Fluorine pollution in a sheep fluorosis area of the northern Helan Mountains, Ningxia, China
Received
6th September 2023
, Accepted 13th November 2023
First published on 14th November 2023
Abstract
The relationship between endemic sheep fluorosis and coal mining and combustion in Helan Mountain, Ningxia, China, is lacking clear research and understanding. A total of 137 samples of topsoil and grass were collected in this area and fluorine, sulfate, and pH levels were determined. The mean fluorine content in topsoil is 707 ± 126 μg g−1 (n = 77), which is significantly higher than that of standard Chinese topsoil. The mean fluorine content of pasture is 218 ± 42 μg g−1 (n = 28), which suggests that sheep fluorosis may be associated with high fluorine levels in topsoil and pasture. Sulfate content in topsoil is 1771 ± 130 μg g−1 (n = 77) and pH is 5.92 ± 0.82. The pH and sulfate content of acidic topsoil are positively correlated (r = 0.72), which implies that the acidity may be due to the presence of acidic sulfates, such as NaHSO4 or KHSO4. High fluorine, sulfate, and acidity may be connected with the long history of coal mining, coal use, and coal combustion, where deposition of fluoride results in coexistence with acidic sulfate.
Environmental significance
Spontaneous combustion of coal fires and ecological damage caused by coal mining have led to a series of environmental pollution problems, including soil pollution, with heavy metal enrichment pollution being the most serious. This study confirms the relationship between fluorine pollution and coal release mechanisms in coal mining areas through sampling monitoring and experimental validation of soil fluorine pollution around a typical coal mining site in the Helan Mountains of Ningxia, China, in the hope of providing a basis and an experimental method for soil protection. This approach has great potential for environmental monitoring and ecological protection in mining areas and is of great significance for the promotion of global sustainable development, especially in the field of energy extraction and environmental protection in mining areas.
|
1. Introduction
Improper use of coal can cause fluorine accumulation in humans and organisms. There are three types of endemic fluorosis suffered by humans, which are caused by pollution from coal combustion and drinking high-fluorine brick tea and high-fluorine water. Fluorosis caused by coal burning is unique to China, where it is mainly distributed in the southwest of the country. Such fluorosis is closely related to the use of coal, and its pathogenesis has attracted attention both nationally and internationally.1–7
Although many consider that fluorine is an essential trace element for animal growth the Scientific Committee on Health and Environmental Risks (SCHER), Directorate General for Health Consumers, European Commission, gave an opinion in a critical review of any new evidence on the hazard profile, health effects, and human exposure to fluoride and the fluoridating agents of drinking water8 that fluorine is not an essential trace element for human growth and development. Excess fluoride intake can cause disease or even death in animals. Animal fluorosis is common globally. In 1932, H. Velu diagnosed fluorosis as chronic poisoning that can occur in both humans and animals.9 Near a coal-fired power plant in Germany, a large number of bees died due to a high fluoride concentration in plants.10 The first case of chronic fluorosis in cattle in Guangxi, China, is suspected to have been caused by industrial waste gas that polluted pasture areas.9 Fluorine poisoning in livestock in Baotou City, Inner Mongolia, China, is extremely serious. Nearly one million goats are affected each year due to industrial fluorine pollution; fluorine-containing fumes are emitted by metallurgical industries, such as steel-making and aluminum smelting, in the region.11 Chronic fluorosis in sheep in Haiyan County, Qinghai Province, China, is also caused by fluoride emissions from local aluminum manufacturers.12 In the past, due to a lack of effective environmental protection measures in indigenous metallurgy industries, a large amount of fluoride was released into the atmosphere, causing serious pollution to the surrounding environment, thereby resulting in chronic animal fluorosis.
The Helan Mountains in Ningxia, China, are a typical area of sheep fluorosis. In recent years, chronic fluorosis, which is characterized by broken teeth and broken rib nodules, has become increasingly common in sheep in this area.13 In addition, the closer the proximity to a mining area, the higher the morbidity. The region is a mountainous area. Although there are no large smelting industries, chronic fluorosis in sheep has been found, the cause of which needs investigation. The area is steep with exposed rock and complex geomorphology, and the soil in valley bottoms is mainly aeolian soil, from the Loess plateau. The Loess Plateau has a carbonate weathering crust, and soils are generally alkaline with a pH above 8.3.14 This study focuses on the topsoil and plants in the northern part of the Helan Mountains, to study the geochemical background of fluorine in the area, in order to deepen the understanding of fluorosis.
2. Materials and methods
2.1 Study area
The Helan Mountains are located at the junction of Ningxia and Inner Mongolia. The area is bordered by the Yinchuan Plain in the east and Ala Shan Desert in the west. The climate is characterized by a strongly continental, fully arid climate with an annual precipitation of 200–400 mm. A prevailing northwesterly wind is observed in this area, with a maximum beau fort scale of more than ten.15
The northern part of the Helan Mountains is rich in coal resources and is the main coal producing area of Ningxia and Inner Mongolia. The coalfield area is about 40 km2, with a reserve of 1.5 billion.16 Industrialized coal mining in the Helan Mountains was initiated in the 1950s, and since then it has grown into a typical mining area, with the emergence of coal mines of various sizes (Fig. 1). Rujigou coal mine is a large-scale coal mine in the region and is the famous “Taixi coal” production base in China. At the end of the last century, underground mining conditions deteriorated with the extension of coal mining. In recent years, large-scale coal mining activities have led to increased exposure of the coal seam, thereby accelerating spontaneous combustion.17 In the early 21st century, exploration by remote sensing showed that a total of 24 fire areas were found in the Rujigou mining area, covering a total area of 3.32 km2.18 Since 2008, the Chinese government has paid great attention to controlling coal fires in this area, but has only combated visible surface flames; therefore the risk of spreading remains.19 Over the past 30 years, the development and utilization of coal mines in this area have increased.
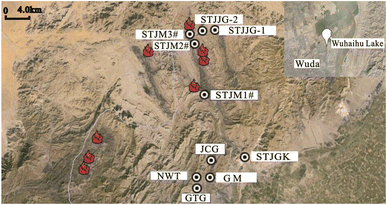 |
| Fig. 1 Distribution of sample points (the red areas are typical of coal mining in Helan Mountain). | |
2.2 Sample collection
2.2.1 Topsoil in the study area.
Samples were collected in the northern Helan Mountains from North Wudang Temple (NWT), Jiucaigou (JCG), the Geological Museum (GM), Shitanjing Goukou (STJGK), Shitanjing Jiangou (STJJG), Shitanjing Mine 1# (STJM1#), Shitanjing Mine 2# (STJM2#), and Shitanjing Mine 3# (STJM3#) (Fig. 1). A total of 137 samples, including topsoil and pasture, were taken. Topsoil samples comprise mainly aeolian soil.
2.2.2 Topsoil in the background area and control area.
At the same time, 11 control samples were collected from the background area-the island in Wuhai Lake (39° 31′N, 106° 44′E, Fig. 1), which is adjacent to the study area. Wuhai Lake is man-made, and the island was formed by stacking while building the lake. The island is adjacent to the Yellow River and has no industrial pollution. The topsoil of the Yellow River is alluvial soil and is formed from the upper reaches of the Loess Plateau.
In addition, a total of 69 topsoil samples were taken within 6 areas (Weifang in Shandong, the Daxing district in Beijing, Qinhuangdao in Hebei, Yuncheng in Shanxi, Huludao in Liaoning and Chifeng in Inner Mongolia), which are the control areas of this study. Sampling in the background area and control areas was conducted in accordance with those in the study area.
2.3 Analytical methods
2.3.1 Determination of pH.
The acidity of topsoil was determined using a pH electrode (Mode METTLER-SG2), which was corrected using three kinds of standard pH buffer solution (at a pH of 4.01, 7.00, and 9.02, provided by the METTLER TOLEDO company) before taking measurements.
2.3.2 Determination of sulfate.
The total sulfate in topsoil was determined by ion chromatography (DX-120), equipped with a Dionex AS14 (250 × 4 mm) anion exchange column and a conductivity detector. The mobile phase was composed of 6.3 mM Na2CO3 and 1.7 mM NaHCO3 at a rate of 1.9 mL min−1. Samples were filtered through 0.25 mm filters before injection. For quality control, the standard reference materials (GSB04-2080-2007), with a reference value of 1000 μg g−1 and determination value of 948 ± 69 μg g−1 (n = 11) were used.
2.3.3 Determination of total fluorine in topsoil.
Fluorine (F) in topsoil was determined by the combustion-hydrolysis fluoride ion-selective electrode method in accordance with the Chinese National Standards (GB4633-1997). For quality control, standard reference materials (GBW07403 [soil, China] and GBW07406 [soil, China]) were randomly analyzed with each batch of soil samples.
2.3.4 Determination of total fluorine in pasture.
Fluorine (F) in pasture was determined by the use of the oxygen flask combustion method with a catalyst.20 0.03 g powdered pasture, 0.01 g catalyst (WO3) and 0.005 g co-catalyst (Sn) were precisely weighed, mixed and transferred on a 40 × 40 (mm) ash-free filter paper. After being folded into a cylindrical shape, the paper with samples was inserted into the platinum cage attached to the flask plug. The fuse was ignited, and the samples were combusted in the usual way in a 500 mL oxygen-filled quartz flask containing 5 mL of water. After the combustion, the flask was shaken for 2 min and allowed to stand for 1 h. In Fig. 2, schematic representation of the OFC procedure is presented. And the pH was adjusted to 5–7. TISAB solution (5 mL) was added, and the volume of the solution was made up to 25 mL. After shaking, the solution was left to stand for more than 30 min, and the fluoride ions in the solution were measured with a composite fluorine ion electrode as mentioned above. The accuracy of the measurements was assessed using the addition method for samples. Recoveries of addition samples were 95.48% and the method repeatability was tested by analyzing five replicates of one soil sample. The coefficient of variation in the coal replicates was 2.26%.
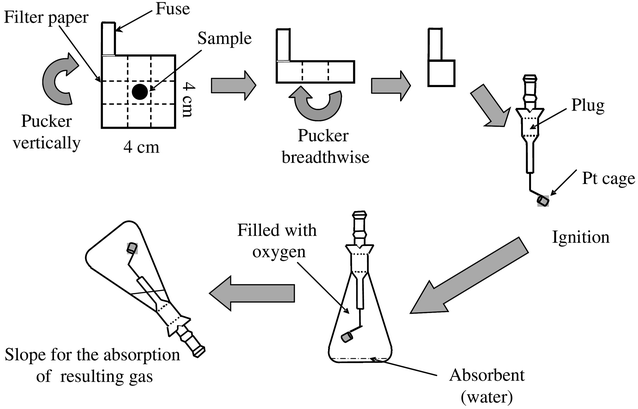 |
| Fig. 2 Procedure of the oxygen flask combustion method. | |
3. Results and discussion
3.1 Fluorine in topsoil
The overall average fluorine content of topsoil in the study area is 704 μg g−1 (439–964 μg g−1) (Table 1). Fluorine content differs across locations. For example, the average fluorine concentration is 799 μg g−1 (677–911 μg g−1, n = 9) in STJM1#, 790 μg g−1 (668–944 μg g−1, n = 10) in STJM2#, and 796 μg g−1 (608–964 μg g−1, n = 18) in STJGK, while that in NWT and JCG is relatively low, averaging 594 μg g−1 and 598 μg g−1 respectively. In general, fluorine concentrations in soil decrease with increasing distance from the mining area.
Table 1 pH, sulfate and fluorine content in topsoil
Location |
Number |
F (μg g−1) |
SO42− (μg g−1) |
pH |
Range |
Average |
SD |
Range |
Average |
SD |
Range |
Average |
SD |
NWT |
6 |
546–679 |
598 |
45 |
897–1459 |
1263 |
338 |
6.03–7.08 |
6.69 |
0.44 |
JCG |
10 |
439–696 |
594 |
83 |
693–2024 |
1267 |
426 |
5.81–7.01 |
6.37 |
0.35 |
GM |
6 |
566–779 |
662 |
79 |
667–1301 |
869 |
231 |
6.47–7.49 |
7.00 |
0.37 |
STJGK |
18 |
608–964 |
796 |
109 |
1543–3318 |
2593 |
581 |
4.65–5.86 |
5.16 |
0.37 |
STJM2# |
10 |
668–944 |
790 |
98 |
1465–4023 |
2752 |
681 |
4.35–6.09 |
5.2 |
0.73 |
STJM1# |
9 |
677–911 |
799 |
83 |
953–2276 |
1544 |
594 |
4.98–6.78 |
5.97 |
0.61 |
STJM3# |
5 |
522–694 |
610 |
81 |
714–1067 |
959 |
143 |
6.62–7.51 |
7.03 |
0.31 |
STJJG1# |
7 |
443–792 |
619 |
120 |
623–2819 |
1647 |
889 |
5.6–6.24 |
5.95 |
0.2 |
STJJG2# |
6 |
769–1331 |
645 |
93 |
769–1331 |
1079 |
240 |
5.93–7.09 |
6.3 |
0.41 |
Total |
77 |
439–964 |
704 |
127 |
714–4023 |
1771 |
873 |
4.35–7.49 |
5.96 |
0.81 |
The mean value of fluorine concentrations in topsoil of the study area is higher than concentrations in the background area (Wuhaihu Island; 240 μg g−1) (Table 4), control areas in China (Table 3), and the Ningxia plain adjacent to the study area (511 μg g−1).20,21 This shows that the topsoil has been polluted by fluorine to a certain degree and also indicates that there may be a fluorine emission source.
The standard value of fluorine concentration in pasture in China and Washington is about 40 μg g−1,22 and the background value of common pastures is in the range of 5.75–8.15 μg g−1.23 The overall average fluorine concentration in the study area reaches 216 μg g−1 (n = 27), ranging from 149 to 291 μg g−1 (Table 2); all values exceed the standard value of fluorine concentration in China.
Table 2 Fluorine content in pasture and sheep manure
Location |
Number |
F (μg g−1) |
Coordinate |
Sample number |
Range |
Average |
SD |
STJGK |
2 |
191–238 |
215 |
33 |
N: 39.09; E: 106.43 |
Pasture |
JCG |
7 |
149–241 |
194 |
29 |
N: 39.09; E: 106.36 |
GM |
1 |
221 |
221 |
|
N: 39.06; E: 106.36 |
NWT |
3 |
189–242 |
228 |
33 |
N: 39.06; E: 106.35 |
GTG |
3 |
150–291 |
224 |
70 |
N: 39.04; E: 106.33 |
STJJG |
6 |
157–236 |
193 |
30 |
N: 39.28; E: 106.37 |
STJM3# |
2 |
225–241 |
233 |
11 |
N: 39.28; E: 106.32 |
STJM1# |
1 |
271 |
271 |
|
N: 39.18; E: 106.35 |
STJJG |
2 |
50–63 |
57 |
9 |
N: 39.28; E: 106.37 |
Sheep manure |
STJM3# |
2 |
68–72 |
70 |
3 |
N: 39.28; E: 106.32 |
JCG |
1 |
49 |
49 |
|
N: 39.09; E: 106.36 |
STJM2# |
2 |
184–207 |
196 |
|
N: 39.17; E: 106.40 |
STJM1# |
6 |
189–267 |
239 |
85 |
N: 39.18; E: 106.35 |
Coal |
JCG |
11 |
165–302 |
228 |
44 |
N: 39.08; E: 106.36 |
Usually, a high-fluorine pasture is closely related to sources of fluorine pollution near the grassland. Fluorine in samples in the vicinity of a smelter reaches 24.5–149.5 μg g−1.23 The maximum value of fluorine concentration in pasture in the vicinity of the aluminum plant in Haibei, Qinghai, can reach up to 3431 μg g−1.24 A high fluorine concentration in pasture indicates that there may be fluorine pollution sources in the area. In addition, the fluorine concentration of sheep manure (50–72 μg g−1) is significantly higher than that in the typical fluorine-polluted zone of China (Baotou, Inner Mongolia; 7.6–31.5 μg g−1),25 which also suggests that the study area has undergone fluorine pollution.
3.2 Sulfate content in topsoil
The sulfate content in topsoil varies greatly (Table 3). The overall average concentration is 1771 μg g−1 (623–4023 μg g−1, n = 77). The topsoil in STJGK and STJM2# has the highest sulfate content (>2593 μg g−1), and that in STJM1#, STJJG, and NWT also exhibits high levels, reaching 1544 μg g−1, 1647 μg g−1, and 1263 μg g−1, respectively, while levels in GM and STJM3# are relatively low (Fig. 3). However, in general, the total sulfate content in topsoil in this region is at a high level.
Table 3 pH, sulfate and fluorine content of topsoil in control areas
Location |
Number |
F (μg g−1) |
SO42− (μg g−1) |
pH |
Average |
Range |
Average |
Range |
Average |
Range |
Weifang |
6 |
281 |
117–325 |
519 |
438–694 |
7.71 |
7.38–8.16 |
Linyi |
9 |
546 |
501–643 |
546 |
423–693 |
7.8 |
7.34–8.03 |
Shanhaiguan |
12 |
403 |
326–579 |
403 |
206–871 |
7.59 |
7.11–8.38 |
Beijing |
9 |
323 |
256–393 |
635 |
414–949 |
7.61 |
7.26–7.93 |
Yuncheng |
8 |
427 |
364–507 |
469 |
402–516 |
7.97 |
7.39–8.24 |
Huludao |
8 |
318 |
275–389 |
648 |
424–849 |
7.87 |
7.7–8.14 |
Chifeng |
18 |
430 |
368–479 |
522 |
261–758 |
8.02 |
7.51–8.34 |
Total |
70 |
404 |
117–721 |
543 |
206–949 |
7.81 |
7.01–8.48 |
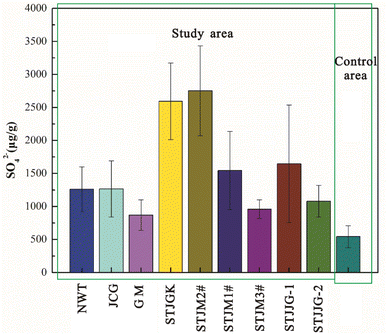 |
| Fig. 3 Comparison of sulfate content in the study area and control area. | |
The sulfate content of topsoil in the study area is higher than that of the control (543 μg g−1, Table 3) and background (561 μg g−1, Table 4) areas, which indicates elevated sulfate concentrations in topsoil in this area. Sulfate content in topsoil has not been widely reported. The average sulfate content in efflorescent clay in late Permian coal-bearing strata in border areas of Yunnan, Guizhou, and Sichuan is 1778 μg g−1. The minimum value of sulfate content in topsoil of Zhijin in Guizhou is 1665 μg g−1.26,27 These values are equivalent to the overall average of sulfate in the topsoil in this study.
Table 4 pH, sulfate and fluorine content in topsoil on the island of Wuhai Lake
No. |
F (μg g−1) |
SO42− (μg g−1) |
pH |
No. |
F (μg g−1) |
SO42− (μg g−1) |
pH |
H1-1 |
256 |
449 |
8.72 |
I4 |
176 |
645 |
8.68 |
H1-2 |
293 |
490 |
8.75 |
I6 |
298 |
834 |
7.82 |
H1-3 |
276 |
534 |
8.43 |
T8 |
323 |
802 |
7.6 |
H1-4 |
264 |
612 |
8.62 |
Min |
186 |
389 |
8.03 |
H2-1 |
198 |
498 |
8.31 |
Max |
293 |
612 |
8.75 |
H2-2 |
237 |
510 |
8.52 |
Average |
240 |
487 |
8.44 |
H2-3 |
214 |
389 |
8.12 |
SD |
38 |
71 |
0.27 |
H2-4 |
186 |
412 |
8.03 |
3.3 Acidity
The acidity of topsoil is represented by its pH (Table 1). The pH of topsoil in the study area ranges from 4.35 to 7.72, with an overall average of 5.92 (n = 77). In general, the acidity of topsoil in STJGK and STJM2# is the strongest, with a pH of around 5.00 (minimum value 4.35), followed by that in STJJG-1 and STJJG-2, with pH of 5.95 and 5.97, respectively, while that near GM and STJM3# is the weakest and almost alkaline (Fig. 4). Acidic soil (pH < 6.5) dominates the study area, accounting for 76.6% of the total sample, while neutral (6.5 < pH < 7.0) and alkaline (pH > 7) soil accounts for a relatively small proportion (Fig. 5). This indicates that topsoil pH in the study area varies greatly, with both strongly acidic and slightly alkaline soil present.
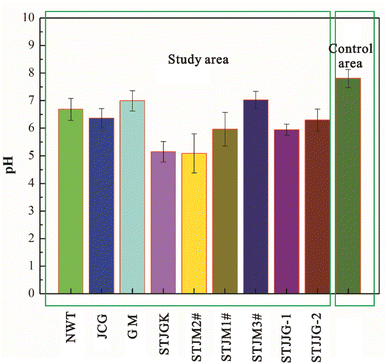 |
| Fig. 4 pH comparison of topsoil in the study area and control area. | |
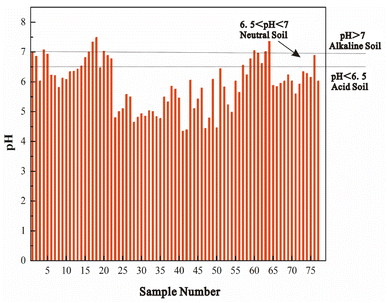 |
| Fig. 5 pH distribution of topsoil. | |
By contrast, the pH of topsoil in northern China ranges from 7.01 to 8.38, with an average of 7.81 (n = 69), and that in the control area ranges from 7.6 to 8.75, with an average of 8.44 (Fig. 4), which is close to the average pH of soil on the Loess Plateau (pH = 8.30).13 Topsoil acidity in the study area is significantly stronger than that in the background and control areas, indicating acid pollution in the study area.
Soil acidity in northwestern China has been widely reported, with results showing that the soil in the area is mostly alkaline. In our study, the average topsoil pH is as low as 5.92 and is significantly lower than that found in Pingluo county, Ningxia (pH = 8.63),28 Longde county, Ningxia (pH = 8.28–8.36),29 and Lanzhou, China (pH = 8.39).30 Although there are some alkaline soil samples (pH > 7), acidity is still higher than the local background value (pH = 8.44), which is good evidence of acid contamination in the study area.
3.4 Acidity and sulfate
In order to explore the possible existence of acid in topsoil, acidic topsoil (pH ≤ 5.6) was selected, and the negative logarithm of sulfate content (−lg
C(SO42−), namely p(SO42−)) was calculated according to the chemical equilibrium principle. Here, C(SO42−) was converted in relation to the sulfate content (μg g−1) in Table 1 (the unit is mol L−1 in line with the volumetric method). There is a significant positive correlation between p1(SO42−) and pH (r = 0.72; Fig. 6).
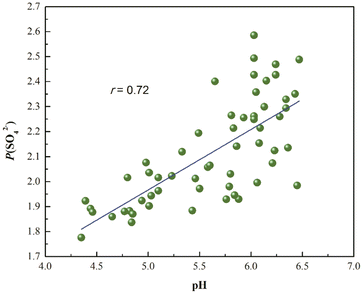 |
| Fig. 6 Relationship between pH and p(SO42−) in acidic topsoil. | |
This suggests that the high sulfate content in the study area is likely in the form of hydrogen sulfate (HSO4−). Since fluorine under alkaline conditions mainly exists in the form of free F-, when the soil environment is reduced by pH, F- is involved in the conversion to AlF2+, which is more easily enriched in the soil,31 indicating that the content of acid, sulfate and fluorine in the topsoil of the study area is relatively rich, which may be related.
3.5 Discussion
In contrast to the sheep fluorosis areas in Haibei, Qinghai and Baotou, Inner Mongolia, there are no known industries emitting fluorine (such as aluminum or rare earth smelting) in the northern Helan Mountains, although coal mining occurs. There is a long history of coal exploitation and domestic coal burning, along with spontaneous combustion of coal caused by coal exploitation. Coal has been mined in Rujigou for 191 years. Coal fires may date back to the Ming and Qing dynasties, over 300 years ago.32
Sulfur and fluorine are common in coal. Spontaneous combustion and use of coal emit SO2 and SO3, which are oxidized to H2SO4 through atmospheric processes. H2SO4 is eventually deposited in the form of sulfate.33 Coal-burning is undoubtedly accompanied by fluorine emissions and sedimentation. The northern Helan Mountains are rich in low-sulfur coal, such as the famous “Taixi coal”, and low fluorine coal (165–302 μg g−1, Table 2). There are no reports of high fluoride coal.34 However, in general, there is a long history of SO2 emission in this region, followed by a long history of sulfuric acid or sulfate wet-dry deposition and fluorine emission, which has eventually led to significantly elevated fluorine and sulfate concentrations and acidic soils (Fig. 7).
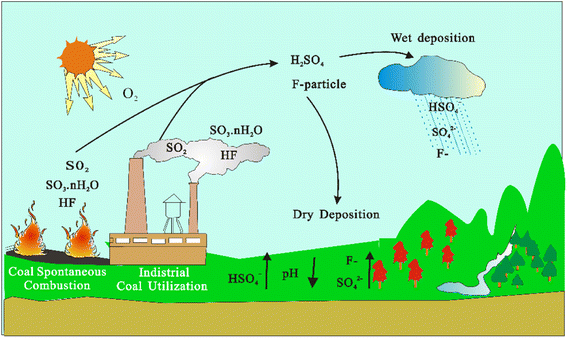 |
| Fig. 7 The diagram of the main sources and pathways of fluorine, sulfate and acidity in the study area. | |
Long-term coal mining, along with the burning and use of coal, increases the acidification of surface soils. It has been shown that the weathering of clay-pyrite in coal produces HSO4− and sulfate, which further promote the release of fluoride from the clay.35 These may be the root cause of the high concentration of fluoride, sulfate and acid in the surface soil of the northern Helan Mountains, resulting in fluorosis in sheep in this area.
4. Conclusions
This study verifies that not only fluorine and sulfate content, but also acidity of topsoil, in the northern Helan Mountains are significantly higher than those in control areas. The fluorine content in pasture is also higher than background values and the fluorine standard in China. This may be due to the long history of coal mining and coal spontaneous combustion in the region, which should be further investigated in the future.
Author contributions
Y. K. and H. X. P. designed the experiments and wrote the original draft. H. D. L. prepared the experimental materials. All authors have read and agreed to the published version of the manuscript.
Conflicts of interest
The authors declare that they have no clear financial interests or personal relationships that could have emerged to affect the work reported in this paper.
Acknowledgements
This research was supported by the Natural Science Research Project of Colleges and Universities in Anhui Province (No. 2022AH050375) and the National Natural Science Foundation of China (Number: 41371449).
References
- B. S. Zheng, J. Zhang, Z. H. Ding, J. M. Zhu, X. Y. Yu, A. M. Wang, D. X. Zhou, D. J. Mao and H. C. Su, Issues of health and disease relating to coal use in southwestern China, Int. J. Coal Geol., 1999, 40, 119–132 CrossRef CAS.
- K. L. Luo, L. Li and S. X. Zhang, Coal-burning roasted corn and chili as the cause of dental fluorosis for children in southwestern China, J. Hazard. Mater., 2011, 185, 1340–1347 CrossRef CAS PubMed.
- S. F. Dai, D. Y. Ren and S. M. Ma, The cause of endemic fluorosis in western Guizhou province, Southwest China, Fuel, 2004, 83, 2095–2098 CrossRef CAS.
- R. B. Finkelman, W. Orem, V. Castranova, C. A. Tatu, H. E. Belkin and B. S. Zheng,
et al., Health impacts of coal and coal use: Possible solutions, Int. J. Coal Geol., 2002, 50, 425–433 CrossRef CAS.
- D. S. Wu, B. S. Zheng, A. M. Wang, G. Q. Yu and Y. Gui, Fluoride exposure from burning coal-clay in Guizhou province, China, Fluoride, 2004, 37, 20–27 CAS.
- X. P. Hong, H. D. Liang, Y. F. Zhang and D. M. Xu, Distribution of acidity of late Permian coal in border area of Yunnan, Guizhou and Sichuan, J. China Coal Soc., 2016, 41, 964–973 CAS.
- X. P. Hong, H. D. Liang and S. Lv, Release of hydrogen fluoride from clay used for coal-combustion in Zhijin County, Guizhou Province, Fluoride, 2017, 50, 152–162 Search PubMed.
-
A. Scoggins, H. D. Vries, A. Conklin, et al., Prepared for the European Commission, Directorate General for Health and Consumers, 2011, pp. 2–4 Search PubMed.
- K. R. Li, Overview of poultry fluoride poisoning, Guangxi Journal of Animal Husbandry & Veterinary Medicine, 1999, 5, 35–37 Search PubMed.
-
P. Chen, Fluorine in coal of China, Coal Geology of China, 2002, vol. 14, pp. 25–28 Search PubMed.
- S. Z. Yi, Z. X. Fang and F. Jin, Treatment Technology of Fluorine-containing Flue Gas in Smelting of Rare Earth Ore, J. Tianjin Univ. Sci. Technol., 2012, 27, 74–79 CAS.
- M. R. Yun, P. Yun and X. L. Gao, The pollution changing tendency and treatment effectiveness analysis of fluoride in the air of Baotou city, Inner Mongolia Environmental Protection, 2005, 17, 17–19 Search PubMed.
-
B. J. Ma and H. X. Si, Fluorosis and fluoride pollution in Shizuishan area, Ningxia, China, Heilongjiang Animal Science and Veterinary Medicine, 2010 Search PubMed.
- H. Y. Jia, P. H. Cheng, S. P. Yong, X. M. Mu, J. M. Cheng and X. L. Peng, Characteristics and ecological environment of soil chemical elements in grassland of Loess plateau, China, J. Soil Water Conserv., 1995, 9, 84–93 Search PubMed.
- B. R. Liu, X. Z. Zhang, T. H. Hu and W. J. Li, Soil microbial diversity under typical vegetation zones along an elevation gradient in Helan Mountains, Acta Ecol. Sin., 2013, 33, 7211–7220 CrossRef CAS.
- P. M. Ding, Coal areas in Helan mountains, Ningxia, China, Bull. Geol. Soc. China, 1960, 40, 89–109 Search PubMed.
- S. Voigt, A. Tetzlaff and J. Zhang,
et al., Integrating satellite remote sensing techniques for detection and analysis of uncontrolled coal seam fires in North China, Int. J. Coal Geol., 2004, 59, 121–136 CrossRef CAS.
- D. Q. Huang, Y. T. Zhao and Z. Q. Xie, The remote sensing survey of coal fire in the Ruijigou coal mine, Ningxia, Geophys. Geochem. Explor., 2007, 31, 41–44 Search PubMed.
-
Y. Lin, Design and Implementation of Coal Fire Remote Sensing Information Monitor System in Rujigou Based on Avenue, Xi’an University and Technology, Xi’an, 2006 Search PubMed.
- W. H. Geng, T. Nakajima, H. Takanashi and A. Ohki, Determination of total fluorine in coal by use of oxygen flask combustion method with catalyst, Fuel, 2007, 86, 715–721 CrossRef CAS.
- D. M. Yu, X. H. Wu and X. H. Cui, Causes and distribution of endemic disease about arseniasis and fluorosis in Pingluo county from a view of geochemistry, J. Agric. Sci., 2011, 32, 45–47 Search PubMed.
- J. P. Yan, Study on Fluorine Content in Forage Grass, Sci. Technol. Baotou Steel, 1999, 2, 46–49 Search PubMed.
- D. Gao and H. W. Liu, Effects of atmospheric fluorine pollution on herbage, Gansu Environmental Study and Monitoring, 1985, 4, 5–9 Search PubMed.
- H. W. Liu and D. Gao, Discussion on chronic fluorosis of livestock and critical range in pasture fluorine, Chin. J. Environ. Sci., 1985, 6, 40–45 CAS.
- H. W. Liu and D. Gao, Discussion on fluorine pollution of pasture and chronic fluorosis of livestock, Environmen. Sci. Series, 1986, 11, 74–78 Search PubMed.
- X. P. Hong, H. D. Liang, S. LV, D. Y. Wang and Y. Zhang, Potential release of fluoride from clay for coal-combustion in Hehua village, Zhijin county, Guizhou province, Geol. Rev., 2015, 6, 852–860 Search PubMed.
- X. P. Hong, H. D. Liang, Y. F. Zhang and D. M. Xu, Acidity characteristic of efflorescent clay in late Permian coal-bearing strata in border areaof Yunnan, Guizhou and Sichuan, Environ. Chem., 2017, 36, 1831–1841 CAS.
- J. C. Huang and L. G. Gui, Effects on growth of chinese wolfberry and soil by Ningxia alkaline soil fertilization technology, Ningxia Journal of Agriculture and Forestry Science and Technology, 2015, 56, 29–31 Search PubMed.
- F. F. Zhang, J. Sun and Z. K. Xie, The influence of fertilization on photosynthesis and yield of oriental lily “Sorbonne” under the alkaline soil in northest China, Acta Prataculturae Sin., 2012, 21, 79–87 Search PubMed.
- W. Li, F. Li, D. Bi, H. Wang, H. Li, S. Zhou, Q. Zhao and X. Yin, Spatial distribution and speciation of selenium in alkaline soils and agricultural products of Lanzhou, Soils, 2012, 44, 632–638 CAS.
- Li Qingguang, Wu Pan and Li Xuexian,
et al., The effect of mining development in karst areas on water acidification and fluorine enrichment in surface watersheds, Ecotoxicol. Environ. Saf., 2022, 242, 113954 CrossRef.
- H. Wang, Y. Liu, X. F. Tang and W. Wang, Rapid treatment technology of small coal mine fire district in Rujigou open pit mine, Coal Technol., 2016, 35, 164–166 CAS.
- M. Y. Zhang, S. J. Wang, G. Q. Ma, H. Z. Zhou and J. Fu, Sulfur isotopic composition and identification of atmospheric environment in central Zhejiang, China, Sci. China: Earth Sci., 2010, 53, 1717–1725 CrossRef CAS.
- W. H. Li, F. Xiong and Y. Jiang, The occurrence of trace hazardous elements in high-sulfur coals, Coal Chem. Ind., 1994, 4, 20–23 Search PubMed.
- X. Hong, K. Yang and H. Liang,
et al., Weathering of Clay-Pyrite of Coal-Bearing Formation in the Endemic Fluorosis Area of Southwest China, Pol. J. Environ. Stud., 2023, 32, 1433–1445 CrossRef CAS.
|
This journal is © The Royal Society of Chemistry 2024 |
Click here to see how this site uses Cookies. View our privacy policy here.