How impurities responsible for recalls emerge in hand sanitizers†
Received
18th August 2023
, Accepted 11th January 2024
First published on 11th January 2024
Abstract
The recent global pandemic created an unprecedented demand for alcohol-based hand sanitizers. This stimulated the entry of many new producers into the market, both to provide an alternative income stream during the initial lockdowns, and to meet the needs of a society suddenly using far more sanitizer than was available due to production and transport interruptions. Similarly, this created a need for alcohol sources well beyond what had been produced for the consumer market. This has led to numerous recalls of formulations in both the United States and Canada for exceeding the limits of several key impurities, generally oxidized derivatives of ethanol, that could risk consumers' health. Some of these recalls likely arise from the use of substandard ingredients, but others are less easily explained. We hypothesized that the inclusion of hydrogen peroxide, and the possible introduction of metal salts from processing could explain the levels of oxidative impurities. This study investigates these questions experimentally using the standard WHO formula as the base formulation and finds that these proscribed impurities readily arise in the presence of metal salts and an oxidizing agent.
Sustainability spotlight
COVID-19 highlighted the fragility of the global healthcare supply chains. This induced the rapid preparation of local sanitizers; but their impurities could themselves prove a health threat. An understanding of how they can arise is essential to avoid this problem happening again. Safe locally-produced sanitizers are essential for enhancing sanitation (SDG6) and good health (SDG3); their deployment is essential for reducing waste and fostering responsible production (SDG12); and careful production is important in sustainable industrial design (SDG9), four of the UN Sustainable Development Goals. Properly constructed and maintained infrastructure, coupled with an understanding of the challenges in converting lines to the emergency production of sanitizer, will be essential for a rapid response to the next, inevitable, global pandemic.
|
1. Introduction
The sudden emergence of SARS-CoV-2,1 its quick spread, and the continual emergence, through at least January 2024, of novel variants of concern showing some avoidance of immunosurveillance, has put the global health, economy, and response to other existential challenges such as climate change, in danger.1–3 As of January 2024, COVID-19 has been implicated in at least 6.9 million deaths and 680 million confirmed positive cases worldwide,4 although excess mortality data suggests a far higher number.5 In the early days of the pandemic, before the airborne nature of the virus was confirmed, fomite transmission was a major concern and there was (and in some cases remains) a focus on sanitizing surfaces and hands.6 Regardless of its utility for SARS-CoV-2, sanitizers will likely continue to be more and more present in public spaces in society moving forward as alcohol-based hand sanitizers (ABHS) and handwashing effectively reduce the spread of pathogens.7 For healthcare settings, the CDC recommends ABHS with 60–95 percent alcohol (isopropanol or ethanol); too little alcohol and the sanitizer is not effective, too much and it also loses miscibility and effectiveness. In most clinical contexts, an ABHS is favoured over soap and water unless hands are visibly soiled (wherein alcohol-based sanitizers are not effective) due to evidence of higher user compliance for ABHS use than for soap and water.8 Many of the hand sanitizers on the market are derived from the WHO recommended liquid formulation. The WHO specifically recommends 80% ethanol or 75% isopropyl alcohol.9 These ABHS are effective against Gram-positive and Gram-negative bacteria, as well as enveloped and non-enveloped viruses, mycobacteria, and fungi; 85% ethanol eliminates 105 (99.999%) Gram negative or Gram positive bacteria in 15 seconds.10 Ethanol is highly efficient against viruses, while isopropanol is thought to be the better bactericidal agent, but both are more than suitable. Alcohols mixed together may have a synergistic impact.11
Along with the alcohol and water, ABHS typically contain a range of thickening agents, humectants, stabilizers, fragrances, emollients, moisturisers, emulsifiers, and plant-sourced essential oils.12 Humectants, such as Glycerol, are widely employed to prevent skin dehydration, extending the time it takes for alcohol to evaporate and boosts its biocidal activity.13 Emollients and moisturizers, like aloe vera, help to replenish part of the water lost from the skin. Likewise, hydrogen peroxide (H2O2) is recommended by the WHO as an antiseptic to eliminate bacterial spores that might be introduced during manufacture.9,14
Raw materials destined for the pharmaceutical and food industries must typically meet minimum quality criteria, such as those defined in monographs published by the Food Chemicals Codex (FCC)15 and the United States Pharmacopeia (USP).16 During the COVID-19 epidemic, there was an unprecedented increase in demand for hand sanitizer products, resulting in global shortages of USP grade, pharmaceutical, and food-grade ethanol, and isopropyl alcohol (IPA).17 The FDA and Health Canada both issued a notice to industry on April 15, 2020 on the time-limited approval of particular sources of fuel or technical-grade ethanol for use in the production of hand sanitizers.18,19 However, the change in production did lead to the introduction of impurities.20
Specific impurities are regulated in North America under these temporary regulations (Table 1). In addition, if the total amount of all other impurities exceeds 300 ppm, all individual impurities must be determined and pass the interim limits listed in Table 2. Despite this relaxation in standards, some new ABHS have been recalled by both the American FDA and Health Canada as they have been determined to contain unacceptable grades of ethanol or excessive amounts of cancerous chemicals such as ethanal (acetaldehyde), ethyl acetate, methanol or the unauthorized medicinal ingredient n-propanol.21,22 In many cases, this likely arose from poor quality feedstocks as methanol and propanol impurities are commonly found in lower grades of ethanol; however, this was not always the cause as many producers obtain their ethanol from certified sources with excellent quality control. Consequently, ethanal and ethyl acetate appear to be arising spontaneously in the product. How is this happening, and how can it be suppressed?
Table 1 FDA and Health Canada limits on class 1 impurities in ABHS
Impurity |
Interim limit under FDA policy19 |
Interim limit under Health Canada policy18 |
Methanol |
NMT 630 ppm |
NMT 200 ppm |
Benzene |
NMT 2 ppm |
NMT 2 ppm |
Ethanal (acetaldehyde) |
NMT 50 ppm |
NMT 75 ppm |
Acetal (1,1-diethoxyethane) |
NMT 50 ppm |
Not reported |
Sum of all other impurities |
NMT 300 ppm |
NMT 300 ppm |
Table 2 FDA limits on class 2 impurities in ABHS should all non-class 1 impurities combined exceed 300 ppm
Impurity |
Interim limit under FDA and Health Canada policy18,19 |
Acetone |
NMT 4400 ppm |
n-propanol (1-propanol) |
NMT 1000 ppm |
Ethyl acetate |
NMT 2200 ppm |
Sec-butanol (2-butanol) |
NMT 6200 ppm |
Iso-butanol (2-methyl-1-propanol) |
NMT 21700 ppm |
n-butanol (1-butanol) |
NMT 1000 ppm |
Iso-amyl alcohol (3-methyl-1-butanol) |
NMT 4100 ppm |
Amyl alcohol |
NMT 4100 ppm |
Of all the potential impurities, ethanal is of specific concern. It may cause cancer in consumers and could lead to serious illness or death.23 Ethanal is suspected to be genotoxic, and potentially carcinogenic, when in direct contact with tissue.18,19 Ethyl acetate, acetal, and acetic acid, the other three impurities of interest are important as they would be downstream byproducts arising from ethanal formation. In this study, we have investigated possible pathways which may lead to the formation of these impurities in ABHS by studying synthetic formulations generated with common additives, and quantifying the content of the impurities of interest as a function of time.
2. Materials and methods
2.1. Materials
USP Ethanol, 200 proof, was purchased from IGPC Ethanol Inc. (Leamington, Canada). Glycerol was purchased from Windy Point Soap Making Supplies Inc. (Calgary, Canada), carbomer (Carbopol 945) was obtained from the Lubrizol Corporation (Wickliffe, OH, USA). Hydrogen Peroxide 35%, ferrous sulphate, triethanolamine 98%, and EDTA were purchased from Sigma-Aldrich (St. Louis, USA). Ethanal and ethyl acetate (HPLC grade) and an ethanal diethyl acetal standard were purchased from Sigma Aldrich, Canada.
2.2. Methods
2.2.1. Preparation of the hand sanitizer.
The ABHS (Table 3) were prepared by adding each ingredient in the order listed at ambient temperature (23.5 °C), one by one, with thorough mixing to homogeneity after each addition. The only exception to this process is Formula F-6, in which the carbomer was added to the water, pH neutralized with triethanolamine (to pH 7.0), before the other ingredients were added. Once all ingredients were added, the solutions were mixed for an additional 30 minutes at 300 rpm. Each formulation was divided and stored in two separate sealed vessels (limited headspace above the sample, but sealed under atmospheric conditions), one at room temperature (RT), and the second at 45 °C for up to 60 days. Over this time period, 1 mL aliquots were withdrawn for analysis by GC-MS at days 0, 1, 2, 7, 14, 21, 28, 42, and 56.
Table 3 Composition of the evaluated ABHS
Ingredients |
F-0 |
F-1 |
F-2 |
F-3 |
F-4 |
F-5(1) |
F-5(2) |
F-6 |
F-7 |
Distilled water |
— |
To 100% |
To 100% |
— |
— |
To 100% |
To 100% |
To 100% |
To 100% |
HPLC water |
— |
— |
— |
To 100% |
To 100% |
— |
— |
— |
— |
Hydrogen peroxide (35%) |
— |
— |
0.36% |
— |
0.36% |
0.36% |
0.36% |
0.36% |
0.36% |
Glycerol |
— |
1.45% |
1.45% |
1.45% |
1.45% |
1.45% |
1.45% |
1.45% |
1.45% |
Ferrous sulfate |
— |
— |
— |
— |
— |
0.10% |
0.02% |
— |
— |
Carbopol 945 |
— |
— |
— |
— |
— |
— |
— |
— |
0.5% |
EDTA |
— |
— |
— |
— |
— |
— |
— |
0.10% |
— |
Ethanol |
100% |
80% |
80% |
80% |
80% |
80% |
80% |
80% |
80% |
2.2.2. GC-MS analysis method.
ABHS analysis was carried out using a PerkinElmer Clarus 590 GC with an SQ 8 MS (EI) detector. The GCMS was equipped with an automatic liquid sampler with a 5 μL volume capacity syringe. An Elite-624sil MS L 30m (ID 0.25 mm, 1.4 μm film) column with flow rates of 1.0 mL min−1 of Helium as a carrier gas was used for separation of the contaminants. Mass spectrometer (MS) scan mode in range of 50–300 amu was used for analysis while the transfer line and source were kept at 150 and 250 °C, respectively. The initial oven temperature was set to 35 °C and held for 2 min. The temperature was ramped first to 75 °C at 10 °C min−1 and held for 1 min; then to 180 °C at 20 °C min−1 and held for 1 min and finally, ramped to 260 °C at 20 °C min−1 and held for 2 min. Carrier gas split ratio was set to 120
:
1 and 1 μL of sample was injected per run.
2.2.3. GC-FID analysis method.
Quantification of ethanal, ethyl acetate, and acetal was performed using a PerkinElmer GC Clarus 590 instrument fitted with a Flame Ionization Detector and equipped with a DB-wax capillary column (Agilent) of dimensions 30 m by 0.25 ID and 0.25 μm film thickness. Injection of 0.5 μL samples was conducted in split mode (1
:
60) under the following conditions: injection port set at 220 °C, oven temperature set at 35 °C for 3 min, then programmed at a rate of 10 °C min−1 to 70 °C and held for 2 min, a further increase in temperature at 20 °C min−1 to 180 °C held for 1 min and a final increase at 20 °C min−1 to 230 °C held for 2 min. Flow rate of the carrier gas nitrogen (5.0 grade) was 1 mL min−1 and FID detector set to 250 °C. Standard dilutions of known concentration were analysed, and calibration curves generated for quantification of target analytes.
2.2.4 Validation of GC methods.
Validation of the GC acquisition method was performed by determining linearity, precision, selectivity, specificity, LOD and LOQ of the method for proper identification and quantification of the target analytes in the samples. Multiple injections per standard dilutions were performed, and their retention times and peak areas were recorded. A range of 0.05 to 99.40 mg mL−1 for ethyl acetate, 0.04 to 81.11 mg mL−1 for ethanal and 0.01 to 41.24 mg mL−1 for acetal were used to determine linearity and precision, as well LOD and LOQ for the instrument used for analysis. For selectivity and specificity, blank injections devoid of the target analytes were acquired and the chromatograms observed within the time window for elution of target analytes. The results are provided as Table S1.†
3. Results and discussion
We considered that ABHS impurities can arise from three sources: (1) impure raw materials; (2) process-related impurities where potential contaminants can be introduced during manufacture, such as gasoline and benzene, where transfer occurs from machinery or containers;19 or emergent formulation-based impurities that arise from chemical reactions of pure ingredients that can be affected by the environment or the presence of unexpected catalysts. The chemical community is aware of how easy it is for even very low and undesirable catalyst loading to affect chemistry.24
3.1 Generation and evaluation of formulations
Nine different formulations were produced to investigate the emergence of the impurities from highly pure feedstocks (Table 3). F-0 was created with 100% ethanol as a control and to determine if measurable impurities arise due to simple storage. F-1 contains 80% ethanol, and the WHO-recommended dose of glycerol – it was completed with deionized water. F-2 is identical to F-1 with the addition of the H2O2. The F-3 and F-4 formulations are identical to F-1 and F-2, respectively, with the distilled water replaced by HPLC-grade water to evaluate the effect of water sources. The difference is expected to be in the processing: deionized water, although highly pure, is prepared in a central facility at the University of Windsor, is transported to the taps through standard copper and PVC tubing: there is the potential for the introduction of impurities. HPLC-grade water on the other hand is rated to contain nearly undetectable levels of impurity. F-5(1) and F-5(2) are prepared by adding 0.1% and 0.02% Fe2SO4 to F-2 respectively. Metal impurities could catalyze the oxidation of ethanol by H2O2 and/or oxygen.25,26 Iron salts would not be expected to be native in ABHS but may be introduced due to the manufacturing environment (e.g., equipment, containers or even ingredients). Formulation F-6 was obtained by adding 0.1% EDTA as a chelating agent to F-2; EDTA would sequester any metals present in the solution and acts as a control on the rest of the formulation. The final formulation, a gel rather than a liquid, F-7, was obtained by adding 0.5% of carbomer to F-2. The physical and chemical properties of all liquid formulae are similar: all are clear colourless liquids, except F-7 which is a gel-liquid formulation with a viscosity of 12
400 cps at 25 °C, imitating leading commercially available gel-like formulations. For the impurity analysis we stored one set of samples in an oven set at 45 °C, and the second set at ambient temperature, ranging between 21 and 23 °C over the study period.
3.2 Formulation stability tests
To determine that all formulations were valid and stable, a centrifugation assay was carried out. The samples were centrifuged at 3000 rpm for 30 minutes at RT, no pellet or separation was observed. The temperature stability of each sample was evaluated by storing aliquots of the materials in duplicate under four different conditions: 5 °C, 23.5 °C, 45 °C and 55 °C for three months; in all cases the materials retained their consistency. On another set of aliquots of the above samples a freeze–thaw cycle was performed, heating and cooling the samples between −10 and +42 °C, with two cycles every 24 h, for 2 weeks (28 cycles total). Daily visual checks were performed.27 All formulations were stable under all these tests.
3.3 Quantitative analysis of impurities
Samples were injected neat into the GC instrument. Ethanol (80%) was a common additive to all samples during the formulation process and its concentration measured to ensure validation of formulation method and stability during storage of samples in −80 °C before GC analysis. The chromatogram (Fig. S1†) shows the proportion of ethanol in two different samples analyzed at different times in the study. There was no relative difference observed in ethanol concentration between samples and taking into consideration that both samples were prepared and analyzed at different times during the study, this observation indicated that there was no meaningful loss of solvent during storage. In addition, samples, once aliquoted from the test samples, were stored in sealed vials with unpunctured septa lined caps at −20 °C to ensure no loss of solvent during storage or analysis.
Validation of GCFID and GCMS acquisition methods for determining specificity showed no co-elution of other analytes with target analytes in this study. The limits of detection and quantification determined for the respective analytes, as well as their retention times are reported in Table S1.† Chromatograms of blanks and samples acquired to determine specificity of the method for detecting target analytes showed no co-elution of other compounds with the target compounds. Furthermore, chromatograms (Fig. S7†) of (a) blank, (b) sample and (c) standards show no co-elution of other compounds with target compounds. For precision of method, repeat injections of the same concentration of standards showed no relative difference in analyte concentration between injections. An RSD value of less than 2% was measured across multiple repeat injections of the three target analytes at the various standard dilutions; the RSD was as low as 0.6% for ethyl acetate. The methods used are appropriate for measuring the content of the hand sanitizers.
We tracked the emergence of ethanal in our formulations and hypothesized it would be time dependent. This was only partially the case. In our pure ethanol, F-0, the concentration began at, and remained below, 54 ppm at maximum, even at 45 °C, with the average remaining well under 50 ppm (Fig. 1). Upon the addition of glycerol and distilled water (F-1), there was no meaningful change in the initial concentration of ethanal; however, at 45 °C, ethanal steadily rose to 298 ppm after 45 days. In F-2, wherein we added the H2O2, even at ambient temperature, the ethanal concentration spiked immediately before falling off (there was no ethanal detected in the source H2O2); in samples stored at 45 °C, this increase is far more significant rising to 300 ppm. In F-3 and F-4 wherein we replaced the distilled water in F-1 and F-2 with HPLC water respectively (Fig. 1b), the pattern resembles F-1 and F-2. Glycerol alone induces a rise in ethanal, and H2O2 a larger rise, and this is accentuated at higher temperatures. The level of purity of the water, within reason, does not seem to be an issue (Fig. 1).
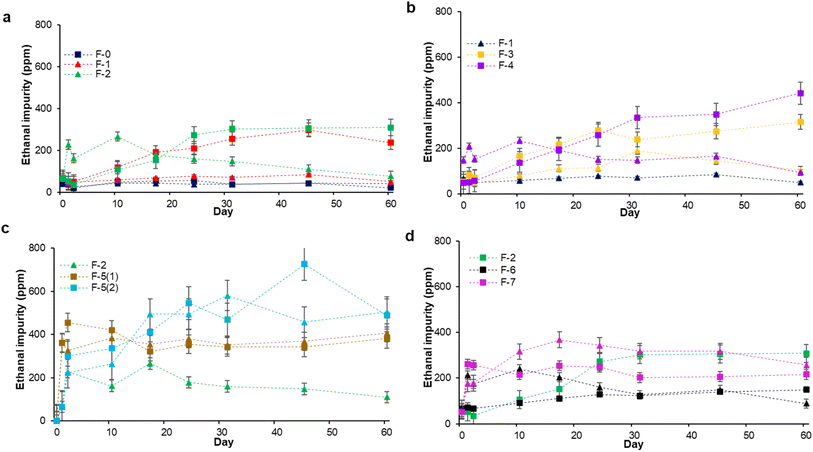 |
| Fig. 1 Analysis of ethanal concentration in ABHS. Analysis at ambient temperature is represented as (▲); that at 45 °C by (■). (a) Comparison of the ethanol blank, and the samples with glycerol, and glycerol and hydrogen peroxide added; (b) comparison with samples using HPLC-grade instead of distilled water; (c) comparison between samples spiked with ferrous sulfate and the metal free formula; (d) comparison between EDTA spiked and distilled water prepared standard formulations, and between standard formulations and the carbomer gel formulation. | |
We were surprised that simply including WHO-recommended levels of highly purified ingredients (not necessarily the feedstock in commercial sanitizers which might use lower grade materials) was sufficient to raise ethanal levels above FDA and Health Canada levels. But we believed that this was not the full story for the recalls. We worried that even small amounts of metal salts could potentially catalyze ethanol decomposition, the generation of reactive oxygen species, and ethanal formation.28 These salts could easily be introduced from any rust in water lines or processing machinery. This would be unthinkable in a well regulated facility, but during the pandemic many new processing lines were retooled or brought on-line that might not have been as well cared for. Consequently, to simulate this situation, we added both 1000 and 200 mg L−1 FeSO4 to F-2 to generate F-5(1) and F-5(2) (Fig. 1c). The ethanal concentration in the presence of 1000 mg L−1 of ferrous sulfate rose right away in the first few days, and this happens at both test temperatures. In F-5(2), with the lower concentration of metal salt, the initial rise is lower, but the maximum ethanal concentration reached is higher (726 ppm, 15-fold the FDA limit); curiously we do not see a significant effect of temperature on either reaction. Ethanal levels are higher than that observed in any other formulation suggesting that catalysis is occurring. For F-6 we added the metal chelator EDTA to F-2 to sequester any metal impurities (Fig. 1d). EDTA does appear to stabilize the solution, especially at 45 °C. Finally, we investigated the effect of adding an “inert” gelling agent (carbomer) on F-7 (Fig. 1d). Carbomer is an acrylic acid polymer, and we neutralized it with ethanolamine to generate the mixed salt in solution as is generally done in the cosmetic industry.29 This also increased the concentration of ethanal compared to the native F-2. Using a GC-FID technique to determine the ethanal content in the formulated ABHSs, gave a similar trend to the values obtained by GC-MS (Fig. S2†). This cross-validation was done to demonstrate that the results were not a figment of the detector choice.
Introducing additives initiates ethanol oxidation to ethanal. Although it is well established that this can arise from yeast or bacterial metabolism,30 this is unlikely in a formally antiseptic formulation. Other data from our lab demonstrates that these formulations are highly effective for eliminating bacterial and yeast cultures (see ESI Fig. S3†). Instead, the data is consistent with the mechanism being partially due to the presence of metal salts that can generate ROS to oxidize ethanol. This is not unprecedented.
Ethanal is generated in the gas phase reaction of ethanol vapour over copper, vanadium, or molybdenum metal oxide catalysts via oxidative dehydrogenation.31 Similarly, in solution phase, adding ethanol to a mixture of vanadyl ions and H2O2 results in a minor yield of ethanal which increases as a function of ethanol concentration.32 Ethanol oxidation by aqueous H2O2 and ferric salts has also been reported.25,26 Merz and Waters' proposed a reasonable free radical chain reaction mechanism to explain the oxidation, with hydroxyl radicals produced by the interaction between the iron centre and hydrogen peroxide initiating the oxidation process.26 The primary products of such a ferric ion-catalyzed oxidation, according to Heitler and collaborators, were ethanal and acetal.25 It seems feasible that a small concentration of H2O2 in the presence of metal impurities such as Na, Mg, Ca, or Fe could be the reason for the observed generation of ethanal in ABHS.
However, it is curious that ethanal concentrations do not always rise steadily in our ABHS formulations: initial high levels can fall off, and the rise also seems to slow at various threshold values. There also appears to be an induction period for some of the examples. We considered whether this was because ethanal was not the final product of the process, but simply an intermediate. In the presence of ethanol and acidic catalysts, ethanal can be readily converted to 1,1-diethoxyethane, confusingly commonly referred to as “acetal” in the regulatory industry (Scheme 1).33–35 Health Canada requests acetal content be provided in the USP alcohol monograph but has no explicit limit, while the FDA mandates less than 50 ppm in ABHS; under normal circumstances this is well above the extant amount present. The acetal is of course in equilibrium with ethanal in the presence of water, favouring ethanal;18 the acetal formation is more favoured in anhydrous conditions which are rarely found in ABHS.33,36 This equilibrium is consequently likely not the reason that ethanal levels are stabilizing. Further oxidation to acetic acid and esterification to ethyl acetate may be one potential end point for this process. To evaluate this possibility, we quantified acetal levels as a function of time (Fig. 2) by using GC-FID.
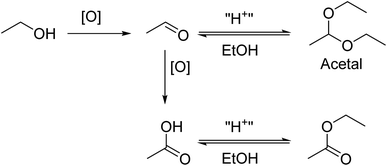 |
| Scheme 1 Proposed oxidation and sequestration pathways. Acetal is contraindicated in ABHS due to the relatively high concentration of water and lack of strong acid catalyst. | |
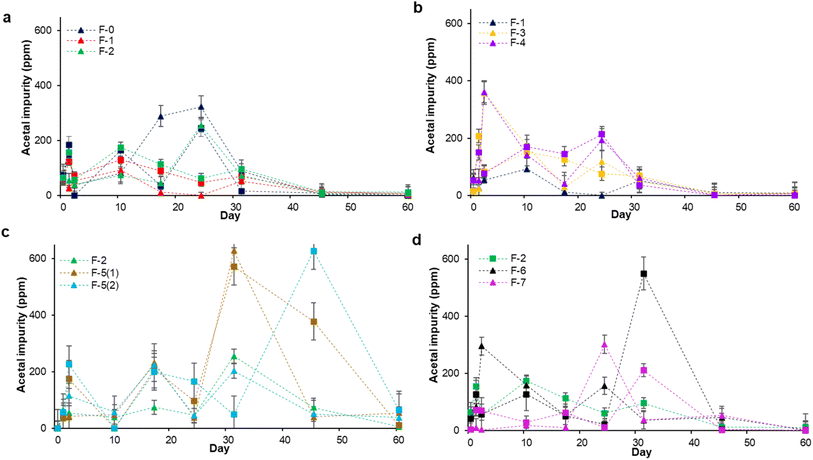 |
| Fig. 2 Analysis of acetal concentration in ABHS. Comparison between (a) F-0, F-1 and F-2; (b) F-1, F-3 and F-4; (c) F-2, F-5(1) and F-5(2) and (d) comparison of F-2, F-6 and F-7 formulations. Analysis in RT was shown by (▲) and in 45 °C was shown by (■). | |
The initial concentration of acetal in all formulations is less than 75 ppm, and it appears that the variation in acetal concentration is temperature independent. Furthermore, the change in acetal in all formulations follows a consistent pattern: the concentration of acetal in the formulations increases and falls multiple times. It may be established that fluctuations in acetal concentration are not caused by formulation or additions. Acetal levels in the presence of a metal salt, F-5, reach a maximum of 628 ppm, higher than that observed in any other formulation. However, even in this case, only a small amount of the acetal remained after two months. Also, the amounts of acetal and ethanal were added together and reported as one figure since it seemed likely that acetal would undergo hydrolysis (Fig. S4†). These total levels remain largely constant over the study for any given formulation, suggesting a steady state is attained.
Ethanal is an intermediate in the production of acetic acid.37 Ethanal (acetaldehyde) derivatives (ACTD) can also be present as impurities. The peak related to this impurity was identified by GC-FID(DB-Wax) (Fig. S5†). In all the formulations the concentration of the ACTD impurity is around 250 ppm at the beginning of the study and after two months it decreases slightly. However, the type of formulation has no noticeable effect on this impurity. It is likely present in the ethanol starting material, even in high purity ethanol.
We then quantified the ethyl acetate levels as a function of time (Fig. 3), especially as it is another regulated impurity (note, the acetic acid intermediate is not regulated, as at low concentrations vinegar is generally regarded as safe and is often used as a food additive).25,26,31,32,38 The concentration of ethyl acetate is lower than 100 ppm in the ethanol feedstock over the entire study regardless of temperature (F-0, Fig. 3a). Even by preparing glycerol and H2O2 containing F-1 and F-2 (Fig. 3a), and F-3 and F-4 (Fig. 3b) the maximum concentration ethyl acetate remains below 100 ppm. Adding the iron salts, F-5(1) and F-5(2), and letting stand at ambient temperature, does slightly increase the concentration of ethyl acetate reaching a maximum of 149 ppm after 45 days (Fig. 3c). However, by increasing temperature to 45 °C the rate of oxidation increases and the and the concentration of ethyl acetate increases daily, reaching 632 ppm, approximately 9 times higher than the formula without heavy metal impurities and around 4 times higher than the ambient temperature. Adding EDTA (F-6) keeps the ethyl acetate levels low, and although the carbomer gelling agent raises the initial levels, this might be due to the presence of residual acetic acid in the polymer being consumed rather than any independent catalytic activity (Fig. 3d). Using the GC-FID technique to determine the ethyl acetate content in the formulated ABHSs, gives a similar pattern to GC-MS (Fig. S6†). In formulae F-5(1) and F-5(2) alone, the GC-FID method does suggest higher concentrations for ethyl acetate than the GC-MS method. The samples are the same and were analyzed at the same time.
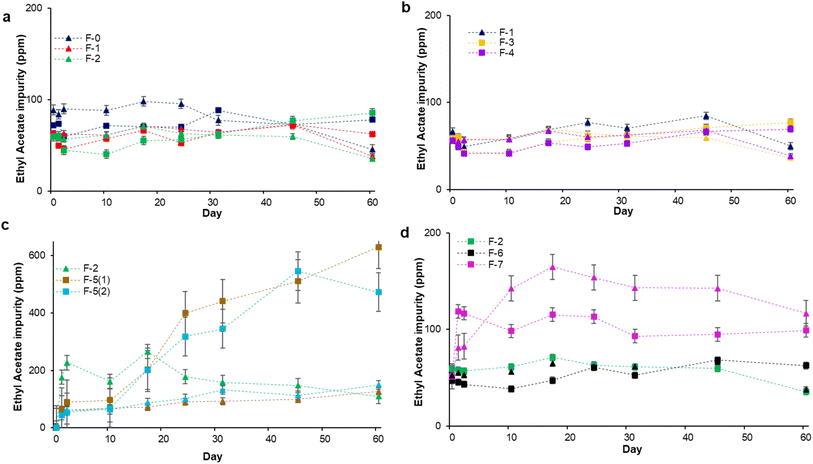 |
| Fig. 3 Analysis of ethyl acetate concentration in ABHS. (a) F-0, F-1 and F-2; (b) F-1, F-3 and F-4; (c) F-2, F-5(1) and F-5(2) and (d) comparison of F-2, F-6 and F-7 formulations. Ambient ageing is represented by (▲) while the samples incubated at 45 °C are shown by (■). | |
Consequently, the only factor that appears to meaningfully increase ethyl acetate content is the presence of metal salts. This is potentially partially due to the increased ethanal content driving oxidation forward, but they might also be acting as Lewis Acids to accelerate the Fischer esterification of acetic acid with ethanol. This is, after all, one of the industrial syntheses of ethyl acetate.39 It is also potentially possible that ethyl acetate is arising directly from the disproportionation and dehydrogenation of ethanol; a process used to make ethyl acetate directly with copper catalysts (eqn (1)).40
| 2C2H5OH → CH3COOC2H5 + 2H2 | (1) |
We consider this latter process extremely unlikely as it does generally require a steel autoclave under high pressure temperature.41 Ethanol can be selectively oxidized to ethyl acetate in the presence of ethanal and phosphoric acid,42 but the strong acid is lacking in ABHS. It is far likely that we are seeing a simple acceleration of the background Fischer process.
Conclusion
Impurities in ABHS have been responsible for multiple recalls of commercial sanitizers as the levels of the impurities present have been deemed to put citizens' health at risk. Technical grade, i.e. insufficiently pure, ethanol was certainly responsible for most of the impurities in hand sanitizer according to the FDA's reports for the recall of hand sanitizers. However, it is not the only problem. In order to inactivate contaminating bacterial spores in the solution, the WHO suggests adding 0.125% of hydrogen peroxide for local production of sanitizers.7 This risks oxidation chemistry leading to the formation of ethanal, acetal, or ethyl acetate contaminants, especially if the materials come in contact with metal salts. As a result, the FDA should investigate the possibility that in some of the recalled hand sanitizers, the main alcohol used in the production stage had an acceptable amount of impurity and that the level of impurity rose owing to the WHO guideline. These results should help producers consider their manufacturing lines and may help identify and prevent such issues in the future. We recommend that if impurities may be present, a small loading of EDTA, a food-safe additive, can compensate. We recognize that the COVID-19 ABHS emergency is long over, as the combination of traditional suppliers ramping up production, and decreased consumer use have reduced stresses on the system. However, experts consider it likely that another pandemic is likely to emerge as global integration, climate change, and human–animal interaction continues.43,44 We hope this information will not be needed. But we unfortunately expect it will be, and will help avoid the mistakes learnt in 2020.
Authors contributions
Conceptualization, JB, and JFT; funding acquisition, JFT and JB; investigation, FS-R, MAE, NM, BRA, PT; methodology, FS-R, MAE, NM, BRA; project administration, JFT; supervision, JFT; visualization, FS-R; writing original draft, FS-R, NM, MAE; writing – review and editing, all authors.
Conflicts of interest
The authors declare no competing financial interest, although Dr Binder is employed by Peak Processing, this work does not have any association with Peak Processing's business. Similarly, FS-R and JFT are associated with Binary Star Research Services. BSRS does not have any business interests in sanitizers. Neither Peak Processing, nor BSRS had any input into the experimental design or the conclusions of the article. JFT, FS-R and BRA declare that they have developed a non-alcohol based sanitizer that may be commercialized in the future with Prodigie Innovation Evolved LLC. The other authors have no conflicts to declare.
Acknowledgements
This study was supported by the Natural Sciences and Engineering Research Council of Canada 2018-06338, ALLRP 550385-20, ALLRP 558269-20, ALLRP 555689-20 (JFT), and MITACS Canada held with Peak Processing IT16323 (JFT, salary for NM and stipend for PT).
References
- Q. Li, X. Guan, P. Wu, X. Wang, L. Zhou, Y. Tong, R. Ren, K. S. M. Leung, E. H. Y. Lau, J. Y. Wong, X. Xing, N. Xiang, Y. Wu, C. Li, Q. Chen, D. Li, T. Liu, J. Zhao, M. Liu, W. Tu, C. Chen, L. Jin, R. Yang, Q. Wang, S. Zhou, R. Wang, H. Liu, Y. Luo, Y. Liu, G. Shao, H. Li, Z. Tao, Y. Yang, Z. Deng, B. Liu, Z. Ma, Y. Zhang, G. Shi, T. T. Y. Lam, J. T. Wu, G. F. Gao, B. J. Cowling, B. Yang, G. M. Leung and Z. Feng, N. Engl. J. Med., 2020, 382, 1199–1207 CrossRef CAS PubMed.
- M. C. J. Stoddart, H. Ramos, K. Foster and T. Ylä-Anttila, Environ. Commun., 2023, 17, 276–292 CrossRef.
- S. A. Schwartz, Explore, 2020, 16, 141–144 CrossRef PubMed.
- E. Dong, H. Du and L. Gardner, Lancet Infect. Dis., 2020, 20, 533–534 CrossRef CAS PubMed.
- W. Msemburi, A. Karlinsky, V. Knutson, S. Aleshin-Guendel, S. Chatterji and J. Wakefield, Nature, 2023, 613, 130–137 CrossRef CAS PubMed.
- G. Kampf and A. Kramer, Clin. Microbiol. Rev., 2004, 17, 863–893 CrossRef PubMed.
- A. Kratzel, D. Todt, P. V'Kovski, S. Steiner, M. Gultom, T. T. N. Thao, N. Ebert, M. Holwerda, J. Steinmann, D. Niemeyer, R. Dijkman, G. Kampf, C. Drosten, E. Steinmann, V. Thiel and S. Pfaender, Emerging Infect. Dis., 2020, 26, 1592–1595 CrossRef CAS PubMed.
-
Healthcare Infection Control Practices Advisory Committee, CDC Core Practices for Infection Prevention in All Healthcare Settings Centres for Disease Control (USA), Atlanta, GA, 2017 Search PubMed.
-
World Health Organization, Guide to Local Production: WHO-Recommended Handrub Formulations, World Health Organization, Geneva, Switzerland, 2010 Search PubMed.
- L. Cure, R. Van Enk and E. Tiong, Health Care Manag. Sci., 2014, 17, 245–258 Search PubMed.
- A. Deshpande, J. Fox, K. K. Wong, J. L. Cadnum, T. Sankar, A. Jencson, S. Schramm, T. G. Fraser, C. J. Donskey and S. Gordon, Infect. Control Hosp. Epidemiol., 2018, 39, 267–271 CrossRef PubMed.
- D. Singh, K. Joshi, A. Samuel, J. Patra and N. Mahindroo, Epidemiol. Infect., 2020, 148, e229 CrossRef CAS PubMed.
- M. O. Roeslan, M. Richi, A. M. Drestia and A. S. Widyarman, J. Indones. Dent. Association, 2021, 4, 96–103 Search PubMed.
- L. K. Medina-Cordoba, L. L. Valencia-Mosquera, G. P. Tarazona-Diaz and J. D. C. Arias-Palacios, Int. J. Phar. Pharmaceut. Sci., 2018, 10, 104–108 CAS.
-
United States Pharmacopeial Convention, Council of Experts and United States Pharmacopeial Convention, Food Ingredient Expert Committee, Food Chemicals Codex, US Pharmacopeia Conv, 2010 Search PubMed.
- B. B. Hubert, Drug Saf., 1990, 5, 88–94 CrossRef PubMed.
- This includes suppliers in Windsor-Essex with whom the authors worked to source and formulate ABHS is March 2020. The authors receive no financial benefits for these sanitizers and are not involved
in their sale or continued production.
-
Technical-grade ethanol for the manufacture of hand sanitizers and hard-surface disinfectants during the COVID-19 pandemic: Risk assessment summary report, http://www.canada.ca/en/health-canada/services/drugs-health-products/natural-non-prescription/legislation-guidelines/covid19-technical-grade-ethanol-hand-sanitizer/risk-assessment-summary-report.html, accessed January 4, 2021.
-
Temporary Policy for Preparation of Certain Alcohol-Based Hand Sanitizer Products During the Public Health Emergency (COVID-19) Guidance for Industry, https://www.fda.gov/media/136289/download, accessed January 4, 2021.
- K. Abuga and N. Nyamweya, Pharmacy, 2021, 9, 64 CrossRef PubMed.
-
FDA Updates on Hand Sanitizers Consumers Should Not Use, U.S. Food and Drug Administration, Washington, DC, 2021 Search PubMed.
-
Health Canada Warns about Certain Hand Sanitizers that May Pose Health Risks (Part 2 – March 31, 2021 to Present), Health Canada, Ottawa, Canada, 2021 Search PubMed.
- M. Salaspuro, J. Dig. Dis., 2011, 12, 51–59 CrossRef CAS PubMed.
- M. Avanthay, R. B. Bedford, C. S. Begg, D. Böse, J. Clayden, S. A. Davis, J.-C. Eloi, G. P. Goryunov, I. V. Hartung, J. Heeley, K. A. Khaikin, M. O. Kitching, J. Krieger, P. S. Kulyabin, A. J. J. Lennox, R. Nolla-Saltiel, N. E. Pridmore, B. J. S. Rowsell, H. A. Sparkes, D. V. Uborsky, A. Z. Voskoboynikov, M. P. Walsh and H. J. Wilkinson, Nat. Catal., 2021, 4, 994–998 CrossRef.
- C. Heitler, D. B. Scaife and B. W. Thompson, J. Chem. Soc. A Inorg. Phys. Theor., 1967, 1409–1413, 10.1039/J19670001409.
- M. L. Kremer, Nature, 1959, 184, 720 CrossRef CAS.
- C. Anchisi, A. M. Maccioni, C. Sinico and D. Valenti, Il Farmaco, 2001, 56, 427–431 CrossRef CAS PubMed.
-
F. S. Forbes and P. A. Van Splinter, in Encyclopedia of Physical Science and Technology, ed. R. A. Meyers, Academic Press, New York, 3rd edn, 2003, pp. 741–777, DOI:10.1016/B0-12-227410-5/00385-9.
- G. Tafuro, A. Costantini, G. Baratto, L. Busata and A. Semenzato, Ind. Eng. Chem. Res., 2019, 58, 23549–23558 CrossRef CAS.
- S.-Q. Liu and G. J. Pilone, Int. J. Food Sci. Technol., 2000, 35, 49–61 CrossRef CAS.
- T. Takei, N. Iguchi and M. Haruta, Catal. Surv. Asia, 2011, 15, 80–88 CrossRef CAS.
- H. N. Ravishankar, A. V. S. Rao and T. Ramasarma, Mol. Cell. Biochem., 1996, 154, 101–106 CrossRef CAS PubMed.
- S. Rudrawar, R. C. Besra and A. K. Chakraborti, Synthesis, 2006, 2006, 2767–2771 CrossRef.
- M. a. R. Capeletti, L. Balzano, G. de la Puente, M. Laborde and U. Sedran, Appl. Catal., A, 2000, 198, L1–L4 CrossRef CAS.
- G. W. Meadows and B. D. Darwent, Can. J. Chem., 1952, 30, 501–506 CrossRef CAS.
-
T. W. Green and P. G. M. Wuts, in Protective Groups in Organic Synthesis, Wiley-Interscience, New York,NY, 3rd edn, 1999, pp. 724–727 Search PubMed.
- M. Kokkinakis, I. Tsakiris, M. Tzatzarakis, E. Vakonaki, A. Alegakis, S. Papachristou, V. Karzi, A. Kokkinaki, M. Goumenou, M. Kallionakis and A. Kalogeraki, Toxicol Rep, 2020, 7, 1057–1065 CrossRef CAS PubMed.
- P. Ratanatriwong, S. A. Barringer and J. Delwiche, J. Food Sci., 2003, 68, 1542–1547 CrossRef CAS.
- E. A. Blyumberg, G. E. Zaikov, Z. K. Maizus and N.
M. Emanuel, Dokl. Akad. Nauk SSSR, 1960, 133, 144 CAS.
- E. Santacesaria, G. Carotenuto, R. Tesser and M. Di Serio, Chem. Eng. J., 2012, 179, 209–220 CrossRef CAS.
- G. E. Zaikov, Z. K. Maizus, M. I. Vinnik and N. M. Emanuel, Neftekhimiya, 1967, 7, 260 CAS.
-
E. T. Denisov, in Comprehensive Chemical Kinetics, ed. C. H. Bamford and C. F. H. Tipper, Elsevier, 1980, vol. 16, pp. 125–203 Search PubMed.
- A. S. Fauci and G. K. Folkers, J. Infect. Dis., 2023, 228, 422–425 CrossRef PubMed.
- D. M. Morens and A. S. Fauci, Cell, 2020, 182, 1077–1092 CrossRef CAS PubMed.
|
This journal is © The Royal Society of Chemistry 2024 |
Click here to see how this site uses Cookies. View our privacy policy here.