DOI:
10.1039/D3SU00292F
(Paper)
RSC Sustain., 2024,
2, 646-654
An antibacterial hydrogel prepared from a licorice residue extract
Received
22nd August 2023
, Accepted 26th December 2023
First published on 19th January 2024
Abstract
A licorice residue extract was used as a precursor to prepare hydrogels in this study. The hydrogels were successfully prepared by dissolving the licorice residue extract in an NaOH/urea aqueous solution system and crosslinking it with epichlorohydrin (ECH). A scanning electron microscope (SEM) and Fourier transform infrared spectrometer (FTIR) were used to analyse the morphology and structure of the hydrogels. The water retention capacity of the hydrogels was also studied. After being characterized, the hydrogels were applied to fruit preservation. Blueberries were selected for the application, and sensory evaluation, weight loss ratio, decay ratio and vitamin C content were taken as indicators to evaluate the effect of hydrogels on fruit preservation. The hydrogels which were brown had good water retention and antibacterial effect on Escherichia coli and Staphylococcus aureus. They can retain the sensory properties of blueberries, significantly reduce the weight loss ratio and rotting ratio, delay the decrease of vitamin C content, and have a good fresh-keeping effect. In summary, this hydrogel could be used as a water-retaining, antibacterial and fresh-keeping material, and provides a more efficient and potential choice for substituting plastic in various branches.
Sustainability spotlight
In recent years, it has been increasingly concerned about environmental pollution caused by oil-based material. The sustainable development of cellulose as a renewable resource has also received widespread attention. Cellulose can play a huge role in food preservation and applications; its molecular chain has a large number of hydroxyl groups, which can react with many small molecule compounds to modify them to produce different cellulose products with antibacterial properties. However, most of the cellulose resources have not been effectively utilized up to now, so we used alkaline extraction to obtain extracts from licorice residue to prepare hydrogels as a new membrane. The comprehensive performance and application prospects in food preservation of hydrogels were studied. The prepared hydrogels had good water retention and antibacterial effect on Escherichia coli and Staphylococcus aureus, and had a good fresh-keeping effect. The licorice residue extract antibacterial hydrogel not only improves the comprehensive utilization rate of licorice, but also reduces environmental pollution because of its biodegradability, providing a more effective and potential option for the replacement of plastics in various fields.
|
1 Introduction
In recent years, with the rapid development of the economy and technology, the problems of environmental pollution and waste of resources are becoming increasingly serious. The utilization and research of renewable resources have gradually attracted extensive attention from relevant personnel.1 As a renewable resource, cellulose is widely distributed in nature and has abundant reserves. Because of its low raw material cost, strong processability, biodegradability and biocompatibility, it is also widely used in medicine, food, pesticides and other fields.2 However, most of the cellulose resources have not been effectively utilized up to now, so how to further effectively separate and extract cellulose to reduce resource waste has become an issue which is worth paying attention to. ref. 3–5.
Most of the food plastic wrap products currently used are PE materials, most of which only prevent contact between external bacteria and food, but cannot inhibit the growth of bacteria. Therefore, to a certain extent, the fresh-keeping effect is limited. This type of fresh-keeping film is difficult to decompose under natural conditions, which also causes pollution to the environment and is not environmentally friendly.6 Due to the excellent film-forming properties, hydrogels have been used as fresh-keeping packaging materials in recent years. A hydrogel is a kind of soft material with a three-dimensional network structure, which can absorb a large amount of water in a short time to reach a swollen state, and be insoluble in water.7,8 At present, the production of the vast majority of hydrogel requires a large amount of non-renewable resources, and it is not easily degraded, causing certain damage to the environment. The hydrogels prepared from natural hydrophilic polymers such as cellulose have excellent biocompatibility and degradability, and have broad application prospects because of their wide range of raw material sources and low cost.9 Although using biopolymers to produce hydrogels is more sustainable in certain aspects, it cannot be used in all applications. For instance, PEG-hydrogels are an excellent candidate for biomedical materials despite their petroleum-based origin, and it is hard to use other types of sources because of the high demand in this area.10 Cellulose has a large number of hydroxyl groups in its molecular chain, which can react with many small molecular compounds and modify them to make antibacterial films. For the preservation of food, it can not only inhibit the growth of bacteria and prolong the preservation time, but also degrade in the natural environment without causing pollution to the environment.11,12 In summary, cellulose or its derivatives can play a huge role in hydrogel and food preservation application.13
According to the source of cellulose, it can be divided into plant, animal and mineral cellulose. The main sources of plant cellulose are cotton, wood and grass (including crop waste). However, there are few reports on the isolation of cellulose from licorice residue.14 The licorice residue is the dregs left by hydrothermal treatment of licorice (similar to the traditional Chinese medicine decoction method). Licorice residue is rich in cellulose and hemicellulose, as well as a large amount of flavonoids (3.0–3.5%), polysaccharides, alkaloids, and trace elements.15,16 China produces about 6–7 million tons of licorice residue every year.17 However, only a small part was reused (just to feed pigs or cows) while most of it was incinerated or discarded as solid waste.18 Up to now, the potential application of licorice residue has not been further explored. The licorice residue is supposed to be a more useful resource rather than a waste. How to realize the efficient utilization of licorice residue has become an attractive direction.
Therefore, we used alkali extraction to obtain cellulose-like extracts from licorice residue, and used ECH as a cross-linking agent to prepare a hydrogel for upgrading it into a new antibacterial film-forming material. Then the hydrogels were characterized using a scanning electron microscope (SEM) and Fourier transform infrared spectrometer (FTIR). In order to further evaluate the bacteriostatic activity of the hydrogels, Escherichia coli (E. coli) and Staphylococcus aureus (S. aureus) were selected as targets. The blueberries that are not easy to preserve were used as the targets of food preservation, and the sensory evaluation, weight loss rate, decay rate, and the content of vitamin C were taken as indicators to study the application prospects of hydrogels in food preservation.
2 Experimental
2.1 Materials
Licorice was used as a raw material and was obtained from Gansu Province to extract cellulose. Epichlorohydrin (ECH), sodium hydroxide (NaOH), and urea (CH4N2O) were analytical grade and purchased in China (Damao Chemical Reagent Factory). Escherichia coli (E. coli) and Staphylococcus aureus (S. aureus) freeze-dried bacterial powders were also purchased in China (Huankai Microbial) to prepare the suspended bacterial cultures. After resuscitation, the suspended bacterial cultures were frozen in a refrigerator at −80 °C until use. Nutrient broth (HB0108), nutrient agar (HB0109), tryptone soy broth (HB4114), Baird-Parker agar base (HB4115) and tellurate yolk enhancer solution (HB4116-1) were also purchased in China (Hopebiol Biotechnology).
2.2 Extraction and pretreatment of licorice
The licorice residue was used as the source raw material, and the cellulose-like extract was obtained by the alkali extraction method, for which Ma Z. et al.19 was referred to to prepare a novel super-absorbent polymer with some modifications. Chang C. et al.20 and C. Jie et al.21 also successfully prepared novel hyperabsorbable hydrogels by heating and freezing in an NaOH/urea/water system using cellulose as a raw material and epichlorohydrin (ECH) as a cross-linking agent. Like the traditional processing for Chinese medicine, 50 g licorice was washed with distilled water and soaked in 500 mL distilled water for 30 min, then heated at 60 °C for 1 h, with heating repeated twice, dried, crushed and sieved through a 100-mesh sieve to obtain the licorice residue powder. Then 15 g of the licorice residue powder was added to 300 mL 4 wt% NaOH solution (the mass ratio of 1
:
20) and magnetically stirred for 3–6 h at 60–80 °C. The mixture was centrifuged at 4000 rpm for 15 min, filtered three times, washed multiple times with distilled water, then dried at 60 °C for 24 h, crushed and stored in sealed containers for storage.
2.3 Preparation of licorice residue extract hydrogels
1.3 g, 1.4 g, and 1.5 g of the licorice residue extract powder were added into 10 mL NaOH/urea/H2O (6
:
4
:
90 by weight ratio) aqueous solution with 13%, 14% and 15% mass ratio, respectively, and stirred with a mechanical agitator for 30 min at room temperature. After that, ECH (1 mL) was added to the mixture (100 g) as cross-linker and stirred mechanically at room temperature for 30 min. Then it was frozen at −20 °C for 12 h.20,21 After thawing, it was heated at 60–80 °C for 30 min, cooled down to room temperature and washed with distilled water to obtain licorice residue extract hydrogels. The hydrogels were named as GL (hydrogel low), GM (hydrogel middle), and GH (hydrogel high) in conformity with the mass ratio of the licorice residue extract powder.
2.4 Characterization
The microstructure of lyophilized hydrogels after water absorption was observed using a scanning electron microscope (SEM, JEOL, JSM-6701F) in order to investigate the stability of hydrogels after absorbing water for 7 days. The hydrogels that were swollen in distilled water at 37 °C for 7 d were frozen in liquid nitrogen for 24 h and sputtered with gold before the SEM observation. The hydrogel samples were crushed into powder and vacuum-dried at 50 °C for 24 h before the element analysis, FTIR and TGA. The content of nitrogen, carbon and hydrogen in the hydrogels was determined using an element analyser (VARIO EL CUBE, Elementar Co., Germany). FTIR was analysed using a Fourier transform infrared spectrometer (NEXUS 670, NICOLET Co., USA) in order to infer the types of functional groups contained in the hydrogels. Thermogravimetric analysis (TGA) was performed by using a thermogravimetric analyser (PT 1600, Linseis Co., Germany) at a heating rate of 15 °C min−1 from 30 °C to 800 °C under a nitrogen atmosphere. Rheological tests were carried out using a rotational rheometer (MCR302 P/N 92002, Austria). Frequency sweep tests for elastic modulus (G′) and viscous modulus (G′′) of hydrogels at normal state (NSH), that was washed with distilled water, and hydrated state (HSH), that was absorbing water for 3 days, were conducted in a range between 0.1 and 100 Hz at 20 °C and 40 °C at a 1% constant strain. Shear viscosity tests were performed at 20 °C and 40 °C, and the shear rate was set in a range between 0.1 and 100 s−1.
2.5 Water retention ratio measurement
The water retention ratio of the hydrogels was used to study the water retention properties of the equilibrium swollen hydrogels. The hydrogels were immersed in distilled water at 37 °C for 7 d until the weight of the hydrogels did not change any more. The hydrogels were weighed using an electronic balance after the water on the surface was lightly wiped off with filter paper.21 The swollen hydrogels were kept in a 40 °C oven and weighed every 12 h. The water retention ratio (WR) was calculated as
where Wt is the weight of the hydrogels at a specified time during the water loss process, and Ws and Wd are the weight of the hydrogel in the swollen and dried states, respectively.22
2.6 Antibacterial activity measurement
E. coli (CMCC(B) 44102) and S. aureus (CMCC(B) 26003) were used to test the antibacterial properties of the hydrogels. The frozen suspended bacterial cultures were taken out from the refrigerator (−80 °C), after which 10 μL of E. coli and S. aureus suspended bacterial culture were added into 5 mL of nutrient broth and tryptone soybean broth respectively, and taken out after being cultured in a constant temperature shaker (37 °C) for 10 h. The resuscitated suspended bacterial cultures were diluted to the concentration of 105 CFU mL−1 for later use. The temperature of the medium after autoclaving was too high, which will lead to changes in bacterial structure and function. Therefore, it is necessary to add bacterial suspension cultured at 37 °C when the medium is cooled to about 50 °C to ensure bacterial activity. 100 μL of E. coli suspension was added to 15 mL of nutrient agar medium, 100 μL S. aureus suspension was added to 15 mL of Baird-Parker agar base (5 mL thawed potassium tellurite booster was added to every 95 mL of medium and mixed evenly), the bacterial suspension and the culture medium were uniformly mixed and then cooled to solidification. The hydrogels were cut into pieces with the same thickness and diameter and the pieces were laid in the centre of the Petri dishes. The Petri dishes were placed in a 37 °C constant temperature incubator for 24 h, and after taking them out, the inhibition zone was measured and photographed and recorded. All the above operation steps were completed in the purification workbench. The hydrogels and the required experimental materials were UV disinfected for 30 min before the experiment starts.23 Each hydrogel was subjected to three sets of parallel experiments in order to ensure reproducibility.
2.7 Fruit preservation application
Blueberries were rinsed with deionized water, then dried completely at room temperature. Blueberries were randomly divided into four groups with an average of three portions per group. The first portion (control group) was stored directly into the fresh-keeping box for preservation; the second was placed in a polystyrene plastic bag and stored in the same fresh-keeping box. The licorice residue extract hydrogel was sliced and laid on the bottom of the same fresh-keeping box as a cushion, where the third group (fresh-keeping pad group) was stored. All containers were sealed to maintain the relative humidity. Finally, they were put into a constant temperature (20 °C) and humidity (75%) box for preservation, and were taken out at 4 consecutive days for physical and chemical analysis.
2.8 Apparent quality and sensory determination
After 0 and 4 days of blueberry storage under different conditions, the researchers (not participating in sensory evaluation) randomly selected 10 samples from each group of blueberries and placed them on filter paper of the same specification. At the same time, three groups of blueberries were placed on the same horizontal plane to obtain their appearance photos with a fixed light source. Under the specified evaluation conditions, the evaluators with normal colour vision did not know the storage conditions for each group of blueberries, comprehensively evaluated the appearance, texture and smell of the samples through observation, smell and taste. During this period, it was guaranteed that no one will interfere or induce each other. The scoring results were summarized and analysed by researchers (not involved in sensory evaluations). The scoring criteria was designed according to GBT 21172 and GHT 1408. Grading by colour, firmness, flavour and fruit appearance were shown in Table 1. The results were expressed as the average of the composite scores of the members of the evaluation team.
Table 1 Sensory scoring standard for the storage effect of blueberries
Score |
Appearance |
Hardness |
Smell |
4 |
Fresh |
Excellent elasticity |
Fruity |
3 |
Normal |
Good elasticity |
Normal |
2 |
Moderate browning |
Become soft |
Slight odor |
1 |
Severe browning, localized putrefaction |
Soft and water stained |
Strong odor of decay |
2.9 Weight loss ratio
The weight loss ratio of blueberries can be used to estimate the loss rate of moisture content in blueberries to a certain extent, and blueberries lost moisture and became less full, which in turn affected their apparent score. Therefore, the fresh-keeping effect can be evaluated by comparing the weight loss ratio of blueberries in each group. The blueberry samples were weighed and recorded with an electronic balance after drying, and the result was marked as W1. The weight of each group was weighed on 4 consecutive days; the result was recorded as W2. The weight loss ratio of all treatment groups was calculated using the following formula:24
2.10 Decay ratio
On the 4 consecutive days of storage, whether blueberries were rotten or not was considered according to the softening condition and smell of blueberries, and the percentage of rotten blueberries in the total number of blueberries is the decay ratio.25
2.11 Vitamin C content
The content of vitamin C in blueberries was determined by GB 2,6-dichlorophenol titration.26
2.12 Data processing and statistical analysis
The sensory assessment was the mean ± standard deviation of all sample scores. SPSS 20.0 statistical analysis software was used to analyse the results of the experimental data. A T-test was used to compare the mean between the two groups, ANOVA was used to compare the mean between multiple groups, and pairwise comparison was conducted between groups. P < 0.05 indicated that the difference was statistically significant.
3 Results and discussion
3.1 Appearance and morphology of the hydrogels
The photos of the GL (a), GM (b), GH (c) in the dehydrated state (a-1, b-1, c-1) and immersed in distilled water for 7 d (a-2, b-2, c-2) are shown in Fig. 1. It can be clearly seen that as the size of the hydrogels was increased, the colour became deeper. The diameter of the three hydrogels increased by 0.2 cm (GL), 0.4 cm (GM), and 0.2 cm (GH) after immersion in distilled water. The colour and size of the hydrogels did not change after 7 d due to the equilibrium of water absorption. Among these hydrogels, the smaller the content of licorice residue extract, the more fragile they became after being soaked in distilled water for 7 days. This demonstrated that the licorice residue extract played a role in supporting the structure of the hydrogels.
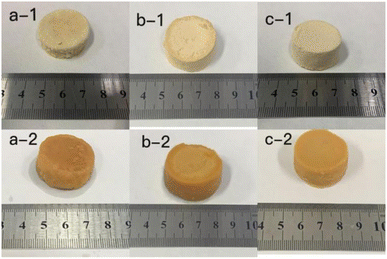 |
| Fig. 1 Photographs of (a) GL, (b) GM, (c) GH in the dry state (1) and hydrated state (2) for 7 d. | |
Fig. 2 shows the surface layer (a) and internal morphology (b) of the hydrogels after being immersed in distilled water for 7 d and then frozen in liquid nitrogen for 24 h, that were observed by scanning electron microscopy (SEM) at 500 (1) and 1000 (2) times magnification. Compared with GL and GH, the SEM observation of GM was the most reference. The SEM clearly exhibited that a large number of porous holes appeared on/in the hydrogel after adsorption. This indicated that plenty of water could be lightly absorbed and diffused into the hydrogel, with the licorice residue extract as a backbone to reinforce the structure of the hydrogel.22
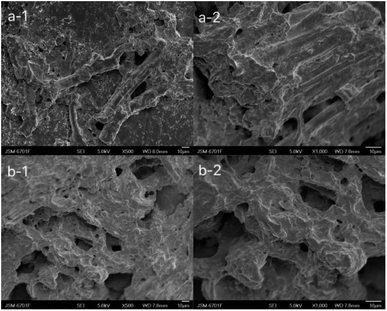 |
| Fig. 2 Scanning electron microscopy of the surface layer (a) and internal structure (b) of GM at 500 (1) and 1000 (2) times magnification. | |
Fig. 3 shows the FTIR spectra of the dehydrated hydrogels, and the three different proportions of hydrogels contained basically similar groups. The analysis of FTIR was performed to identify the main functional groups of the hydrogels. The main peak at 3350 cm−1 was due to the –OH stretching vibration on the backbone. The symmetric stretching vibration of CH2 was proved by the appearance of the absorption peak at 2918 cm−1. And the band observed at 1666 cm−1 to 1485 cm−1 in the hydrogels was attributed to symmetric vibration of the carboxyl group.21 The presence of C–O–C was proved by the appearance of the absorption peak at 1059 cm−1. The results showed that the prepared gels had a similar structure but also some differences because of the content, especially at 3350, 1666 and 1059 cm−1.
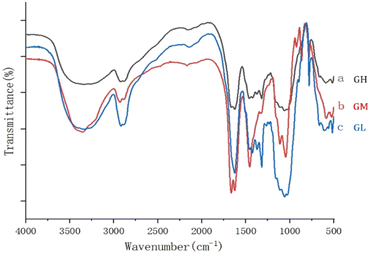 |
| Fig. 3 FTIR spectra of the dehydrated hydrogels: (a) GH, (b) GM, (c) GL. | |
The NSH and HSH were selected as the models for the prepared hydrogels to investigate their rheological behavior. The magnitude of G value in the linear viscoelastic region of hydrogels can be used for assessing the strength of gels, and a strong gel usually has a large G′ value and a high G′/G′′ ratio.27 It’s exhibited that the G′ value of these hydrogels had a distinct upward trend, as the frequency increased in Fig. 4(a and b). The G′ values of the hydrated state hydrogel (HSH) were much smaller than that of the normal state hydrogel (NSH), which indicated that the strength of the hydrogel decreased after water absorption. The curves for viscosity versus shear rate of NSH and HSH are presented in Fig. 4c. They were viscous in the low shear rate range and showed declining viscosity along with increasing shear rate.
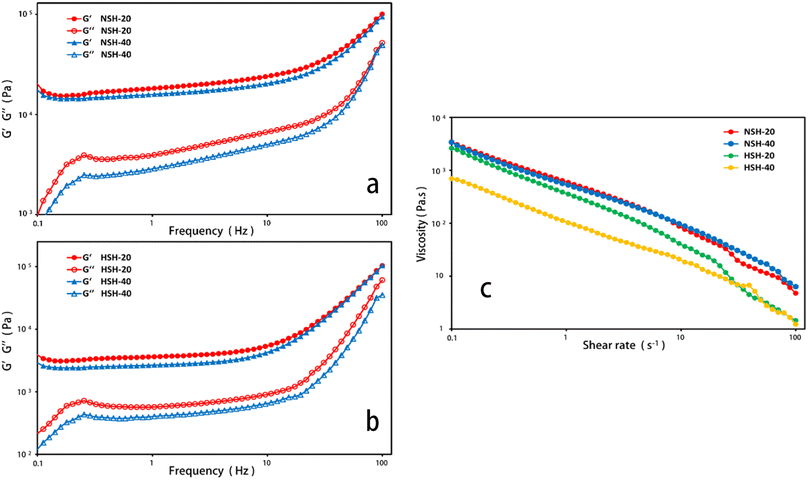 |
| Fig. 4 Frequency sweep spectra (a and b) of elastic modulus (G′) and viscous modulus (G′′), and viscosity versus shear rate (20 °C and 40 °C ) (c) for normal state hydrogel (NSH) and hydrated state hydrogel (HSH). | |
3.2 Water retention capacity of the hydrogels
The hydrogels were put into a 40 °C vacuum dryer for drying to study the water retention capacity, the water retention ratio was calculated and the results are shown in Fig. 5. The water retention ratio of the hydrogels was an important index to consider whether the hydrogels can be applied further. The results suggested that the hydrogels can keep moisture for up to 48 hours at 40 °C, and GH has the best water retention among three hydrogels. The water retention ratio was increased with increasing licorice residue extract content from which it was speculated that the hydrogels have better stability and stronger water retention capacity.
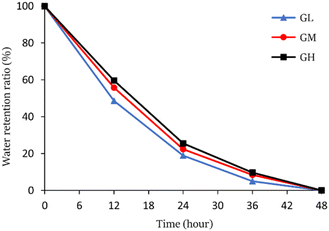 |
| Fig. 5 Water retention ratio of the hydrogels: GL, GM, GH. | |
3.3 Antibacterial activity measurement
The inhibition zone method was used to evaluate the antibacterial activity of the hydrogels against E. coli and S. aureus.28 It can be seen from Fig. 6 that all hydrogels have shown strong antibacterial properties against E. coli and S. aureus. The antibacterial effect of hydrogels on E. coli was better than on S. aureus. With the increase of licorice residue extract content, the radius of the inhibition zone of the hydrogels against E. coli increased from 0.20 cm to 0.57 cm. At the same time, the radius of the inhibition zone of the hydrogels against S. aureus was also increased. The radius of the inhibition zone of the hydrogels against S. aureus increased from 0.19 cm to 0.44 cm with the increase of licorice residue extract content. All of this suggested that the hydrogels had good antibacterial properties against both Gram-negative and Gram-positive bacteria. It can be observed that the three hydrogels have different antimicrobial properties when the diameter of the inhibition zone changed with the increase of the content of licorice extract. It's assumed that antibacterial performance should be provided by the licorice residue extract. The hydrogel can be widely used as a bacteriostatic material.
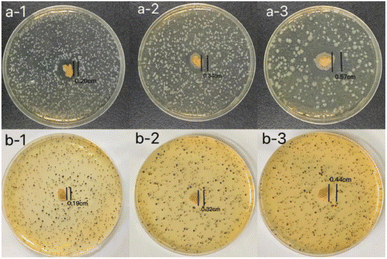 |
| Fig. 6 Inhibition zone of GL (1), GM (2), and GH (3) for E. coli (a) and S. aureus (b). | |
4 Fruit preservation application
The blueberries in the control group were stored directly into the fresh-keeping box for preservation. The blueberries in the plastic bag group were placed in a polystyrene plastic bag and stored in the same crisper box. The licorice residue extract hydrogel was sliced and laid on the bottom of the same fresh-keeping box as a cushion, where the blueberries in fresh-keeping pad group were stored in.
4.1 Sensory assessment results
Fig. 7 shows the appearance of blueberries stored at 0 and 4 days in different groups, sensory evaluation score was performed on this basis. From Table 2, the average score was 5.06 ± 0.31 in the control group, 6.60 ± 0.31 in the plastic bag group and 7.73 ± 0.14 in the fresh-keeping pad group. The score of blueberries in fresh-keeping pad groups was higher than that in plastic bag and control group, and the difference was statistically significant (P < 0.05).
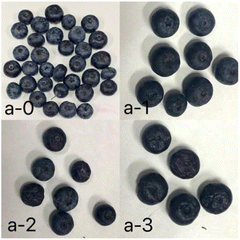 |
| Fig. 7 Appearance photos of blueberries stored at 0 (a-0) and 4 days in the fresh-keeping pad group (a-1), plastic bag group (a-2), and control group (a-3). | |
Table 2 Sensory evaluation score table of blueberry
Group |
Average score |
Indicates that the difference is statistically significant compared to the control group.
Indicates that the difference is statistically significant compared to the plastic group.
|
Control group |
5.06 ± 0.31 |
Plastic bag group |
6.60 ± 0.31a |
Fresh-keeping pad group |
7.73 ± 0.14a,b |
4.2 Weight loss ratio
Fig. 8 shows that the weight loss ratio of blueberries continued to increase with the extension of the storage time. The weight loss ratio of the control group was higher than others (P < 0.05). After 4 days of storage, the weight loss ratio of blueberries was (7.71 ± 0.29)%, (5.94 ± 0.27)%, (5.10 ± 0.14)% for the control group, the plastic bag group and the fresh-keeping pad group respectively. And the difference between the plastic bag group and the fresh-keeping pad group was statistically significant (P < 0.05).
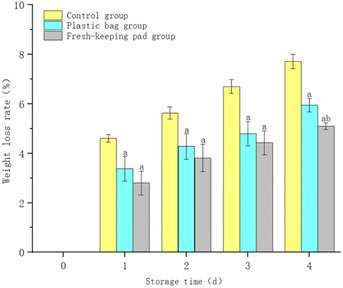 |
| Fig. 8 Effects of different storage methods on the blueberry weight loss ratio. aIndicates that the difference is statistically significant compared to the control group; bindicates that the difference is statistically significant compared to the plastic bag group. | |
4.3 Decay ratio
Fig. 9 shows that the decay ratio of blueberries continued to increase with the extension of the storage time. The decay ratio of the control group was significantly higher than that of the others (P < 0.05); the difference between the plastic bag group and the fresh-keeping pad group was not statistically significant (P > 0.05). After 4 days, the decay ratio of blueberries had reached (70.0 ± 10.00)% for the control group, and (53.33 ± 12.47)% and (36.67 ± 5.77)% for the plastic bag group and the fresh-keeping pad group respectively.
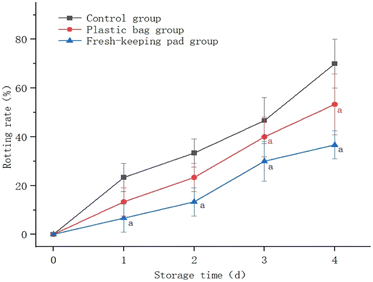 |
| Fig. 9 Effects of different storage methods on the blueberry decay ratio. aIndicates that the difference is statistically significant compared to the control group. | |
4.4 Vitamin C content
Table 3 shows that the vitamin C content of blueberries gradually declined in the storage process. The vitamin C content of the plastic bag group and fresh-keeping pad group reduced slowly, while the decrease in the control group was obvious. After storage for 4 days, the blueberries' residual vitamin C content was 3.38 mg/100 g, 5.66 mg/100 g, and 7.04 mg/100 g, respectively, and the difference between the three groups was statistically significant (P < 0.05).
Table 3 Effect of different storage methods on the vitamin C content of blueberries mg per 100 g
Storage time (d) |
Group |
Control group |
Plastic bag group |
Fresh-keeping pad group |
Indicates that the difference is statistically significant compared to the control group.
Indicates that the difference is statistically significant compared to the plastic bag group.
|
0 |
8.71 (8.4, 9.02) |
8.93 (8.71, 9.15) |
8.74 (8.63, 8.85) |
1 |
7.97 (7.57, 8.37) |
8.6 (8.48, 8.72) |
8.2 (8.19, 8.21) |
2 |
7.01 (6.4, 7.62) |
7.97 (7.43, 8.51) |
7.7 (7.47, 7.93) |
3 |
6.39 (6.01, 6.77) |
6.85 (6.27, 7.43) |
7.14 (6.95, 7.33)a |
4 |
3.38 (2.52, 4.24) |
5.66 (5.09, 6.23)a |
7.04 (6.85, 7.23)a,b |
We are located in one of the main areas of licorice production in China, when licorice is also the most used herb in traditional Chinese Medicine. China produces tens of thousands of tons of licorice residue every year. Only a small part was reused (just to feed pigs or cows) when most of them were regarded as solid waste, which is a burden for environment and also bad for sustainable development. Some other raw materials, such as corn straw and wheat straw, were also used as fuel or burned to feed back to the soil as the natural fertilizer in the vast rural areas. Therefore, considering with the other choices, we selected the licorice residue as a precursor to prepare hydrogels in this study. Compared with the others, this hydrogel has great water retention,29–31 which would bring more application potential, and better antibacterial activity.31 However, we chose ECH as crosslinking agents that it's toxic might not be ignored. Paulina Sapuła et al. (2023)32 suggested that one solution to this problem could be using bio-cross-linking agents that are obtained from natural resources, which are considered to be safer, free of toxic effects. So we still need to further explore whether toxicity can be tolerated especially when the hydrogels come in contact with food in the subsequent work.
5 Conclusion
Cellulose, as the most abundant natural polymer in nature, has many advantages such as good biodegradability and biocompatibility. In recent years, it has been proved to have important physiological functions. However, a considerable part of cellulose, such as licorice residue, has not been effectively used, but has been used as livestock feed or as waste to pollute the environment. In this paper licorice was used as a raw material for cellulose extraction to effectively improve the availability of licorice. Water-absorbing, fresh-keeping and antibacterial hydrogels were successfully prepared from the licorice residue extract. The extract from licorice residue was used as a backbone to support the structure of the hydrogels when the hydrogels could retain its appearance after absorbing water for at least 7 days. The retention time of water at 40 °C can reach 48 h. The hydrogels show good antibacterial activity against both E. coli and S. aureus. Therefore, blueberries were used to evaluated the preservation effect of the licorice residue extract hydrogels. Compared with the control group and the plastic bag group, the preservation effect of the licorice residue extract hydrogel is more obvious, which can reduce the weight loss rate and decay rate of blueberries and delay the decrease of vitamin C content. These results all indicated that this new hydrogel could be used as a water-retaining, antibacterial and fresh-keeping material. It provides a more efficient and potential choice for substituting plastic in various branches and will be studied further in our lab.
Conflicts of interest
There are no conflicts to declare.
Acknowledgements
This work was supported by grants from the Gansu Youth Science and Technology Fund (21JR7RA506).
References
- M. He, H. Chen and X. J. Zhang,
et al., Construction of novel cellulose/chitosan composite hydrogels and films and their applications, Cellulose, 2018, 25(3), 1987–1996 CrossRef CAS.
- H. Kargarzadeh, M. Mariano and D. Gopakumar,
et al., Advances in cellulose nanomaterials, Cellulose, 2018, 25(4), 2151–2189 CrossRef CAS.
- Q. Xu, C. Chen and K. Rosswurm,
et al., A facile route to prepare cellulose-based films, Carbohydr. Polym., 2016, 149, 274–281 CrossRef CAS PubMed.
- X. Sun, F. Xu and R. Sun,
et al., Characteristics of degraded cellulose obtained from steam-exploded wheat straw, Carbohydr. Res., 2005, 340(1), 97–106 CrossRef CAS PubMed.
- J. Chandra, C. S. Neena George and S. K. Narayanankutty, Isolation and characterization of cellulose nanofibrils from arecanut husk fibre, Carbohydr. Polym., 2016, 142, 158–166 CrossRef PubMed.
- C. Chang, L. Zhang and J. Zhou,
et al., Structure and properties of hydrogels prepared from cellulose in NaOH/urea aqueous solutions, Carbohydr. Polym., 2010, 82(1), 122–127 CrossRef CAS.
- L. Zhou, F. Xie and H. Li,
et al., Preparation and properties of regenerated cellulose hydrogels, IOP Conf. Ser.: Mater. Sci. Eng., 2017, 170(1), 12038–12043 Search PubMed.
- H. Geng, A one-step approach to make cellulose-based hydrogels of various transparency and swelling degrees, Carbohydr. Polym., 2018, 186, 208–216 CrossRef CAS PubMed.
-
H. Liu, W. Xu and D. Li, Development of New Plastic Packaging Film for Food Packaging, Springer, 2018, pp. 805–810 Search PubMed.
- K. Nishi, K. Fujii, Y. Katsumoto, T. Sakai and M. Shibayama, Kinetic Aspect on Gelation Mechanism of Tetra-PEG Hydrogel, Macromolecules, 2014, 47, 3274–3281 CrossRef CAS.
- M. Li, G. Chen, Z. Yu, R. Guo and X. Huang, Research Progress in Materials for Fruit and Vegetable Preservation, Packaging Journal, 2017, 9(5), 86–94 Search PubMed.
- L. Ye, Q. Fan, X. Tong and W. Jiang, Research Progress of Cellulose-based Antibacterial Composites, Chem. Ind. Times, 2019, 33(2), 36–40 Search PubMed.
- S. Bandyopadhyay and N. Saha, Bacterial cellulose and guar gum based modified PVP-CMC hydrogel films: characterized for packaging fresh berries, Food Packag. Shelf Life, 2019, 22, 2214–2894 Search PubMed.
- S. Wang, X. Wang and W. Liu,
et al., Fabricating cellulose nanofibril from licorice residues and its cellulose composite incorporated with natural nanoparticles, Carbohydr. Polym., 2019, 229, 115–464 Search PubMed.
- J. Zhang, F. Liu, L. Ning, D. Qing, Y. Sun, X. Jia and H. Ni, Identification of flavonoids gruffs of Glycyrrhiza inflate waste by UPLC-TOF-MS, Drugs and Clinic, 2012, 27(6), 558–561 CrossRef.
- Q. Zhang and M. Ye, Chemical analysis of the Chinese herbal medicine Gan-Cao (licorice), J. Chromatogr. A, 2009, 1216(11), 1954–1969 CrossRef CAS PubMed.
- X. Gui, G. Wang and X. Li,
et al., Fungus-assisted mild acid pretreatment of Glycyrrhiza uralensis residues to enhance enzymatic hydrolysis and oil production by green microalga Chlorella protothecoides, Ind. Crops Prod., 2014, 62, 466–473 CrossRef CAS.
- Y. Copur, A. Tozluoglu and A. Karademir, Pulping of licorice (Glycyrrhiza glabra): an alternative raw material to produce pulp, Cellul. Chem. Technol., 2007, 41(2–3), 155–159 CAS.
- Z. Ma, L. Qian and Q. Yue,
et al., Synthesis and characterization of a novel super-absorbent based on wheat straw, Bioresour. Technol., 2011, 102(3), 2853–2858 CrossRef CAS PubMed.
- C. Chang, B. Duan and J. Cai,
et al., Superabsorbent hydrogels based on cellulose for smart swelling and controllable delivery, Eur. Polym. J., 2010, 46(1), 92–100 CrossRef CAS.
- C. Jie and L. Zhang, Unique Gelation Behavior of Cellulose in NaOH/Urea Aqueous Solution, Biomacromolecules, 2006, 7(1), 183–189 CrossRef PubMed.
- T. Hu, C. Chang and L. Zhang, Efficient adsorption of Hg2+ ions on chitin/cellulose composite membranes prepared via environmentally friendly pathway, Chem. Eng. J., 2011, 173(3), 689–697 CrossRef.
- Y. Wang, Z. Wang and K. Wu,
et al., Synthesis of cellulose-based double-network hydrogels demonstrating high strength, self-healing, and antibacterial properties, Carbohydr. Polym., 2017, 168, 112–120 CrossRef CAS PubMed.
- Y. Deng, G. Huang and W. Zhao,
et al., A self-matching, ultra-fast film forming and washable removal bio-crosslinked hydrogel films for perishable fruits, Carbohydr. Polym., 2021, 267(10), 118–177 Search PubMed.
- E. Oregel-Zamudio, M. V. Angoa-Pérez and G. Oyoque-Salcedo,
et al., Effect of candelilla wax edible coatings combined with biocontrol bacteria on strawberry quality during the shelf-life, Sci. Hortic., 2017, 214, 273–279 CrossRef CAS.
- R. Li, X. Zhao and Y. Gao, Determination of Ascorbic Acid Content of Fruits and Vegetables with Red Color by 2,6-Dichlorophenolindophenol Converse Titration Method, Acta Nutr. Sin., 2012, 34(5), 3 Search PubMed.
- A. H. Clark and S. B. Ross-Murphy, Structural and mechanical properties of biopolymer gels, Adv. Polym. Sci, 1987, 57–192 CrossRef CAS.
- J. Yan, A. M. Abdelgawad and M. El-Naggar,
et al., Antibacterial activity of silver nanoparticles synthesized In situ by solution spraying onto cellulose, Carbohydr. Polym., 2016, 147, 500–508 CrossRef CAS PubMed.
- H. Ma, Y. Zhao and Z. Lu,
et al., Citral-loaded chitosan/carboxymethyl cellulose copolymer hydrogel microspheres with improved antimicrobial effects for plant protection, Int. J. Biol. Macromol., 2020, 164, 986–993 CrossRef CAS PubMed.
- Y. Deng, G. Huang and W. Zhao,
et al., A self-matching, ultra-fast film forming and washable removal bio-crosslinked hydrogel films for perishable fruits, Carbohydr. Polym., 2021, 267(10), 118–177 Search PubMed.
- C.-Q. Ruan, X. Kang and K. Zeng, Preparation of water-soluble dialdehyde cellulose enhanced chitosan coating and its application on the preservation of mandarin fruit, Int. J. Biol. Macromol., 2022, 203, 184–194 CrossRef CAS PubMed.
- P. Sapuła, K. Bialik-Wąs and K. Malarz, Are Natural Compounds a Promising Alternative to Synthetic Cross-Linking Agents in the Preparation of Hydrogels?, Pharmaceutics, 2023, 15, 253 CrossRef.
Footnote |
† These authors contributed equally to this work and should be considered as co-first authors. |
|
This journal is © The Royal Society of Chemistry 2024 |