Valorization of cocoa (Theobroma cacao L.) pod husks as a fruit pulp substitute in mango jam formulations: effects on jam qualities during storage and sensory discrimination†
Received
11th November 2024
, Accepted 23rd December 2024
First published on 27th December 2024
Abstract
In this study, fruit pulp obtained from cocoa pod husks (CPHs) was utilized as a substitute in mango jam formulations at two different ratios of 25% and 50% with and without the addition of commercial pectin, in comparison with normal jam made from 100% mango pulp as the control. The results showed that the addition of CPH pulp significantly increased the phenolic content by 3.0–3.9 times and DPPH antioxidant activity by 1.5 times compared to the control sample. Moreover, the retention of phenolics in CPH-pulp-substituted jam was significantly enhanced during 4-week storage while DPPH activity declined remarkably by 60.3–72.7% after four weeks. In terms of appearance, all CPH–mango jams exhibited a characteristic yellow color similar to the control, although darker. CPH pulp reduced hardness and increased adhesiveness and springiness of mango jam without commercial pectin and CPH–mango jam showed slight differences in consumer sensory discrimination.
Sustainability spotlight
Fruit waste valorization is a critical component in achieving the UN SDGs, particularly those related to sustainable consumption and production, climate action, and economic growth. The global food waste problem, with fruits and vegetables contributing significantly due to their perishable nature, presents both a challenge and an opportunity for sustainable development. Valorization strategies have been explored to transform these wastes into stable, value-added products, thereby reducing food wastage and aligning with the SDG target of halving food waste by 2030. In this study, the circular economy framework offers a promising approach for the valorization of cocoa pod husks, where waste is converted into pectin-rich pulp, showing potential as a substitute for commercial pectin and fruit pulp in the formulation of mango jam.
|
Introduction
Mango (Mangifera indica L.), a member of the Anacardiaceae family, is cultivated in over ninety tropical and subtropical nations, where hundreds of varieties exist.1,2 Mango, which contains an abundance of bioactive chemicals, carbohydrates, lipids and fatty acids, proteins, organic acids, vitamins, and minerals, is the most widely consumed tropical fruit. The biological features of phenolic compounds (phenolic alcohols, phenolic acids, and flavonoids) and carotenoids (α- and β-carotene) are characteristic of mango flesh.1,3 The Food and Agriculture Organization estimates that the annual global production of mangoes was approximately 52 million tons in 2018, with a consistent upward trend in output. India is the leading mango producer globally, with an impressive annual output of 15 million tons.4,5 Green mangoes can be processed into powder, pickles, preserves, desserts, or chili sauce. On the other hand, ripe mangoes can be processed into concentrated mango products, dried mangoes, mango wine, mango juice, jams, jellies, syrups, and canned mangoes.6 However, fresh mango has a maximum shelf life of 30 days when stored at 4–5 °C, and it can be much shorter under less than ideal conditions. Decomposition and oxidation of vitamins and polyphenol components are two ways in which this impacts the nutritional content of raw materials. Efforts have been made in the past to diversify mango products, including mango jam, canned mango juice, dried mangoes,7 and mango leather sheets,8 in order to cater to a wider range of consumers' demands, increase the value of mangoes and boost regional and national economies.9 Among these products, jam, classified as a medium-moisture food item, is produced through the process of boiling fruit pulp, pectin, acids, sugar (sucrose), and other additives until a specific consistency is achieved.10,11 Jam is an excellent source of vital amino acids, vitamins, minerals, sugar, and fiber with negligible fat or cholesterol, leading to a low possibility of developing cardiovascular diseases upon jam consumption.12 As per the guidelines set forth by the Bureau of Indian Standards and the Prevention of Food Adulteration, jam must have a minimum fruit content of 45% and a total soluble solid (TSS) content of 68.5% or higher.13 Similarly, as mandated by the Codex Alimentarius Commission, it is necessary for finished jam to comprise a minimum TSS of 65%. For consistent gel strength in industrial jam production, commercial pectin (0–10 g per kg of jam) may be necessary.12 Pectins, which are natural polymers commonly extracted from a variety of plant sources such as passion fruit, grapes, mangoes, citrus fruits, pumpkins, apples, and carrots,14,15 have extensive utility in diverse applications such as fat or sugar substitutes, thickeners, stabilizers, and emulsifiers.16 Pectin is marketed in the form of dry powder and is used as a stabilizer in several culinary products, including fruit drinks, fruit powders, and tomatoes. Additionally, pectin can be utilized in the formulation of marmalades, jams, and jellies due to its gelling properties.17
Cocoa (Theobroma cacao L.) holds significant economic value in developing nations, with global cocoa bean production reaching approximately 5
252
377 tons in 2018 and a harvested area of 11
834
970 hectares.18,19 In parallel, it was estimated that 16 million tons of waste were produced during the collection of cocoa seeds, accounting for 80% of the fruit mass.18 The cocoa pod husk (CPH) is the rough, oval and relatively thick outer part of the cocoa fruit (exocarp), consisting of cellulose (35.0%), lignin (14.6%), hemicellulose (11.0%), pectin (6.1%) and protein (5.9%), in addition to minerals and water.20,21 CPH is obtained after removing the seeds and accounts for 70–80% of the dry weight of the fruit.22 Furthermore, the fiber content in CPH ranges from 61.05% to 83.39%, with dietary fiber accounting for 46.98% to 51.11%.23 This high fiber content highlights its potential as a functional ingredient in food products and enhances its application in sustainable packaging solutions.24,25 In addition to its fiber content, CPH extract has been identified as a rich source of bioactive phenolic compounds. Previous studies have identified the phenolic components in CPH, such as quercetin, epicatechin, gallic acid, coumaric acid, and protocatechuic acid.26,27 These studies showed that the phenolic compounds in cocoa pod shells have significant potential in reducing the risk of developing chronic diseases, including cardiovascular disorders, cancer, and other chronic inflammatory diseases, confirming this raw material's nutritional and medicinal value.
On account of its high pectin content and characteristic color similar to mango, CPH pulp emerges as a highly viable substitute for mango pulp throughout the jam manufacturing procedure, resulting in a cost reduction without compromising product quality or consumer reception. In this way, the issue of managing agricultural residues is also alleviated, contributing to sustainable development. Currently, there is a scarcity of data pertaining to the potential impact of substituting CPH pulp for mango pulp on jam quality during storage. Therefore, this study was conducted to evaluate the feasibility of the substitution of mango pulp with CPH pulp with the focus on textural attributes and sensory differences compared with traditional mango jam.
Experimental
Materials and chemicals
Fresh Trinitario cocoa (Theobroma cacao L. cv. Trinitario) fruits were collected from Binh Phuoc (Vietnam). After harvesting, the samples were stored under refrigeration at 4 °C for further use. Mango (Mangifera indica L. cv. ‘Cat Hoa Loc’) fruits from Tien Giang (Vietnam) were chosen for their maturity, uniform size, and lack of bruises and damage. The mangoes were stored at room temperature and used the same day. Other chemicals used in this study were of analytical grade.
Preparation of CPH pulp-substituted mango jam
After washing, cocoa pod husks were cut into 2-cm thick pieces and steamed for 10 min. Then, CPH was ground with a CPH
:
water ratio of 1
:
1.5 (g mL−1), followed by filtration through a 100-mesh sieve to obtain CPH pulp which was stored at −4 °C until further use. For mango jam production, the mango fruits were washed, peeled and cut into 2-cm thick slices. After steam blanching for 5 min, mango slices were pureed, sieved through a 100-mesh sieve and promptly analyzed for dry matter content (%). The mango jam formulation used in this study includes mango pulp 100 g, sucrose 120 g, water 100 g, citric acid 3 g, and commercial pectin 1.5 g. The mixture was concentrated at 100 °C under continuous stirring until the soluble solid content reached 65 °Brix, poured into a sterilized glass jar, cooled and stored at room temperature.
To investigate the substitution of CPH pulp for mango pulp and commercial pectin, mango pulp was replaced with 25% and 50% CPH pulp in the mango jam formulation, both with and without commercial pectin. Because CPH has no distinctive flavor, it is only partially substituted in the mango jam recipe. The investigated samples are presented in Table 1.
Table 1 Formulation of CPH pulp-substituted mango jama
|
Mango pulp (g) |
Sucrose (g) |
Water (g) |
Citric acid (g) |
Pectin (g) |
CPH pulp (g) |
Mx_y, where x is the substitution ratio (%) of CPH pulp and y is the amount of commercial pectin in the formulation.
|
M0_1.5 (control) |
100 |
120 |
100 |
3 |
1.5 |
— |
M25_1.5 |
75 |
120 |
100 |
3 |
1.5 |
26.5 |
M25_0 |
75 |
120 |
100 |
3 |
0 |
28 |
M50_1.5 |
50 |
120 |
100 |
3 |
1.5 |
51.5 |
M50_0 |
50 |
120 |
100 |
3 |
0 |
53 |
Analytical methods
Proximate analysis.
Moisture, crude protein, fat, and ash content were determined using official methods, namely, oven drying to constant mass, the micro-Kjeldahl method, Soxhlet extraction and dry ashing.28 Total carbohydrate content was calculated by difference. Titratable acidity (TA) was measured by NaOH titration using phenolphthalein as an indicator and expressed as g citric acid per 100 g sample on a dry weight basis (g/100 g DW).
Pectin content.
The determination of pectin content was conducted based on the procedure described in the literature with some modifications.29 Briefly, 10 g of CPH pulp (M) was extracted with 10 mL of 20% citric acid solution. The mixture was then heated at 80 °C for 2 h before being centrifuged at 5000 rpm for 10 min to collect the supernatant. Next, the pH was adjusted to 4.5 by using 0.1 N HCl and 40 mL of pure ethanol was added. The mixture was refrigerated and left overnight for pectin precipitation. Finally, the precipitate was recovered by centrifugation and then dried to a constant mass (m1). The pectin content was calculated using the following formula:
pH, total soluble solids, and color attributes.
pH and total soluble solids (°Brix) were measured using a HI 2211-02 pH meter (Hanna Instruments, Romania) and a Master-53M hand-held refractometer (Atago Ltd, Japan), respectively. Color attributes in the CIELAB color space, including lightness (L*), redness (a*), yellowness (b*), chroma (C*) and hue (h°), were measured using an NR110 precision colorimeter (3NH Technology Co. Ltd, China) at five random points on the surface.
Total phenolic content and DPPH free radical scavenging assay.
To prepare sample aliquots for the determination of total phenolic content and DPPH activity, the jam was 10-fold diluted with distilled water, vortexed for 30 s at 2000 rpm using a ZX4 advanced IR vortex mixer (VELP Scientifica, Italy) and sonicated for 10 min at 240 W in a Pro 100 ultrasonic cleaner (Asonic, Slovenia). The supernatant was collected by centrifugation in a PLC-05 centrifuge (Gemmy Industrial Corp., Taiwan). The total phenolic content (mg gallic acid equivalent per 100 g, mg GAE/100 g) and antioxidant activity (mg Trolox equivalent per 100 g, mg TE/100 g) were estimated using the Folin–Ciocalteu method according to ISO 14502-1:2005,30 and DPPH free radical scavenging assay.31
Reducing sugar content.
For the determination of reducing sugar content, the above clear solution (5 mL) was neutralized using 0.1 M NaOH in the presence of phenolphthalein, followed by protein precipitation with 2 mL of 20% (w/v) lead acetate before filtration through Whatman No. 2 filter paper. The total reducing sugar content (g glucose per 100 g) was measured according to the spectrophotometric method using the 2,3-dinitrosalicylic acid (DNS) reagent.32
Texture profile analysis (TPA).
Texture measurements were performed after 1-week storage using a CT3 texture analyzer (AMETEK Brookfield Inc., Middleboro, USA) with a cylindrical measuring head (TA4/1000, diameter of 38.1 mm) with fixed settings as follows: distance between the probe and sample of 30 mm, a trigger load of 10 g, a probe speed of 1 mm s−1 and a returning speed of 1 mm s−1. Textural attributes (hardness, adhesiveness, and springiness) were recorded using Texture Proc CT V1.3 Build 15 software.
Microbiological qualities of CPH–mango jams during storage.
Microbiological analysis was performed after 4-week storage according to ISO 4833-1:2013 for total aerobic bacteria count (incubation for 72 h at 30 °C), ISO 4832:2006 for coliform count (incubation for 24 h at 37 °C), ISO 16649-2:2001 for E. coli (incubation for 24 h at 44 °C), ISO 21527-2:2008 for total yeasts and molds (incubation for 5 days at 25 °C). The microbial counts were expressed as the logarithmic number of colony forming units per gram of sample (log CFU g−1) and calculated using the following formula:
Microbial count (CFU g−1) = ΣC/[V × (n1 + 0.1 × n2) × df] |
where, ΣC is the sum of all colonies counted on the two dishes retained from two successive dilutions; n1 and n2 are the number of plates used in the first and second dilutions, respectively; df is the first dilution factor; and V is the volume of inoculum placed in each plate in mL.
Sensory evaluation – difference from control (DFC) test.
Three mango jams, namely M0_1.5 (Control), M25_0 and M50_0 were evaluated for their sensory differences by 30 untrained panelists (20 females and 10 males) within the age range of 19–30 years. All participants in the DFC tests were consecutively provided with a control sample denoted as “C” and an array of three test samples spread on sandwich slices, consisting of a blind control, M25_0, and M_50 0, each of which was assigned a random three-digit code. The test participants evaluated the difference between the test samples and the control sample using a 5-point difference scale (0 = “no difference”, 1 = “very slight difference”, 2 = “slight difference”, 3 = “moderate difference”, 4 = “large difference”, and 5 = “very large difference”).33
Statistical analysis
All statistical techniques, including the normality test (Shapiro–Wilk's test), homoscedasticity of variances (Levene's test), Student's t-test for comparison of mango pulp and CPH pulp, and one-way ANOVA along with the post-hoc Tukey test for quality changes during jam storage, were performed at a 5% significance level using SPSS Statistics v22 (IBM Corp., USA). For the sensory difference test, the Dunnett test was used to distinguish the differences between two samples and the blind control at a 5% significance level.
Results and discussion
Physicochemical properties and proximate analysis of CPH and mango pulps
The physicochemical properties and approximate composition of CPH and mango pulp are presented in Table 2.
Table 2 Proximate analysis and physicochemical properties of cocoa pod husks (CPH) and mango pulpa
|
Mango pulp |
CPH pulp |
Data are presented as mean ± standard deviation of triplicates and values in the same rows with different letters represent significant differences (p < 0.05).
|
Moisture (%) |
82.56 ± 0.34a |
96.07 ± 0.08b |
Protein (%) |
1.01 ± 0.05a |
0.34 ± 0.01b |
Ash (%) |
0.21 ± 0.01a |
0.40 ± 0.01b |
Lipid (%) |
0.68 ± 0.02a |
0.65 ± 0.01b |
Carbohydrate (%) |
12.41 ± 0.34a |
1.21 ± 0.08b |
Reducing sugar (%) |
2.87 ± 0.07a |
0.71 ± 0.02b |
Pectin (%) |
— |
0.20 ± 0.02 |
Total soluble solids (°Brix) |
16.65 ± 0.52a |
2.07 ± 0.06b |
Total acidity (g citric acid/100 g) |
0.25 ± 0.01 |
— |
Phenolic (mg GAE/100 g) |
14.08 ± 0.09a |
316.84 ± 2.19b |
DPPH (mg TE/100 g) |
89.09 ± 1.91a |
96.76 ± 0.52b |
L* |
53.74 ± 0.51a |
62.72 ± 0.42b |
a* |
12.72 ± 0.04a |
1.20 ± 0.04b |
b* |
58.67 ± 0.23a |
24.25 ± 0.49b |
C* |
60.03 ± 0.23a |
24.28 ± 0.49b |
h° |
77.76 ± 0.05a |
87.48 ± 0.53b |
It can be seen that both CPH and mango pulps had a high moisture content (>80%), which is in accordance with some studies on cocoa pod husk34 and mango fruits.35 However, mango pulp has a significantly higher carbohydrate content (12.41%) than CPH pulp (1.21%). Similar trends were also observed for total soluble solids (TSSs) and reducing sugar content. In terms of bioactive compounds, although the total phenolic content of CPH pulp (316.84 mg GAE/100 g) was 22 times higher than that of mango pulp (14.08 mg GAE/100 g), their DPPH antioxidant activity was quite comparable. A possible justification is that CPH pulp predominantly comprises hydrophilic phenolic compounds, particularly flavan-3-ol compounds including epicatechin, quercetin, catechin, gallic acid, coumaric acid, and protocatechuic acid.36 In contrast, mango pulp primarily comprises high concentrations of carotenoids, in addition to other flavonoid compounds.37 In addition, pectin also accounted for a significant amount in CPH pulp (0.20%, corresponding to 5.10% on a dry basis), showing the potential of CPH pulp in stabilizing the structure of jam in formulations where mango pulp is replaced and pectin content is reduced.
As visually shown in Table 2 and Fig. 1, the L* value of CPH pulp (62.72) is greater than that of mango pulp (53.74), indicating that mango pulp is darker in color than CPH pulp. The a* and b* values (12.72 and 58.67, respectively) observed in mango pulp indicate its substantial content of carotenoids and other yellow-red compounds. Considering that the h° values of CPH pulp and mango pulp range between 77.76 and 87.48, it is evident that both materials possess a distinctive yellow hue.
 |
| Fig. 1 (A) Cocoa fruits, (B) cocoa pod husk pulp, (C) mango fruits, and (D) mango pulp. | |
Hence, CPH pulp emerges as a highly viable substitute for mango pulp in the manufacturing of jam, serving the dual purpose of utilization of resources from the cocoa sector. By incorporating CPH pulp into the jam-making process, manufacturing costs are reduced and available raw materials are utilized in a more sustainable manner.
Changes in the qualities of CPH pulp-substituted mango jam during storage
Color and appearance.
The color change of CPH pulp-substituted mango jam samples during storage is presented in Table 3 and Fig. 2. It is apparent that the control sample had a higher lightness (L* = 63.76) than the other samples; nevertheless, the M50_0 sample exhibited no significant difference on the initial day of storage. In addition, the L* value of all samples tended to decrease significantly with increasing storage time, and the lightness of all mango jam samples ranged from 33.53–48.74 at the end of storage. This behavior could be attributed to the Maillard reaction, which generates a brown pigment,38 or to elevated temperatures during jam storage, which darken the color of the jam.39,40
Table 3 Changes in color attributes and total acidity of CPH pulp-substituted mango jam during storagea
Week |
CPH pulp-substituted mango jamb (Mx_y) |
M0_1.5 |
M25_1.5 |
M25_0 |
M50_1.5 |
M50_0 |
Data are presented as mean ± standard deviation of triplicates. For each quality attribute, values within the same rows with different lowercase letters and values within the same column with different uppercase letters represent significant differences (p < 0.05).
Mx_y, where x is the substitution ratio (%) of CPH pulp and y is the amount of commercial pectin in the formulation.
|
L*
|
0 |
63.76 ± 1.40aA |
56.24 ± 1.25bA |
55.71 ± 1.84bA |
57.43 ± 1.64bA |
62.65 ± 1.80aA |
1 |
61.55 ± 2.52aA |
55.50 ± 1.18bA |
54.45 ± 1.09bA |
53.24 ± 2.19cbB |
51.32 ± 1.00cB |
2 |
51.59 ± 1.25aB |
45.43 ± 1.16bB |
43.59 ± 0.75cB |
43.31 ± 0.58cC |
46.10 ± 0.76bC |
4 |
44.16 ± 1.54aC |
33.53 ± 1.86bC |
48.74 ± 0.61cC |
34.74 ± 1.74bD |
41.93 ± 0.84aD |
![[thin space (1/6-em)]](https://www.rsc.org/images/entities/char_2009.gif) |
a*
|
0 |
7.06 ± 0.21aA |
12.68 ± 0.35bA |
13.07 ± 0.49bA |
13.23 ± 0.54bA |
11.44 ± 0.33cA |
1 |
8.82 ± 0.69aB |
12.88 ± 0.52bA |
16.63 ± 0.58cB |
15.78 ± 0.21cB |
15.37 ± 0.62cB |
2 |
10.82 ± 0.43aC |
10.58 ± 0.38aB |
14.53 ± 0.35bA |
14.96 ± 0.17cB |
14.12 ± 0.73bC |
4 |
9.87 ± 0.30aD |
13.52 ± 0.27bC |
31.83 ± 1.39cC |
17.64 ± 0.81dC |
16.77 ± 0.66dD |
![[thin space (1/6-em)]](https://www.rsc.org/images/entities/char_2009.gif) |
b*
|
0 |
74.43 ± 2.75aA |
65.27 ± 1.88bA |
69.11 ± 2.17cA |
55.02 ± 1.67dA |
54.13 ± 2.28dA |
1 |
76.36 ± 0.34aB |
62.34 ± 3.03bB |
77.71 ± 3.13aAB |
63.61 ± 1.88bcB |
67.44 ± 2.05cB |
2 |
55.19 ± 1.36aC |
43.30 ± 1.18bcC |
44.01 ± 1.44cC |
41.68 ± 0.83bC |
43.34 ± 1.81bcC |
4 |
78.26 ± 3.11aB |
48.82 ± 0.55bD |
73.98 ± 3.34cB |
57.05 ± 1.31dA |
59.79 ± 2.64dD |
![[thin space (1/6-em)]](https://www.rsc.org/images/entities/char_2009.gif) |
C*
|
0 |
69.44 ± 2.60baA |
66.53 ± 1.96aA |
76.02 ± 2.6bA |
56.59 ± 1.72cA |
55.45 ± 2.44cC |
1 |
78.08 ± 1.08aB |
62.68 ± 1.19bB |
78.20 ± 3.17aA |
65.78 ± 2.24bB |
69.91 ± 1.90cB |
2 |
56.17 ± 1.55aC |
44.63 ± 1.05bcC |
46.36 ± 1.42cB |
44.28 ± 0.81bC |
45.67 ± 1.53bcC |
4 |
74.66 ± 3.11aB |
50.62 ± 0.58bB |
84.82 ± 3.74cC |
59.63 ± 1.17dD |
61.89 ± 2.33dD |
![[thin space (1/6-em)]](https://www.rsc.org/images/entities/char_2009.gif) |
h°
|
0 |
84.02 ± 1.20aA |
78.90 ± 0.56bA |
78.40 ± 1.63bA |
76.48 ± 0.40cA |
77.54 ± 0.87bcA |
1 |
82.84 ± 1.82aA |
78.14 ± 0.37bA |
77.86 ± 1.33bA |
75.42 ± 0.65bcA |
73.01 ± 3.36cBC |
2 |
79.34 ± 1.33aB |
76.10 ± 1.22bB |
71.74 ± 0.56cB |
70.25 ± 0.32dB |
71.66 ± 1.55cdB |
4 |
82.39 ± 0.38aC |
75.40 ± 1.13bB |
68.65 ± 2.16cC |
71.71 ± 2.23dB |
75.12 ± 1.69bAC |
![[thin space (1/6-em)]](https://www.rsc.org/images/entities/char_2009.gif) |
Total acidity (g citric acid/100 g)
|
0 |
1.43 ± 0.07aA |
1.54 ± 0.06abA |
1.70 ± 0.09bA |
1.50 ± 0.03aAB |
1.52 ± 0.06aA |
1 |
1.37 ± 0.02aA |
1.53 ± 0.02bA |
1.61 ± 0.02cAB |
1.54 ± 0.02bA |
1.52 ± 0.02bA |
2 |
1.40 ± 0.01aA |
1.49 ± 0.01bAB |
1.54 ± 0.01cB |
1.48 ± 0.01bB |
1.57 ± <0.01dA |
4 |
1.17 ± 0.01aB |
1.43 ± <0.01dB |
1.37 ± 0.01cC |
1.25 ± 0.01bC |
1.31 ± 0.01cB |
![[thin space (1/6-em)]](https://www.rsc.org/images/entities/char_2009.gif) |
TSS (°Brix)
|
0 |
60.75 ± 4.68aA |
70.20 ± 3.12bA |
75.60 ± 2.20cA |
75.15 ± 1.56cA |
70.88 ± 2.59bA |
1 |
58.73 ± 0.45aAB |
67.73 ± 1.13bAB |
74.93 ± 2.97cA |
57.35 ± 7.05aB |
68.85 ± 4.73bA |
2 |
62.33 ± 1.13aA |
72.00 ± 0.10bA |
73.13 ± 2.97bA |
64.80 ± 3.20aC |
68.85 ± 1.56cA |
4 |
61.88 ± 2.59aA |
71.33 ± 1.35bA |
72.00 ± 0.73bA |
63.90 ± 5.30aC |
72.45 ± 0.90bAB |
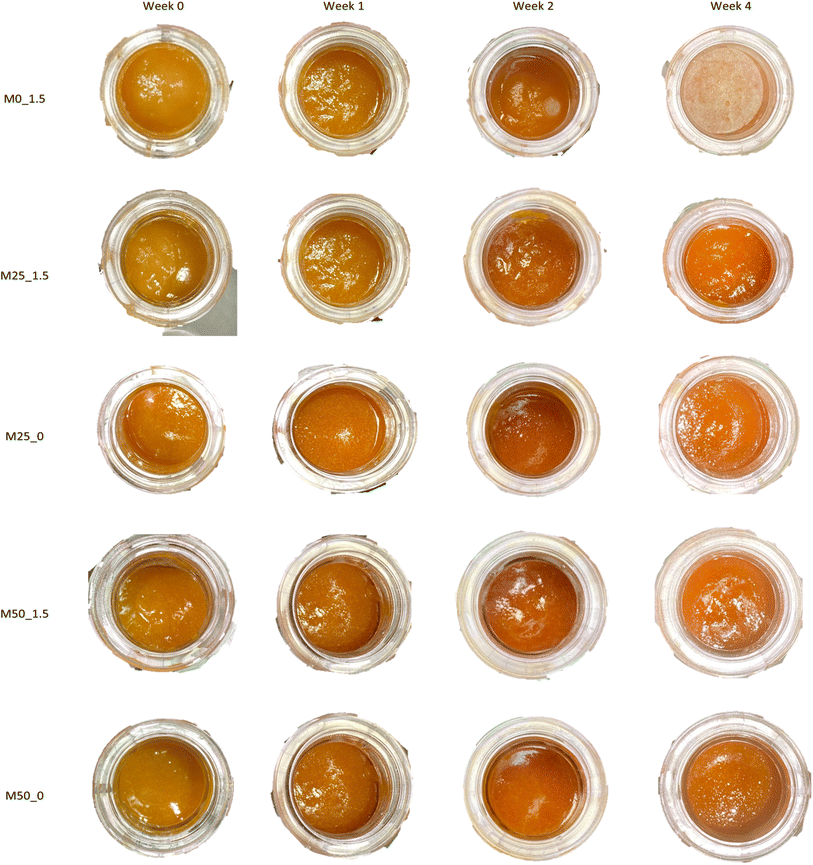 |
| Fig. 2 Appearance of CPH pulp-substituted mango jam during storage. Notes: Mx_y, where x is the substitution ratio (%) of CPH pulp and y is the amount of commercial pectin in the formulation. | |
In general, the increase in CPH pulp content decreased the b* value (yellowness) of the mango jam samples throughout the storage, indicating that the addition of CPH pulp to the sample resulted in a less yellow product. In the meantime, the b* values of the mango jam samples at the end of storage decreased slightly compared to the first day of storage. The change in the b* value (yellowness) during storage may be due to the decomposition of carotenoids and chlorophyll pigments.41 During storage, the control sample (M0_1.5) exhibited a range of h° values between 79° and 84°, as illustrated by its yellow to green hue. In contrast, the h° values of all samples substituted with CPH pulp fall within the range of 68–79°, indicating that these samples do not differ in color from the control sample. According to Kumar et al. (2021),42 the high carotenoid content of CPH pulp (64.35 mg g−1) exhibited colors comparable to the control sample.
Reducing sugar, TSS and total acidity.
Changes in reducing sugar content of CPH pulp-substituted mango jam samples during storage are presented in Fig. 3. The data indicate that the reducing sugar content of mango jam samples varied between 19.20 and 25.25 g/100 g on the initial day of storage. Notably, this value exhibited a substantial increase (p < 0.05) as the storage period progressed. Similar findings were documented in studies pertaining to the storage of apple jam,43 grape jam and apricot jam.44 During storage, temperature and acidic conditions catalyze the conversion of sucrose to reducing sugars, which results in an increase in reducing sugars.45
 |
| Fig. 3 Change in reducing sugar content (g/100 g) of CPH pulp-substituted mango jam during storage. Notes: Mx_y, where x is the substitution ratio (%) of CPH pulp and y is the amount of commercial pectin in the formulation; different lowercase letters and uppercase letters indicate the significant differences at the 95% confidence level (p < 0.05) among samples at the same storage time and among different storage times for the same samples, respectively. | |
Regarding the total soluble solid content, the results showed that increasing the ratio of pectin replacement with CPH pulp significantly increased (p < 0.05) the TSS value and tended to increase gradually with the increasing storage time of mango jam (Table 3). Compared with the control sample (M0_1.5), the TSS of M50_0 was significantly higher than that of the control sample throughout the storage period. The increase in TSS of the mango jam samples may be due to the degradation of polysaccharides into monosaccharides, oligosaccharides, and sugar fermentation.46,47
Total acidity is one of the most important physicochemical parameters that affect product quality, especially the sensory value and, to a certain extent, is effective against the growth of microorganisms.38,48Table 3 reveals that the variation in titratable acidity across the five jam samples was negligible. Furthermore, this parameter exhibited a slight decline as the jam samples were subjected to storage, averaging 1.17–1.43 g of citric acid/100 g. The decrease in titratable acidity of mango jam during storage may be due to the degradation of ascorbic acid (vitamin C) present in mango, which is influenced by storage time and temperature.49,50
Total phenolic content and DPPH antioxidant activity.
Phenolic content plays a significant role in foods as the major antioxidant components.51 The change in phenolic content of mango jams over storage time is presented in Fig. 4.
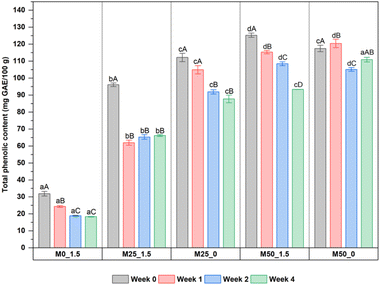 |
| Fig. 4 Change in total phenolic content (mg GAE/100 g) of CPH pulp-substituted mango jam during storage. Notes: Mx_y, where x is the substitution ratio (%) of CPH pulp and y is the amount of commercial pectin in the formulation; different lowercase letters and uppercase letters indicate the significant differences at the 95% confidence level (p < 0.05) among samples at the same storage time and among different storage times for the same samples, respectively. | |
It is noticeable that the addition of CPH pulp remarkably enhanced phenolic content of mango jams, from 31.91 mg GAE/100 g for the control sample to 96.10–125.28 mg GAE/100 g for four CPH–mango jam samples. This result is due to the inherent high phenolic content of CPH pulp (Table 2), which contributes to the total content of CPH–mango jams and its retention. Considering the effect of storage time, the phenolic content tended to decrease throughout the storage and showed stability for the last two weeks. The gradual decrease in TPC with increasing storage time is attributed to the non-enzymatic oxidation caused by high temperature during storage.52
A similar trend to phenolic content was also observed for DPPH antioxidant activity. Specifically, the addition of CPH pulp increased antioxidant activity by 1.5 times, from 57.76 mg TE/100 g for the control sample to 73.30–83.08 mg TE/100 g for four CPH–mango jam samples (Fig. 5). It should be mentioned that unlike the phenolic content, DPPH activity did not increase substantially when CPH was added. However, after two weeks of room temperature storage, there was a notable decline (60.3–72.7%), followed by stability in the last two weeks with values ranging from 21.13–32.87 mg TE/100 g for all samples. A decrease in antioxidant activity during storage was also reported for blueberry jam,53 and grape peel jam.54 One explanation for the different trends between phenolic content and DPPH activity may be that during storage, highly biologically active components such as flavonoids, betalains and carotenoids are significantly decomposed.52
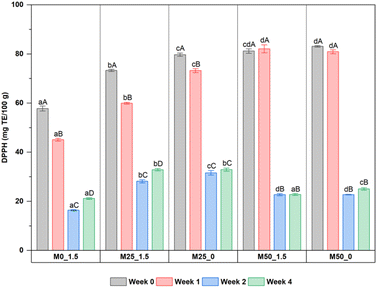 |
| Fig. 5 Change in DPPH antioxidant activity (mg TE/100 g) of CPH pulp-substituted mango jam during storage. Notes: Mx_y, where x is the substitution ratio (%) of CPH pulp and y is the amount of commercial pectin in the formulation; different lowercase letters and uppercase letters indicate the significant differences at the 95% confidence level (p < 0.05) among samples at the same storage time and among different storage times for the same samples, respectively. | |
Textural properties.
Textural attributes including hardness, adhesiveness, and springiness of CPH pulp-substituted mango jam after 1-week storage are presented in Fig. 6. Hardness is defined as the amount of force required to achieve a specific level of deformation55 and is a typical parameter for estimating the product's gel structure and consistency or homogeneity. 56 Obviously, the hardness of the jam was increased by roughly 15–25% compared to the control sample upon CPH substitution at a fixed content of commercial pectin (M25_1.5 and M50_1.5). Meanwhile, when commercial pectin was excluded from the formulation (M25_0 and M50_0), a reduction of up to 43.5% in hardness was observed. In addition, Fig. 6 shows that adhesiveness and springiness tended to gradually increase with the increasing CPH pulp ratio, from 0.15 mJ and 3.12 mm for the control to 0.8–0.85 mJ and 2.60–3.48 mm for M25, and 0.90–1.12 mJ and 1.57–3.84 mm for M50, respectively.
 |
| Fig. 6 Texture profiles of CPH pulp-substituted mango jam after 1-week storage. Notes: Mx_y, where x is the substitution ratio (%) of CPH pulp and y is the amount of commercial pectin in the formulation; different lowercase letters for each textural attributes indicate the significant differences at the 95% confidence level (p < 0.05). | |
One of the reasons leading to the increase in hardness of samples M25_1.5 and M50_1.5 is that CPH pulp contains a high level of dietary fibers and thickening agents, especially pectin, 20 which contributes to an increase in the number of interfacial zones. 57,58 In addition, the high mineral content such as calcium in CPH pulp also contributes to increasing the hardness of CPH–mango jam. The high amounts of hemicellulose and gelling agents in cocoa fruits20,21 are responsible for the increased adhesiveness.59 Meanwhile, insoluble fiber in CPH pulp increases water retention capacity, thereby improving the springiness of mango jam.60 Belović et al. (2017)61 suggested that jams with added pectins often demonstrate greater adhesiveness and spreadability owing to enhanced integration of fruit solids within the pectin matrix.
Microbiological qualities.
Fruit jam is susceptible to microbial degradation due to its substantial moisture content, which can reach 40% on average.51 Microbiological qualities of CPH pulp-substituted mango jam after 4 weeks of storage are presented in Table 4. It can be seen that the total plate counts, molds and yeasts of all jam samples were of good quality and within the allowable limits. In addition, no E. coli and coliforms was detected in any of the mango jam samples, suggesting that processed jam, with a low water activity of approximately 0.86, inhibits the growth of the majority of bacteria.51 These results may be also attributed to the presence of β-carotene and acidic nature of jam.62
Table 4 Microbiological quality of CPH pulp-substituted mango jam after 4-week storagea
Microbiological attribute (CFU g−1) |
CPH-substituted mango jamb (Mx_y) |
M0_1.5 |
M25_1.5 |
M25_0 |
M50_1.5 |
M50_0 |
n.d. – not detected.
Mx_y, where x is the substitution ratio (%) of CPH pulp and y is the amount of commercial pectin in the formulation.
|
Total plate count |
n.d. |
n.d. |
1 × 101 |
n.d. |
1 × 101 |
E. coli
|
n.d. |
n.d. |
n.d. |
n.d. |
n.d. |
Coliforms |
n.d. |
n.d. |
n.d. |
n.d. |
n.d. |
Total yeasts and molds |
1 × 101 |
1 × 101 |
2 × 101 |
1 × 101 |
n.d. |
Difference from the control test for CPH pulp-substituted mango jam.
The sensory differences between the CPH pulp-substituted mango jam (M25_0 and M50_0) and the control sample (M0_1.5) are shown in Fig. 7. The sensory discrimination between the jam samples supplemented with CPH pulp (M25_0 and M50_0) and the control sample is perceptible to consumers, although there is no significant difference between the two samples with difference scores being 2.83 and 3.13, respectively. This distinction can be attributed to the characteristic sour flavor and aroma of mango pulp, which was lowered in these two samples. However, it is interesting that the inclusion of the blind control in the test samples also resulted in a difference score of 2.27, indicating a slight difference between the CPH–mango jam and normal mango jam. This may be due to the discriminating power of the panelists, which is verified through the introduction of the blind control.
 |
| Fig. 7 Difference from the control test of CPH pulp-substituted mango jam, compared with the M0_1.5 sample as the blind control. Notes: Mx_y, where x is the substitution ratio (%) of CPH pulp and y is the amount of commercial pectin in the formulation. | |
Conclusions
The feasibility of replacing mango pulp in jam formulation with pulp obtained from cocoa pod husks, an agricultural waste from cocoa cultivation and production, was investigated with the aim of utilization of agricultural resources. CPH pulp obtained from fresh cocoa fruits showed potential as a substitute for mango pulp owing to its high pectin content and characteristic yellow color similar to mango. Furthermore, the antioxidant content and activity of CPH–mango jam are enhanced by the substantial phenolic content present in CPH pulp. The characteristic flavor of mango jam was not altered by the plant taste of CPH pulp, leading to a minor sensory difference compared to traditional mango jam. The present study collectively highlighted the applicability of pectin-rich pulp from CPH for pulp substitution in fruit jams, offering unique benefits in lowering the production cost and addressing environmental challenges for sustainability.
Data availability
The data supporting this article have been included as part of the ESI.†
Author contributions
Vinh-Lam Nguyen: data curation; visualization; investigation; writing – original draft. Minh-Thoai Tran: data curation; investigation. Thuy-Diem Nguyen-Thi: data curation; investigation. Minh-Anh Nguyen: data curation; investigation. Minh-Tri Le: data curation; investigation. Tra-My Nguyen: data curation; investigation. Quoc-Duy Nguyen: conceptualization; data curation; visualization; investigation; methodology; writing – original draft; writing – review & editing.
Conflicts of interest
There are no conflicts to declare.
Acknowledgements
The authors would like to thank Nguyen Tat Thanh University for granting permission and providing facilities during the research period. Additionally, the authors express their gratitude to Ms Thi-Hoa Ngo and Ms Nhu-Ngoc Nguyen for their guidance on jam recipes and microbiological assays, respectively.
Notes and references
- M. García-Mahecha, H. Soto-Valdez, E. Carvajal-Millan, T. J. Madera-Santana, M. G. Lomelí-Ramírez and C. Colín-Chávez, Molecules, 2023, 28, 458 CrossRef PubMed.
- V. Coman, B.-E. Teleky, L. Mitrea, G. A. Martău, K. Szabo, L.-F. Călinoiu and D. C. Vodnar, Adv. Food Nutr. Res., 2020, 91, 157–225 CrossRef CAS PubMed.
- B. Kaur, P. S. Panesar, A. K. Anal and S. Chu-Ky, Food Rev. Int., 2023, 39, 4159–4179 CrossRef CAS.
- V. M. Lieb, L. K. Schuster, A. Kronmüller, H.-G. Schmarr, R. Carle and C. B. Steingass, Food Res. Int., 2019, 116, 527–537 CrossRef CAS PubMed.
- P. W. Mwaurah, S. Kumar, N. Kumar, A. Panghal, A. K. Attkan, V. K. Singh and M. K. Garg, Compr. Rev. Food Sci. Food Saf., 2020, 19, 2421–2446 CrossRef CAS PubMed.
- M. Bekele, N. Satheesh and J. A. Sadik, Sci. Afr., 2020, 7, e00277 CAS.
- B. Dereje and S. Abera, Cogent Food Agric., 2020, 6, 1747961 CrossRef.
-
M. Siddiq, D. S. Sogi and S. Roidoung, Handbook of Mango Fruit: Production, Postharvest Science, Processing Technology and Nutrition, 2017, pp. 195–216 Search PubMed.
- N. D. Vu, V. M. Nguyen and T. T. Tran, Int. J. Food Sci., 2023, 2023, 6618300 Search PubMed.
- R. A. Baker, N. Berry, Y. H. Hui and D. M. Barrett, Process. Fruits:Sci. Technol., 2005, 2, 112–125 Search PubMed.
- S. Basu and U. S. Shivhare, J. Food Eng., 2010, 100, 357–365 CrossRef CAS.
- H. S. Vibhakara and A. S. Bawa, Handb. Fruits Fruit Process., 2006, 187–204 Search PubMed.
- S. Garg, P. Ghosh, S. S. Rana and R. C. Pradhan, Int. J. Fruit Sci., 2019, 19, 29–44 CrossRef.
- F. Munarin, M. C. Tanzi and P. Petrini, Int. J. Biol. Macromol., 2012, 51, 681–689 CrossRef CAS PubMed.
- M. Moslemi, Carbohydr. Polym., 2021, 254, 117324 CrossRef CAS PubMed.
-
T. Vanitha and M. Khan, in Pectins-Extraction, Purification, Characterization and Applications, IntechOpen, London, 2019, vol. 10, pp. 85–105 Search PubMed.
- E. D. Mahmood Akhtar, J. Mazoyer and V. Langendorff, Gums Stab. Food Ind. Appl., 2002, 11, 311 Search PubMed.
- C.-L. Lee, C.-C. Chang, H.-W. Kuo and W. Cheng, Fish Shellfish Immunol., 2020, 107, 357–366 CrossRef CAS PubMed.
-
Food and Agriculture Organization (FAO), FAOSTAT Statistical Database, 2018 Search PubMed.
- J. P. Jarrín-Chacón, J. Núñez-Pérez, R. d. C. Espín-Valladares, L. A. Manosalvas-Quiroz, H. M. Rodríguez-Cabrera and J. M. Pais-Chanfrau, Foods, 2023, 12, 590 CrossRef PubMed.
- R. Campos-Vega, K. H. Nieto-Figueroa and B. D. Oomah, Trends Food Sci. Technol., 2018, 81, 172–184 CrossRef CAS.
-
K. P. Prabhakaran Nair, in The Agronomy and Economy of Important Tree Crops of the Developing World, 2010, pp. 131–180 Search PubMed.
- D. Velásquez-Jiménez, I. Luzardo-Ocampo, M. Gaytán-Martínez and R. Campos-Vega, Food Biosci., 2023, 56, 103199 CrossRef.
-
A. Dewan, M. Malik, V. Chaudhary and A. Sharma, in Agro-Wastes for Packaging Applications, CRC Press, 2024, pp. 264–287 Search PubMed.
- T. Belwal, C. Cravotto, S. Ramola, M. Thakur, F. Chemat and G. Cravotto, Foods, 2022, 11, 798 CrossRef CAS PubMed.
- A. E. K. Afedzi, F. Obeng-Boateng, M. S. Aduama-Larbi, X. Zhou and Y. Xu, Biocatal. Agric. Biotechnol., 2023, 102835 CrossRef CAS.
- F. Ramos-Escudero, A. Rojas-García, M. d. l. L. Cádiz-Gurrea and A. Segura-Carretero, Ultrason. Sonochem., 2024, 106, 106887 CrossRef CAS PubMed.
-
AOAC International, Official methods of analysis of AOAC International, 17th edn, 2000 Search PubMed.
- P. H. F. Pereira, T. Í. S. Oliveira, M. F. Rosa, F. L. Cavalcante, G. K. Moates, N. Wellner, K. W. Waldron and H. M. C. Azeredo, Int. J. Biol. Macromol., 2016, 88, 373–379 CrossRef CAS PubMed.
-
ISO, in ISO 14502-1 International Standardization, International Organization for Standardization, Switzerland, 2005, p. 10 Search PubMed.
- G. Marinova and V. Batchvarov, Bulg. J. Agric. Sci., 2011, 17, 11–24 Search PubMed.
- G. L. Miller, Anal. Chem., 1959, 31, 426–428 CrossRef CAS.
- J. Leong, C. Kasamatsu, E. Ong, J. T. Hoi and M. N. Loong, Food Sci. Nutr., 2016, 4, 469–478 CrossRef CAS PubMed.
- O. A. A. Eletta, A. G. Adeniyi, J. O. Ighalo, D. V. Onifade and F. O. Ayandele, Environ. Technol. Rev., 2020, 9, 20–36 CrossRef.
- S. Sarkiyayi, M. Mohammed and A. Yakubu, Res. J. Appl. Sci., Eng. Technol., 2013, 5, 387–391 CrossRef CAS.
- R. Martínez, P. Torres, M. A. Meneses, J. G. Figueroa, J. A. Pérez-Álvarez and M. Viuda-Martos, Food Res. Int., 2012, 49, 39–45 CrossRef.
- E. N. Ellong, S. Adenet and K. Rochefort, Food Nutr. Sci., 2015, 6, 242 CAS.
- N. Touati, M. P. Tarazona-Díaz, E. Aguayo and H. Louaileche, Food Chem., 2014, 145, 23–27 CrossRef CAS PubMed.
- A. Dubey, A. Kumar and P. S. Rao, J. Food Meas. Char., 2021, 15, 961–975 CrossRef.
- J. Vukoja, A. Pichler and M. Kopjar, Foods, 2019, 8, 255 CrossRef CAS PubMed.
- M. Maskan, J. Food Eng., 2001, 48, 169–175 CrossRef.
-
D. Kumar, M. Shamim, S. K. Arya, M. W. Siddiqui, D. Srivastava and S. Sindhu, Challenges and Opportunities of Circular Economy in Agri-Food Sector: Rethinking Waste, 2021, pp. 191–226 Search PubMed.
- T. U. Rahman, N. T. Amanullah, A. Tahir, A. U. Rahman and A. Khan, Pure Appl. Biol., 2018, 7, 1064–1073 Search PubMed.
- E. B. Ehsan, Z. P. Naeem, A. Javed and A. Nazir, Pak. J. Food Sci., 2003, 13, 11–15 Search PubMed.
- S. Wisal, M. A. Mashwani and S. Noor, Int. J. Basic Appl. Sci., 2014, 14, 36–42 Search PubMed.
- A. Q. Chong, N. L. Chin, R. A. Talib and R. K. Basha, J. Sci. Food Agric., 2024, 105, 285–293 CrossRef PubMed.
- S. Ali, S. Ishtiaq, A. Nawaz, S. Naz, S. Ejaz, M. W. Haider, A. A. Shah, M. M. Ali and S. Javad, Int. J. Biol. Macromol., 2024, 256, 128429 CrossRef CAS PubMed.
-
P. Myintzaw and M. Callanan, in Basic Protocols in Predictive Food Microbiology, ed. V. O. Alvarenga, Springer US, New York, NY, 2023, pp. 47–64 Search PubMed.
- R. A. Morais, C. M. d. S. Soares, R. R. da Silva, L. d. S. Gualberto, B. C. B. de Freitas, E. E. N. Carvalho and G. A. d. S. Martins, Food Sci. Technol., 2022, 42, e116321 CrossRef.
- A. P. R. Campos, R. C. Chisté and R. d. S. Pena, J. Food Process. Preserv., 2022, 46, e16509 CAS.
- A. Aslam, T. Zahoor, M. R. Khan, A. Khaliq, M. Nadeem, A. Sagheer, M. F. J. Chugtai and M. W. Sajid, J. Food Process. Preserv., 2019, 43, e13941 CrossRef.
- T. Wang, L. Liu, A. Rakhmanova, X. Wang, Y. Shan, Y. Yi, B. Liu, Y. Zhou and X. Lü, Food Biosci., 2020, 38, 100788 CrossRef CAS.
- T. Lafarga, I. Aguiló-Aguayo, G. Bobo, A. V Chung and B. K. Tiwari, J. Food Process. Preserv., 2018, 42, e13666 CrossRef.
- F. L. Amorim, M. B. de Cerqueira Silva, M. G. Cirqueira, R. S. Oliveira, B. A. S. Machado, R. G. Gomes, C. O. de Souza, J. I. Druzian, E. de Souza Ferreira and M. A. Umsza-Guez, Food Sci. Nutr., 2019, 7, 1584–1594 CrossRef CAS PubMed.
- M. Mousavi, A. Heshmati, A. D. Garmakhany, A. Vahidinia and M. Taheri, LWT, 2019, 102, 80–88 CrossRef CAS.
- S. Jribi, M. Ouhaibi, H. Boukhris, C. Damergi and H. Debbabi, J. Food Meas. Char., 2021, 15, 1578–1587 CrossRef.
- N. K. Alqahtani, T. M. Alnemr, A. R. Ahmed and S. Ali, Processes, 2022, 10, 2442 CrossRef CAS.
- A. Nourmohammadi, E. Ahmadi and A. Heshmati, Food Sci. Nutr., 2021, 9, 2483–2496 CrossRef CAS PubMed.
- M. Abid, H. Yaich, H. Hidouri, H. Attia and M. A. Ayadi, Food Chem., 2018, 239, 1047–1054 CrossRef CAS PubMed.
- L. E. Figueroa and D. B. Genovese, Food Technol. Biotechnol., 2018, 56, 441–453 CAS.
- M. Belović, A. Torbica, I. Pajić-Lijaković and J. Mastilović, Food Chem., 2017, 237, 1226–1233 CrossRef PubMed.
- N. A. Afoakwah, F. K. Amagloh, G. K. Mahunu, S. W. Ayyub, W. Tchabo and P. Owusu-Ansah, J. Agric. Food Res., 2023, 12, 100540 CAS.
|
This journal is © The Royal Society of Chemistry 2025 |
Click here to see how this site uses Cookies. View our privacy policy here.