DOI:
10.1039/D4SC04760E
(Edge Article)
Chem. Sci., 2024,
15, 15456-15462
Catalytic enantioselective synthesis of 2-pyrazolines via one-pot condensation/6π-electrocyclization: 3,5-bis(pentafluorosulfanyl)-phenylthioureas as powerful hydrogen bond donors†
Received
17th July 2024
, Accepted 25th August 2024
First published on 26th August 2024
Abstract
A new conjugate-base-stabilized carboxylic acid (CBSCA) containing a 3,5-bis(pentafluorosulfanyl)phenylthiourea functionality catalyses challenging one-pot condensations/6π-electrocyclizations of hydrazines and α,β-unsaturated ketones under mild conditions. Structurally diverse N-aryl 2-pyrazolines are obtained in good yields and enantioselectivities. The superior performance of 3,5-bis(SF5)phenylthioureas over the widely used 3,5-bis(CF3)phenylthioureas is further demonstrated in the Michael addition of dimethyl malonate to nitrostyrene, using a new Takemoto-type catalyst.
Introduction
2-Pyrazolines are valuable structural motifs, exhibiting a range of biological activities including anticancer, anti-inflammatory, antimicrobial, antiviral, and antidiabetic.1 While 2-pyrazolines are most commonly prepared as racemates,2 several mechanistically distinct catalytic enantioselective approaches have emerged. Kanemasa and Kanai reported the first catalytic enantioselective synthesis of 2-pyrazolines: a chiral Lewis acid catalysed 1,3-dipolar cycloaddition of trimethylsilyldiazomethane with chelating dipolarophiles such as 3-crotonoyl-2-oxazolidinone (Scheme 1a(a)).3 Subsequent reports disclosed related catalytic enantioselective 1,3-dipolar cycloadditions of various diazo compounds and dipolarophiles.4,5 Sibi et al. utilized hydrazonyl bromides as precursors to nitrile imines in asymmetric Lewis acid catalysed cycloadditions with 3-crotonoyl-2-oxazolidinone and related substrates (Scheme 1a(b)).6 Brière and coworkers reported an asymmetric phase transfer approach to the synthesis of 2-pyrazolines, involving the conjugate addition of N-Boc hydrazine to α,β-unsaturated ketones, followed by condensation (Scheme 1a(c)).7 Employing related substrates, an iminium catalysis strategy was reported by Deng and coworkers where the initial conjugate addition is facilitated by a 9-epi-amino cinchona alkaloid catalyst (Scheme 1a(d)).8 Dixon et al. used monoalkyl-substituted hydrazine-derived hydrazones, which, in the presence of a cinchona alkaloid derived bifunctional organocatalyst, undergo enantioselective conjugate addition to α,β-unsaturated ketones (Scheme 1a(e)).9,10 Subsequent hydrolysis followed by intramolecular condensation provides the corresponding 2-pyrazolines. N-Sulfonyl hydrazones with pendent alkenes have been shown to undergo enantioselective formation of 2-pyrazolines via organocatalytic iodoaminocyclization11 or via palladium-catalysed aza-Wacker-type cyclization (Scheme 1a(f)).12 Hu and coworkers reported a method for the synthesis of 2-pyrazolines from propargylic acetates and hydrazines, involving a (3 + 2) cycloaddition facilitated by a chiral copper catalyst (Scheme 1a(g)).13 A unique approach to the catalytic enantioselective synthesis of medicinally relevant N-aryl 2-pyrazolines was reported by List and coworkers (Scheme 1b).14 In this transformation, which was first described in its racemic form by Fischer and Knoevenagel,15 hydrazones derived from α,β-unsaturated ketones undergo cyclization in the presence of a BINOL-derived phosphoric acid catalyst to provide N-aryl 2-pyrazolines in good to high enantioselectivity. This represents the first catalytic enantioselective 6π-electrocyclization, a challenging type of reaction for which there are still only a limited number of examples.16,17 Here we report a one-pot condensation/6π-electrocyclization approach to access highly enantioenriched N-aryl 2-pyrazolines directly from hydrazines and α,β-unsaturated ketones (Scheme 1c).18 Reactions are catalysed by a new conjugate-base-stabilized carboxylic acid (CBSCA) containing a 3,5-bis(pentafluorosulfanyl)-phenylthiourea functionality which has not yet been successfully utilized in asymmetric organocatalysis. We further demonstrate the significant potential of 3,5-bis(pentafluorosulfanyl)phenylthioureas in the context of bifunctional catalysis.
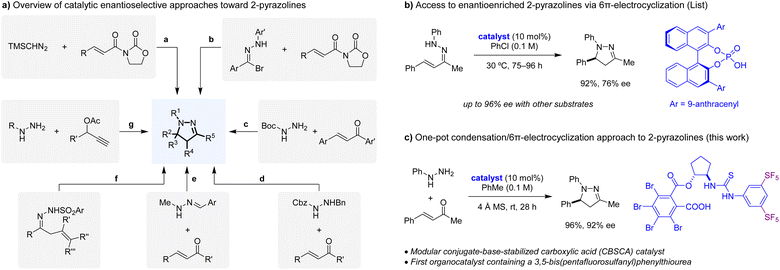 |
| Scheme 1 Relevant precedent on the catalytic enantioselective synthesis of 2-pyrazolines and current work. | |
Results and discussion
Asymmetric Brønsted acid catalysis continues to be dominated by BINOL-derived phosphoric acids and structurally related species.19 With few exceptions, simple carboxylic acids typically lack sufficient acidity to promote a diverse array of reactions.19g,l,20 Driven in large part by the desire to enhance the structural diversity of chiral Brønsted acid catalysts and the reactions they facilitate, our group previously introduced CBSCAs.21 These catalysts contain both a (thio)urea and a carboxylic acid functionality and efficiently facilitate reactions involving iminium and oxocarbenium ions.21 The acidity of CBSCAs, which can exceed that of typical BINOL-derived phosphoric acids,21f,g,22 is largely derived from conjugate base stabilization via anion binding.23 In an effort to further expand the utility of these versatile catalysts, we decided to evaluate CBSCAs in the challenging asymmetric 6π-electrocyclization first reported by List and coworkers.14a,b Preformed hydrazone 1a was selected as the model substrate (Table 1). In the presence of 20 mol% of (1R,2R)-cyclohexane-1,2-diamine-derived carboxylic acid 3a, 1a underwent transformation into the desired 2-pyrazoline 2a at room temperature (Table 1, entry 1). Product 2a was obtained with an encouraging level of enantioselectivity. Remarkably, the closely related (1R,2R)-2-aminocyclohexan-1-ol-derived carboxylic acid catalyst 3b provided a significant boost to both reactivity and enantioselectivity (Table 1, entry 2). Further modifications to the catalyst backbone and aryl substituents on the thiourea functionality did not result in any further improvements (Table 1, entries 3–8).
Table 1 Reaction development with preformed hydrazonea
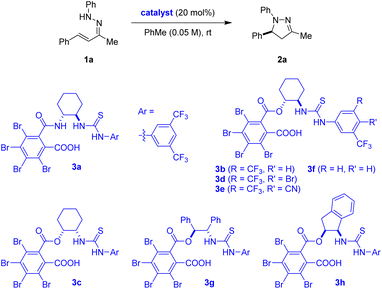
|
Entry |
Catalyst |
Time [h] |
Yield (%) |
ee (%) |
Reactions were performed with 0.1 mmol of 1a. Yields correspond to isolated yields of chromatographically purified products. The ee values were determined by supercritical fluid chromatography analysis.
The opposite enantiomer was obtained.
|
1 |
3a
|
96 |
68 |
75 |
2 |
3b
|
24 |
91 |
90 |
3 |
3c
|
24 |
69 |
5 |
4 |
3d
|
24 |
78 |
87 |
5 |
3e
|
24 |
44 |
39 |
6 |
3f
|
96 |
94 |
84 |
7 |
3g
|
24 |
90 |
61b |
8 |
3h
|
48 |
53 |
31 |
We next sought to develop a one-pot approach to 2-pyrazolines starting from arylhydrazines and α,β-unsaturated ketones, with the additional goals of lowering the catalyst loading and further increasing catalyst efficiency. While the List group also reported a streamlined synthesis of enantioenriched N-aryl pyrazolines from arylhydrazines and α,β-unsaturated ketones, their protocol required initial heating of these starting materials at 50 °C in the presence of molecular sieves but, importantly, in the absence of catalyst. For reasons that remain unclear, the hydrazone formation step (a commonly acid-catalysed process) was found to be incompatible with the phosphoric acid catalyst, and the spent dehydrating agent had to be removed by filtration prior to the addition of the chiral Brønsted acid.14a,b We were pleased to observe that CBSCA 3b efficiently promotes the condensation/6π-electrocyclization of phenylhydrazine with 4-phenyl-3-buten-2-one at room temperature in the presence of 4 Å molecular sieves, providing 2a in excellent yield and enantioselectivity (Table 2, entry 1). Not surprisingly, considering the reduced catalyst loading, the reaction time required doubled compared to the synthesis of 2a from the preformed hydrazone (cf.Table 1, entry 2). Consistent with the results shown in Table 1, catalysts 3d and 3f proved less effective than 3b.
Table 2 Development of the one-pot approacha
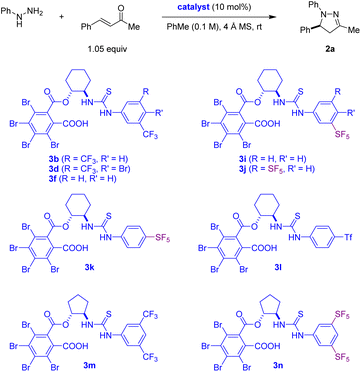
|
Entry |
Catalyst |
Time [h] |
Yield (%) |
ee (%) |
Reactions were performed with 0.1 mmol of phenylhydrazine. Yields correspond to isolated yields of chromatographically purified products. The ee values were determined by supercritical fluid chromatography analysis.
|
1 |
3b
|
48 |
90 |
89 |
2 |
3d
|
48 |
82 |
87 |
3 |
3f
|
96 |
74 |
83 |
4 |
3i
|
40 |
90 |
85 |
5 |
3j
|
28 |
93 |
90 |
6 |
3k
|
48 |
92 |
63 |
7 |
3l
|
48 |
89 |
71 |
8 |
3m
|
48 |
93 |
91 |
9 |
3n
|
28 |
96 |
92 |
To potentially generate more active catalysts, we explored the replacement of trifluoromethyl substituents with pentafluorosulfanyl (SF5) groups. Compared to a CF3 substituent, the SF5 group offers several potential advantages, including increased bulk, electronegativity, and lipophilicity, properties which may lead to favourable characteristics of the corresponding catalysts.24 While CF3 groups are ubiquitous substituents in chiral organocatalysts, and the 3,5-bis(CF3)phenyl substituent in particular is well-recognized as a privileged group,25 the occurrence of SF5 groups in chiral organocatalysts remains rare.26–28 For example, successful applications were reported in the context of asymmetric Brønsted acid catalysis with BINOL-derived catalysts in which 3,5-bis(SF5)phenyl substituents are placed in the 3,3′ positions of the BINOL backbone.26 In the reported cases, these catalysts generally outperform the corresponding catalysts containing 3,5-bis(CF3)phenyl groups. A 3,5-bis(SF5)phenyl group was also incorporated into an axially chiral bifunctional catalyst containing both phosphite and 3,5-bis(CF3)phenylurea functionalities.27 We are aware of only one catalyst containing a 3,5-bis(SF5)phenylthiourea group.28 However, in this case, this substituent offered no advantages over the most effective aryl group. In our case, catalyst 3i containing one SF5 group in the 3-position significantly outperformed the corresponding catalyst 3f containing a single CF3 group (Table 2, entry 4). The performance improved even more dramatically with catalyst 3j containing a 3,5-bis(SF5)phenyl substituent (Table 2, entry 5). Catalysts 3k and 3l containing a SF5 or a triflyl (Tf) group in the 4-position offered no advantages over 3j (Table 2, entries 6 and 7). Finally, a change of the (1R,2R)-2-aminocyclohexan-1-ol backbone to (1R,2R)-2-aminocyclopentan-1-ol provided a boost in enantioselectivity. While this improvement was seen for both 3,5-bis(CF3)phenylthiourea catalyst 3m and 3,5-bis(SF5)phenylthiourea catalyst 3n, the latter outperformed the former in regard to both reactivity and enantioselectivity (Table 2, entries 8 and 9).
With the optimal reaction conditions in hand, the scope of the transformation was evaluated with regard to arylhydrazines and α,β-unsaturated ketones (Scheme 2). Various electronically diverse substituents on the aromatic ring of the enones were well tolerated (products 2a–2i). A furan substituent was also successfully accommodated (product 2j). Similarly, electron-rich and electron-poor hydrazines engaged in the title reaction, providing the corresponding N-aryl 2-pyrazolines in good to high yields and enantioselectivities (products 2l–2n). Addressing a previous limitation,14a,b hydrazones formed in situ from arylhydrazines and enones bearing alkyl groups other than methyl also engaged in enantioselective 6π-electrocyclization reactions. Good enantioselectivities were obtained for non-branched aliphatic substituents (products 2o and 2p) while the introduction of an isopropyl substituent led to a small drop in ee (product 2q). A further drop in enantioselectivity was noted in N-aryl 2-pyrazolines containing a tert-butyl group (products 2r–2t). The synthesis of these materials in enantioenriched form is nevertheless significant. For example, 2-pyrazoline 2t has been identified as a potent and selective allosteric inhibitor of PKCζ, a therapeutic target in pulmonary and hepatic inflammatory diseases.29
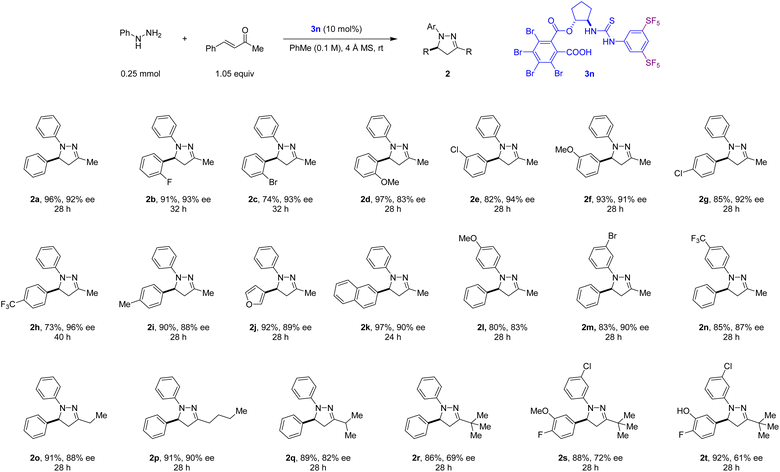 |
| Scheme 2 Reaction scope. | |
To further explore the potential of 3,5-bis(SF5)phenylthioureas as hydrogen bond donor motifs in asymmetric catalysis, we decided to prepare an analogue of the eponymous Takemoto catalyst, replacing the 3,5-bis(CF3)phenylthiourea (Scheme 3). The Takemoto catalyst (5a) and related bifunctional catalysts have been shown to catalyse a broad range of transformations. Catalyst 5a was first utilized in a catalytic enantioselective Michael addition of 1,3-dicarbonyl compounds to nitroalkenes.30,31 In our hands, using catalyst 5a, the reaction of dimethyl malonate to nitrostyrene provided product 4 in 91% yield and 90% ee, following a reaction time of 10 h. The new 3,5-bis(SF5)phenylthiourea containing catalyst 5b furnished 4 in 95% yield and 94% ee within 6 h. The superior performance of 5b in regard to both reactivity and enantioselectivity suggests that the further exploration of bis(SF5)phenylthioureas in asymmetric organocatalysis is warranted.
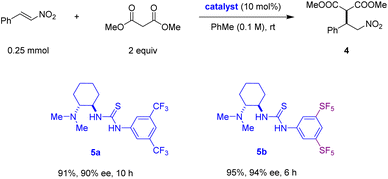 |
| Scheme 3 Enantioselective Michael addition with a new Takemoto-type catalyst. | |
Conclusions
In summary, we have achieved a practical and efficient one-step asymmetric synthesis of 1,3,5-trisubstituted 2-pyrazolines. This method allows for the incorporation of diverse substitution patterns into the 2-pyrazoline scaffold, thereby providing a valuable platform for the synthesis of enantioenriched N-aryl 2-pyrazolines exhibiting promising biological activities. As part of this investigation, we have introduced a new conjugate-base-stabilized carboxylic acid (CBSCA) containing a 3,5-bis(pentafluorosulfanyl)phenylthiourea functionality, a catalyst that was shown to significantly outperform the corresponding catalyst containing a 3,5-bis(trifluoromethyl)phenylthiourea group. The superiority of the 3,5-bis(pentafluorosulfanyl)-phenylthiourea as a hydrogen bond donor was further demonstrated in the context of a catalytic enantioselective Michael addition of dimethyl malonate to nitrostyrene, using a new Takemoto-type catalyst.
Data availability
All supplementary data are available in the ESI.†
Author contributions
M. A. R. R. performed experiments and wrote the original drafts of the manuscript and ESI.† S. D. performed experiments and edited the ESI.† M. O. and C. M. also performed experiments. D. S. conceived of and directed the project and edited the manuscript.
Conflicts of interest
There are no conflicts to declare.
Acknowledgements
This material is based upon work supported by the National Science Foundation under CHE-1856613 and CHE-2154292 (grants to D. S.) We are grateful to Professor William R. Dolbier Jr and Dr Oleksandr Kanishchev (both University of Florida) for a generous donation of 1-bromo-3,5-bis(pentafluorosulfanyl)-benzene. M. O. thanks the Program for Advancing Strategic International Networks to Accelerate the Circulation of Talented Researchers (R2801) from the Japan Society for the Promotion of Science (JSPS). Mass spectrometry instrumentation at the University of Florida was supported by grants from the NIH (S10OD021758-01A1 and S10OD030250-01A1).
Notes and references
- Selected reviews on the importance of 2-pyrazolines in drug discovery:
(a) M. R. Shaaban, A. S. Mayhoub and A. M. Farag, Expert Opin. Ther. Pat., 2012, 22, 253–291 CrossRef CAS PubMed;
(b) A. Marella, M. Rahmat Ali, M. T. Alam, R. Saha, O. Tanwar, M. Akhter, M. Shaquiquzzaman and M. Mumtaz Alam, Mini-Rev. Med. Chem., 2013, 13, 921–931 CrossRef CAS PubMed;
(c) B. Nehra, S. Rulhania, S. Jaswal, B. Kumar, G. Singh and V. Monga, Eur. J. Med. Chem., 2020, 205, 112666 CrossRef CAS PubMed;
(d) D. Matiadis and M. Sagnou, Int. J. Mol. Sci., 2020, 21, 5507 CrossRef CAS PubMed;
(e) M. J. Ahsan, A. Ali, A. Ali, A. Thiriveedhi, M. A. Bakht, M. Yusuf, Salahuddin, O. Afzal and A. S. A. Altamimi, ACS Omega, 2022, 7, 38207–38245 CrossRef CAS PubMed;
(f) R. Kumar, H. Singh, A. Mazumder, Salahuddin and R. K. Yadav, Top. Curr. Chem., 2023, 381, 12 CrossRef CAS PubMed;
(g) L. Ravindar, S. A. Hasbullah, K. P. Rakesh and N. I. Hassan, Eur. J. Pharm. Sci., 2023, 183, 106365 CrossRef CAS PubMed.
- Selected reviews on the synthesis of 2-pyrazolines:
(a) T. Vahedpour, M. Hamzeh-Mivehroud, S. Hemmati and S. Dastmalchi, ChemistrySelect, 2021, 6, 6483–6506 CrossRef CAS;
(b) D. Matiadis, Adv. Synth. Catal., 2023, 365, 1934–1969 CrossRef CAS.
- S. Kanemasa and T. Kanai, J. Am. Chem. Soc., 2000, 122, 10710–10711 CrossRef CAS.
- Examples of other catalytic enantioselective syntheses of 2-pyrazolines via 1,3-dipolar cycloaddition:
(a) T. Kano, T. Hashimoto and K. Maruoka, J. Am. Chem. Soc., 2006, 128, 2174–2175 CrossRef CAS PubMed;
(b) M. P. Sibi, L. M. Stanley and T. Soeta, Org. Lett., 2007, 9, 1553–1556 CrossRef CAS PubMed;
(c) L. Gao, G.-S. Hwang, M. Y. Lee and D. H. Ryu, Chem. Commun., 2009, 5460–5462 RSC;
(d) H. Suga, Y. Furihata, A. Sakamoto, K. Itoh, Y. Okumura, T. Tsuchida, A. Kakehi and T. Baba, J. Org. Chem., 2011, 76, 7377–7387 CrossRef CAS PubMed;
(e) T. Hashimoto, Y. Takiguchi and K. Maruoka, J. Am. Chem. Soc., 2013, 135, 11473–11476 CrossRef CAS PubMed;
(f) S. I. Lee, K. E. Kim, G.-S. Hwang and D. H. Ryu, Org. Biomol. Chem., 2015, 13, 2745–2749 RSC;
(g) B. Zheng, H. Chen, L. Zhu, X. Hou, Y. Wang, Y. Lan and Y. Peng, Org. Lett., 2019, 21, 593–597 CrossRef CAS PubMed;
(h) N. Huang, X. Tong, S. Zhou, Q. Guo and Y. Peng, Adv. Synth. Catal., 2019, 361, 4805–4810 CrossRef CAS;
(i) T. Seo, K. Y. Park, J. Y. Kim and D. H. Ryu, Asian J. Org. Chem., 2023, 12, e202300288 CrossRef CAS.
- Examples of catalytic enantioselective cycloadditions leading to structurally related pyrazolidines:
(a) Y. Yamashita and S. Kobayashi, J. Am. Chem. Soc., 2004, 126, 11279–11282 CrossRef CAS PubMed;
(b) M. Rueping, M. S. Maji, H. B. Küçük and I. Atodiresei, Angew. Chem., Int. Ed., 2012, 51, 12864–12868 CrossRef CAS PubMed;
(c) M. Fernández, E. Reyes, J. L. Vicario, D. Badía and L. Carrillo, Adv. Synth. Catal., 2012, 354, 371–376 CrossRef;
(d) L. Deiana, G.-L. Zhao, H. Leijonmarck, J. Sun, C. W. Lehmann and A. Córdova, ChemistryOpen, 2012, 1, 134–139 CrossRef CAS PubMed;
(e) O. V. Serdyuk, A. Zamfir, F. Hampel and S. B. Tsogoeva, Adv. Synth. Catal., 2012, 354, 3115–3121 CrossRef CAS.
- M. P. Sibi, L. M. Stanley and C. P. Jasperse, J. Am. Chem. Soc., 2005, 127, 8276–8277 CrossRef CAS PubMed.
-
(a) O. Mahé, I. Dez, V. Levacher and J.-F. Brière, Angew. Chem., Int. Ed., 2010, 49, 7072–7075 CrossRef PubMed;
(b) O. Mahé, I. Dez, V. Levacher and J.-F. Brière, Org. Biomol. Chem., 2012, 10, 3946–3954 RSC.
- N. R. Campbell, B. Sun, R. P. Singh and L. Deng, Adv. Synth. Catal., 2011, 353, 3123–3128 CrossRef CAS PubMed.
- C. J. Thomson, D. M. Barber and D. J. Dixon, Angew. Chem., Int. Ed., 2019, 58, 2469–2473 CrossRef CAS PubMed.
- For a recent report using hydrazine in the preparation of bicyclic 2-pyrazolines, see: D. Das, C. Kamilya and P. Ghorai, Org. Lett., 2023, 25, 6993–6998 CrossRef CAS PubMed.
- C. B. Tripathi and S. Mukherjee, Org. Lett., 2014, 16, 3368–3371 CrossRef CAS PubMed.
-
(a) X. Kou, Q. Shao, C. Ye, G. Yang and W. Zhang, J. Am. Chem. Soc., 2018, 140, 7587–7597 CrossRef CAS PubMed . For related strategies, see also: ;
(b) F. Hu, H. Zhang, Y. Chu and X.-P. Hui, Org. Chem. Front., 2022, 9, 2734–2738 RSC;
(c) S. Zhang, S. Wu, Q. Wang, S. Xu, Y. Han, C.-G. Yan, J. Zhang and L. Wang, Angew. Chem., Int. Ed., 2023, 62, e202300309 CrossRef CAS PubMed.
- D.-Y. Zhang, L. Shao, J. Xu and X.-P. Hu, ACS Catal., 2015, 5, 5026–5030 CrossRef CAS.
-
(a) S. Mueller and B. List, Angew. Chem., Int. Ed., 2009, 48, 9975–9978 CrossRef CAS PubMed;
(b) S. Muller and B. List, Synthesis, 2010, 2171–2178 Search PubMed . For a computational analysis of this reaction, see: ;
(c) B. Heggen, M. Patil and W. Thiel, J. Comput. Chem., 2016, 37, 280–285 CrossRef CAS PubMed.
- E. Fischer and O. Knoevenagel, Justus Liebigs Ann. Chem., 1887, 239, 194–206 CrossRef.
- Selected reviews on (asymmetric) electrocyclization reactions:
(a) R. Huisgen, Angew Chem. Int. Ed. Engl., 1980, 19, 947–973 CrossRef;
(b) C. M. Beaudry, J. P. Malerich and D. Trauner, Chem. Rev., 2005, 105, 4757–4778 CrossRef CAS PubMed;
(c) J. L. Vicario and D. Badia, ChemCatChem, 2010, 2, 375–378 CrossRef CAS;
(d) S. Thompson, A. G. Coyne, P. C. Knipe and M. D. Smith, Chem. Soc. Rev., 2011, 40, 4217–4231 RSC;
(e) S. P. Roche, Organics, 2021, 2, 376–387 CrossRef CAS;
(f) Z. Huang, W. Liu and W.-X. Zhang, Chin. J. Chem., 2023, 41, 725–740 CrossRef CAS.
- Examples of catalytic enantioselective 6π electrocyclizations:
(a) E. E. Maciver, S. Thompson and M.
D. Smith, Angew. Chem., Int. Ed., 2009, 48, 9979–9982 CrossRef CAS PubMed;
(b) E. E. Maciver, P. C. Knipe, A. P. Cridland, A. L. Thompson and M. D. Smith, Chem. Sci., 2012, 3, 537–540 RSC;
(c) A. Das, C. M. R. Volla, I. Atodiresei, W. Bettray and M. Rueping, Angew. Chem., Int. Ed., 2013, 52, 8008–8011 CrossRef CAS PubMed;
(d) X.-P. Yin, X.-P. Zeng, Y.-L. Liu, F.-M. Liao, J.-S. Yu, F. Zhou and J. Zhou, Angew. Chem., Int. Ed., 2014, 53, 13740–13745 CrossRef CAS PubMed;
(e) X.-Q. Zhu, Z.-S. Wang, B.-S. Hou, H.-W. Zhang, C. Deng and L.-W. Ye, Angew. Chem., Int. Ed., 2020, 59, 1666–1673 CrossRef CAS PubMed;
(f) X. Wu and C. Sparr, Angew. Chem., Int. Ed., 2022, 61, e202201424 CrossRef CAS PubMed;
(g) B. A. Jones, P. Solon, M. V. Popescu, J.-Y. Du, R. Paton and M. D. Smith, J. Am. Chem. Soc., 2023, 145, 171–178 CrossRef CAS PubMed;
(h) S. Ričko, R. S. Bitsch, M. Kaasik, J. Otevřel, M. H. Madsen, A. Keimer and K. A. Jørgensen, J. Am. Chem. Soc., 2023, 145, 20913–20926 CrossRef PubMed.
- Selected reviews on one-pot reactions:
(a) C. Grondal, M. Jeanty and D. Enders, Nat. Chem., 2010, 2, 167–178 CrossRef CAS PubMed;
(b) C. M. R. Volla, I. Atodiresei and M. Rueping, Chem. Rev., 2014, 114, 2390–2431 CrossRef CAS PubMed;
(c) Y. Hayashi, Chem. Sci., 2016, 7, 866–880 RSC;
(d) Y. Hayashi, Acc. Chem. Res., 2021, 54, 1385–1398 CrossRef CAS PubMed.
- Selected reviews on asymmetric Brønsted acid catalysis:
(a) H. Yamamoto and K. Futatsugi, Angew. Chem., Int. Ed., 2005, 44, 1924–1942 CrossRef CAS PubMed;
(b) T. Akiyama, Chem. Rev., 2007, 107, 5744–5758 CrossRef CAS PubMed;
(c) M. Terada, Synthesis, 2010, 1929–1982 CrossRef CAS;
(d) D. Parmar, E. Sugiono, S. Raja and M. Rueping, Chem. Rev., 2014, 114, 9047–9153 CrossRef CAS PubMed;
(e) T. Akiyama and K. Mori, Chem. Rev., 2015, 115, 9277–9306 CrossRef CAS PubMed;
(f)
M. Rueping, D. Parmar and E. Sugiono, Asymmetric Brønsted Acid Catalysis, Wiley-VCH, Weinheim, 2015 CrossRef;
(g) C. Min and D. Seidel, Chem. Soc. Rev., 2017, 46, 5889–5902 RSC;
(h) R. Mitra and J. Niemeyer, ChemCatChem, 2018, 10, 1221–1234 CrossRef CAS;
(i) L. Schreyer, R. Properzi and B. List, Angew. Chem., Int. Ed., 2019, 58, 12761–12777 CrossRef CAS PubMed;
(j) M. C. Benda and S. France, Org. Biomol. Chem., 2020, 18, 7485–7513 RSC;
(k) G. Caballero-García and J. M. Goodman, Org. Biomol. Chem., 2021, 19, 9565–9618 RSC;
(l) C. Sun and T. Li, ChemistrySelect, 2023, 8, e202300010 CrossRef CAS;
(m) B. Michelet, A. Martin-Mingot, J. Rodriguez, S. Thibaudeau and D. Bonne, Chem.–Eur. J., 2023, 29, e202300440 CrossRef CAS PubMed;
(n) J. K. Cheng, S.-H. Xiang and B. Tan, Chin. J. Chem., 2023, 41, 685–694 CrossRef CAS;
(o) E. I. Jiménez, Org. Biomol.
Chem., 2023, 21, 3477–3502 RSC;
(p) Z.-Y. Han and L.-Z. Gong, Chem. Rec., 2023, 23, e202300049 CrossRef CAS PubMed;
(q) J. P. Handjaya, N. Patankar and J. P. Reid, Chem.–Eur. J., 2024, e202400921 CrossRef CAS PubMed.
- Selected examples of asymmetric Brønsted acid catalysis with chiral carboxylic acids by others:
(a) H. Tohma, S. Takizawa, H. Watanabe, Y. Fukuoka, T. Maegawa and Y. Kita, J. Org. Chem., 1999, 64, 3519–3523 CrossRef CAS PubMed;
(b) N. Momiyama and H. Yamamoto, J. Am. Chem. Soc., 2005, 127, 1080–1081 CrossRef CAS PubMed;
(c) H. Ube, S. Fukuchi and M. Terada, Tetrahedron: Asymmetry, 2010, 21, 1203–1205 CrossRef CAS;
(d) T. Kodama, P. N. Moquist and S. E. Schaus, Org. Lett., 2011, 13, 6316–6319 CrossRef CAS PubMed;
(e) Y. Luan, K. S. Barbato, P. N. Moquist, T. Kodama and S. E. Schaus, J. Am. Chem. Soc., 2015, 137, 3233–3236 CrossRef CAS PubMed;
(f) T. Hashimoto and K. Maruoka, J. Am. Chem. Soc., 2007, 129, 10054–10055 CrossRef CAS PubMed;
(g) T. Hashimoto, M. Hirose and K. Maruoka, J. Am. Chem. Soc., 2008, 130, 7556–7557 CrossRef CAS PubMed;
(h) T. Hashimoto, A. O. Gálvez and K. Maruoka, J. Am. Chem. Soc., 2013, 135, 17667–17670 CrossRef CAS PubMed.
-
(a) C. Min, N. Mittal, D. X. Sun and D. Seidel, Angew. Chem., Int. Ed., 2013, 52, 14084–14088 CrossRef CAS PubMed;
(b) N. Mittal, D. X. Sun and D. Seidel, Org. Lett., 2014, 16, 1012–1015 CrossRef CAS PubMed;
(c) C. Zhao and D. Seidel, J. Am. Chem. Soc., 2015, 137, 4650–4653 CrossRef CAS PubMed;
(d) C. Min, C.-T. Lin and D. Seidel, Angew. Chem., Int. Ed., 2015, 54, 6608–6612 CrossRef CAS PubMed;
(e) M. Odagi, H. Araki, C. Min, E. Yamamoto, T. J. Emge, M. Yamanaka and D. Seidel, Eur. J. Org Chem., 2019, 2019, 486–492 CrossRef CAS;
(f) Z. Zhu, M. Odagi, C. Zhao, K. A. Abboud, H. U. Kirm, J. Saame, M. Lõkov, I. Leito and D. Seidel, Angew. Chem., Int. Ed., 2020, 59, 2028–2032 CrossRef CAS PubMed;
(g) Z. Zhu, M. Odagi, N. Supantanapong, W. Xu, J. Saame, H.-U. Kirm, K. A. Abboud, I. Leito and D. Seidel, J. Am. Chem. Soc., 2020, 142, 15252–15258 CrossRef CAS PubMed;
(h) A. Adili, A. V. Sole, B. Das, M. E. Matter and D. Seidel, Synthesis, 2022, 55, 1724–1735 CrossRef;
(i) X. Hu, Z. Zhu, Z. Li, A. Adili, M. Odagi, K. A. Abboud and D. Seidel, Angew. Chem., Int. Ed., 2024, 63, e202315759 CrossRef CAS PubMed.
- K. Kaupmees, N. Tolstoluzhsky, S. Raja, M. Rueping and I. Leito, Angew. Chem., Int. Ed., 2013, 52, 11569–11572 CrossRef CAS PubMed.
- Selected reviews on asymmetric anion-binding, ion pairing, and cooperative catalysis:
(a) Z. Zhang and P. R. Schreiner, Chem. Soc. Rev., 2009, 38, 1187–1198 RSC;
(b) S. Piovesana, D. M. S. Schietroma and M. Bella, Angew. Chem., Int. Ed., 2011, 50, 6216–6232 CrossRef CAS PubMed;
(c) R. J. Phipps, G. L. Hamilton and F. D. Toste, Nat. Chem., 2012, 4, 603–614 CrossRef CAS PubMed;
(d) A. E. Allen and D. W. C. MacMillan, Chem. Sci., 2012, 3, 633–658 RSC;
(e) J.-F. Brière, S. Oudeyer, V. Dalla and V. Levacher, Chem. Soc. Rev., 2012, 41, 1696–1707 RSC;
(f) M. Mahlau and B. List, Angew. Chem., Int. Ed., 2013, 52, 518–533 CrossRef CAS PubMed;
(g) K. Brak and E. N. Jacobsen, Angew. Chem., Int. Ed., 2013, 52, 534–561 CrossRef CAS PubMed;
(h) D. Seidel, Synlett, 2014, 25, 783–794 CrossRef CAS;
(i) N. Busschaert, C. Caltagirone, W. Van Rossom and P. A. Gale, Chem. Rev., 2015, 115, 8038–8155 CrossRef CAS PubMed;
(j)
Cooperative Catalysis, ed. R. Peters, Wiley-VCH Verlag GmbH & Co. KGaA, Weinheim, Germany, 2015 Search PubMed;
(k) M. D. Visco, J. Attard, Y. Guan and A. E. Mattson, Tetrahedron Lett., 2017, 58, 2623–2628 CrossRef CAS;
(l) J. W. Attard, K. Osawa, Y. Guan, J. Hatt, S.-i. Kondo and A. Mattson, Synthesis, 2019, 51, 2107–2115 CrossRef CAS PubMed;
(m) M. C. Gimeno and R. P. Herrera, Eur. J. Org Chem., 2020, 2020, 1057–1068 CrossRef CAS;
(n) L.-M. Entgelmeier and O. G. Mancheño, Synthesis, 2022, 54, 3907–3927 CrossRef CAS;
(o)
O. G. Mancheño, Anion-Binding Catalysis, Wiley-VCH GmbH, 2022 CrossRef.
- Selected reviews on the synthesis and characteristics of compounds containing pentafluorosulfanyl (SF5) groups:
(a) S. Altomonte and M. Zanda, J. Fluorine Chem., 2012, 143, 57–93 CrossRef CAS;
(b) P. R. Savoie and J. T. Welch, Chem. Rev., 2015, 115, 1130–1190 CrossRef CAS PubMed;
(c)
O. S. Kanishchev and W. R. Dolbier, in Adv. Heterocycl. Chem., ed. E. F. V. Scriven and C. A. Ramsden, Academic Press, 2016, vol. 120, pp. 1–42 Search PubMed;
(d) M. Magre, S. Ni and J. Cornella, Angew. Chem., Int. Ed., 2022, 61, e202200904 CrossRef CAS PubMed;
(e) G. Haufe, Tetrahedron, 2022, 109, 132656 CrossRef CAS;
(f) M. Sani and M. Zanda, Synthesis, 2022, 54, 4184–4209 CrossRef CAS;
(g) R. Kordnezhadian, B.-Y. Li, A. Zogu, J. Demaerel, W. M. De Borggraeve and E. Ismalaj, Chem.–Eur. J., 2022, 28, e202201491 CrossRef CAS PubMed.
- Z. Zhang, Z. Bao and H. Xing, Org. Biomol. Chem., 2014, 12, 3151–3162 RSC.
-
(a) J.-W. Lee and B. List, J. Am. Chem. Soc., 2012, 134, 18245–18248 CrossRef CAS PubMed;
(b) S. Prévost, N. Dupré, M. Leutzsch, Q. Wang, V. Wakchaure and B. List, Angew. Chem., Int. Ed., 2014, 53, 8770–8773 CrossRef PubMed;
(c) J.-H. Tay, A. J. Arguelles and P. Nagorny, Org. Lett., 2015, 17, 3774–3777 CrossRef CAS PubMed;
(d) L. Liu, H. Kim, Y. Xie, C. Farès, P. S. J. Kaib, R. Goddard and B. List, J. Am. Chem. Soc., 2017, 139, 13656–13659 CrossRef CAS PubMed;
(e) J. Lee, A. Borovika, Y. Khomutnyk and P. Nagorny, Chem. Commun., 2017, 53, 8976–8979 RSC;
(f) S. Brunen, B. Mitschke, M. Leutzsch and B. List, J. Am. Chem. Soc., 2023, 145, 15708–15713 CrossRef CAS PubMed.
- Y. Sawamura, Y. Ogura, H. Nakatsuji, A. Sakakura and K. Ishihara, Chem. Commun., 2016, 52, 6068–6071 RSC.
- N. Hayama, R. Kuramoto, T. Földes, K. Nishibayashi, Y. Kobayashi, I. Pápai and Y. Takemoto, J. Am. Chem. Soc., 2018, 140, 12216–12225 CrossRef CAS PubMed.
- M. Abdel-Halim, B. Diesel, A. K. Kiemer, A. H. Abadi, R. W. Hartmann and M. Engel, J. Med. Chem., 2014, 57, 6513–6530 CrossRef CAS PubMed.
-
(a) T. Okino, Y. Hoashi and Y. Takemoto, J. Am. Chem. Soc., 2003, 125, 12672–12673 CrossRef CAS PubMed;
(b) T. Okino, Y. Hoashi, T. Furukawa, X. Xu and Y. Takemoto, J. Am. Chem. Soc., 2005, 127, 119–125 CrossRef CAS PubMed.
- For the most up-to-date mechanistic understanding of this transformation, see: J. A. Izzo, Y. Myshchuk, J. S. Hirschi and M. J. Vetticatt, Org. Biomol. Chem., 2019, 17, 3934–3939 RSC.
Footnote |
† Electronic supplementary information (ESI) available: Experimental procedures and characterization data including NMR spectra. See DOI: https://doi.org/10.1039/d4sc04760e |
|
This journal is © The Royal Society of Chemistry 2024 |
Click here to see how this site uses Cookies. View our privacy policy here.