DOI:
10.1039/D4VA00019F
(Perspective)
Environ. Sci.: Adv., 2024,
3, 1364-1384
Chemical transformation, exposure assessment, and policy implications of fluorotelomer alcohol partitioning from consumer products to the indoor and outdoor environment—from production to end-of-life
Received
25th January 2024
, Accepted 26th July 2024
First published on 7th August 2024
Abstract
Fluorotelomer (FT) alcohols (FTOHs) belong to the subclass of per- and polyfluoroalkyl substances (PFAS) and are used as building blocks of FT-based side chain fluorinated polymers (SCFPs), which are applied to consumer products to provide hydro- and oleophobic characteristics. FTOHs are consistently detected in consumer products, indicating FTOHs as major degradation products of FT-based SCFPs. Literature on FTOHs indicates that much is known about the release of FTOHs during the production, throughout the lifetime, and at the end-of-life of consumer products. This Perspective combines information from FTOHs in consumer products with sufficient knowledge on FTOH volatility, partitioning to the gas phase, and transformation to perfluorocarboxylates (PFCAs) to understand the extent of FTOH release to the environment. In the indoor environment, FTOHs are released in textile factories to the air during the production of consumer products, indicating a potential inhalation risk for the workers. Meanwhile, indoor air FTOH levels at residential sites are estimated to pose low inhalation risk to humans based on studies of 8:2 FTOH, which is known to undergo human metabolism to perfluorooctanoate (PFOA). Release of FTOHs from FT-based SCFP-applied consumer products to the indoor environment throughout the lifetime of the products is known, as well as release to the outdoor environment through washing, weathering, or drying. At the end-of-life of consumer products, FTOHs are released to air from landfills and can be detected in biosolids. Future policies need to not only account for FTOH presence in consumer products, but also the known FTOH volatility, partitioning to the gas phase, and transformation to PFCAs.
Environmental significance
Fluorotelomer (FT) alcohols (FTOHs) are per- and polyfluoroalkyl substances (PFAS) sub-class consistently detected in consumer products. Much is known about FTOHs, indicating their volatility, partitioning to the gas-phase, and transformation to perfluoroalkyl carboxylates (PFCAs). Based on the literature from 2000 to 2024, a Perspective on FTOHs in consumer products and FTOH release from consumer products to the indoor and outdoor environment is presented. Release of FTOHs from consumer products to indoor air is known during the production and throughout the lifetime of consumer products, indicating potential inhalation risk to humans. Release of FTOHs from consumer products to outdoor air occurs at landfills, indicating sources of FTOHs to the atmosphere. Future policies on FTOHs can be tied to their known transformation to PFCAs.
|
1. Introduction
Per- and polyfluoroalkyl substances (PFAS) are a group of compounds known to be present in consumer products.1 Fluorotelomer (FT) alcohols (FTOHs) belong to the PFAS sub-class and are consistently detected in consumer products,1–42 with 8:2 or higher chain FTOHs as the predominant compounds found in studies published from 2000s–2010s and 6:2 FTOH as the predominant compound found in recent years.1 The presence of FTOHs in consumer products can originate from the degradation products of the unreacted raw materials and reaction intermediates of FT-based side-chain fluorinated polymers (SCFPs),43,44 and from the degradation of FT-based SCFPs once applied to consumer products.44 Unlike fluoropolymers, where the backbone is fluorinated (i.e., containing the –CF2– fragment),45–48 FT-based SCFPs can be composed of a fluorinated or non-fluorinated backbone as long as the side-chain is fluorinated (Fig. 1).44,49,50 Some SCFP-containing products are sold directly to the public, including those for carpet treatment,2,49,51,52 and application of SCFPs in some food contact materials is approved.11,53 The FTOHs found in PFAS-containing textiles are known to originate from FT-based SCFPs.19,25,36,38,41,44,54–61 While there are multiple types of SCFPs,44 only FT-based SCFPs, such as FT-acrylate and -urethane SCFPs,44 will degrade to FTOHs (Fig. 1).
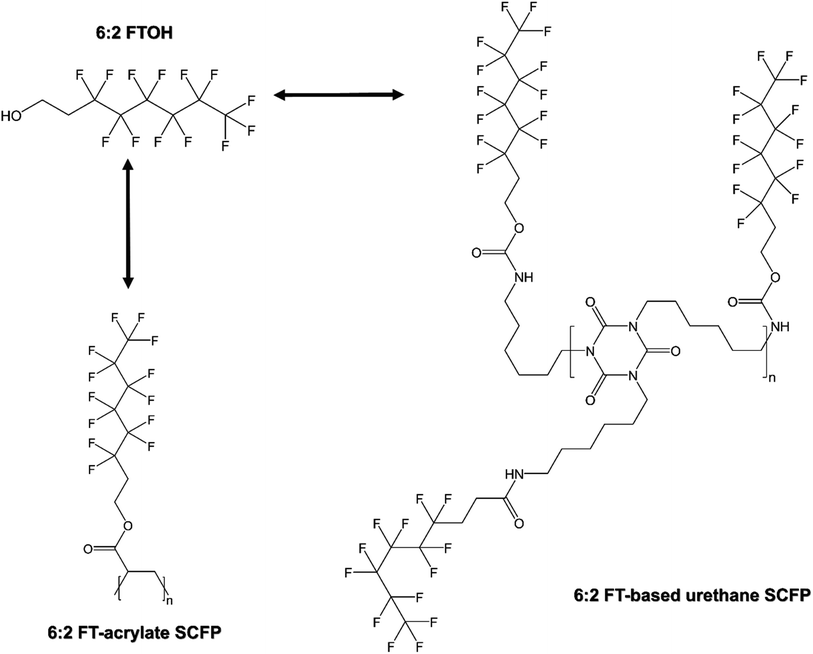 |
| Fig. 1 Example of two FT-based SCFP structures that can degrade to FTOHs because FTOHs are the building blocks of SCFPs, with SCFP structures adapted from ref. 44. Bidirectional arrows indicate that FTOHs can be degradation products of FT-based SCFPs. | |
A strong body of work exists on FTOH physicochemical properties, namely FTOH volatility and partitioning to the gas phase.62 Studies by Endo and colleagues,63–65 and by Dreyer et al.,66 using a combination of experimental (e.g., hexane–water, octanol–water partitioning, and gas and liquid chromatography) and computational (e.g., COSMOtherm) approaches, indicate the volatile nature of FTOHs. FTOHs are volatile because at environmentally-relevant pH, FTOHs are neutral (i.e., pKa > 14);67–71 in contrast, perfluoroalkyl carboxylates (PFCAs), such as perfluorooctanoate (PFOA), are charged (i.e., pKa < 0–4).68–70,72–74 In air samples, FTOHs are known to partition to the gas-phase relative to the particle-phase.35,39,62,68,70,72,75–104 When FTOH levels in the gas-phase are compared to sum FTOH levels in the gas- and particle phases, FTOHs in the gas-phase associated fraction represent 90% of the sum,72,75,86,105 whereas FTOHs in the particle-phase associated fraction represent 3–40% of the sum.72,85,86,105
There is sufficient knowledge to indicate that any n:2 FTOH (n = 6, 8, 10, …) consistently degrades to Cn and C[n − 2] PFCAs, and [n − 1]:3 FTCA, with [n − 1]:3 FT-unsaturated-carboxylate (FTUCA) and [n − 1]:2 secondary FTOH (sFTOH) as some of the observed intermediate products (Fig. 2).62 The transformation from FTOHs to PFCAs can occur in environmental and biological systems.106 FTOHs are not only known to undergo atmospheric transformation to PFCAs,76,98,107–122 but also long range atmospheric transport, thus partially explaining the presence of PFCAs in remote areas.79,91,100,113,121,123–128 However, volatility, wet deposition, and scavenging can impact the amount of PFCAs formed from atmospheric transformation of FTOHs.122 Biotransformation of FTOHs in soil,129–150 sludge,151–159 and sediment160–162 also results in PFCAs. However, FTOHs in soil can be taken up by plants before biotransforming to PFCAs.163–169 Aerobic conditions promote faster degradation (less than 1 to 14 days half-life) than anaerobic conditions (less than 1 to more than 365 days half-life) of FTOHs,170 and nutrients and redox conditions are known to impact biotransformations.143 Meanwhile, FTOHs in water samples are known to undergo photolysis to PFCAs,118,171,172 but microbial biodegradation of 8:2 FTOH to perfluorooctanoate (PFOA) in brackish water is known as well.173 Nitrate and hydroxyl radicals promote photolysis, but dissolved organic carbon inhibits photolysis.171 In biological systems, mammalian,174–193 avian,194 and fish195,196 metabolisms biotransform FTOHs to PFCAs.
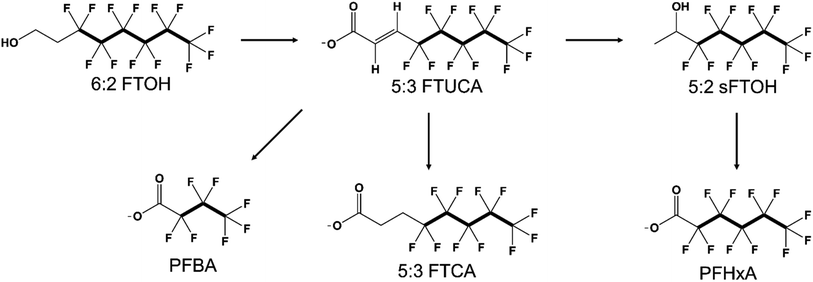 |
| Fig. 2 A degradation pathway of 6:2 FTOH leading to the formation of PFCAs, namely perfluorohexanoate (PFHxA) and perfluorobutanoate (PFBA), and 5:3 FTCA with 5:3 FTUCA and 5:2 sFTOH as intermediate products. Carbon–fluorine bonds are highlighted in bold. Detailed degradation pathways are outlined in ref. 62. | |
The knowledge on the presence of FTOHs in consumer products and on the volatility, partitioning, and transformation of FTOHs in environmental and biological systems indicates that much is known about FTOHs. Therefore, in this personal review (Perspective), studies concerning the release of FTOHs from production to end-of-life of consumer products and on FTOHs are combined to provide further understanding in the field of PFAS. Release of FTOHs in the context of consumer products is important to examine not only due to the potential exposure of FTOHs to humans (i.e., release to the indoor environment),197 but also due to the eventual degradation of FTOHs to PFCAs in the outdoor environment.107 Policy implications from the presence of FTOHs in consumer products and from transformation of FTOHs to PFCAs in the environment are briefly discussed.
2. Methods
This Perspective is based on publications concerning FTOHs from 2000 to 2024, collated by searching for publications in Google Scholar and Web of Science using the term “fluorotelomer alcohol”. In each of the papers, both the cited and citing references are consulted and this manual iterative process finishes once there is no newer, relevant citing reference. Publications prior to 2000 relevant to the Perspective are included to account for knowledge that has existed for more than two decades. Relevant, newer (e.g., 2024) publications are collated through daily search using the keyword “PFAS” on the search function of the American Chemical Society, the Royal Society of Chemistry, and Elsevier publication websites from mid-2023 till May 2024.
3. Release of FTOHs to the indoor environment
3.1 Release to the indoor environment during the production of consumer products
The padding process, a technique where chemicals are applied to fibers by way of immersion in a bath, followed by a curing step in an oven55,198,199 (i.e., heating, which leads to volatilization) is a method to deposit FT-based SCFPs onto textiles.60,200 When U.S. EPA scientists replicated the textile padding process to apply C6 FT-based SCFPs in a laboratory setting,200 6:2 FTOH was released to the indoor air, but 6:2 FTOH concentration was not provided in the study. The result from the U.S. EPA study corroborated the results from a 2016 study performed at a Chinese textile factory201 where FTOHs (9.5–90 × 106 pg m−3) were present in indoor air collected from the workshop area where the padding processes to apply FT-based SCFPs occur. The FTOHs can be detected in dusts in industrial sites and a thorough review and perspective was provided by Paris-Davila et al.202
3.2 Release to the indoor environment throughout the lifetime of consumer products
Partitioning of FTOHs from consumer products known to contain FT-based SCFPs to indoor air occurred in the orders of hours-to-months depending on the materials and factors, including temperature and light.4,7,33,35,104,203–205 Sinclair et al.4 measured losses of 25–630 pg cm−2 and 220–260 ng of FTOHs following heating of nonstick frying pans between 180 and 230 °C, 20 min and microwaving of popcorn bags for 3 min, respectively. The experiment by Davern et al.104 on microwaved popcorn bags (less than 2 min) corroborated the results from Sinclair et al. through the detection of 480
000 pg m−3 of 6:2 FTOH released to the air.
Fast food packaging was also known to emit FTOHs as indicated by Schwartz-Narbonne et al.33 when an average of 85% decrease in FTOH levels (130–2400 ng) was measured following storage of ∼2 years at room temperature. Textiles were also known to emit FTOHs to the air, including children rain trousers and rain jackets (750–490
000 pg m−3 after 3 h, 25 °C),203 and cotton clothes (210–53
000 pg m−3 after 9 months, 25 °C).35 Emissions of FTOHs from mattress pads and membrane apparel (200–800 ng g−1 loss after 2 h, 40–100 °C),7 from mattress pads and a cleaner product (230 and 12
400 ng g−1 loss after 125 and 28 h, respectively, 25 °C),205 and from firefighter turnout gears (8:2 FTOH detected after 30 min, 200 °C)204 were also known. Products containing FT-based SCFPs were known sources of FTOHs in indoor air.
Levels of FTOHs above the limit of quantitation were reported in the range of 0.04–1
900
000 pg m−3 in indoor air,39,70,87,181,203,206–220 which were two-to-three orders of magnitude lower than the recorded indoor air levels at a Chinese textile factory (Fig. 3). While the partitioning of FTOHs from consumer products to the air was already assessed, the half-lives of FTOHs in indoor air were not studied. Once FTOHs were off-gassed from the material indoors, FTOHs were known to sorb dust in addition to air particles.221,222 Comprehensive reviews and perspectives on FTOHs in house dusts were provided previously by Shoeib et al.,14 Savvaides et al.,20 and Zhu et al.223
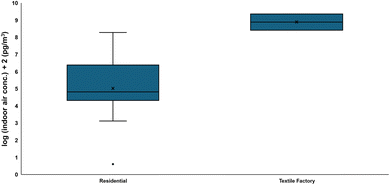 |
| Fig. 3 Box and whisker plot comparison between min and max of reported FTOH indoor air concentration at residential sites and at a textile factory. The indoor air residential data are obtained from ref. 70. | |
3.3 Assessments of human exposures to FTOHs in the indoor environment and the associated potential human health implications
Inhalation is determined to be the major exposure route of FTOHs for humans, therefore, occupational settings where FTOHs are used, such as fluorochemical manufacturing and textile industries,16,182,184,200–202,224,225 are likely to encounter FTOH inhalation exposure.181,202,226–229 However, a significant gap exists with regard to exposure studies on FTOHs at occupational sites, therefore, comparisons with exposure to FTOHs at residential sites still need to be performed. Meanwhile, studies of human exposure from inhalation of 8:2 FTOH in residential indoor air indicate that risk of human exposure to PFOA from inhalation of 8:2 FTOH in air or dust is low.14,87,203,208,211,216,217,230,231 A recent review on human exposure pathways to PFAS exclude FTOH inhalation, indicating the low potency for human health impacts from inhalation of FTOHs.232 Langer et al. and Kim et al. estimate that 5 and 2.5% of 8:2 FTOH are transformed to PFOA through human metabolism, respectively, indicating low 8:2 FTOH contribution toward overall PFOA exposure.87,208 Corroborating results from Shoeib et al.,14 Schlummer et al.,203 Winkens et al.,216 Padilla-Sánchez et al.,217 Xu et al.,230 and Tian et al.231 indicate that the risk of exposure to PFOA following inhalation of 8:2 FTOH in air or dust does not exceed the tolerable daily intake of PFOA (1500 ng per kg bw per day). Poothong et al. further indicate that only 3% of PFOA exposure originates from indirect exposure, including house dust ingestion and indoor air inhalation.233 Shoeib et al. indicate that inhalation of 8:2 FTOH in air is more relevant than ingestion of 8:2 FTOH in house dusts, but the overall exposure is still less than the estimated Canadian adult dietary intake limit of PFOA (70 ng per day).211 Dermal absorption of FTOHs from air is not predicted to be a major route of exposure of FTOHs to humans.234,235
Ingestion of FTOHs can be another route of exposure for humans,13 as the 6:2, 8:2, 10:2, and 12:2 FTOHs can be transferred to real food samples.32,236 The 6:2 FTOH is also predicted to be as toxic as 8:2 FTOH through ingestion.237 However, ingestion is a route of exposure that does not result from partitioning of FTOHs to the indoor environment (e.g., inhalation or dermal absorption from air). Therefore, the partitioning of FTOHs to food samples (i.e., ingestion) is beyond the scope of this study.
Similarly, while 6:2 FTOH can lead to reproductive dysfunction in mice male offsprings238 or abnormal adult anxiety behaviors in Zebrafish (Danio rerio) trans-generationally,239 the route of exposure in these studies is not through partitioning of FTOHs to the indoor or outdoor environment (i.e., directly to embryos and through ingestion, respectively). However, it is noted that in a 6:2 FTOH inhalation study involving rats, the elimination half-lives of 6:2 range from 1 to 15 h and the FTOH metabolites yield is 2% mol.183
4. Release of FTOHs to the outdoor environment
4.1 Release to air during the production of consumer products
Levels of FTOHs released to air collected near industrial sites, namely near textile and carpet manufacturing and treatment,77,102,201,240 and fluorochemical manufacturing facilities,241,242 are two-to-three orders of magnitude and one order of magnitude higher than the levels of FTOHs collected near non-industrial sites,70,75,79–83,86–88,91,93,96,113,114,117,121,207,211,212,243–266 respectively (Fig. 4). Of note, FTOH release from textile and carpet manufacturing and treatment facilities can be one-to-two orders of magnitude higher than the amount emitted from fluorochemical manufacturing facilities.
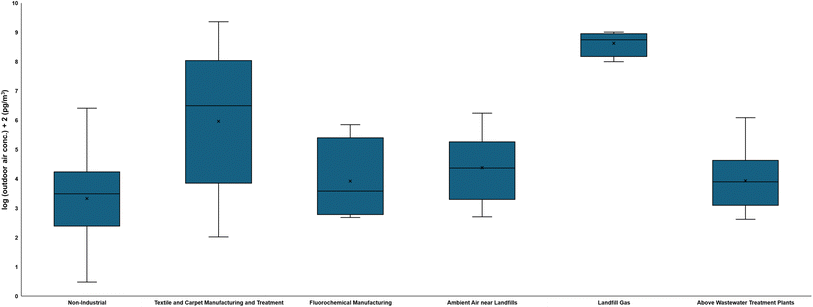 |
| Fig. 4 Box and whisker plot comparison between min and max of reported FTOH outdoor air concentration at different sites. The non-industrial data are obtained from ref. 70. | |
The composition of FTOHs at the global level is still dominated by 8:2 FTOH,100,117 which is attributed to the historical use of C8-based PFAS. At textile and carpet manufacturing and treatment sites, the dominant FTOH in studies before 2020 is 8:2 FTOH,77,201 while the dominant FTOH in studies after 2020 is 6:2 FTOH,102,240 likely reflecting the shift from C8- to C6-based PFAS. Similarly, at fluorochemical manufacturing sites, 8:2 FTOH is the dominant FTOH in the study performed before 2020,241 while 6:2 FTOH is the dominant FTOH in the study performed after 2020.242
Once released, FTOHs undergo atmospheric transformation to PFCAs and there have been efforts to model the contribution of FTOHs to the PFCA level globally. Modeling studies by Wallington et al.108 and Yarwood et al.110 estimated that 1–10% contribution of global PFOA levels are due to atmospheric degradation of 8:2 FTOH based on reported outdoor air concentrations of 8:2 FTOH. Thackray and Selin also performed modeling work based on the presence and subsequent transformation of FTOHs to PFCAs, but their modeling effort predicted 25% contribution of the global level of PFCAs from FTOH atmospheric degradation.120,122
4.2 Release to air at the end-of-life of consumer products
Once used, FT-based SCFP-applied consumer products are disposed to the landfills,267–270 where hydrolysis can occur and degrade FT-based SCFPs to FTOHs,19,27,33,34,36,41,58 followed by the partitioning of FTOHs to the gas-phase.271–273 Release of 8:2 FTOH and its transformation product is documented in a laboratory-scale landfill leachate-sediment microcosm study,162 while Goukeh et al. demonstrate the degradations of FT-based SCFP-applied materials and subsequent volatilization of FTOHs in laboratory-scale landfill-simulated settings.34 Landfill gas (LFG) is known to contain FTOHs (1–10 × 106 pg m−3).34,274 Landfills are thus known to emit FTOHs based on ambient air samples collected near landfills (5–17
000 pg m−3).97,275–277 Levels of FTOHs in ambient air near landfills are comparable to the levels of FTOHs in the air collected outside fluorochemical manufacturing facilities (Fig. 4). The FTOHs found in ambient air near landfills are dominated by 8:2 and 10:2 FTOHs from studies in the 2010s,275,276 but 6:2 FTOH starts to dominate in recent years,97,274,277 which is reflective of the 6:2 FTOH detected in consumer products in recent years.1
The release of FTOHs from landfills indicates that while landfills are a sink of FT-based SCFP-applied consumer products, landfills are a source of FTOHs to the atmosphere as well. Studies by Li et al.,278 Washington et al.279 and van Zelm et al.280 foresee the emission of FTOHs from landfills as a major contributor of PFCAs in the environment through the continual degradation of FT-based SCFPs and the subsequent release of FTOHs, followed by transformation to PFCAs. However, the existing global inventory of PFCAs based on outdoor air concentrations and atmospheric transformation models115,122,281,282 are yet to account for FTOHs released from landfills, which represents a research gap.
4.3 Release of FTOHs to the outdoor environment throughout the lifetime of consumer products
In the case of textiles, myriad processes degrade FT-based SCFPs applied in consumer products, as described by Schellenberger, van der Veen, and colleagues: loss of fibers, increased and/or rough surface area, disruption of non-/covalent bonding between and among monomers (e.g., loss due to laundering and rain, volatilization), and UV-degradation.19,25,55,56,59,283 Schellenberger and van der Veen et al. also assess the release of FTOHs to the outdoor environment throughout the lifetime of consumer products through comparisons of FTOH concentrations pre- and post-washing, weathering (e.g., temperature, humidity, and light), and drying.19,25,56,59 The FTOHs are detected in wastewater treatment plants (WWTPs) not known to be impacted by textile factories,284–291 indicating washing of FT-based SCFP-applied consumer products as a potential source of FTOHs to WWTPs. However, estimate of FTOH release to WWTPs in Europe following washing of FTOH-containing consumer products was minimal (∼0.7 t per year).56
4.4 Release to water during the production of consumer products
While FTOHs are volatile and partition to the gas-phase, FTOHs can be detected in water samples, including those sampled from WWTPs near textile factories and fluorochemical manufacturing facilities.102,240,292–295 Levels of FTOHs in water samples recorded near fluorochemical manufacturing facilities are three-to-six orders of magnitude higher than levels recorded near textile factories (Fig. 5). However, the range of FTOHs in water samples collected from near textile factories (2.1–43 ng L−1)240,294,295 is comparable to the range of FTOHs in water samples collected from rivers and WWTPs not known to be impacted by textile factories (0.003–78 ng L−1) (Fig. 5).284–291 In studies concerning WWTPs impacted by textile factories, FTOHs in the influents are higher than in the effluents, indicating transformation of FTOHs to PFCAs in WWTPs.294,295 In the only published study of its kind, FTOHs are below the limit of detection (<LOD) in groundwater samples collected from an active fluorochemical manufacturing facility.296 More data on FTOHs in any water samples can be useful, but prior data, supported by known partitioning preference of FTOHs to the gas phase and transformation of FTOHs to PFCAs, need to be acknowledged in terms of the potential results of <LODs in future studies.
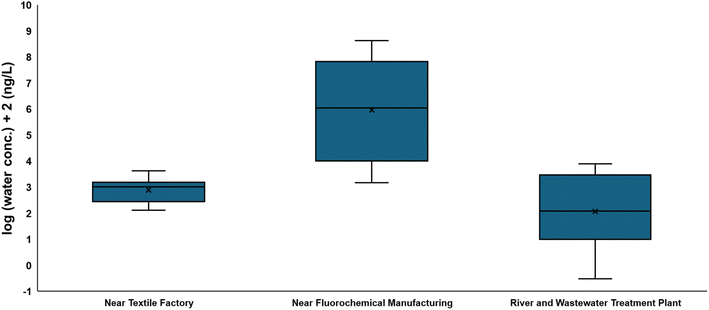 |
| Fig. 5 Box and whisker plot comparison between min and max of reported FTOH water concentration at different sites. | |
4.5 Release to water at the end-of-life of consumer products
Due to disposal of FT-based SCFP-applied consumer products to municipal solid waste,267–270 as well as the volatilization of FTOHs (Fig. 4) and the transformation of FTOHs to PFCAs, release of FTOHs to water at the end-of-life of consumer products is estimated to be insignificant.289,293 In landfills, FTOHs in LFG are known to transform to FTOH degradation products through studies on LFG condensate and landfill leachates.297,298 Of note, 5:3 FTCA is an intermediate transformation product between 6:2 FTOH and PFHxA62 (Fig. 2), and is a major PFAS consistently measured in landfill leachates.299–301
4.6 Release to soil during the production of consumer products
Comparable amount of FTOHs were measured in soil samples collected near fluorochemical manufacturing in the U.S. (60–36
000 and 100–243
000 pg g−1),296,302 China (70–7200 and 2600–50
000 pg g−1),169,241 and Japan (100–12
000 pg g−1).242 The levels of FTOHs in soil near fluorochemical manufacturing facilities were two-to-three orders of magnitude higher than levels of FTOHs collected from roadside in the U.S. (0.51–10 pg g−1).39 No publication on FTOHs in soil samples collected near textile and carpet manufacturing and treatment facilities was located by the author.
4.7 Release to soil at the end-of-life of consumer products
The pathway for FTOHs to be released to soil at the end-of-life of consumer products involves biosolids,303,304 which are collected from WWTPs. Studies by Ellington et al.,133 Yoo et al.,153 and Zhang et al.163 indicate FTOH concentration in the range of 13
000–57
000, 4000–820
000, and 1000–170
000 pg g−1, respectively. The levels of FTOHs in biosolid-amended soil are two-to-five orders of magnitude higher than FTOHs in roadside soil samples (0.5–10 pg g−1).39 A potential source of FTOHs in biosolids is FT-based SCFPs as the estimated degradation half-lives of FT-based SCFPs can vary between 10 and 110 years305–308 or up to a thousand years.309–311 Any FTOHs remaining in the biosolids can breakdown to PFCAs and further transformation products, indicating a potential pathway of PFOA exposure to humans when biosolids are applied to soils for farming.
5. Policy implications of release of FTOHs associated with consumer products
Within the U.S., aside from states' initiatives on consumer products312,313 and the U.S. Food and Drug Administration (FDA)'s announcement of 6:2 FTOH voluntary phase-out from the food contact material (FCM) market,314 there are no regulations on the release of FTOHs to the environment. The phase-out of FTOH-containing FCMs indicates that less FTOH-containing FCMs will end up in landfills, which will also lead to the reduction of FTOHs and PFCAs released to the environment. The phase-out of FTOH-containing textile315 likely also leads to less FTOHs and PFCAs in the environment, but regulations on active and closed textile mills are still needed due to the potential formation of PFCAs downstream.316
The lack of existing regulations on FTOHs may be indicative of legislative and general public understanding and approaches toward PFAS that are still limited to ionic PFAS including, PFOA, perfluorooctane sulfonate, and their PFAS-replacements, such as hexafluoropropylene oxide-dimer acid.317–320 Lawmakers can thus consider tying the transformation of FTOHs released from FT-based SCFPs in landfills to the presence of PFCAs in the atmosphere within existing regulations as the foundation for any policies regarding FTOH use in consumer products.
An approach where entities that emit FTOHs are capped at certain levels can be considered. This approach can be relevant for landfill operators because FTOHs are estimated to continue to be emitted from landfills to the atmosphere due to past and current FT-based SCFP use.56,278,279,321,322 However, the proportion of FTOHs released to the gas-phase due to hydrolysis of FT-based SCFP-applied products in landfills is yet to be studied. Therefore, there are likely to be pushbacks from landfill operators on any proposed regulations of FTOHs. For comparison, hydrolysis experiments performed in laboratory settings indicate an increase in FTOH concentrations in FT-based SFCP-applied consumer products up to 1500× pre- and post-hydrolysis.19,27,33,36,58
Policies discouraging the use of FTOHs can also be considered as alternatives to FTOHs are available, such as silicone-based polymers, wax, hyperbranched polymers, silicone-based PFAS, and melamin.323–326 However, the environmental impacts of alternatives to FTOHs are unclear, though the presence of silicone-based PFAS monomers in soil and earthworms is known.326,327
Future policies can also promote treatment efforts known to mitigate the release of FTOHs to the environment. Researchers from the U.S. EPA indicate that thermal treatments containing calcium oxide and alumina-based catalysts can be useful to treat FTOHs through mineralization.328,329 Calcium oxides are distributed in a flameless tube furnace operated between 400 and 800 °C as gas-phase FTOHs are flowing, and degradation efficiency of FTOHs following experiments achieves 85–99% removal of FTOHs.328 Comparable degradation efficiency can also be achieved with the use of alumina catalysts at a lower temperature range of 200–500 °C.329 If these techniques can be recreated and applied on a large scale, such as in landfills where gas-phase FTOHs are detected, then the potential for FTOHs to transform into PFCAs in the environment can be inhibited.
Data availability
No new data were generated as part of this Perspective.
Conflicts of interest
The author declares no competing interest. No artificial intelligence330 was used in this work. The Table of Contents (TOC) art was created with https://www.BioRender.com.
Acknowledgements
This work was made possible in part by Grant BAA-0004-21 acquired by Dr Jennifer Field. Its contents are solely the responsibility of the author and do not necessarily represent the official view of the U.S. Defense Logistics Agency, of the U.S. Department of Defense, or of Dr Jennifer Field. Oregon State University in Corvallis, Oregon, is located within the traditional homelands of the Mary's River or Ampinefu Band of Kalapuya. Following the Willamette Valley Treaty of 1855, Kalapuya people were forcibly removed to reservations in Western Oregon. Today, living descendants of these people are a part of the Confederated Tribes of Grand Ronde Community of Oregon (https://www.grandronde.org) and the Confederated Tribes of the Siletz Indians (https://www.ctsi.nsn.us).
References
- P. Dewapriya, L. Chadwick, S. G. Gorji, B. Schulze, S. Valsecchi, S. Samanipour, K. V. Thomas and S. L. Kaserzon, Per- and polyfluoroalkyl substances (PFAS) in consumer products: current knowledge and research gaps, J. Hazard. Mater. Lett., 2023, 4, 100086 CrossRef CAS.
- M. J. A. Dinglasan-Panlilio and S. A. Mabury, Significant Residual Fluorinated Alcohols Present in Various Fluorinated Materials, Environ. Sci. Technol., 2006, 40, 1447–1453 CrossRef CAS PubMed.
- B. S. Larsen, P. Stchur, B. Szostek, S. F. Bachmura, R. C. Rowand, K. B. Prickett, S. H. Korzeniowski and R. C. Buck, Method development for the determination of residual fluorotelomer raw materials and perflurooctanoate in fluorotelomer-based products by gas chromatography and liquid chromatography mass spectrometry, J. Chromatogr. A, 2006, 1110, 117–124 CrossRef CAS PubMed.
- E. Sinclair, S. K. Kim, H. B. Akinleye and K. Kannan, Quantitation of Gas-Phase Perfluoroalkyl Surfactants and Fluorotelomer Alcohols Released from Nonstick Cookware and Microwave Popcorn Bags, Environ. Sci. Technol., 2007, 41, 1180–1185 CrossRef CAS PubMed.
- S. Fiedler, G. Pfister and K.-W. Schramm, Poly- and perfluorinated compounds in household consumer products, Toxicol. Environ. Chem., 2010, 92, 1801–1811 CrossRef CAS.
- D. Herzke, E. Olsson and S. Posner, Perfluoroalkyl and polyfluoroalkyl substances (PFASs) in consumer products in Norway – A pilot study, Chemosphere, 2012, 88, 980–987 CrossRef CAS PubMed.
- X. Liu, Z. Guo, E. E. Folk and N. F. Roache, Determination of fluorotelomer alcohols in selected consumer products and preliminary investigation of their fate in the indoor environment, Chemosphere, 2015, 129, 81–86 CrossRef CAS PubMed.
- F. Ye, Y. Zushi and S. Masunaga, Survey of perfluoroalkyl acids (PFAAs) and their precursors present in Japanese consumer products, Chemosphere, 2015, 127, 262–268 CrossRef CAS PubMed.
- R. Vestergren, D. Herzke, T. Wang and I. T. Cousins, Are imported consumer products an important diffuse source of PFASs to the Norwegian environment?, Environ. Pollut., 2015, 198, 223–230 CrossRef CAS PubMed.
- M. Kotthoff, J. Müller, H. Jürling, M. Schlummer and D. Fiedler, Perfluoroalkyl and polyfluoroalkyl substances in consumer products, Environ. Sci. Pollut. Res., 2015, 22, 14546–14559 CrossRef CAS PubMed.
- P. A. Rice, C6-Perfluorinated Compounds: The New Greaseproofing Agents in Food Packaging, Curr. Environ. Health Rep., 2015, 2, 33–40 CrossRef CAS PubMed.
- C. Gremmel, T. Frömel and T. P. Knepper, Systematic determination of perfluoroalkyl and polyfluoroalkyl substances (PFASs) in outdoor jackets, Chemosphere, 2016, 160, 173–180 CrossRef CAS PubMed.
- G. Yuan, H. Peng, C. Huang and J. Hu, Ubiquitous Occurrence of Fluorotelomer Alcohols in Eco-Friendly Paper-Made Food-Contact Materials and Their Implication for Human Exposure, Environ. Sci. Technol., 2016, 50, 942–950 CrossRef CAS PubMed.
- T. Shoeib, Y. Hassan, C. Rauert and T. Harner, Poly- and perfluoroalkyl substances (PFASs) in indoor dust and food packaging materials in Egypt: Trends in developed and developing countries, Chemosphere, 2016, 144, 1573–1581 CrossRef CAS PubMed.
- A. E. Robel, K. Marshall, M. Dickinson, D. Lunderberg, C. Butt, G. Peaslee, H. M. Stapleton and J. A. Field, Closing the Mass Balance on Fluorine on Papers and Textiles, Environ. Sci. Technol., 2017, 51, 9022–9032 CrossRef CAS PubMed.
- P. T. J. Scheepers, L. Masen-Poos, F. G. B. G. J. van Rooy, A. Oerlemans, E. van Daalen, R. Cremers, H. Lichtenbeld, B. Biesma, J. B. Sørli, I. K. Koponen, S. T. Larsen, P. Wolkoff and A. W. Nørgaard, Pulmonary injury associated with spray of a water-based nano-sized waterproofing product: a case study, J. Occup. Med. Toxicol., 2017, 12, 1–15 CrossRef PubMed.
- J. N. Rewerts, J. T. Morré, S. L. Massey Simonich and J. A. Field, In-Vial Extraction Large Volume Gas Chromatography Mass Spectrometry for Analysis of Volatile PFASs on Papers and Textiles, Environ. Sci. Technol., 2018, 52, 10609–10616 CrossRef CAS PubMed.
- Y. Wu, G. Z. Miller, J. Gearhart, G. Peaslee and M. Venier, Side-chain Fluorotelomer based Polymers in Children Car Seats, Environ. Pollut., 2020, 268(Part B), 115477 Search PubMed.
- I. van der Veen, A.-C. Hanning, A. Stare, P. E. G. Leonards, J. de Boer and J. M. Weiss, The effect of weathering on per- and polyfluoroalkyl substances (PFASs) from durable water repellent (DWR) clothing, Chemosphere, 2020, 249, 126100 CrossRef CAS PubMed.
- T. Savvaides, J. P. Koelmel, Y. Zhou, E. Z. Lin, P. Stelben, J. J. Aristizabal-Henao, J. A. Bowden and K. J. Godri Pollitt, Prevalence and Implications of Per- and Polyfluoroalkyl Substances (PFAS) in Settled Dust, Curr. Environ. Health Rep., 2021, 8, 323–335 CrossRef CAS PubMed.
- H. D. Whitehead, M. Venier, Y. Wu, E. Eastman, S. Urbanik, M. L. Diamond, A. Shalin, H. Schwartz-Narbonne, T. A. Bruton, A. Blum, Z. Wang, M. Green, M. Tighe, J. T. Wilkinson, S. McGuinness and G. F. Peaslee, Fluorinated Compounds in North American Cosmetics, Environ. Sci. Technol. Lett., 2021, 8, 538–544 CrossRef CAS.
- R. C. Buck, S. H. Korzeniowski, E. Laganis and F. Adamsky, Identification and classification of commercially relevant per- and poly-fluoroalkyl substances (PFAS), Integr. Environ. Assess. Manage., 2021, 17, 1045–1055 CrossRef CAS PubMed.
- C. Wang, H. Zhang, L. Zhu, W. Hu and Z. Lin, Simultaneous determination of 11 volatile perfluorinated compound precursors in textiles using gas chromatography-triple quadrupole mass spectrometry, SePu Chin. J. Chromatogr., 2021, 39, 1239–1246 CrossRef CAS PubMed.
- D. J. Muensterman, I. A. Titaley, G. F. Peaslee, L. D. Minc, L. Cahuas, A. E. Rodowa, Y. Horiuchi, S. Yamane, T. N. J. Fouquet, J. C. Kissel, C. C. Carignan and J. A. Field, Disposition of Fluorine on New Firefighter Turnout Gear, Environ. Sci. Technol., 2022, 56, 974–983 CrossRef CAS PubMed.
- I. van der Veen, S. Schellenberger, A.-C. Hanning, A. Stare, J. de Boer, J. M. Weiss and P. E. G. Leonards, Fate of Per- and Polyfluoroalkyl Substances from Durable Water-Repellent Clothing during Use, Environ. Sci. Technol., 2022, 56, 5886–5897 CrossRef CAS PubMed.
- L. Cahuas, D. J. Muensterman, M. L. Kim-Fu, P. N. Reardon, I. A. Titaley and J. A. Field, Paints: A Source of Volatile PFAS in Air–Potential Implications for Inhalation Exposure, Environ. Sci. Technol., 2022, 56, 17070–17079 CrossRef CAS PubMed.
- C. Xia, M. L. Diamond, G. F. Peaslee, H. Peng, A. Blum, Z. Wang, A. Shalin, H. D. Whitehead, M. Green, H. Schwartz-Narbonne, D. Yang and M. Venier, Per- and Polyfluoroalkyl Substances in North American School Uniforms, Environ. Sci. Technol., 2022, 56, 13845–13857 CrossRef CAS PubMed.
- N. J. Herkert, C. D. Kassotis, S. Zhang, Y. Han, V. F. Pulikkal, M. Sun, P. L. Ferguson and H. M. Stapleton, Characterization of Per- and Polyfluorinated Alkyl Substances Present in Commercial Anti-fog Products and Their In Vitro Adipogenic Activity, Environ. Sci. Technol., 2022, 56, 1162–1173 CrossRef CAS PubMed.
- P. Siao, S.-H. Tseng and C.-Y. Chen, Determination of perfluoroalkyl substances in food packaging in Taiwan using ultrasonic extraction and ultra-performance liquid chromatography/tandem mass spectrometry, J. Food Drug Anal., 2022, 30, 11–25 CrossRef PubMed.
- L. Minet, Z. Wang, A. Shalin, T. A. Bruton, A. Blum, G. F. Peaslee, H. Schwartz-Narbonne, M. Venier, H. Whitehead, Y. Wu and M. L. Diamond, Use and release of per- and polyfluoroalkyl substances (PFASs) in consumer food packaging in U.S. and Canada, Environ. Sci.: Processes Impacts, 2022, 24, 2032–2042 RSC.
- R. Seró, J. F. Ayala-Cabrera, F. J. Santos and E. Moyano, Paper spray-atmospheric pressure photoionization-high resolution mass spectrometry for the direct analysis of neutral fluorinated compounds in waterproof impregnation sprays, Anal. Chim. Acta, 2022, 1204, 339720 CrossRef PubMed.
- M. Lerch, K. H. Nguyen and K. Granby, Is the use of paper food contact materials treated with per- and polyfluorinated alkyl substances safe for high-temperature applications? – Migration study in real food and food simulants, Food Chem., 2022, 393, 133375 CrossRef CAS PubMed.
- H. Schwartz-Narbonne, C. Xia, A. Shalin, H. D. Whitehead, D. Yang, G. F. Peaslee, Z. Wang, Y. Wu, H. Peng, A. Blum, M. Venier and M. L. Diamond, Per- and Polyfluoroalkyl Substances in Canadian Fast Food Packaging, Environ. Sci. Technol. Lett., 2023, 10, 343–349 CrossRef CAS PubMed.
- M. N. Goukeh, T. Abichou and Y. Tang, Measurement of fluorotelomer alcohols based on solid phase microextraction followed by gas chromatography-mass spectrometry and its application in solid waste study, Chemosphere, 2023, 345, 140460 CrossRef CAS PubMed.
- C. M. A. Eichler, N. Y. Chang, E. A. Cohen Hubal, D. E. Amparo, J. Zhou, J. D. Surratt, G. C. Morrison and B. J. Turpin, Cloth–Air Partitioning of Neutral Per- and Polyfluoroalkyl Substances (PFAS) in North Carolina Homes during the Indoor PFAS Assessment (IPA) Campaign, Environ. Sci. Technol., 2023, 57, 15173–15183 CrossRef CAS PubMed.
- J. Zweigle, C. Capitain, F. M. Simon, P. Roesch, B. Bugsel and C. Zwiener, Non-extractable PFAS in functional textiles – Characterization by complementary methods: oxidation, hydrolysis, and fluorine sum parameters, Environ. Sci.: Processes Impacts, 2023, 25, 1298–1310 RSC.
- P. Zuccaro, J. Licato, E. A. Davidson, D. C. Thompson and V. Vasiliou, Assessing extraction-analysis methodology to detect fluorotelomer alcohols (FTOH), a class of perfluoroalkyl and polyfluoroalkyl substances (PFAS), in artificial turf fibers and crumb rubber infill, Case Stud. Chem. Environ. Eng., 2023, 7, 100280 CrossRef CAS PubMed.
- P. Roesch, A. Schinnen, M. Riedel, T. Sommerfeld, G. Sawal, N. Bandow, C. Vogel, U. Kalbe and F.-G. Simon, Investigation of pH-dependent extraction methods for PFAS in (fluoropolymer-based) consumer products: A comparative study between targeted and sum parameter analysis, Chemosphere, 2024, 351, 141200 CrossRef CAS PubMed.
- W. Li and K. Kannan, A Simple and Sensitive Method for Simultaneous Analysis of 58 Neutral and Ionic PFAS Using UPLC-MS/MS, Environ. Sci. Technol. Lett., 2024, 11, 308–314 CrossRef CAS.
- P. Vázquez Loureiro, K.-H. Nguyen, A. Rodríguez Bernaldo de Quirós, R. Sendón, K. Granby and A. A. Niklas, Identification and quantification of per- and polyfluorinated alkyl substances (PFAS) migrating from food contact materials (FCM), Chemosphere, 2024, 360, 142360 CrossRef PubMed.
- H. Matsukami, J. Saito, Q. Wang and Y. Miyake, Impact of tightening environmental regulations against long-chain perfluoroalkyl acids on composition of durable water repellents containing side-chain fluorinated polymers, Sci. Total Environ., 2024, 173708 CrossRef CAS PubMed.
- K. Bouma, D. Kalsbeek-van Wijk, L. Steendam, D. T. H. M. Sijm, T. de Rijk, R. Kause, R. Hoogenboom and S. van Leeuwen, Plant-based food contact materials: presence of hazardous substances, Food Addit. Contam.: Part A, 2024, 1–10 Search PubMed.
-
E. Kissa, Fluorinated Surfactants and Repellents, CRC Press, 2nd edn, 2001 Search PubMed.
-
OECD, Synthesis Report on Understanding Side-Chain Fluorinated Polymers and Their Life Cycle, Organisation for Economic Co-operation and Development (OECD), Paris, 2022 Search PubMed.
- H. W. Fox and W. A. Zisman, The spreading of liquids on low energy surfaces. I. Polytetrafluoroethylene, J. Colloid Sci., 1950, 5, 514–531 CrossRef CAS.
- M. Yamauchi, T. Hirono, S. Kodama and M. Mastuo, The evaluation of new fluoropolymer emulsion for exterior paint use, Surf. Coat. Int. JOCCA J. Oil Colour Chem. Assoc., 1996, 79, 312–318 CAS.
- J. Gardiner, Fluoropolymers: Origin, Production, and Industrial and Commercial Applications, Aust. J. Chem., 2014, 68, 13–22 CrossRef.
-
A. R. Bock and B. E. Laird, in Perfluoroalkyl Substances Synthesis, Applications, Challenges and Regulations, ed. B. Améduri, Royal Society of Chemistry, 2022, pp. 1–21 Search PubMed.
- F. Audenaert, H. Lens, D. Rolly and P. Vander Elst, Fluorochemical Textile Repellents—Synthesis and Applications: A 3M Perspective, J. Text. Inst., 1999, 90, 76–94 CrossRef.
- V. Castelvetro, M. Aglietto, F. Ciardelli, O. Chiantore, M. Lazzari and L. Toniolo, Structure control, coating properties, and durability of fluorinated acrylic-based polymers, J. Coat. Technol., 2002, 74, 57–66 CrossRef CAS.
-
3M Company, Fluorochemical Use, Distribution and Release Overview, 1999 Search PubMed.
- S. Chu and R. J. Letcher, Side-chain fluorinated polymer surfactants in aquatic sediment and biosolid-augmented agricultural soil from the Great Lakes basin of North America, Sci. Total Environ., 2017, 607–608, 262–270 CrossRef CAS PubMed.
-
B. Bokkers, B. van de Ven, P. Janssen, W. Bil, F. van Broekhuizen, M. Zeilmaker and A. G. Oomen, Per- and Polyfluoroalkyl Substances (PFASs) in Food Contact Materials, Rijksinstituut voor Volksgezondheid en Milieu RIVM, 2019 Search PubMed.
- K. Rankin and S. A. Mabury, Matrix Normalized MALDI-TOF Quantification of a Fluorotelomer-Based Acrylate Polymer, Environ. Sci. Technol., 2015, 49, 6093–6101 CrossRef CAS PubMed.
- H. Holmquist, S. Schellenberger, I. van der Veen, G. M. Peters, P. E. G. Leonards and I. T. Cousins, Properties, performance and associated hazards of state-of-the-art durable water repellent (DWR) chemistry for textile finishing, Environ. Int., 2016, 91, 251–264 CrossRef CAS PubMed.
- S. Schellenberger, C. Jönsson, P. Mellin, O. A. Levenstam, I. Liagkouridis, A. Ribbenstedt, A.-C. Hanning, L. Schultes, M. M. Plassmann, C. Persson, I. T. Cousins and J. P. Benskin, Release of Side-Chain Fluorinated Polymer-Containing Microplastic Fibers from Functional Textiles During Washing and First Estimates of Perfluoroalkyl Acid Emissions, Environ. Sci. Technol., 2019, 53, 14329–14338 CrossRef CAS PubMed.
- I. Liagkouridis, R. Awad, S. Schellenberger, M. M. Plassmann, I. T. Cousins and J. P. Benskin, Combined Use of Total Fluorine and Oxidative Fingerprinting for Quantitative Determination of Side-Chain Fluorinated Polymers in Textiles, Environ. Sci. Technol. Lett., 2021, 9, 30–36 CrossRef.
- V. A. Nikiforov, Hydrolysis of FTOH precursors, a simple method to account for some of the unknown PFAS, Chemosphere, 2021, 276, 130044 CrossRef CAS PubMed.
- S. Schellenberger, I. Liagkouridis, R. Awad, S. Khan, M. Plassmann, G. Peters, J. P. Benskin and I. T. Cousins, An Outdoor Aging Study to Investigate the Release of Per- And Polyfluoroalkyl Substances (PFAS) from Functional Textiles, Environ. Sci. Technol., 2022, 56, 3471–3479 CrossRef CAS PubMed.
- P. Roesch, C. Vogel, P. Wittwer, T. Huthwelker, C. Borca, T. Sommerfeld, S. Kluge, C. Piechotta, U. Kalbe and F.-G. Simon, Taking a Look at the Surface: μ-XRF Mapping and Fluorine K-edge μ-XANES Spectroscopy of Organofluorinated Compounds in Environmental Samples and Consumer Products, Environ. Sci.: Processes Impacts, 2023, 25, 1213–1223 RSC.
- R. Lohmann and R. J. Letcher, The universe of fluorinated polymers and polymeric substances and potential environmental impacts and concerns, Curr. Opin. Green Sustainable Chem., 2023, 41, 100795 CrossRef CAS PubMed.
- M. G. Evich, M. J. B. Davis, J. P. McCord, B. Acrey, J. A. Awkerman, D. R. U. Knappe, A. B. Lindstrom, T. F. Speth, C. Tebes-Stevens, M. J. Strynar, Z. Wang, E. J. Weber, W. M. Henderson and J. W. Washington, Per- and polyfluoroalkyl substances in the environment, Science, 2022, 375, eabg9065 CrossRef CAS PubMed.
- J. Hammer and S. Endo, Volatility and Nonspecific van der Waals Interaction Properties of Per- and Polyfluoroalkyl Substances (PFAS): Evaluation Using Hexadecane/Air Partition Coefficients, Environ. Sci. Technol., 2022, 56, 15737–15745 CrossRef CAS PubMed.
- S. Endo, J. Hammer and S. Matsuzawa, Experimental Determination of Air/Water Partition Coefficients for 21 Per- and Polyfluoroalkyl Substances Reveals Variable Performance of Property Prediction Models, Environ. Sci. Technol., 2023, 57, 8406–8413 CrossRef CAS PubMed.
- S. Endo, Intermolecular Interactions, Solute Descriptors, and Partition Properties of Neutral Per- and Polyfluoroalkyl Substances (PFAS), Environ. Sci. Technol., 2023, 57, 17534–17541 CrossRef CAS PubMed.
- A. Dreyer, V. Langer and R. Ebinghaus, Determination of Octanol–Air Partition Coefficients (KOA) of Fluorotelomer Acrylates, Perfluoroalkyl Sulfonamids, and Perfluoroalkylsulfonamido Ethanols, J. Chem. Eng. Data, 2009, 54, 3022–3025 CrossRef CAS.
- Y. D. Lei, F. Wania, D. Mathers and S. A. Mabury, Determination of Vapor Pressures, Octanol–Air, and Water–Air Partition Coefficients for Polyfluorinated Sulfonamide, Sulfonamidoethanols, and Telomer Alcohols, J. Chem. Eng. Data, 2004, 49, 1013–1022 CrossRef CAS.
- I. Abusallout, C. Holton, J. Wang and D. Hanigan, Henry's Law Constants of 15 Per- and Polyfluoroalkyl Substances Determined by Static Headspace Analysis, J. Hazard. Mater. Lett., 2022, 3, 100070 CrossRef CAS.
- J. Murillo-Gelvez, O. Dmitrenko, T. L. Torralba-Sanchez, P. G. Tratnyek and D. M. D. Toro, pKa prediction of per- and polyfluoroalkyl acids in water using in silico gas phase stretching vibrational frequencies and infrared intensities, Phys. Chem. Chem. Phys., 2023, 25, 24745–24760 RSC.
- M. A. G. Wallace, M. G. Smeltz, J. M. Mattila, H. K. Liberatore, S. R. Jackson, E. P. Shields, X. Xhani, E. Y. Li and J. H. Johansson, A Review of Sample Collection and Analytical Methods for Detecting Per- and Polyfluoroalkyl Substances in Indoor and Outdoor Air, Chemosphere, 2024, 358, 142129 CrossRef CAS PubMed.
- W. Qin, B. I. Escher, J. Huchthausen, Q. Fu and L. Henneberger, Species Difference? Bovine, Trout, and Human Plasma Protein Binding of Per- and Polyfluoroalkyl Substances, Environ. Sci. Technol., 2024, 58, 9954–9966 CrossRef CAS PubMed.
- L. Ahrens, T. Harner, M. Shoeib, D. A. Lane and J. G. Murphy, Improved Characterization of Gas–Particle Partitioning for Per- and Polyfluoroalkyl Substances in the Atmosphere Using Annular Diffusion Denuder Samplers, Environ. Sci. Technol., 2012, 46, 7199–7206 CrossRef CAS PubMed.
- L. J. Musegades, O. P. Curtin and J. D. Cyran, Determining the Surface pKa of Perfluorooctanoic Acid, J. Phys. Chem. C, 2024, 128, 1946–1951 CrossRef CAS PubMed.
- R. Patel, L. E. Saab, P. J. Brahana, K. T. Valsaraj and B. Bharti, Interfacial Activity and Surface pKa of Perfluoroalkyl Carboxylic Acids (PFCAs), Langmuir, 2024, 40, 3651–3658 CAS.
- A. Dreyer and R. Ebinghaus, Polyfluorinated compounds in ambient air from ship- and land-based measurements in northern Germany, Atmos. Environ., 2009, 43, 1527–1535 CrossRef CAS.
- D. A. Ellis, J. W. Martin, S. A. Mabury, M. D. Hurley, M. P. Sulbaek Andersen and T. J. Wallington, Atmospheric Lifetime of Fluorotelomer Alcohols, Environ. Sci. Technol., 2003, 37, 3816–3820 CrossRef CAS PubMed.
- N. L. Stock, F. K. Lau, D. A. Ellis, J. W. Martin, D. C. G. Muir and S. A. Mabury, Polyfluorinated Telomer Alcohols and Sulfonamides in the North American Troposphere, Environ. Sci. Technol., 2004, 38, 991–996 CrossRef CAS PubMed.
- D. P. Cobranchi, M. Botelho, L. W. Buxton, R. C. Buck and M. A. Kaiser, Vapor pressure determinations of 8-2 fluorortelomer alcohol and 1-H perfluorooctane by capillary gas chromatography: Relative retention time versus headspace methods, J. Chromatogr. A, 2006, 1108, 248–251 CrossRef CAS PubMed.
- M. Shoeib, T. Harner and P. Vlahos, Perfluorinated Chemicals in the Arctic Atmosphere, Environ. Sci. Technol., 2006, 40, 7577–7583 CrossRef CAS PubMed.
- N. L. Stock, V. I. Furdui, D. C. G. Muir and S. A. Mabury, Perfluoroalkyl Contaminants in the Canadian Arctic: Evidence of Atmospheric Transport and Local Contamination, Environ. Sci. Technol., 2007, 41, 3529–3536 CrossRef CAS PubMed.
- S. Oono, K. H. Harada, M. A. M. Mahmoud, K. Inoue and A. Koizumi, Current levels of airborne polyfluorinated telomers in Japan, Chemosphere, 2008, 73, 932–937 CrossRef CAS PubMed.
- S. Oono, E. Matsubara, K. H. Harada, S. Takagi, S. Hamada, A. Asakawa, K. Inoue, I. Watanabe and A. Koizumi, Survey of Airborne Polyfluorinated Telomers in Keihan Area, Japan, Bull. Environ. Contam. Toxicol., 2008, 80, 102–106 CrossRef CAS PubMed.
- A. Dreyer, I. Weinberg, C. Temme and R. Ebinghaus, Polyfluorinated Compounds in the Atmosphere of the Atlantic and Southern Oceans: Evidence for a Global Distribution, Environ. Sci. Technol., 2009, 43, 6507–6514 CrossRef CAS PubMed.
- A. Dreyer, M. Shoeib, S. Fiedler, J. Barber, T. Harner, K.-W. Schramm, K. C. Jones and R. Ebinghaus, Field intercomparison on the determination of volatile and semivolatile polyfluorinated compounds in air, Environ. Chem., 2010, 7, 350–358 CrossRef CAS.
- L. Vierke, L. Ahrens, M. Shoeib, E. J. Reiner, R. Guo, W.-U. Palm, R. Ebinghaus and T. Harner, Air concentrations and particle–gas partitioning of polyfluoroalkyl compounds at a wastewater treatment plant, Environ. Chem., 2011, 8, 363–371 CrossRef CAS.
- M. Cai, Z. Xie, A. Möller, Z. Yin, P. Huang, M. Cai, H. Yang, R. Sturm, J. He and R. Ebinghaus, Polyfluorinated compounds in the atmosphere along a cruise pathway from the Japan Sea to the Arctic Ocean, Chemosphere, 2012, 87, 989–997 CrossRef CAS PubMed.
- S.-K. Kim, M. Shoeib, K.-S. Kim and J.-E. Park, Indoor and outdoor poly- and perfluoroalkyl substances (PFASs) in Korea determined by passive air sampler, Environ. Pollut., 2012, 162, 144–150 CrossRef CAS PubMed.
- Z. Xie, Z. Zhao, A. Möller, H. Wolschke, L. Ahrens, R. Sturm and R. Ebinghaus, Neutral poly- and perfluoroalkyl substances in air and seawater of the North Sea, Environ. Sci. Pollut. Res., 2013, 20, 7988–8000 CrossRef CAS PubMed.
- Z. Xie, Z. Wang, W. Mi, A. Möller, H. Wolschke and R. Ebinghaus, Neutral Poly-/perfluoroalkyl Substances in Air and Snow from the Arctic, Sci. Rep., 2015, 5, 8912 CrossRef CAS PubMed.
- Z. Wang, Z. Xie, A. Möller, W. Mi, H. Wolschke and R. Ebinghaus, Estimating dry deposition and gas/particle partition coefficients of neutral poly-/perfluoroalkyl substances in northern German coast, Environ. Pollut., 2015, 202, 120–125 CrossRef CAS PubMed.
- Z. Wang, Z. Xie, W. Mi, A. Möller, H. Wolschke and R. Ebinghaus, Neutral Poly/Per-Fluoroalkyl Substances in Air from the Atlantic to the Southern Ocean and in Antarctic Snow, Environ. Sci. Technol., 2015, 49, 7770–7775 CrossRef CAS PubMed.
- Z. Zhao, J. Tang, L. Mi, C. Tian, G. Zhong, G. Zhang, S. Wang, Q. Li, R. Ebinghaus, Z. Xie and H. Sun, Perfluoroalkyl and polyfluoroalkyl substances in the lower atmosphere and surface waters of the Chinese Bohai Sea, Yellow Sea, and Yangtze River estuary, Sci. Total Environ., 2017, 599–600, 114–123 CrossRef CAS PubMed.
- X. Fang, Q. Wang, Z. Zhao, J. Tang, C. Tian, Y. Yao, J. Yu and H. Sun, Distribution and dry deposition of alternative and legacy perfluoroalkyl and polyfluoroalkyl substances in the air above the Bohai and Yellow Seas, China, Atmos. Environ., 2018, 192, 128–135 CrossRef CAS.
- T. P. Riedel, J. R. Lang, M. J. Strynar, A. B. Lindstrom and J. H. Offenberg, Gas-Phase Detection of Fluorotelomer Alcohols and Other Oxygenated Per- and Polyfluoroalkyl Substances by Chemical Ionization Mass Spectrometry, Environ. Sci. Technol. Lett., 2019, 6, 289–293 CrossRef CAS PubMed.
- Q. Wang, Z. Zhao, Y. Ruan, X. Hua, H. Chen, Y. Wang, L. Jin, M. M. P. Tsui, Y. Yao, P. K. S. Lam and H. Sun, Occurrence and seasonal distribution of legacy and emerging per- and polyfluoroalkyl substances (PFASs) in different environmental compartments from areas around ski resorts in northern China, J. Hazard. Mater., 2021, 407, 124400 CrossRef CAS PubMed.
- E. Yamazaki, S. Taniyasu, X. Wang and N. Yamashita, Per- and polyfluoroalkyl substances in surface water, gas and particle in open ocean and coastal environment, Chemosphere, 2021, 272, 129869 CrossRef CAS PubMed.
- H. Lin, J.-Y. Lao, Q. Wang, Y. Ruan, Y. He, P. K. H. Lee, K. M. Y. Leung and P. K. S. Lam, Per- and polyfluoroalkyl substances in the atmosphere of waste management infrastructures: Uncovering secondary fluorotelomer alcohols, particle size distribution, and human inhalation exposure, Environ. Int., 2022, 167, 107434 CrossRef CAS PubMed.
- S. Wang, X. Lin, Q. Li, C. Liu, Y. Li and X. Wang, Neutral and ionizable per-and polyfluoroalkyl substances in the urban atmosphere: Occurrence, sources and transport, Sci. Total Environ., 2022, 823, 153794 CrossRef CAS PubMed.
- P. Shen, X. Song, N. Li and C. Zhao, Concentrations and distributions of fluorotelomer alcohols and perfluoroalkane sulfonamido substances in the atmosphere in the Pearl River Delta, China, J. Environ. Sci. Health, Part A: Toxic/Hazard. Subst. Environ. Eng., 2023, 58, 183–190 CrossRef CAS PubMed.
- A. Saini, S. Chinnadurai, J. K. Schuster, A. Eng and T. Harner, Per- and polyfluoroalkyl substances and volatile methyl siloxanes in global air: Spatial and temporal trends, Environ. Pollut., 2023, 323, 121291 CrossRef CAS PubMed.
- B. Qiao, D. Song, B. Fang, H. Yu, X. Li, L. Zhao, Y. Yao, L. Zhu, H. Chen and H. Sun, Nontarget Screening and Fate of Emerging Per- and Polyfluoroalkyl Substances in Wastewater Treatment Plants in Tianjin, China, Environ. Sci. Technol., 2023, 57, 20127–20137 CrossRef CAS PubMed.
- S. Mok, S. Lee, Y. Choi, J. Jeon, Y. Hee Kim and H.-B. Moon, Target and non-target analyses of neutral per- and polyfluoroalkyl substances from fluorochemical industries using GC-MS/MS and GC-TOF: Insights on their environmental fate, Environ. Int., 2023, 182, 108311 CrossRef CAS PubMed.
- J. Liu, L. Dong, L. Zhou, W. Yang, S. Shi, S. Dong, H. Zhang, X. Zhang, J. Guo and L. Zhang, Tree barks for retrospective measurement and source appointment of airborne perfluoroalkyl and polyfluoroalkyl substances, Environ. Pollut., 2024, 344, 123346 CrossRef CAS PubMed.
- M. J. Davern, G. V. West, C. M. A. Eichler, B. J. Turpin, Y. Zhang and J. D. Surratt, External Liquid Calibration Method for Iodide Chemical Ionization Mass Spectrometry Enables Quantification of Gas-Phase Per- and Polyfluoroalkyl Substances (PFAS) Dynamics in Indoor Air, Analyst, 2024, 149, 3405–3415 RSC.
- L. Ahrens, M. Shoeib, T. Harner, D. A. Lane, R. Guo and E. J. Reiner, Comparison of Annular Diffusion Denuder and High Volume Air Samplers for Measuring Per- and Polyfluoroalkyl Substances in the Atmosphere, Anal. Chem., 2011, 83, 9622–9628 CrossRef CAS PubMed.
- E. J. Weber, C. Tebes-Stevens, J. W. Washington and R. Gladstone, Development of a PFAS reaction library: identifying plausible transformation pathways in environmental and biological systems, Environ. Sci.: Processes Impacts, 2022, 24, 689–753 RSC.
- D. A. Ellis, J. W. Martin, A. O. De Silva, S. A. Mabury, M. D. Hurley, M. P. Sulbaek Andersen and T. J. Wallington, Degradation of Fluorotelomer Alcohols: A Likely Atmospheric Source of Perfluorinated Carboxylic Acids, Environ. Sci. Technol., 2004, 38, 3316–3321 CrossRef CAS PubMed.
- T. J. Wallington, M. D. Hurley, J. Xia, D. J. Wuebbles, S. Sillman, A. Ito, J. E. Penner, D. A. Ellis, J. Martin, S. A. Mabury, O. J. Nielsen and M. P. Sulbaek Andersen, Formation of C7F15COOH (PFOA) and Other Perfluorocarboxylic Acids during the Atmospheric Oxidation of 8:2 Fluorotelomer Alcohol, Environ. Sci. Technol., 2006, 40, 924–930 CrossRef CAS PubMed.
- S. Kutsuna, Y. Nagaoka, K. Takeuchi and H. Hori, TiO2-Induced Heterogeneous Photodegradation of a Fluorotelomer Alcohol in Air, Environ. Sci. Technol., 2006, 40, 6824–6829 CrossRef CAS PubMed.
- G. Yarwood, S. Kemball-Cook, M. Keinath, R. L. Waterland, S. H. Korzeniowski, R. C. Buck, M. H. Russell and S. T. Washburn, High-Resolution Atmospheric Modeling of Fluorotelomer Alcohols and Perfluorocarboxylic Acids in the North American Troposphere, Environ. Sci. Technol., 2007, 41, 5756–5762 CrossRef CAS PubMed.
- F. Wania, A Global Mass Balance Analysis of the Source of Perfluorocarboxylic Acids in the Arctic Ocean, Environ. Sci. Technol., 2007, 41, 4529–4535 CrossRef CAS PubMed.
-
C. J. Young and S. A. Mabury, in Reviews of Environmental Contamination and Toxicology Volume 208: Perfluorinated Alkylated Substances, ed. P. De Voogt, Springer, New York, NY, 2010, pp. 1–109 Search PubMed.
- S. D. Vento, C. Halsall, R. Gioia, K. Jones and J. Dachs, Volatile per- and polyfluoroalkyl compounds in the remote atmosphere of the western Antarctic Peninsula: an indirect source of perfluoroalkyl acids to Antarctic waters?, Atmos. Pollut. Res., 2012, 3, 450–455 CrossRef.
- C. E. Müller, A. C. Gerecke, C. Bogdal, Z. Wang, M. Scheringer and K. Hungerbühler, Atmospheric fate of poly- and perfluorinated alkyl substances (PFASs): I. Day–night patterns of air concentrations in summer in Zurich, Switzerland, Environ. Pollut., 2012, 169, 196–203 CrossRef PubMed.
- Z. Wang, M. Scheringer, M. MacLeod, C. Bogdal, C. E. Müller, A. C. Gerecke and K. Hungerbühler, Atmospheric fate of poly- and perfluorinated alkyl substances (PFASs): II. Emission source strength in summer in Zurich, Switzerland, Environ. Pollut., 2012, 169, 204–209 CrossRef CAS PubMed.
- S. A. Styler, A. L. Myers and D. J. Donaldson, Heterogeneous Photooxidation of Fluorotelomer Alcohols: A New Source of Aerosol-Phase Perfluorinated Carboxylic Acids, Environ. Sci. Technol., 2013, 47, 6358–6367 CrossRef CAS PubMed.
- A. Gawor, C. Shunthirasingham, S. J. Hayward, Y. D. Lei, T. Gouin, B. T. Mmereki, W. Masamba, C. Ruepert, L. E. Castillo, M. Shoeib, S. C. Lee, T. Harner and F. Wania, Neutral polyfluoroalkyl substances in the global Atmosphere, Environ. Sci.: Processes Impacts, 2014, 16, 404–413 RSC.
- J. Kongpran, S. Tanaka, S. Fujii, Y. Suzuki, N. Sakui and N. Saito, Wet Deposition and Photodegradation of Perfluoroalkyl Carboxylates and Fluorotelomer Alcohols: Distribution Pathways from Air to Water Environment, J. Water Environ. Technol., 2014, 12, 481–499 CrossRef.
- Z. Wang, J. M. Boucher, M. Scheringer, I. T. Cousins and K. Hungerbühler, Toward a Comprehensive
Global Emission Inventory of C4–C10 Perfluoroalkanesulfonic Acids (PFSAs) and Related Precursors: Focus on the Life Cycle of C8-Based Products and Ongoing Industrial Transition, Environ. Sci. Technol., 2017, 51, 4482–4493 CrossRef CAS PubMed.
- C. P. Thackray and N. E. Selin, Uncertainty and variability in atmospheric formation of PFCAs from fluorotelomer precursors, Atmos. Chem. Phys., 2017, 17, 4585–4597 CrossRef CAS.
- Q. Wang, Y. Ruan, H. Lin and P. K. S. Lam, Review on perfluoroalkyl and polyfluoroalkyl substances (PFASs) in the Chinese atmospheric environment, Sci. Total Environ., 2020, 737, 139804 CrossRef CAS PubMed.
- C. P. Thackray, N. E. Selin and C. J. Young, A global atmospheric chemistry model for the fate and transport of PFCAs and their precursors, Environ. Sci.: Processes Impacts, 2020, 22, 285–293 RSC.
- C. M. Butt, U. Berger, R. Bossi and G. T. Tomy, Levels and trends of poly- and perfluorinated compounds in the arctic environment, Sci. Total Environ., 2010, 408, 2936–2965 CrossRef CAS PubMed.
- C. J. Young, V. I. Furdui, J. Franklin, R. M. Koerner, D. C. G. Muir and S. A. Mabury, Perfluorinated Acids in Arctic Snow: New Evidence for Atmospheric Formation, Environ. Sci. Technol., 2007, 41, 3455–3461 CrossRef CAS PubMed.
- U. Schenker, M. Scheringer, M. MacLeod, J. W. Martin, I. T. Cousins and K. Hungerbühler, Contribution of Volatile Precursor Substances to the Flux of Perfluorooctanoate to the Arctic, Environ. Sci. Technol., 2008, 42, 3710–3716 CrossRef CAS PubMed.
- M. Cai, H. Yang, Z. Xie, Z. Zhao, F. Wang, Z. Lu, R. Sturm and R. Ebinghaus, Per- and polyfluoroalkyl substances in snow, lake, surface runoff water and coastal seawater in Fildes Peninsula, King George Island, Antarctica, J. Hazard. Mater., 2012, 209–210, 335–342 CrossRef CAS PubMed.
- F. Wong, H. Hung, H. Dryfhout-Clark, W. Aas, P. Bohlin-Nizzetto, K. Breivik, M. N. Mastromonaco, E. B. Lundén, K. Ólafsdóttir, Á. Sigurðsson, K. Vorkamp, R. Bossi, H. Skov, H. Hakola, E. Barresi, E. Sverko, P. Fellin, H. Li, A. Vlasenko, M. Zapevalov, D. Samsonov and S. Wilson, Time trends of persistent organic pollutants (POPs) and Chemicals of Emerging Arctic Concern (CEAC) in Arctic air from 25 years of monitoring, Sci. Total Environ., 2021, 775, 145109 CrossRef CAS PubMed.
- W. F. Hartz, M. K. Björnsdotter, L. W. Y. Yeung, A. Hodson, E. R. Thomas, J. D. Humby, C. Day, I. E. Jogsten, A. Kärrman and R. Kallenborn, Levels and distribution profiles of Per- and Polyfluoroalkyl Substances (PFAS) in a high Arctic Svalbard ice core, Sci. Total Environ., 2023, 871, 161830 CrossRef CAS PubMed.
- M. J. A. Dinglasan, Y. Ye, E. A. Edwards and S. A. Mabury, Fluorotelomer Alcohol Biodegradation Yields Poly- and Perfluorinated Acids, Environ. Sci. Technol., 2004, 38, 2857–2864 CrossRef CAS PubMed.
- N. Wang, B. Szostek, R. C. Buck, P. W. Folsom, L. M. Sulecki, V. Capka, W. R. Berti and J. T. Gannon, Fluorotelomer Alcohol Biodegradation Direct Evidence that Perfluorinated Carbon Chains Breakdown, Environ. Sci. Technol., 2005, 39, 7516–7528 CrossRef CAS PubMed.
- J. Liu, L. S. Lee, L. F. Nies, C. H. Nakatsu and R. F. Turco, Biotransformation of 8:2 Fluorotelomer Alcohol in Soil and by Soil Bacteria Isolates, Environ. Sci. Technol., 2007, 41, 8024–8030 CrossRef CAS PubMed.
- N. Wang, B. Szostek, R. C. Buck, P. W. Folsom, L. M. Sulecki and J. T. Gannon, 8-2 Fluorotelomer alcohol aerobic soil biodegradation: Pathways, metabolites, and metabolite yields, Chemosphere, 2009, 75, 1089–1096 CrossRef CAS PubMed.
- J. J. Ellington, J. W. Washington, J. J. Evans, T. M. Jenkins, S. C. Hafner and M. P. Neill, Analysis of fluorotelomer alcohols in soils: Optimization of extraction and chromatography, J. Chromatogr. A, 2009, 1216, 5347–5354 CrossRef CAS PubMed.
- J. Liu, N. Wang, R. C. Buck, B. W. Wolstenholme, P. W. Folsom, L. M. Sulecki and C. A. Bellin, Aerobic biodegradation of [14C] 6:2 fluorotelomer alcohol in a flow-through soil incubation system, Chemosphere, 2010, 80, 716–723 CrossRef CAS PubMed.
- J. Liu, N. Wang, B. Szostek, R. C. Buck, P. K. Panciroli, P. W. Folsom, L. M. Sulecki and C. A. Bellin, 6-2 Fluorotelomer alcohol aerobic biodegradation in soil and mixed bacterial culture, Chemosphere, 2010, 78, 437–444 CrossRef CAS PubMed.
- M. H. Kim, N. Wang, T. McDonald and K.-H. Chu, Biodefluorination and biotransformation of fluorotelomer alcohols by two alkane-degrading Pseudomonas strains, Biotechnol. Bioeng., 2012, 109, 3041–3048 CrossRef CAS PubMed.
- C. M. Butt, D. C. G. Muir and S. A. Mabury, Biotransformation pathways of fluorotelomer-based polyfluoroalkyl substances: A review, Environ. Toxicol. Chem., 2014, 33, 243–267 CrossRef CAS PubMed.
- N. Tseng, N. Wang, B. Szostek and S. Mahendra, Biotransformation of 6:2 fluorotelomer alcohol (6:2 FTOH) by a wood-rotting fungus, Environ. Sci. Technol., 2014, 48, 4012–4020 CrossRef CAS PubMed.
- M. H. Kim, N. Wang and K. H. Chu, 6:2 Fluorotelomer alcohol (6:2 FTOH) biodegradation by multiple microbial species under different physiological conditions, Appl. Microbiol. Biotechnol., 2014, 98, 1831–1840 CrossRef CAS PubMed.
- M. Lewis, M.-H. Kim, N. Wang and K.-H. Chu, Engineering artificial communities for enhanced FTOH degradation, Sci. Total Environ., 2016, 572, 935–942 CrossRef CAS PubMed.
- N. Merino, M. Wang, R. Ambrocio, K. Mak, E. O'Connor, A. Gao, E. L. Hawley, R. A. Deeb, L. Y. Tseng and S. Mahendra, Fungal biotransformation of 6:2 fluorotelomer alcohol, Remed. J., 2018, 28, 59–70 CrossRef.
- X. Yu, F. Nishimura and T. Hidaka, Enhanced generation of perfluoroalkyl carboxylic acids (PFCAs) from fluorotelomer alcohols (FTOHs) via ammonia-oxidation process, Chemosphere, 2018, 198, 311–319 CrossRef CAS PubMed.
- Y. J. Choi, D. E. Helbling, J. Liu, C. I. Olivares and C. P. Higgins, Microbial biotransformation of aqueous film-forming foam derived polyfluoroalkyl substances, Sci. Total Environ., 2022, 824, 153711 CrossRef CAS PubMed.
- P.-F. Yan, S. Dong, K. E. Manz, C. Liu, M. J. Woodcock, M. P. Mezzari, L. M. Abriola, K. D. Pennell and N. L. Cápiro, Biotransformation of 8:2 Fluorotelomer Alcohol in Soil from Aqueous Film-Forming Foams (AFFFs)-Impacted Sites under Nitrate-, Sulfate-, and Iron-Reducing Conditions, Environ. Sci. Technol., 2022, 56, 13728–13739 CrossRef CAS PubMed.
- M. F. Khan and C. D. Murphy, Fluorotelomer alcohols are efficiently biotransformed by Cunninghamella elegans, Environ. Sci. Pollut. Res., 2023, 30, 23613–23623 CrossRef CAS PubMed.
- S. Dong, P.-F. Yan, C. Liu, K. E. Manz, M. P. Mezzari, L. M. Abriola, K. D. Pennell and N. L. Cápiro, Assessing aerobic biotransformation of 8:2 fluorotelomer alcohol in aqueous film-forming foam (AFFF)-impacted soils: Pathways and microbial community dynamics, J. Hazard. Mater., 2023, 446, 130629 CrossRef CAS PubMed.
- N. Merino, N. Wang, Y. Gao, M. Wang and S. Mahendra, Roles of various enzymes in the biotransformation of 6:2 fluorotelomer alcohol (6:2 FTOH) by a white-rot fungus, J. Hazard. Mater., 2023, 450, 131007 CrossRef CAS PubMed.
- A. Berhanu, I. Mutanda, J. Taolin, M. A. Qaria, B. Yang and D. Zhu, A review of microbial degradation of per- and polyfluoroalkyl substances (PFAS): Biotransformation routes and enzymes, Sci. Total
Environ., 2023, 859, 160010 CrossRef CAS PubMed.
- S.-H. Yang, L. Shan and K.-H. Chu, Root exudates enhanced 6:2 FTOH defluorination, altered metabolite profiles and shifted soil microbiome dynamics, J. Hazard. Mater., 2024, 466, 133651 CrossRef CAS PubMed.
- J. Popovic, C. J. Bee, K. H. Beam, K. Dovantzis, J. M. Stapleton, M. A. Barba, M. R. P. Callier and W. Yoon, Network Meta-Analysis and Systematic Review of Conditions Facilitating Microbial Biotransformation of 6:2 and 8:2 Fluorotelomer Alcohols to Perfluoroalkyl Carboxylates, Environ. Sci. Technol. Lett., 2024, 11, 292–300 CrossRef CAS.
- N. Wang, B. Szostek, P. W. Folsom, L. M. Sulecki, V. Capka, R. C. Buck, W. R. Berti and J. T. Gannon, Aerobic Biotransformation of 14C-Labeled 8-2 Telomer B Alcohol by Activated Sludge from a Domestic Sewage Treatment Plant, Environ. Sci. Technol., 2005, 39, 531–538 CrossRef CAS PubMed.
- M. Sáez, P. de Voogt and J. R. Parsons, Persistence of perfluoroalkylated substances in closed bottle tests with municipal sewage sludge, Environ. Sci. Pollut. Res., 2008, 15, 472–477 CrossRef PubMed.
- H. Yoo, J. W. Washington, J. J. Ellington, T. M. Jenkins and M. P. Neill, Concentrations, Distribution, and Persistence of Fluorotelomer Alcohols in Sludge-Applied Soils near Decatur, Alabama, USA, Environ. Sci. Technol., 2010, 44, 8397–8402 CrossRef CAS.
- L. Zhao, P. K. McCausland, P. W. Folsom, B. W. Wolstenholme, H. Sun, N. Wang and R. C. Buck, 6:2 Fluorotelomer alcohol aerobic biotransformation in activated sludge from two domestic wastewater treatment plants, Chemosphere, 2013, 92, 464–470 CrossRef CAS.
- S. Zhang, B. Szostek, P. K. McCausland, B. W. Wolstenholme, X. Lu, N. Wang and R. C. Buck, 6:2 and 8:2 Fluorotelomer Alcohol Anaerobic Biotransformation in Digester Sludge from a WWTP under Methanogenic Conditions, Environ. Sci. Technol., 2013, 47, 4227–4235 CrossRef CAS PubMed.
- X. Yu, Y. Takabe, K. Yamamoto, C. Matsumura and F. Nishimura, Biodegradation Property of 8:2 Fluorotelomer Alcohol (8:2 FTOH) under Aerobic/Anoxic/Anaerobic Conditions, J. Water Environ. Technol., 2016, 14, 177–190 CrossRef.
- F. Li, Q. Su, Z. Zhou, X. Liao, J. Zou, B. Yuan and W. Sun, Anaerobic biodegradation of 8:2 fluorotelomer alcohol in anaerobic activated sludge: Metabolic products and pathways, Chemosphere, 2018, 200, 124–132 CrossRef CAS.
- X. Yu, F. Nishimura and T. Hidaka, Effects of microbial activity on perfluorinated carboxylic acids (PFCAs) generation during aerobic biotransformation of fluorotelomer alcohols in activated sludge, Sci. Total Environ., 2018, 610–611, 776–785 CrossRef CAS PubMed.
- W. Qiao, J. Miao, H. Jiang and Q. Yang, Degradation and effect of 6:2 fluorotelomer alcohol in aerobic composting of sludge, Biodegradation, 2021, 32, 99–112 CrossRef CAS PubMed.
- L. Zhao, P. W. Folsom, B. W. Wolstenholme, H. Sun, N. Wang and R. C. Buck, 6:2 Fluorotelomer alcohol biotransformation in an aerobic river sediment system, Chemosphere, 2013, 90, 203–209 CrossRef CAS PubMed.
- S. Zhang, N. Merino, N. Wang, T. Ruan and X. Lu, Impact of 6:2 fluorotelomer alcohol aerobic biotransformation on a sediment microbial community, Sci. Total Environ., 2017, 575, 1361–1368 CrossRef CAS PubMed.
- H. Hamid, L. Y. Li and J. R. Grace, Aerobic biotransformation of fluorotelomer compounds in landfill leachate-sediment, Sci. Total Environ., 2020, 713, 136547 CrossRef CAS PubMed.
- H. Zhang, B. Wen, X. Hu, Y. Wu, L. Luo, Z. Chen and S. Zhang, Determination of fluorotelomer alcohols and their degradation products in biosolids-amended soils and plants using ultra-high performance liquid chromatography tandem mass spectrometry, J. Chromatogr. A, 2015, 1404, 72–80 CrossRef CAS PubMed.
- H. Zhang, B. Wen, X. Hu, Y. Wu, Y. Pan, H. Huang, L. Liu and S. Zhang, Uptake, Translocation, and Metabolism of 8:2 Fluorotelomer Alcohol in Soybean (Glycine max L. Merrill), Environ. Sci. Technol., 2016, 50, 13309–13317 CrossRef CAS PubMed.
- S. Zhao and L. Zhu, Uptake and metabolism of 10:2 fluorotelomer alcohol in soil-earthworm (Eisenia fetida) and soil-wheat (Triticum aestivum L.) systems, Environ. Pollut., 2017, 220, 124–131 CrossRef CAS PubMed.
- M. Muschket, N. Keltsch, H. Paschke, T. Reemtsma and U. Berger, Determination of transformation products of per- and polyfluoroalkyl substances at trace levels in agricultural plants, J. Chromatogr. A, 2020, 1625, 461271 CrossRef CAS PubMed.
- Y. Yao, Z. Lan, H. Zhu, J. Xu and H. Sun, Foliar uptake overweighs root uptake for 8:2 fluorotelomer alcohol in ryegrass (Lolium perenne L.): A closed exposure chamber study, Sci. Total Environ., 2022, 829, 154660 CrossRef CAS PubMed.
- H. Just, B. Göckener, R. Lämmer, L. Wiedemann-Krantz, T. Stahl, J. Breuer, M. Gassmann, E. Weidemann, M. Bücking and J. Kowalczyk, Degradation and Plant Transfer Rates of Seven Fluorotelomer Precursors to Perfluoroalkyl Acids and F-53B in a Soil-Plant System with Maize (Zea mays L.), J. Agric. Food Chem., 2022, 70, 8920–8930 CrossRef CAS PubMed.
- Y. Zhang, S. Wu, Q.-Q. Chen, P.-F. Yu, H.-M. Zhao, N.-X. Feng, B.-L. Liu, Y.-W. Li, Q.-Y. Cai, L. Xiang, C.-H. Mo and Q. X. Li, Highly Efficient and Simultaneous Analysis of Three Common Fluorotelomer Alcohols in Vegetables and Soils, J. Agric. Food Chem., 2023, 71, 11704–11715 CrossRef CAS.
- I. A. Titaley, J. Khattak, J. Dong, C. I. Olivares, B. DiGuiseppi, C. C. Lutes and J. A. Field, Neutral Per- and Polyfluoroalkyl Substances, Butyl Carbitol, and Organic Corrosion Inhibitors in Aqueous Film-Forming Foams: Implications for Vapor Intrusion and the Environment, Environ. Sci. Technol., 2022, 56, 10785–10797 CrossRef CAS.
- S. A. Gauthier and S. A. Mabury, Aqueous photolysis of 8:2 fluorotelomer alcohol, Environ. Toxicol. Chem., 2005, 24, 1837–1846 CrossRef CAS PubMed.
- S. Taniyasu, N. Yamashita, E. Yamazaki, G. Petrick and K. Kannan, The environmental photolysis of perfluorooctanesulfonate, perfluorooctanoate, and related fluorochemicals, Chemosphere, 2013, 90, 1686–1692 CrossRef CAS PubMed.
- J. Keränen, H. Ahkola, J. Knuutinen, S. Herve, M. Reinikainen and J. Koistinen, Formation of PFOA from 8:2 FTOH in closed-bottle experiments with brackish water, Environ. Sci. Pollut. Res., 2013, 20, 8001–8012 CrossRef PubMed.
- J. W. Martin, S. A. Mabury and P. J. O'Brien, Metabolic products and pathways of fluorotelomer alcohols in isolated rat hepatocytes, Chem.-Biol. Interact., 2005, 155, 165–180 CrossRef CAS.
- W. J. Fasano, S. C. Carpenter, S. A. Gannon, T. A. Snow, J. C. Stadler, G. L. Kennedy, R. C. Buck, S. H. Korzeniowski, P. M. Hinderliter and R. A. Kemper, Absorption, Distribution, Metabolism, and Elimination of 8-2 Fluorotelomer Alcohol in the Rat, Toxicol. Sci., 2006, 91, 341–355 CrossRef CAS PubMed.
- D. L. Nabb, B. Szostek, M. W. Himmelstein, M. P. Mawn, M. L. Gargas, L. M. Sweeney, J. C. Stadler, R. C. Buck and W. J. Fasano, In Vitro Metabolism of 8-2 Fluorotelomer Alcohol: Interspecies Comparisons and Metabolic Pathway Refinement, Toxicol. Sci., 2007, 100, 333–344 CrossRef CAS PubMed.
- W. M. Henderson, E. J. Weber, S. E. Duirk, J. W. Washington and M. A. Smith, Quantification of fluorotelomer-based chemicals in mammalian matrices by monitoring perfluoroalkyl chain fragments with GC/MS, J. Chromatogr. B, 2007, 846, 155–161 CrossRef CAS PubMed.
- W. J. Fasano, L. M. Sweeney, M. P. Mawn, D. L. Nabb, B. Szostek, R. C. Buck and M. L. Gargas, Kinetics of 8-2 fluorotelomer alcohol and its metabolites, and liver glutathione status following daily oral dosing for 45 days in male and female rats, Chem.-Biol. Interact., 2009, 180, 281–295 CrossRef CAS.
- H. Nilsson, A. Kärrman, A. Rotander, B. van Bavel, G. Lindström and H. Westberg, Inhalation Exposure to Fluorotelomer Alcohols Yield Perfluorocarboxylates in Human Blood?, Environ. Sci. Technol., 2010, 44, 7717–7722 CrossRef CAS PubMed.
- H. Nilsson, A. Kärrman, H. Westberg, A. Rotander, B. van Bavel and G. Lindström, A Time Trend Study of Significantly Elevated Perfluorocarboxylate Levels in Humans after Using Fluorinated Ski Wax, Environ. Sci. Technol., 2010, 44, 2150–2155 CrossRef CAS PubMed.
- A. J. Fraser, T. F. Webster, D. J. Watkins, J. W. Nelson, H. M. Stapleton, A. M. Calafat, K. Kato, M. Shoeib, V. M. Vieira and M. D. McClean, Polyfluorinated Compounds in Serum Linked to Indoor Air in Office Environments, Environ. Sci. Technol., 2012, 46, 1209–1215 CrossRef CAS PubMed.
- H. Nilsson, A. Kärrman, A. Rotander, B. van Bavel, G. Lindström and H. Westberg, Biotransformation of fluorotelomer compound to perfluorocarboxylates in humans, Environ. Int., 2013, 51, 8–12 CrossRef CAS PubMed.
- M. H. Russell, M. W. Himmelstein and R. C. Buck, Inhalation and oral toxicokinetics of 6:2 FTOH and its metabolites in mammals, Chemosphere, 2015, 120, 328–335 CrossRef CAS PubMed.
- M. I. Gomis, R. Vestergren, H. Nilsson and I. T. Cousins, Contribution of Direct and Indirect Exposure to Human Serum Concentrations of Perfluorooctanoic Acid in an Occupationally Exposed Group of Ski Waxers, Environ. Sci. Technol., 2016, 50, 7037–7046 CrossRef CAS PubMed.
- S. Dagnino, M. J. Strynar, R. L. McMahen, C. S. Lau, C. Ball, S. Garantziotis, T. F. Webster, M. D. McClean and A. B. Lindstrom, Identification of Biomarkers of Exposure to FTOHs and PAPs in Humans Using a Targeted and Nontargeted Analysis Approach, Environ. Sci. Technol., 2016, 50, 10216–10225 CrossRef CAS PubMed.
- C. M. Makey, T. F. Webster, J. W. Martin, M. Shoeib, T. Harner, L. Dix-Cooper and G. M. Webster, Airborne Precursors Predict Maternal Serum Perfluoroalkyl Acid Concentrations, Environ. Sci. Technol., 2017, 51, 7667–7675 CrossRef CAS.
- M. C. Huang, V. G. Robinson, S. Waidyanatha, A. L. Dzierlenga, M. J. DeVito, M. A. Eifrid, S. T. Gibbs and C. R. Blystone, Toxicokinetics of 8:2 fluorotelomer alcohol (8:2-FTOH) in male and female Hsd:Sprague Dawley SD rats after intravenous and gavage administration, Toxicol Rep, 2019, 6, 924–932 CrossRef CAS.
- S. Xie, Y. Cui, Y. Yang, K. Meng, Y. Pan, Z. Liu and D. Chen, Tissue distribution and bioaccumulation of 8:2 fluorotelomer alcohol and its metabolites in pigs after oral exposure, Chemosphere, 2020, 249, 126016 CrossRef CAS.
- S. V. Kabadi, J. W. Fisher, D. R. Doerge, D. Mehta, J. Aungst and P. Rice, Characterizing biopersistence potential of the metabolite 5:3 fluorotelomer carboxylic acid after repeated oral exposure to the 6:2 fluorotelomer alcohol, Toxicol. Appl. Pharmacol., 2020, 388, 114878 CrossRef CAS PubMed.
- O. Daramola and A. A. Rand, Emerging investigator series: human CYP2A6 catalyzes the oxidation of 6:2 fluorotelomer alcohol, Environ. Sci.: Processes Impacts, 2021, 23, 1688–1695 RSC.
- N. Xu, H. Lin, J.-M. Lin, J. Cheng, P. Wang and L. Lin, Microfluidic Chip-Based Modeling of Three-Dimensional Intestine–Vessel–Liver Interactions in Fluorotelomer Alcohol Biotransformation, Anal. Chem., 2023, 96, 17064–17072 CrossRef PubMed.
- N. Xu, H. Lin, Q. Du, S. Dong, J. Cheng, P. Wang and J.-M. Lin,
In situ investigation of detoxification and metabolic effects of polyfluoroalkyl substances on metal–organic frameworks combined with cell-cultured microfluidics, Lab Chip, 2023, 23, 3062–3069 RSC.
- P. A. Rice, S. V. Kabadi, D. R. Doerge, M. M. Vanlandingham, M. I. Churchwell, V. P. Tryndyak, J. W. Fisher, J. Aungst and F. A. Beland, Evaluating the toxicokinetics of some metabolites of a C6 polyfluorinated compound, 6:2 fluorotelomer alcohol in pregnant and nonpregnant rats after oral exposure to the parent compound, Food Chem. Toxicol., 2024, 183, 114333 CrossRef CAS PubMed.
- D. Chen, Y. Zhao, W. Xu, Y. Pan, Q. Wei and S. Xie, Biotransformation and tissue bioaccumulation of 8:2 fluorotelomer alcohol in broiler by oral exposure, Environ. Pollut., 2020, 267, 115611 CrossRef CAS PubMed.
- C. M. Butt, D. C. G. Muir and S. A. Mabury, Elucidating the Pathways of Poly- and Perfluorinated Acid Formation in Rainbow Trout, Environ. Sci. Technol., 2010, 44, 4973–4980 CrossRef CAS PubMed.
- S. H. Brandsma, M. Smithwick, K. Solomon, J. Small, J. de Boer and D. C. G. Muir, Dietary exposure of rainbow trout to 8:2 and 10:2 fluorotelomer alcohols and perfluorooctanesulfonamide: Uptake, transformation and elimination, Chemosphere, 2011, 82, 253–258 CrossRef CAS.
- A. A. Rand and S. A. Mabury, Is there a human health risk associated with indirect exposure to perfluoroalkyl carboxylates (PFCAs)?, Toxicology, 2017, 375, 28–36 CrossRef CAS PubMed.
- R. W. Speke, Variables in Padding Processes, J. Soc. Dyers Colour., 1954, 70, 221–226 CrossRef.
-
U. Sayed and P. Dabhi, in Waterproof and Water Repellent Textiles and Clothing, ed. J. Williams, Woodhead Publishing, 2014, pp. 139–152 Search PubMed.
- L. C. Wickersham, J. M. Mattila, J. D. Krug, S. R. Jackson, M. A. G. Wallace, E. P. Shields, H. Halliday, E. Y. Li, H. K. Liberatore, S. Farrior, W. Preston, J. V. Ryan, C.-W. Lee and W. P. Linak, Characterization of PFAS Air Emissions from Thermal Application of Fluoropolymer Dispersions on Fabrics, J. Air Waste Manage. Assoc., 2023, 73, 533–552 CrossRef CAS PubMed.
- F. Heydebreck, J. Tang, Z. Xie and R. Ebinghaus, Emissions of Per- and Polyfluoroalkyl Substances in a Textile Manufacturing Plant in China and Their Relevance for Workers' Exposure, Environ. Sci. Technol., 2016, 50, 10386–10396 CrossRef CAS PubMed.
- T. Paris-Davila, L. G. T. Gaines, K. Lucas and L. A. Nylander-French, Occupational exposures to airborne per- and polyfluoroalkyl substances (PFAS)—A review, Am. J. Ind. Med., 2023, 66, 393–410 CrossRef CAS PubMed.
- M. Schlummer, L. Gruber, D. Fiedler, M. Kizlauskas and J. Müller, Detection of fluorotelomer alcohols in indoor environments and their relevance for human exposure, Environ. Int., 2013, 57–58, 42–49 CrossRef CAS PubMed.
- A. Shinde and R. B. Ormond, Headspace sampling-gas chromatograph-mass spectrometer as a screening method to thermally extract fireground contaminants from retired firefighting turnout jackets, Fire Mater., 2020, 45, 415–428 CrossRef PubMed.
- Z. G. Robbins, X. Liu, B. A. Schumacher, M. G. Smeltz and H. K. Liberatore, Method development for thermal desorption-gas chromatography-tandem mass spectrometry (TD-GC–MS/MS) analysis of trace level fluorotelomer alcohols emitted from consumer products, J. Chromatogr. A, 2023, 1705, 464204 CrossRef CAS PubMed.
- A. Jahnke, S. Huber, C. Temme, H. Kylin and U. Berger, Development and application of a simplified sampling method for volatile polyfluorinated alkyl substances in indoor and environmental air, J. Chromatogr. A, 2007, 1164, 1–9 CrossRef CAS PubMed.
- J. L. Barber, U. Berger, C. Chaemfa, S. Huber, A. Jahnke, C. Temme and K. C. Jones, Analysis of per- and polyfluorinated alkyl substances in air samples from Northwest Europe, J. Environ. Monit., 2007, 9, 530–541 RSC.
- V. Langer, A. Dreyer and R. Ebinghaus, Polyfluorinated Compounds in Residential and Nonresidential Indoor Air, Environ. Sci. Technol., 2010, 44, 8075–8081 CrossRef CAS PubMed.
- L. S. Haug, S. Huber, M. Schlabach, G. Becher and C. Thomsen, Investigation on Per- and Polyfluorinated Compounds in Paired Samples of House Dust and Indoor Air from Norwegian Homes, Environ. Sci. Technol., 2011, 45, 7991–7998 CrossRef CAS PubMed.
- S. Huber, L. S. Haug and M. Schlabach, Per- and polyfluorinated compounds in house dust and indoor air from northern Norway – A pilot study, Chemosphere, 2011, 84, 1686–1693 CrossRef CAS PubMed.
- M. Shoeib, T. Harner, G. M. Webster and S. C. Lee, Indoor Sources of Poly- and Perfluorinated Compounds (PFCS) in Vancouver, Canada: Implications for Human Exposure, Environ. Sci. Technol., 2011, 45, 7999–8005 CrossRef CAS PubMed.
- I. Ericson Jogsten, M. Nadal, B. van Bavel, G. Lindström and J. L. Domingo, Per- and polyfluorinated compounds (PFCs) in house dust and indoor air in Catalonia, Spain: Implications for human exposure, Environ. Int., 2012, 39, 172–180 CrossRef CAS PubMed.
- Y. Wu and V. W.-C. Chang, Development of analysis of volatile polyfluorinated alkyl substances in indoor air using thermal desorption-gas chromatography–mass spectrometry, J. Chromatogr. A, 2012, 1238, 114–120 CrossRef CAS PubMed.
- W. Liu, S. Takahashi, Y. Sakuramachi, K. H. Harada and A. Koizumi, Polyfluorinated telomers in indoor air of Japanese houses, Chemosphere, 2013, 90, 1672–1677 CrossRef CAS PubMed.
- H. Fromme, A. Dreyer, S. Dietrich, L. Fembacher, T. Lahrz and W. Völkel, Neutral polyfluorinated compounds in indoor air in Germany – The LUPE 4 study, Chemosphere, 2015, 139, 572–578 CrossRef CAS PubMed.
- K. Winkens, J. Koponen, J. Schuster, M. Shoeib, R. Vestergren, U. Berger, A. M. Karvonen, J. Pekkanen, H. Kiviranta and I. T. Cousins, Perfluoroalkyl acids and their precursors in indoor air sampled in children's bedrooms, Environ. Pollut., 2017, 222, 423–432 CrossRef CAS PubMed.
- J. A. Padilla-Sánchez, E. Papadopoulou, S. Poothong and L. S. Haug, Investigation of the Best Approach for Assessing Human Exposure to Poly- and Perfluoroalkyl Substances through Indoor Air, Environ. Sci. Technol., 2017, 51, 12836–12843 CrossRef PubMed.
- B. Sha, A.-K. Dahlberg, K. Wiberg and L. Ahrens, Fluorotelomer alcohols (FTOHs), brominated flame retardants (BFRs), organophosphorus flame retardants (OPFRs) and cyclic volatile methylsiloxanes (cVMSs) in indoor air from occupational and home environments, Environ. Pollut., 2018, 241, 319–330 CrossRef CAS PubMed.
- Y. Yao, Y. Zhao, H. Sun, S. Chang, L. Zhu, A. C. Alder and K. Kannan, Per- and Polyfluoroalkyl Substances (PFASs) in Indoor Air and Dust from Homes and Various Microenvironments in China: Implications for Human Exposure, Environ. Sci. Technol., 2018, 52, 3156–3166 CrossRef CAS PubMed.
- M. E. Morales-McDevitt, J. Becanova, A. Blum, T. A. Bruton, S. Vojta, M. Woodward and R. Lohmann, The Air That We Breathe: Neutral and Volatile PFAS in Indoor Air, Environ. Sci. Technol. Lett., 2021, 8, 897–902 CrossRef CAS PubMed.
- C. M. A. Eichler and J. C. Little, A framework to model exposure to per- and polyfluoroalkyl substances in indoor environments, Environ. Sci.: Processes Impacts, 2020, 22, 500–511 RSC.
- C. M. A. Eichler, C. Bi, C. Wang and J. C. Little, A modular mechanistic framework for estimating exposure to SVOCs: Next steps for modeling emission and partitioning of plasticizers and PFAS, J. Exposure Sci. Environ. Epidemiol., 2022, 32, 356–365 CrossRef CAS PubMed.
- L. Zhu, P. Hajeb, P. Fauser and K. Vorkamp, Endocrine disrupting chemicals in indoor dust: A review of temporal and spatial trends, and human exposure, Sci. Total Environ., 2023, 874, 162374 CrossRef CAS PubMed.
- H. Nilsson, A. Kärrman, A. Rotander, B. van Bavel, G. Lindström and H. Westberg, Professional ski waxers' exposure to PFAS and aerosol concentrations in gas phase and different particle size fractions, Environ. Sci.: Processes Impacts, 2013, 15, 814–822 RSC.
- K. A. Crawford, B. T. Doherty, D. Gilbert-Diamond, M. E. Romano and B. Claus Henn, Waxing activity as a potential source of exposure to per- and polyfluoroalkyl substances (PFAS) and other environmental contaminants among the US ski and snowboard community, Environ. Res., 2022, 215, 114335 CrossRef CAS PubMed.
- C. Holder, N. DeLuca, J. Luh, P. Alexander, J. M. Minucci, D. A. Vallero, K. Thomas and E. A. Cohen Hubal, Systematic Evidence Mapping of Potential Exposure Pathways for Per- and Polyfluoroalkyl Substances Based on Measured Occurrence in Multiple Media, Environ. Sci. Technol., 2023, 57, 5107–5116 CrossRef CAS PubMed.
- L. G. T. Gaines and L. A. Nylander-French, Occupational exposure to PFAS: Research and protection needed, Am. J. Ind. Med., 2023, 66, 424–426 CrossRef PubMed.
- K. A. Crawford and N. Hartmann, Respiratory Exposure to Highly Fluorinated Chemicals via Application of Ski Wax and Related Health Effects, Curr. Environ. Health Rep., 2024, 11, 39–45 CrossRef CAS PubMed.
- S. Niu, X. Zhu, R. Chen, A. Winchell, P. Gao, A. Barchowsky, J. M. Buchanich and C. Ng, Personal Wearable Sampler for Per- and Polyfluoroalkyl Substances Exposure Assessment, Environ. Sci. Technol. Lett., 2024, 11, 301–307 CrossRef CAS.
- Z. Xu, S. Fiedler, G. Pfister, B. Henkelmann, C. Mosch, W. Völkel, H. Fromme and K.-W. Schramm, Human exposure to fluorotelomer alcohols, perfluorooctane sulfonate and perfluorooctanoate via house dust in Bavaria, Germany, Sci. Total Environ., 2013, 443, 485–490 CrossRef CAS PubMed.
- Z. Tian, S.-K. Kim, M. Shoeib, J.-E. Oh and J.-E. Park, Human exposure to per- and polyfluoroalkyl substances (PFASs) via house dust in Korea: Implication to exposure pathway, Sci. Total Environ., 2016, 553, 266–275 CrossRef CAS PubMed.
- N. M. DeLuca, J. M. Minucci, A. Mullikin, R. Slover and E. A. Cohen Hubal, Human exposure pathways to poly- and perfluoroalkyl substances (PFAS) from indoor media: A systematic review, Environ. Int., 2022, 162, 107149 CrossRef CAS PubMed.
- S. Poothong, E. Papadopoulou, J. A. Padilla-Sánchez, C. Thomsen and L. S. Haug, Multiple pathways of human exposure to poly- and perfluoroalkyl substances (PFASs): From external exposure to human blood, Environ. Int., 2020, 134, 105244 CrossRef CAS PubMed.
- J. C. Kissel, I. A. Titaley, D. J. Muensterman and J. A. Field, Evaluating Neutral PFAS for Potential Dermal Absorption from the Gas Phase, Environ. Sci. Technol., 2023, 57, 4951–4958 CrossRef CAS PubMed.
- J. C. Kissel, I. A. Titaley, D. J. Muensterman and J. A. Field, Correction to “Evaluating Neutral PFAS for Potential Dermal Absorption from the Gas Phase”, Environ. Sci. Technol., 2023, 57, 9894 CrossRef CAS PubMed.
- A. Ramírez Carnero, A. Lestido-Cardama, P. Vazquez Loureiro, L. Barbosa-Pereira, A. Rodríguez Bernaldo de Quirós and R. Sendón, Presence of Perfluoroalkyl and Polyfluoroalkyl Substances (PFAS) in Food Contact Materials (FCM) and Its Migration to Food, Foods, 2021, 10, 1443 CrossRef PubMed.
- P. A. Rice, J. Aungst, J. Cooper, O. Bandele and S. V. Kabadi, Comparative analysis of the toxicological databases for 6:2 fluorotelomer alcohol (6:2 FTOH) and perfluorohexanoic acid (PFHxA), Food Chem. Toxicol., 2020, 138, 111210 CrossRef CAS PubMed.
- Y. Xia, L. Hao, Y. Li, Y. Li, J. Chen, L. Li, X. Han, Y. Liu, X. Wang and D. Li, Embryonic 6:2 FTOH exposure causes reproductive toxicity by disrupting the formation of the blood-testis barrier in offspring mice, Ecotoxicol. Environ. Saf., 2023, 250, 114497 CrossRef CAS PubMed.
- Y. Rericha, L. Truong, C. Leong, D. Cao, J. A. Field and R. L. Tanguay, Dietary Perfluorohexanoic Acid (PFHxA) Exposures in Juvenile Zebrafish Produce Subtle Behavioral Effects across Generations, Toxics, 2022, 10, 372 CrossRef CAS PubMed.
- M. E. Morales-McDevitt, M. Dunn, A. Habib, S. Vojta, J. Becanova and R. Lohmann, Poly- and Perfluorinated Alkyl Substances in Air and Water from Dhaka, Bangladesh, Environ. Toxicol. Chem., 2022, 41, 334–342 CrossRef CAS PubMed.
- H. Chen, Y. Yao, Z. Zhao, Y. Wang, Q. Wang, C. Ren, B. Wang, H. Sun, A. C. Alder and K. Kannan, Multimedia Distribution and Transfer of Per- and Polyfluoroalkyl Substances (PFASs) Surrounding Two Fluorochemical Manufacturing Facilities in Fuxin, China, Environ. Sci. Technol., 2018, 52, 8263–8271 CrossRef CAS PubMed.
- P. Thepaksorn, P. Lee, Y. Shiwaku, B. Zheng, A. Koizumi and K. H. Harada, Formation and Degradability of Per- and Polyfluoroalkyl Substances in River Soils around a Fluoropolymer-Manufacturing Plant in Osaka, Japan, Arch. Environ. Contam. Toxicol., 2023, 84, 427–435 CrossRef CAS PubMed.
- A. M. Piekarz, T. Primbs, J. A. Field, D. F. Barofsky and S. Simonich, Semivolatile Fluorinated Organic Compounds in Asian and Western U.S. Air Masses, Environ. Sci. Technol., 2007, 41, 8248–8255 CrossRef CAS PubMed.
- T. Primbs, A. Piekarz, G. Wilson, D. Schmedding, C. Higginbotham, J. Field and S. M. Simonich, Influence of Asian and Western United States Urban Areas and Fires on the Atmospheric Transport of Polycyclic Aromatic Hydrocarbons, Polychlorinated Biphenyls, and Fluorotelomer Alcohols in the Western United States, Environ. Sci. Technol., 2008, 42, 6385–6391 CrossRef CAS PubMed.
- J. W. Martin, D. C. G. Muir, C. A. Moody, D. A. Ellis, W. C. Kwan, K. R. Solomon and S. A. Mabury, Collection of Airborne Fluorinated Organics and Analysis by Gas Chromatography/Chemical Ionization Mass Spectrometry, Anal. Chem., 2002, 74, 584–590 CrossRef CAS PubMed.
- A. Jahnke, L. Ahrens, R. Ebinghaus and C. Temme, Urban versus Remote Air Concentrations of Fluorotelomer Alcohols and Other Polyfluorinated Alkyl Substances in Germany, Environ. Sci. Technol., 2007, 41, 745–752 CrossRef CAS PubMed.
- A. Jahnke, L. Ahrens, R. Ebinghaus, U. Berger, J. L. Barber and C. Temme, An improved method for the analysis of volatile polyfluorinated alkyl substances in environmental air samples, Anal. Bioanal. Chem., 2007, 387, 965–975 CrossRef CAS PubMed.
- A. Jahnke, U. Berger, R. Ebinghaus and C. Temme, Latitudinal Gradient of Airborne Polyfluorinated Alkyl Substances in the Marine Atmosphere between Germany and South Africa (53° N–33° S), Environ. Sci. Technol., 2007, 41, 3055–3061 CrossRef CAS PubMed.
- M. Loewen, F. Wania, F. Wang and G. Tomy, Altitudinal Transect of Atmospheric and Aqueous Fluorinated Organic Compounds in Western Canada, Environ. Sci. Technol., 2008, 42, 2374–2379 CrossRef CAS PubMed.
- A. Dreyer, V. Matthias, C. Temme and R. Ebinghaus, Annual Time Series of Air Concentrations of Polyfluorinated Compounds, Environ. Sci. Technol., 2009, 43, 4029–4036 CrossRef CAS PubMed.
- A. Jahnke, J. L. Barber, K. C. Jones and C. Temme, Quantitative trace analysis of polyfluorinated alkyl substances (PFAS) in ambient air samples from Mace Head (Ireland): A method intercomparison, Atmos. Environ., 2009, 43, 844–850 CrossRef CAS.
- S. Genualdi, S. C. Lee, M. Shoeib, A. Gawor, L. Ahrens and T. Harner, Global Pilot Study of Legacy and Emerging Persistent Organic Pollutants using Sorbent-Impregnated Polyurethane Foam Disk Passive Air Samplers, Environ. Sci. Technol., 2010, 44, 5534–5539 CrossRef CAS PubMed.
- L. Ahrens, M. Shoeib, S. D. Vento, G. Codling and C. Halsall, Polyfluoroalkyl compounds in the Canadian Arctic atmosphere, Environ. Chem., 2011, 8, 399–406 CrossRef CAS.
- L. Ahrens, T. Harner, M. Shoeib, M. Koblizkova and E. J. Reiner, Characterization of Two Passive Air Samplers for Per- and Polyfluoroalkyl Substances, Environ. Sci. Technol., 2013, 47, 14024–14033 CrossRef CAS PubMed.
- Y. Wu and V. W.-C. Chang, Comparison of solvent extraction and thermal desorption methods for determination of volatile polyfluorinated alkyl substances in the urban atmosphere, Anal. Methods, 2013, 5, 3410–3417 RSC.
- Y. Yao, S. Chang, H. Sun, Z. Gan, H. Hu, Y. Zhao and Y. Zhang, Neutral and ionic per- and polyfluoroalkyl substances (PFASs) in atmospheric and dry deposition samples over a source region (Tianjin, China), Environ. Pollut., 2016, 212, 449–456 CrossRef CAS PubMed.
- S. Lai, J. Song, T. Song, Z. Huang, Y. Zhang, Y. Zhao, G. Liu, J. Zheng, W. Mi, J. Tang, S. Zou, R. Ebinghaus and Z. Xie, Neutral polyfluoroalkyl substances in the atmosphere over the northern South China Sea, Environ. Pollut., 2016, 214, 449–455 CrossRef CAS PubMed.
- Z. Xu, L. Li, B. Henkelmann and K.-W. Schramm, Occurrence of fluorotelomer alcohols at two Alpine summits: sources, transport and temporal trends, Environ. Chem., 2017, 14, 215–223 CrossRef CAS.
- C. Rauert, M. Shoieb, J. K. Schuster, A. Eng and T. Harner, Atmospheric concentrations and trends of poly- and perfluoroalkyl substances (PFAS) and volatile methyl siloxanes (VMS) over 7 years of sampling in the Global Atmospheric Passive Sampling (GAPS) network, Environ. Pollut., 2018, 238, 94–102 CrossRef CAS PubMed.
- C. Rauert, T. Harner, J. K. Schuster, A. Eng, G. Fillmann, L. E. Castillo, O. Fentanes, M. Villa Ibarra, K. S. B. Miglioranza, I. Moreno Rivadeneira, K. Pozo and B. H. Aristizábal Zuluaga, Atmospheric Concentrations of New Persistent Organic Pollutants and Emerging Chemicals of Concern in the Group of Latin America and Caribbean (GRULAC) Region, Environ. Sci. Technol., 2018, 52, 7240–7249 CrossRef CAS PubMed.
- X. Wang, J. Schuster, K. C. Jones and P. Gong, Occurrence and spatial distribution of neutral perfluoroalkyl substances and cyclic volatile methylsiloxanes in the atmosphere of the Tibetan Plateau, Atmos. Chem. Phys., 2018, 18, 8745–8755 CrossRef CAS.
- E. Dixon-Anderson and R. Lohmann, Field-testing polyethylene passive samplers for the detection of neutral polyfluorinated alkyl substances in air and water, Environ. Toxicol. Chem., 2018, 37, 3002–3010 CrossRef CAS PubMed.
- Z. Lu, R. Lu, H. Zheng, J. Yan, L. Song, J. Wang, H. Yang and M. Cai, Risk exposure assessment of per- and polyfluoroalkyl substances (PFASs) in drinking water and atmosphere in central eastern China, Environ. Sci. Pollut. Res., 2018, 25, 9311–9320 CrossRef CAS PubMed.
- R. Wu, H. Lin, E. Yamazaki, S. Taniyasu, M. Sörengård, L. Ahrens, P. K. S. Lam, H. Eun and N. Yamashita, Simultaneous analysis of neutral and ionizable per- and polyfluoroalkyl substances in air, Chemosphere, 2021, 280, 130607 CrossRef CAS PubMed.
- H. Lin, S. Taniyasu, E. Yamazaki, R. Wu, P. K. S. Lam, H. Eun and N. Yamashita, Fluorine mass balance analysis and per- and polyfluoroalkyl substances in the atmosphere, J. Hazard. Mater., 2022, 435, 129025 CrossRef CAS PubMed.
- C. Xia, S. L. Capozzi, K. A. Romanak, D. C. Lehman, A. Dove, V. Richardson, T. Greenberg, D. McGoldrick and M. Venier, The Ins and Outs of Per- and Polyfluoroalkyl Substances in the Great Lakes: The Role of Atmospheric Deposition, Environ. Sci. Technol., 2024, 58, 9303–9313 CrossRef CAS PubMed.
- T. Stoiber, S. Evans and O. V. Naidenko, Disposal of products and materials containing per- and polyfluoroalkyl substances (PFAS): A cyclical problem, Chemosphere, 2020, 260, 127659 CrossRef CAS PubMed.
- H. Hamid, L. Y. Li and J. R. Grace, Review of the fate and transformation of per- and polyfluoroalkyl substances (PFASs) in landfills, Environ. Pollut., 2018, 235, 74–84 CrossRef CAS PubMed.
- D. R. Reinhart, S. C. Bolyard and J. Chen, Fate of Per- and Polyfluoroalkyl Substances in Postconsumer Products during Waste Management, J. Environ. Eng., 2023, 149, 03123002 CrossRef CAS.
- T. Tolaymat, N. Robey, M. Krause, J. Larson, K. Weitz, S. Parvathikar, L. Phelps, W. Linak, S. Burden, T. Speth and J. Krug, A critical review of perfluoroalkyl and polyfluoroalkyl substances (PFAS) landfill disposal in the United States, Sci. Total Environ., 2023, 905, 167185 CrossRef CAS PubMed.
- E. S. Coffin, D. M. Reeves and D. P. Cassidy, PFAS in Municipal Solid Waste Landfills: Sources, Leachate Composition, Chemical Transformations, and Future Challenges, Curr. Opin. Environ. Sci. Health, 2022, 31, 100418 CrossRef.
- Q. Wang, X. Gu, S. Tang, A. Mohammad, D. N. Singh, H. Xie, Y. Chen, X. Zuo and Z. Sun, Gas transport in landfill cover system: A critical appraisal, J. Environ. Manage., 2022, 321, 116020 CrossRef CAS PubMed.
- L. Tang, X. Yu, W. Zhao, D. Barceló, S. Lyu and Q. Sui, Occurrence, behaviors, and fate of per- and polyfluoroalkyl substances (PFASs) in typical municipal solid waste disposal sites, Water Res., 2024, 252, 121215 CrossRef CAS PubMed.
- I. A. Titaley, F. B. De la Cruz, M. A. Barlaz and J. A. Field, Neutral Per- and Polyfluoroalkyl Substances in In Situ Landfill Gas by Thermal Desorption–Gas Chromatography–Mass Spectrometry, Environ. Sci. Technol. Lett., 2023, 10, 214–221 CrossRef CAS.
- L. Ahrens, M. Shoeib, T. Harner, S. C. Lee, R. Guo and E. J. Reiner, Wastewater Treatment Plant and Landfills as Sources of Polyfluoroalkyl Compounds to the Atmosphere, Environ. Sci. Technol., 2011, 45, 8098–8105 CrossRef CAS PubMed.
- I. Weinberg, A. Dreyer and R. Ebinghaus, Landfills as sources of polyfluorinated compounds, polybrominated diphenyl ethers and musk fragrances to ambient air, Atmos. Environ., 2011, 45, 935–941 CrossRef CAS.
- Y. Tian, Y. Yao, S. Chang, Z. Zhao, Y. Zhao, X. Yuan, F. Wu and H. Sun, Occurrence and Phase Distribution of Neutral and Ionizable Per- and Polyfluoroalkyl Substances (PFASs) in the Atmosphere and Plant Leaves around Landfills: A Case Study in Tianjin, China, Environ. Sci. Technol., 2018, 52, 1301–1310 CrossRef CAS PubMed.
- L. Li, J. Liu, J. Hu and F. Wania, Degradation of Fluorotelomer-Based Polymers Contributes to the Global Occurrence of Fluorotelomer Alcohol and Perfluoroalkyl Carboxylates: A Combined Dynamic Substance Flow and Environmental Fate Modeling Analysis, Environ. Sci. Technol., 2017, 51, 4461–4470 CrossRef CAS PubMed.
- J. W. Washington and T. M. Jenkins, Abiotic Hydrolysis of Fluorotelomer-Based Polymers as a Source of Perfluorocarboxylates at the Global Scale, Environ. Sci. Technol., 2015, 49, 14129–14135 CrossRef CAS PubMed.
- R. van Zelm, M. A. J. Huijbregts, M. H. Russell, T. Jager and D. van de Meent, Modeling the environmental fate of perfluorooctanoate and its precursors from global fluorotelomer acrylate polymer use, Environ. Toxicol. Chem., 2008, 27, 2216–2223 CrossRef CAS PubMed.
- R. Holland, M. A. H. Khan, R. Chhantyal-Pun, A. J. Orr-Ewing, C. J. Percival, C. A. Taatjes and D. E. Shallcross, Investigating the Atmospheric Sources and Sinks of Perfluorooctanoic Acid Using a Global Chemistry Transport Model, Atmosphere, 2020, 11, 407 CrossRef CAS.
- A. L. Ling, Estimated scale of costs to remove PFAS from the environment at current emission rates, Sci. Total Environ., 2024, 918, 170647 CrossRef CAS PubMed.
- L.-P. Sung, S. Vicini, D. L. Ho, L. Hedhli, C. Olmstead and K. A. Wood, Effect of microstructure of fluorinated acrylic coatings on UV degradation testing, Polymer, 2004, 45, 6639–6646 CrossRef CAS.
- M. A. M. Mahmoud, A. Kärrman, S. Oono, K. H. Harada and A. Koizumi, Polyfluorinated telomers in precipitation and surface water in an urban area of Japan, Chemosphere, 2009, 74, 467–472 CrossRef CAS PubMed.
- T. Portolés, L. E. Rosales, J. V. Sancho, F. J. Santos and E. Moyano, Gas chromatography–tandem mass spectrometry with atmospheric pressure chemical ionization for fluorotelomer alcohols and perfluorinated sulfonamides determination, J. Chromatogr. A, 2015, 1413, 107–116 CrossRef PubMed.
- J. F. Ayala-Cabrera, F. Javier Santos and E. Moyano, Negative-ion atmospheric pressure ionisation of semi-volatile fluorinated compounds for ultra-high-performance liquid chromatography tandem mass spectrometry analysis, Anal. Bioanal. Chem., 2018, 410, 4913–4924 CrossRef CAS PubMed.
- X. Hua, J. Luo, Z. Zhao, Q. Wang and H. Sun, Neutral polyfluoroalkyl and perfluoroalkyl substances in surface water and sediment from the Haihe River and Dagu Drainage Canal deserve more attention, Environ. Sci. Pollut. Res., 2019, 26, 32911–32918 CrossRef CAS PubMed.
- J. F. Ayala-Cabrera, E. Moyano and F. J. Santos, Gas chromatography and liquid chromatography coupled to mass spectrometry for the determination of fluorotelomer olefins, fluorotelomer alcohols, perfluoroalkyl sulfonamides and sulfonamido-ethanols in water, J. Chromatogr. A, 2020, 1609, 460463 CrossRef CAS PubMed.
- C. Chen, J. Wang, L. Li, W. Xu and J. Liu, Comparison of fluorotelomer alcohol emissions from wastewater treatment plants into atmospheric and aquatic environments, Environ. Int., 2020, 139, 105718 CrossRef CAS PubMed.
- S. Taniyasu, L. W. Y. Yeung, H. Lin, E. Yamazaki, H. Eun, P. K. S. Lam and N. Yamashita, Quality assurance and quality control of solid phase extraction for PFAS in water and novel analytical techniques for PFAS analysis, Chemosphere, 2022, 288, 132440 CrossRef CAS PubMed.
- A. Habib, E. N. Landa, K. L. Holbrook, W. S. Walker and W.-Y. Lee, Rapid, efficient, and green analytical technique for determination of fluorotelomer alcohol in water by stir bar sorptive extraction, Chemosphere, 2023, 338, 139439 CrossRef CAS PubMed.
- C. Bach, V. Boiteux, J. Hemard, A. Colin, C. Rosin, J.-F. Munoz and X. Dauchy, Simultaneous determination of perfluoroalkyl iodides, perfluoroalkane sulfonamides, fluorotelomer alcohols, fluorotelomer iodides and fluorotelomer acrylates and methacrylates in water and sediments using solid-phase microextraction-gas chromatography/mass spectrometry, J. Chromatogr. A, 2016, 1448, 98–106 CrossRef CAS PubMed.
- X. Dauchy, V. Boiteux, C. Bach, A. Colin, J. Hemard, C. Rosin and J.-F. Munoz, Mass flows and fate of per- and polyfluoroalkyl substances (PFASs) in the wastewater treatment plant of a fluorochemical manufacturing facility, Sci. Total Environ., 2017, 576, 549–558 CrossRef CAS PubMed.
- H. Chen, H. Peng, M. Yang, J. Hu and Y. Zhang, Detection, Occurrence, and Fate of Fluorotelomer Alcohols in Municipal Wastewater Treatment Plants, Environ. Sci. Technol., 2017, 51, 8953–8961 CrossRef CAS PubMed.
- C. Ma, H. Peng, H. Chen, W. Shang, X. Zheng, M. Yang and Y. Zhang, Long-term trends of fluorotelomer alcohols in a wastewater treatment plant impacted by textile manufacturing industry, Chemosphere, 2022, 299, 134442 CrossRef CAS PubMed.
- B. A. Schumacher, J. H. Zimmerman, A. C. Williams, C. C. Lutes, C. W. Holton, E. Escobar, H. Hayes and R. Warrier, Distribution of Select Per- and Polyfluoroalkyl Substances at a Chemical Manufacturing Plant, J. Hazard. Mater., 2023, 464, 133025 CrossRef PubMed.
- T. J. Smallwood, N. M. Robey, Y. Liu, J. A. Bowden, T. M. Tolaymat, H. M. Solo-Gabriele and T. G. Townsend, Per- and polyfluoroalkyl substances (PFAS) distribution in landfill gas collection systems: leachate and gas condensate partitioning, J. Hazard. Mater., 2023, 448, 130926 CrossRef CAS PubMed.
- Y. Chen, H. Zhang, Y. Liu, J. A. Bowden, T. M. Tolaymat, T. G. Townsend and H. M. Solo-Gabriele, Evaluation of per- and polyfluoroalkyl substances (PFAS) in leachate, gas condensate, stormwater and groundwater at landfills, Chemosphere, 2023, 318, 137903 CrossRef CAS PubMed.
- B. M. Allred, J. R. Lang, M. A. Barlaz and J. A. Field, Physical and Biological Release of Poly- and Perfluoroalkyl Substances (PFASs) from Municipal Solid Waste in Anaerobic Model Landfill Reactors, Environ. Sci. Technol., 2015, 49, 7648–7656 CrossRef CAS PubMed.
- J. R. Lang, B. M. Allred, J. A. Field, J. W. Levis and M. A. Barlaz, National Estimate of Per- and Polyfluoroalkyl Substance (PFAS) Release to U.S. Municipal Landfill Leachate, Environ. Sci. Technol., 2017, 51, 2197–2205 CrossRef CAS PubMed.
- N. T. Joseph, T. Schwichtenberg, D. Cao, G. D. Jones, A. E. Rodowa, M. A. Barlaz, J. A. Charbonnet, C. P. Higgins, J. A. Field and D. E. Helbling, Target and Suspect Screening Integrated with Machine Learning to Discover Per- and Polyfluoroalkyl Substance Source Fingerprints, Environ. Sci. Technol., 2023, 57, 14351–14362 CrossRef CAS PubMed.
- W. M. Henderson, M. G. Evich, J. W. Washington, T. T. Ward, B. A. Schumacher, J. H. Zimmerman, Y. D. Kim, E. J. Weber, A. C. Williams, M. G. Smeltz and D. A. Glinski, Analysis of Legacy and Novel Neutral Per- and Polyfluoroalkyl Substances in Soils from an Industrial Manufacturing Facility, Environ. Sci. Technol., 2024, 58, 10729–10739 CrossRef CAS PubMed.
- C. P. Goossen, R. E. Schattman and J. D. MacRae, Evidence of compost contamination with per- and polyfluoroalkyl substances (PFAS) from “compostable” food serviceware, Biointerphases, 2023, 18, 030501 CrossRef CAS PubMed.
- G. W. Link, D. M. Reeves, D. P. Cassidy and E. S. Coffin, Per- and Polyfluoroalkyl Substances (PFAS) in Final Treated Solids (Biosolids) from 190 Michigan Wastewater Treatment Plants, J. Hazard. Mater., 2023, 463, 132734 CrossRef PubMed.
- K. Rankin, H. Lee, P. J. Tseng and S. A. Mabury, Investigating the Biodegradability of a Fluorotelomer-Based Acrylate Polymer in a Soil–Plant Microcosm by Indirect and Direct Analysis, Environ. Sci. Technol., 2014, 48, 12783–12790 CrossRef CAS PubMed.
- J. W. Washington, J. E. Naile, T. M. Jenkins and D. G. Lynch, Characterizing Fluorotelomer and Polyfluoroalkyl Substances in New and Aged Fluorotelomer-Based Polymers for Degradation Studies with GC/MS and LC/MS/MS, Environ. Sci. Technol., 2014, 48, 5762–5769 CrossRef CAS PubMed.
- J. W. Washington, T. M. Jenkins, K. Rankin and J. E. Naile, Decades-Scale Degradation of Commercial, Side-Chain, Fluorotelomer-Based Polymers in Soils and Water, Environ. Sci. Technol., 2015, 49, 915–923 CrossRef CAS PubMed.
- J. W. Washington, K. Rankin, E. L. Libelo, D. G. Lynch and M. Cyterski, Determining global background soil PFAS loads and the fluorotelomer-based polymer degradation rates that can account for these loads, Sci. Total Environ., 2019, 651, 2444–2449 CrossRef CAS PubMed.
- M. H. Russell, W. R. Berti, B. Szostek and R. C. Buck, Investigation of the Biodegradation Potential of a Fluoroacrylate Polymer Product in Aerobic Soils, Environ. Sci. Technol., 2008, 42, 800–807 CrossRef CAS PubMed.
- J. W. Washington, J. J. Ellington, T. M. Jenkins, J. J. Evans, H. Yoo and S. C. Hafner, Degradability of an Acrylate-Linked, Fluorotelomer Polymer in Soil, Environ. Sci. Technol., 2009, 43, 6617–6623 CrossRef CAS PubMed.
- M. H. Russell, W. R. Berti, B. Szostek, N. Wang and R. C. Buck, Evaluation of PFO formation from the biodegradation of a fluorotelomer-based urethane polymer product in aerobic soils, Polym. Degrad. Stab., 2010, 95, 79–85 CrossRef CAS.
- J. Chen, L. Tang, W.-Q. Chen, G. F. Peaslee and D. Jiang, Flows, Stock, and Emissions of Poly- and Perfluoroalkyl Substances in California Carpet in 2000–2030 under Different Scenarios, Environ. Sci. Technol., 2020, 54, 6908–6918 CrossRef CAS PubMed.
- D. Jiang and T. Zhang, State-level material flow analysis suggests the need to reconsider current monitoring practice and mitigation policies for poly-
and perfluoroalkyl substances in carpet, J. Ind. Ecol., 2022, 26, 815–823 CrossRef.
- J. B. Pollack, I. Q. Carey and V. Y. Xu, Regulation of Products with PFAS, Environ. Law Rep., 2024, 54, 10148 Search PubMed.
-
C. Hogue, California bans cosmetics and apparel with PFAS, https://cendigitalmagazine.acs.org/2022/10/09/california-bans-cosmetics-and-apparel-with-pfas-3/content.html, accessed 10 October 2022 Search PubMed.
- M. Dunn, N. Noons, S. Vojta, J. Becanova, H. Pickard, E. M. Sunderland and R. Lohmann, Unregulated Active and Closed Textile Mills Represent a Significant Vector of PFAS Contamination into Coastal Rivers, ACS ES&T Water, 2024, 4, 114–124 Search PubMed.
- R. Bakhshoodeh and R. M. Santos, Comparative bibliometric trends of microplastics and perfluoroalkyl and polyfluoroalkyl substances: how these hot environmental remediation research topics developed over time, RSC Adv., 2022, 12, 4973–4987 RSC.
- H. Tian, C. Gaines, L. Launi, A. Pomales, G. Vazquez, A. Goharian, B. Goodnight, E. Haney, C. M. Reh and R. D. Rogers, Understanding Public Perceptions of Per- and Polyfluoroalkyl Substances: Infodemiology Study of Social Media, J. Med. Internet Res., 2022, 24, e25614 CrossRef PubMed.
- T. A. Berthold, A. McCrary, S. deVilleneuve and M. Schramm, Let's talk about PFAS: Inconsistent public awareness about PFAS and its sources in the United States, PLoS One, 2023, 18, e0294134 CrossRef CAS PubMed.
- C. Moody, EPA Finalizes Standards for Six PFAS, J. AWWA, 2024, 116, 8 CrossRef.
- Z. Wang, I. T. Cousins, M. Scheringer, R. C. Buck and K. Hungerbühler, Global emission inventories for C4–C14 perfluoroalkyl carboxylic acid (PFCA) homologues from 1951 to 2030, Part I: production and emissions from quantifiable sources, Environ. Int., 2014, 70, 62–75 CrossRef CAS PubMed.
- Z. Wang, I. T. Cousins, M. Scheringer, R. C. Buck and K. Hungerbühler, Global emission inventories for C4–C14 perfluoroalkyl carboxylic acid (PFCA) homologues from 1951 to 2030, part II: The remaining pieces of the puzzle, Environ. Int., 2014, 69, 166–176 CrossRef CAS PubMed.
- H. Holmquist, S. Roos, S. Schellenberger, C. Jönsson and G. Peters, What difference can drop-in substitution actually make? A life cycle assessment of alternative water repellent chemicals, J. Cleaner Prod., 2021, 329, 129661 CrossRef CAS.
- Y. Cheng, S. Wang, Z. Xu, L. Jiang and Y. Zhao, Non-fluorine oil repellency: To what extent can it substitute perfluoroalkyl substances?, Prog. Org. Coat., 2023, 183, 107726 CrossRef CAS.
- G. Zheng and A. Salamova, Are Melamine and Its Derivatives the Alternatives for Per- and Polyfluoroalkyl Substance (PFAS) Fabric Treatments in Infant Clothes?, Environ. Sci. Technol., 2020, 54, 10207–10216 CrossRef CAS PubMed.
- L. Zhi, H. Sun, L. Xu and Y. Cai, Distribution and Elimination of Trifluoropropylmethylsiloxane Oligomers in Both Biosolid-Amended Soils and Earthworms, Environ. Sci. Technol., 2021, 55, 985–993 CrossRef CAS PubMed.
- M. S. McLachlan, A. Kierkegaard, M. Radke, A. Sobek, A. Malmvärn, T. Alsberg, J. A. Arnot, T. N. Brown, F. Wania, K. Breivik and S. Xu, Using Model-Based Screening to Help Discover Unknown Environmental Contaminants, Environ. Sci. Technol., 2014, 48, 7264–7271 CrossRef CAS PubMed.
- T. P. Riedel, M. A. G. Wallace, E. P. Shields, J. V. Ryan, C. W. Lee and W. P. Linak, Low temperature thermal treatment of gas-phase fluorotelomer alcohols by calcium oxide, Chemosphere, 2021, 272, 129859 CrossRef CAS PubMed.
- E. P. Shields and M. A. G. Wallace, Low temperature destruction of gas-phase per- and polyfluoroalkyl substances using an alumina-based catalyst, J. Air Waste Manage. Assoc., 2023, 763, 525–532 CrossRef PubMed.
- J. M. Parrilla, ChatGPT use shows that the grant-application system is broken, Nature, 2023, 623, 443 CrossRef CAS PubMed.
|
This journal is © The Royal Society of Chemistry 2024 |
Click here to see how this site uses Cookies. View our privacy policy here.