia, 2011, 82(8), 1190–1197 CrossRef CAS PubMed.
- B. K. Tiwari, C. P. O'Donnell, K. Muthukumarappan and P. J. Cullen, Effect of sonication on orange juice quality parameters during storage, Int. J. Food Sci. Technol., 2009, 44(3), 586–595 CrossRef CAS.
- D. A. Anang, R. A. Pobee, E. Antwi, E. M. Obeng, A. Atter, F. K. Ayittey and J. T. Boateng, Nutritional, microbial and sensory attributes of bread fortified with defatted watermelon seed flour, Int. J. Food Sci. Technol., 2018, 53(6), 1468–1475 CrossRef CAS.
- S. A. Adeyeye, T. B. Olushola, T. A. Abegunde, A. O. Adebayo-Oyetoro, H. K. Tiamiyu and F. Idowu-Adebayo, Evaluation of nutritional composition, physico-chemical and sensory properties of ‘Robo’(A Nigerian traditional snack) produced from watermelon (Citrullus lanatus) (Thunb.) seeds, Food Res., 2020, 4(1), 216–223 Search PubMed.
- U. B. Ejinkeonye, O. C. Nduka and B. I. OffiaOlua, Effect of fermentation duration on the nutritional and antinutritional content of watermelon seeds and sensory properties of their ogiri products, Eur. J. Food Sci. Technol., 2018, 6(2), 1–6 Search PubMed.
- D. Khalaf, M. Krüger, M. Wehland, M. Infanger and D. Grimm, The effects of oral l-arginine and l-citrulline supplementation on blood pressure, Nutrients, 2019, 11(7), 1679 CrossRef CAS PubMed.
- O. C. Nzeagwu and C. O. Raphael, Chemical, functional and sensory properties of watermelon (citrullus lanatus) seeds' flour blends as soup thickeners, JDAN, 2018, 9, 36–46 Search PubMed.
- S. Zia, M. R. Khan, R. M. Aadil and M. Shahid, Development and storage stability of value-added watermelon fruit butter by incorporating watermelon rind byproduct, J. Food Process. Preserv., 2022, 46(11), e17031 CAS.
- D. A. Anang, R. A. Pobee, E. Antwi, E. M. Obeng, A. Atter, F. K. Ayittey and J. T. Boateng, Nutritional, microbial and sensory attributes of bread fortified with defatted watermelon seed flour, Int. J. Food Sci. Technol., 2018, 53(6), 1468–1475 CrossRef CAS.
- M. S. Sanusi, M. O. Sunmonu and S. O. Alasi, Influence of extrusion conditions on functional and textural properties of brown rice-watermelon seeds extruded snacks, Acta Period. Technol., 2023,(54), 81–91 CrossRef CAS.
|
This journal is © The Royal Society of Chemistry 2025 |
Click here to see how this site uses Cookies. View our privacy policy here.