DOI:
10.1039/D4SC02755H
(Edge Article)
Chem. Sci., 2024,
15, 14968-14976
Triptycene-like naphthopleiadene as a readily accessible scaffold for supramolecular and materials chemistry†
Received
25th April 2024
, Accepted 12th August 2024
First published on 27th August 2024
Abstract
Triptycene derivatives are used extensively in supramolecular and materials chemistry, however, most are prepared using a multi-step synthesis involving the generation of a benzyne intermediate, which hinders production on a large scale. Inspired by the ease of the synthesis of resorcinarenes, we report the rapid and efficient preparation of triptycene-like 1,6,2′,7′-tetrahydroxynaphthopleiadene directly from 2,7-dihydroxynaphthalene and phthalaldehyde. Structural characterisation confirms the novel bridged bicyclic framework, within which the planes of the single benzene ring and two naphthalene units are fixed at an angle of ∼120° relative to each other. Other combinations of aromatic 1,2-dialdehydes and 2,7-disubstituted naphthalenes also provided similar triptycene-like products. The low cost of the precursors and undemanding reaction conditions allow for rapid multigram synthesis of 1,6,2′,7′-tetrahydroxynaphthopleiadene, which is shown to be a useful precursor for making the parent naphthopleiadene hydrocarbon. The great potential for the use of the naphthopleiadene scaffold in supramolecular and polymer chemistry is demonstrated by the preparation of a rigid novel cavitand, a microporous network polymer, and a solution-processable polymer of intrinsic microporosity.
Introduction
Triptycene is a bridged bicyclic hydrocarbon composed of three benzene rings fused to two sp3 hybridised carbon bridgeheads (Fig. 1).1–3 The symmetry and rigidity of triptycene results in its derivatives being used extensively as scaffolds in supramolecular4–10 and materials chemistry.11–20 For example, triptycenes are key components in molecular machines (such as gears,21–24 brakes,25 rotors26), crystal engineering,27,28 macrocycles,7,29,30 cages,31–33 optoelectronic materials,34–40 self-assembling monolayers at interfaces,41–45 and ligands for metal catalysts.46–50 The shape of triptycene, with the three benzene rings fused at an angle of 120° relative to each other, is particularly attractive for providing the vertices within ordered porous materials such as 2D honeycomb-like polymers,51,52 molecular crystals,53,54 nanotubes,55 covalent organic frameworks,56–58 and metal organic frameworks.26,59 However, our long-term interest in triptycene derivatives has been in their use as monomers for making amorphous Polymers of Intrinsic Microporosity (PIMs),60–63 an interest which is shared with several other research groups.64–71 PIMs exploit the rigidity72 of triptycene and the internal molecular free volume (IMFV)73 that originates from its concavities (Fig. 1). Triptycene-derived PIMs have great potential for making high performance gas separation membranes.74,75 Indeed, data from these polymers define the current upper bounds for the trade-off between permeability and selectivity for the separation of several important gas pairs,76,77 including those of interest for post combustion carbon capture and natural gas purification.78
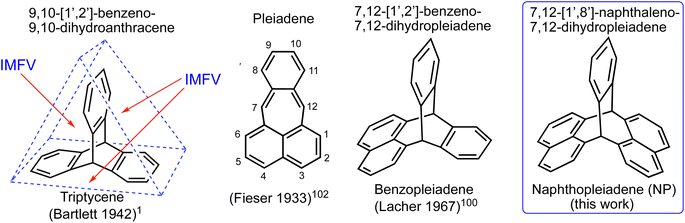 |
| Fig. 1 The structure and nomenclature of triptycene, pleiadene and the two triptycene-like molecular frameworks obtained from the formal fusing of either benzene or naphthalene, via two of its peri-carbons, to the 7,12 sites of pleiadene. The intramolecular free volume (IMFV)73 is indicated for triptycene and this will be enhanced for naphthopleiadene due to the greater width of the naphthalene units. | |
For the diverse applications listed above, the triptycene component generally requires a multi-step synthesis and laborious chromatographic purification of intermediates and product.79,80 Despite some newly developed methods,81–85 triptycene and its derivatives are still predominantly prepared by the Diels–Alder reaction between an anthracene derivative and a reactive benzyne intermediate.86,87 It should be noted that even unsubstituted triptycene is an expensive starting material, presumably due to the inherent difficulties and hazards in scaling-up reactions involving benzyne.88,89 Frustrated by the time-consuming preparation of triptycene monomers for making PIMs, we are developing methods for their rapid synthesis that can be readily performed on a large-scale. Here we report the simple one-step synthesis of a triptycene-like compound, which has a novel bridged bicyclic framework composed of a single benzene and two naphthalene units fused to two carbon bridging atoms, for which we propose the name naphthopleiadene (NP, Fig. 1). The readily prepared derivative NP 1 (Fig. 2), which contains in-built hydroxyl functionality, is useful for further synthetic elaboration as demonstrated by the synthesis of a very rigid cavitand, a microporous network polymer, a solution-processable PIM, and the unsubstituted NP hydrocarbon (Fig. 4).
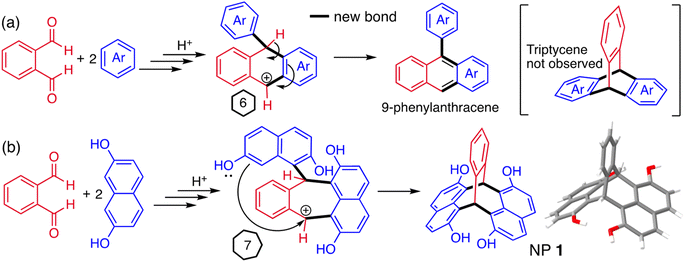 |
| Fig. 2 Proposed partial mechanisms for (a) formation of 9-phenylanthracenes from the reaction between phthalaldehyde and benzene derivatives (Ar) via a carbocation containing in a six-membered ring,90 and (b) the reaction between phthalaldehyde and 2,7-dihydroxynaphthalene to give naphthopleiadene 1 (NP 1), via a carbocation contained within a seven-membered ring which cannot aromatise via loss of proton. | |
Results and discussion
Acid-mediated reactions between aldehydes and electron-rich aromatic compounds were considered for the synthesis of triptycene derivatives, similar to those used for the efficient assembly of resorcinarenes (ESI Fig. 1†).91–93 Naïvely, it was anticipated that these could be formed from the double addition of an appropriate aromatic compound to phthalaldehyde, however, literature reports of such reactions with benzene derivatives present no evidence of any triptycene product.90,94–97 Instead, it is proposed that 9-phenyl anthracenes are produced via a carbocation contained within a six-membered ring, from which the loss of a proton enables the efficient formation of the extended aromatic system (Fig. 2a). On consideration of this mechanism, it was reasoned that a triptycene-like product may be formed if the reaction pathway to 9-phenylanthracene could be blocked by avoiding the six-membered ring intermediate. For example, the double addition of 2,7-dihydroxynaphthalene to phthalaldehyde was anticipated to form a carbocation intermediate contained within a seven-membered ring, which would not be compatible with aromatization (Fig. 2b).
Using reaction conditions optimised for the synthesis of resorcinarenes (ESI Fig. 1†),98,99 heating an acidified ethanol solution of a 2
:
1 mole ratio of 2,7-dihydroxynaphthalene and phthalaldehyde rapidly produced a colourless solid. Intriguingly, 1H and 13C NMR analysis showed that a highly symmetric product was obtained in high purity. A single crystal X-ray diffraction study confirmed the formation of a novel bridged bicyclic framework with two sp3 hybridised bridging carbons, to which are fused a single benzene ring and two naphthalene units, the latter at their peri-(1,8)-positions. Like triptycene, the planes of the three fused aromatic units are fixed at an angle of ∼120° relative to each other (Fig. 2b). The related triptycene-like framework, consisting of two benzene rings and a single naphthalene unit, was reported in 1967.100,101 This bridged bicyclic compound was considered a derivative of the unsaturated hydrocarbon pleiadene,102,103 for which the seven-membered ring evoked the Pleiades of Greek mythology, therefore, it was named 7,12-(1′,2′-benzeno)-7,12-dihydropleiadene (Fig. 1).100,101 Using the same IUPAC endorsed nomenclature,104 we suggest the systematic name of 7,12-(1′,8′-naphthaleno)-7,12-dihydropleiadene for the novel molecular framework, and the trivial name naphthopleiadene (NP) for the parent hydrocarbon (Fig. 1), the synthesis of which is described below (Fig. 4).
Optimisation of the reaction conditions for the synthesis of 1,6,2′,7′-tetrahydroxynaphthopleiadene (NP 1) indicated that only a few drops of concentrated aqueous HCl (37%) are required and that simple alcohols perform best as solvent (ESI Table 1†). NP 1 was produced directly in good yield (∼70%, first crop) and required no further purification except for the removal of alcohol in a drying oven. Additional product (∼20%, second crop) can be obtained by removal of the solvent from the filtrate followed by recrystallisation. Remarkably, the maximum yield is produced within only a few minutes after heating to reflux. Alternatively, a similar yield and purity of product was obtained at ambient temperature, but the reaction took longer to complete (∼24 h). The low cost of the precursors and undemanding reaction conditions allows for large-scale synthesis with, for example, 70 g of NP 1 being obtained easily (ESI Table 1 and Fig. 2†). Water as reaction solvent gave a good crude yield but the resulting solid contained a purple impurity, which required recrystallisation from ethanol to remove, therefore, negating any potential benefits from its use.
Replacement of phthalaldehyde in the reaction with 2,3-naphthalenedialdehyde or 1,2-thiophenedialdehyde also provided triptycene-like products 2 and 3, respectively (Fig. 3). Similarly, 2-hydroxy-7-methoxynapthalene reacts with phthalaldehyde to give a mixture of the isomeric products 1,7′-dihydroxy-6,2′-dimethoxy-naphthopleiadene (4a) and 1,2′-dihydroxy-6,7′-dimethoxy-naphthopleiadene (4b), which could be separated by using the relatively poor solubility of the former in acetone. The same reaction conditions using 2,7-dimethoxynaphthalene and phthalaldehyde failed to provide 1,6,2′,7′-tetramethoxynaphthopleiadene (5). However, by adapting an efficient preparation of methoxy-substituted triphenylmethanes,105 using BF3·OEt2 in dichloromethane, NP 5 was obtained in moderate yield with its structure also confirmed by single crystal XRD analysis.
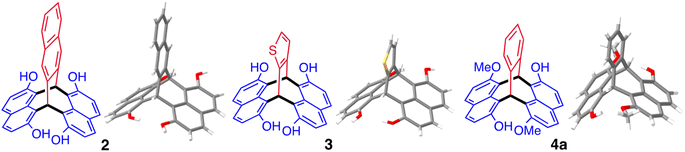 |
| Fig. 3 The structures derived from single crystal; XRD of triptycene-like compounds 2, 3 and 4a. | |
The structure of NP 1, with a short distance between adjacent hydroxyl groups (O to O distance = 2.74 Å), suggested a similar reactivity to that of the resorcinarenes, for which Cram demonstrated deep cavitand formation using the efficient reaction with 2,3-dichloroquinoxaline (ESI Fig. 1†).106 Similarly, the reaction between NP 1 and 2,3-dichloroquinoxaline proceeds in very high yield via the formation of two nine-membered tribenzo-1,4-dioxonine rings (Fig. 4a). A single crystal XRD analysis of the product NP-QO shows a symmetrical cavitand-like structure that contains a single molecule of dichloromethane held within the cavity (Fig. 4b). Unlike Cram's deep cavitands, which exists in rapidly exchanging vase and kite conformers,92,107 the structure of NP-QO appears fully fixed by the NP framework. Indeed, simple molecular mechanical modelling suggest that the conformer produce by the inversion of a single tribenzo-1,4-dioxonine ring is ∼60 kJ mol−1 higher in energy due to the steric effect of the NP bridgehead hydrogen (ESI Fig. 3†).
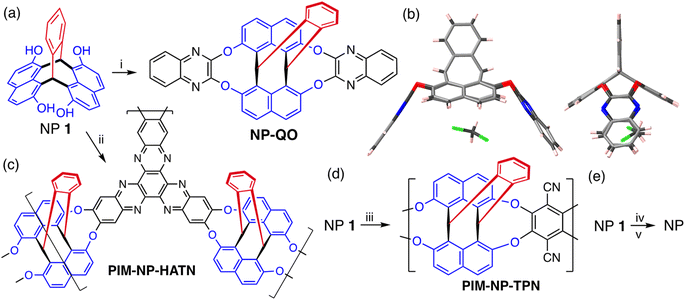 |
| Fig. 4 (a) The synthesis of cavitand NP-QO from NP 1: (i) 2,3-dichloroquinoxaline, K2CO3, DMF, 80 °C, 48 h, yield 100%. (b) Two views of the XRD structure of cavitand NP-QO showing the included CH2Cl2 molecule. (c) The synthesis of an ultramicroporous network polymer PIM-NP-HATN: (ii) 2,3,8,9,14,15-hexafluoro-5,6,11,12,17,18-hexaazatrinaphthylene, K2CO3, DMF, 120 °C, 48 h, yield 86%. (d) The synthesis of soluble PIM-NP-TPN: (iii) 2,3,5,6-tetrafluoroterephthalonitrile, K2CO3, DMF, 30–120 °C, 160 h, yield 84%. (e) (iv) Trifluoromethanesulfonic anhydride, pyridine, 25 °C, 16 h. (v) Pd(OAc)2, 1,3-bis(diphenylphosphino)propane, Et3SiH, DMF, 60 °C, 1 h. | |
The highly efficient formation of the tribenzo-1,4-dioxonine unit, demonstrated by the synthesis of cavitand NP-QO, suggested that polymers may also be prepared by similar aromatic nucleophilic substitution reactions. Hence, the synthesis of both a network and a non-network polymer was attempted by the reactions between NP 1 and 2,3,8,9,14,15-hexafluoro-5,6,11,12,17,18-hexaazatrinaphthylene108 or 2,3,5,6-tetrafluorophthalonitrile, respectively (Fig. 4c and d), with structural characterisation performed by solid-state NMR (ESI Fig. 4†). Thermal Gravimetric Analysis (TGA) of both polymers showed a mass loss of only ∼27% at 850 °C, providing very high char yields that are consistent with the predominately aromatic structure of the polymers (ESI Fig. 5†). For the resulting network polymer NP-HATN, there was low N2 adsorption at 77 K with the shape of the isotherm suggesting slow rate of uptake (ESI Fig. 6†). However, an impressive CO2 uptake of 3.3 mmol g−1 at 1 bar (273 K) suggests significant microporosity with the pore size distribution being predominantly ultramicroporous (<0.7 nm), which combined with high rigidity, severely reduces the rate of N2 adsorption (ESI Fig. 7†). Gas adsorption of the powdered form of the non-network polymer NP-TPN confirmed intrinsic microporosity with significant N2 uptake at 77 K allowing an apparent SABET of 635 m2 g−1 and total pore volume of 0.40 ml g−1 to be estimated. It may be that the non-network structure of PIM-NP-TPN allows some swelling during N2 adsorption, which facilitates greater total uptake. PIM-NP-TPN proved soluble in polar aprotic solvents, and once the polymerisation was optimised, a high average molecular mass (Mn = 140
000 g mol−1) was obtained as measured by gel permeation chromatography. Despite this, only brittle self-standing films of PIM-NP-TPN could be cast from DMF solution, which proved too fragile for gas permeability measurements. It should be noted that the ability to fabricate robust self-standing films from many PIMs, despite their fused ring structures, has been attributed to the relative flexibility of the dibenzodioxane linking group.109 However, a comparison of the flexibility of the dibenzodioxane linking group with that of tribenzo-1,4-dioxonine, using molecular mechanical modelling (ESI Fig. 8†), suggests that the latter is much more rigid, which is likely to be detrimental to the film forming properties of PIM-NP-TPN. Nevertheless, a solution-processable PIM, that contains cavitand-like structures, may be useful for making adsorbents or sensors and, hence, microporous polymers derived from NP 1 is the focus of continuing work.110
As suggested by a reviewer, the viability of using NP 1 as a precursor for making the previously unreported parent NP hydrocarbon (Fig. 1) was established by using the protodehalogenation of its pseudohalide triflate ester (Fig. 4e).111 Although optimisation of this reaction is required, the successful synthesis of NP was confirmed by 1H and 13C NMR and high-resolution mass spectroscopy. It was found that the chemical shift of the 1H NMR signal for the bridgehead hydrogens of the resulting NP (δH = 5.4 ppm, ESI Fig. 15†) is similar to those of triptycene and benzopleiadene.101 In comparison, the bridgehead hydrogens of NPs 1–5 are strongly deshielded relative to those of unsubstituted NP with values in the range 6.8–7.3 ppm, similar to those reported for triptycene derivatives that also contain hydroxyl or methoxy groups adjacent to the bridgehead.50,112,113 The bridgehead hydrogens of NP-QO have an extraordinary chemical shift of 8.79 ppm, which may be attributed to the electron-withdrawing nature of the quinoxaline unit combined with the effect of its ring current.
Conclusions
With the increasing complexity of modern synthetic chemistry, it is gratifying to obtain a novel compound, which possesses both an interesting structure and useful reactivity, from a very simple reaction between two readily available precursors. The ease in which NP 1 is prepared suggests that it will have further applications beyond cavitand and microporous polymer synthesis. For example, NP 1 could replace various hydroxylated triptycene precursors, each of which require a complex multi-step synthesis,114 in the preparation of pincer catalysts50 and materials for which the structure and properties are controlled by triptycene self-assembly. Potential target materials include liquid crystals,112 highly ordered thin-films113 and polymers with unusual mechanical properties.12,14,115 As noted by Swager, triptycene self-assembly is driven by the requirement to eliminate intermolecular free volume (IMFV), thus favouring the interlocking of triptycene units.17,18 As the naphthalene units of NP generate larger IMFV than the benzene rings of triptycene (Fig. 1), the NP component may enhance self-assembly in these materials, in addition to being suitable for scale-up for commercial development. Finally, like their 2,7-dihydroxynaphthalene and 2,7-dimethoxynaphthalene precursors, solutions of NP 1 and NP 5 show visible blue fluorescence (ESI Fig. 16 and 17†), which may form the basis for applications in sensing.116
Data availability
The data supporting this article have been included as part of the ESI.† Crystallographic data has been deposited at the CCDC with the following accession numbers: NP 1 (2286805); NP 2 (2286808); NP 3 (2286809); NP 4a (2286807); NP 5 (2286804) and NP-QO (2286806) and can be obtained from https://www.ccdc.cam.ac.uk/structures/.
Author contributions
MKA led the synthetic effort. CY prepared PIM-NP-TPN, SP prepared NP 5. YL prepared NP 2 and its precursor. DT and SP prepared the NP parent hydrocarbon. DT obtained the UV/Visible and fluorescence spectra. GSN carried out single crystal XRD analysis, NBM conceived the project and wrote the first draft of the manuscript. All authors contributed to writing the final version of the manuscript.
Conflicts of interest
There are no conflicts to declare.
Acknowledgements
MKA thanks the Commonwealth Scholarship Commission and Khulna University for funding. NBM, CY and DT thank the Engineering and Physical Science Research Council, UK, for finding through the programme grant SynHiSel (EP/V047078) and EP/V027735/1.
References
- P. D. Bartlett, M. J. Ryan and S. G. Cohen, Triptycene (9,10-o-benzenoanthracene), J. Am. Chem. Soc., 1942, 64, 2649–2653 CrossRef.
- M. Woźny, A. Mames and T. Ratajczyk, Triptycene derivatives: from their synthesis to their unique properties, Molecules, 2022, 27, 250 CrossRef.
- L. W. Zhao, Z. Li and T. Wirth, Triptycene derivatives: synthesis and applications, Chem. Lett., 2010, 39, 658–667 CrossRef.
- J. H. Chong and M. J. MacLachlan, Iptycenes in supramolecular and materials chemistry, Chem. Soc. Rev., 2009, 38, 3301–3315 RSC.
- Y. Han, Z. Meng, Y.-X. Ma and C.-F. Chen, Iptycene-derived crown ether hosts for molecular recognition and self-assembly, Acc. Chem. Res., 2014, 47, 2026–2040 CrossRef CAS PubMed.
- J. Li, X.-N. Han, H.-Y. Zhou, Y. Han and C.-F. Chen, Helic[1]triptycene[3]arene: synthesis, complexation, and formation of [2]rotaxane shuttle, J. Org. Chem., 2020, 85, 11465–11474 CrossRef CAS PubMed.
- Y. He, X. Yang, M. Qi and C. Chen, Triptycene-derived heterocalixarene: a new type of macrocycle-based stationary phases for gas chromatography, Chin. Chem. Lett., 2021, 32, 2043–2046 CrossRef CAS.
- S. Hasegawa, A. Baksi, B. Chen and G. H. Clever, Guest encapsulation scope of a triptycene-based Pd2L4 coordination cage, Organic Materials, 2022, 4, 222–227 CrossRef CAS.
- M. Kato, T. Fukui, H. Sato, Y. Shoji and T. Fukushima, Capturing the trajectory of metal-ion-cluster formation: stepwise accumulation of Zn(II) ions in a robust coordination space formed by a rigid tridentate carboxylate ligand, Inorg. Chem., 2022, 61, 3649–3654 CrossRef CAS PubMed.
- Z. Liu, W. Song, S. Yang, C. Yuan, Z. Liu, H.-L. Zhang and X. Shao, Marriage of heterobuckybowls with triptycene: molecular waterwheels for separating C60 and C70, Chem.–Eur. J., 2022, 28, e202200306 CrossRef CAS PubMed.
- T. M. Swager, Iptycenes in the design of high performance polymers, Acc. Chem. Res., 2008, 41, 1181–1189 CrossRef.
- F. Ishiwari, G. Okabe, H. Ogiwara, T. Kajitani, M. Tokita, M. Takata and T. Fukushima, Terminal functionalization with a triptycene motif that dramatically changes the structural and physical properties of an amorphous polymer, J. Am. Chem. Soc., 2018, 140, 13497–13502 CrossRef.
- L. Xie, R. Yang, N. Li, P. Froimowicz and K. Zhang, Competitive study of novel triptycene-containing benzoxazine monomers and a thermoresponsive linear main chain-type benzoxazine copolymer: synthesis, polymerization, and thermal properties of their thermosets, Macromolecules, 2022, 55, 6973–6981 CrossRef.
- J. Yu, A. Itagaki, Y. Chen, T. Fukui, F. Ishiwari, T. Kajitani and T. Fukushima, Effective design for long-range polymer ordering using triptycene-containing side chains, Macromolecules, 2023, 56, 4556–4565 CrossRef.
- E. Hoffmeister, J. E. Kropp, T. L. McDowell, R. H. Michel and W. L. Rippie, Triptycene polymers, J. Polym. Sci., Part A: Polym. Chem., 1969, 7, 55–63 CrossRef CAS.
- F. Akutsu, M. Inoki, M. Kondo, T. Inagawa, K. Kayaki and Y. Kasashima, Synthesis and properties of novel polyarylates and poly(ether ether ketone)s derived from 2,7-triptycenediol, Polym. J., 1997, 29, 1023–1028 CrossRef CAS.
- N. T. Tsui, A. J. Paraskos, L. Torun, T. M. Swager and E. L. Thomas, Minimization of internal molecular free volume: a mechanism for the simultaneous enhancement of polymer stiffness, strength, and ductility, Macromolecules, 2006, 39, 3350–3358 CrossRef CAS.
- N. T. Tsui, L. Torun, B. D. Pate, A. J. Paraskos, T.
M. Swager and E. L. Thomas, Molecular barbed wire: threading and interlocking for the mechanical reinforcement of polymers, Adv. Funct. Mater., 2007, 17, 1595–1602 CrossRef CAS.
- Y. C. Liu, S. R. Turner and G. Wilkes, Melt-phase synthesis and properties of triptycene-containing copolyesters, Macromolecules, 2011, 44, 4049–4056 CrossRef CAS.
- S. Mondal and N. Das, Triptycene based organosoluble polyamides: synthesis, characterization and study of the effect of chain flexibility on morphology, RSC Adv., 2014, 4, 61383–61393 RSC.
- W. D. Hounshell, C. A. Johnson, A. Guenzi, F. Cozzi and K. Mislow, Stereochemical consequences of dynamic gearing in substituted bis(9-triptycyl) methanes and related molecules, Proc. Natl. Acad. Sci. U. S. A., 1980, 77, 6961–6964 CrossRef CAS.
- X. Jiang, S. Yang, M. J. Jellen, K. N. Houk and M. Garcia-Garibay, Molecular spur gears with triptycene rotators and a norbornane-based Stator, Org. Lett., 2020, 22, 4049–4052 CrossRef CAS PubMed.
- Y. Gisbert, S. Abid, C. Kammerer and G. Rapenne, Molecular gears: from solution to surfaces, Chem.–Eur. J., 2021, 27, 12019–12031 CrossRef.
- M. J. Jellen, I. Liepuoniute, M. Jin, C. G. Jones, S. Yang, X. Jiang, H. M. Nelson, K. N. Houk and M. A. Garcia-Garibay, Enhanced gearing fidelity achieved through macrocyclization of a solvated molecular spur gear, J. Am. Chem. Soc., 2021, 143, 7740–7747 CrossRef PubMed.
- H. Wang, Q. Guan and X. Wang, Theoretical research of covalent and controllable molecular brake based on 9-triptycene, Theor. Chem. Acc., 2021, 140, 52 Search PubMed.
- M. J. Jellen, X. Jiang, S. Benders, A. Adams and M. A. Garcia-Garibay, Slip/stick viscosity models of nanoconfined liquids: solvent-dependent rotation in metal–organic frameworks, J. Org. Chem., 2022, 87, 1780–1790 CrossRef.
- R. Ushiroguchi, Y. Shuku, R. Suizu and K. Awaga, Variable host–guest charge-transfer interactions in 1D channels formed in a molecule-based honeycomb lattice of phenazine analogue of triptycene, Cryst. Growth Des., 2020, 20, 7593–7597 CrossRef.
- L. Ueberricke and M. Mastalerz, Triptycene end-capping as strategy in materials chemistry to control crystal packing and increase solubility, Chem. Rec., 2021, 21, 558–573 CrossRef CAS PubMed.
- J. C. Lauer, B. Kohl, F. Braun, F. Rominger and M. Mastalerz, A hexagonal shape-persistent nanobelt of elongated rhombic symmetry with orthogonal π-planes by a one-pot reaction, Eur. J. Org Chem., 2022, 2022, e202101317 CrossRef CAS.
- C.-F. Chen and Y. Han, Triptycene-derived macrocyclic arenes: from calixarenes to helicarenes, Acc. Chem. Res., 2018, 51, 2093–2106 CrossRef CAS PubMed.
- S. Hasegawa, S. L. Meichsner, J. J. Holstein, A. Baksi, M. Kasanmascheff and G. H. Clever, Long-lived C60 radical anion stabilized inside an electron-deficient coordination cage, J. Am. Chem. Soc., 2021, 143, 9718–9723 CrossRef CAS.
- M. Holsten, S. Feierabend, S. M. Elbert, F. Rominger, T. Oeser and M. Mastalerz, Soluble congeners of prior insoluble shape-persistent imine cages, Chem.–Eur. J., 2021, 27, 9383–9390 CrossRef CAS PubMed.
- K. Kajiyama, E. Tsurumaki, K. Wakamatsu, G. Fukuhara and S. Toyota, Complexation of an anthracene-triptycene nanocage host with fullerene guests through CH⋯π contacts, ChemPlusChem, 2021, 86, 716–722 CrossRef CAS.
- K. Kawasumi, T. Wu, T. Zhu, H. S. Chae, T. Van Voorhis, M. A. Baldo and T. M. Swager, Thermally activated delayed fluorescence materials based on homoconjugation effect of donor–acceptor triptycenes, J. Am. Chem. Soc., 2015, 137, 11908–11911 CrossRef.
- S. R. Peurifoy, E. Castro, F. Liu, X. Y. Zhu, F. Ng, S. Jockusch, M. L. Steigerwald, L. Echegoyen, C. Nuckolls and T. J. Sisto, Three-dimensional graphene nanostructures, J. Am. Chem. Soc., 2018, 140, 9341–9345 CrossRef PubMed.
- Q. Liu, X. Chen, Z. Zhou, T. Huang, Y. Wang, S. Xie, Z. Zeng and B. Z. Tang, Spiro-fused bicyclo[3,2,2] octatriene-cored triptycene: synthesis, molecular packing, and functional aggregates, Sci. China: Chem., 2021, 64, 1976–1984 CrossRef.
- Y. Duan, G. Zhang, X. Liu, F. Shi, T. Wang, H. Yan, H. Xu and L. Zhang, Acene-extended triptycenes: synthesis, characterization, and singlet exciton fission properties, J. Org. Chem., 2022, 87, 8841–8848 CrossRef PubMed.
- H. Langhals, C. Dietl and P. Mayer, FRET in orthogonal, increasingly strain-rigidified systems, Isr. J. Chem., 2022, 62, e202100021 CrossRef CAS.
- J.-R. Mistry, S. Montanaro and I. A. Wright, Homoconjugation effects in triptycene based organic optoelectronic materials, Mater. Adv., 2023, 4, 787–803 RSC.
- Z.-Y. Tao, Z.-H. Pan, Y.-J. Wang, J. Zhang, Q.-S. Wang, Q.-F. Zhang, B.-H. Tong, M.-K. Fung and H. Kong, Molecular engineering with triptycene groups endows homoleptic Ir(iii) complexes with enhanced electroluminescence properties, Inorg. Chem. Front., 2023, 10, 49–60 RSC.
- S. Das, G. Nascimbeni, R. O. de la Morena, F. Ishiwari, Y. Shoji, T. Fukushima, M. Buck, E. Zojer and M. Zharnikov, Porous honeycomb self-assembled monolayers: tripodal adsorption and hidden chirality of carboxylate anchored triptycenes on Ag, ACS Nano, 2021, 15, 11168–11179 CrossRef CAS.
- L. Grossmann, E. Ringel, A. Rastgoo-Lahrood, B. T. King, J. Rosen, W. M. Heckl, D. Opris, J. Björk and M. Lackinger, Steering self-assembly of three-dimensional iptycenes on Au(111) by Tuning Molecule-Surface Interactions, Angew. Chem., Int. Ed., 2022, 61, e202201044 CrossRef.
- E. Kaletová, C. S. Hurtado, I. Císařová, S. J. Teat and J. Kaleta, Triptycene-based molecular rods for Langmuir-Blodgett monolayers, ChemPlusChem, 2022, 87, e202200023 CrossRef PubMed.
- S. Das, F. Ishiwari, Y. Shoji, T. Fukushima and M. Zharnikov, Triptycene-based self-assembled monolayer as a template for successive click reactions, J. Phys. Chem. C, 2023, 127, 5178–5185 CrossRef.
- S. Das, F. Ishiwari, Y. Shoji, T. Fukushima and M. Zharnikov, Triptycene-based tripodal self-assembled monolayer on indium tin oxide, Nachr. Chem., Tech. Lab., 2023, 127, 2088–2097 Search PubMed.
- F. K.-C. Leung, F. Ishiwari, Y. Shoji, T. Nishikawa, R. Takeda, Y. Nagata, M. Suginome, Y. Uozumi, Y. M. A. Yamada and T. Fukushima, Synthesis and catalytic applications of a triptycene-based monophosphine ligand for palladium-mediated organic transformations, ACS Omega, 2017, 2, 1930–1937 CrossRef CAS PubMed.
- V. A. Kirkina, G. A. Silantyev, S. De-Botton, O. A. Filippov, E. M. Titova, A. A. Pavlov, N. V. Belkova, L. M. Epstein, D. Gelman and E. S. Shubina, Stereoisomerism as an origin of different reactivities of Ir(III) PC(sp3)P pincer catalysts, Inorg. Chem., 2020, 59, 11962–11975 CrossRef CAS.
- I. Kisets and D. Gelman, Synthesis of rigid chiral C3-symmetric triptycene-based ligands and their application in enantioselective Cu(I)-catalyzed aziridination of chalcones, J. Organomet. Chem., 2021, 941, 121804 CrossRef CAS.
- P. S. Kulyabin, G. P. Goryunov, M. I. Sharikov, V. V. Izmer, A. Vittoria, P. H. M. Budzelaar, V. Busico, A. Z. Voskoboynikov, C. Ehm, R. Cipullo and D. V. Uborsky, Ansa-zirconocene catalysts for isotactic-selective propene polymerization at high temperature: a long story finds a happy ending, J. Am. Chem. Soc., 2021, 143, 7641–7647 CrossRef CAS.
- J. Yu, D. Zhang and Q. Wang, Rigid Triptycene-based di- and trinuclear salicylaldiminato nickel cooperative polymerization catalysts, Organometallics, 2023, 42, 307–315 CrossRef CAS.
- D. J. Murray, D. D. Patterson, P. Payamyar, R. Bhola, W. Song, M. Lackinger, A. D. Schlüter and B. T. King, Large area synthesis of a nanoporous two-dimensional polymer at the air/water interface, J. Am. Chem. Soc., 2015, 137, 3450–3453 CrossRef CAS.
- V. Müller, A. Hinaut, M. Moradi, M. Baljozovic, T. A. Jung, P. Shahgaldian, H. Möhwald, G. Hofer, M. Kröger, B. T. King, E. Meyer, T. Glatzel and A. D. Schlüter, A two-dimensional polymer synthesized at the air/water interface, Angew. Chem., Int. Ed., 2018, 57, 10584–10588 CrossRef PubMed.
- C. Zhao, L. Chen, Y. Che, Z. Pang, X. Wu, Y. Lu, H. Liu, G. M. Day and A. I. Cooper, Digital navigation of energy–structure–function maps for hydrogen-bonded porous molecular crystals, Nat. Commun., 2021, 12, 817 CrossRef CAS.
- B. J. Eckstein, L. C. Brown, B. C. Noll, M. P. Moghadasnia, G. J. Balaich and C. M. McGuirk, A porous chalcogen-bonded organic framework, J. Am. Chem. Soc., 2021, 143, 20207–20215 CrossRef CAS PubMed.
- K. Koner, S. Karak, S. Kandambeth, S. Karak, N. Thomas, L. Leanza, C. Perego, L. Pesce, R. Capelli, M. Moun, M. Bhakar, T. G. Ajithkumar, G. M. Pavan and R. Banerjee, Porous covalent organic nanotubes and their assembly in loops and toroids, Nat. Chem., 2022, 14, 507–514 CrossRef CAS PubMed.
- H. Li, J. Ding, X. Guan, F. Chen, C. Li, L. Zhu, M. Xue, D. Yuan, V. Valtchev, Y. Yan, S. Qiu and Q. Fang, Three-dimensional large-pore covalent organic framework with stp topology, J. Am. Chem. Soc., 2020, 142, 13334–13338 CrossRef.
- C. Yu, H. Li, Y. Wang, J. Suo, X. Guan, R. Wang, V. Valtchev, Y. Yan, S. Qiu and Q. Fang, Three-dimensional triptycene-functionalized covalent organic frameworks with hea net for hydrogen adsorption, Angew. Chem., Int. Ed., 2022, 61, e202117101 CrossRef PubMed.
- Y. Zhao, S. Das, T. Sekine, H. Mabuchi, T. Irie, J. Sakai, D. Wen, W. Zhu, T. Ben and Y. Negishi, Record ultralarge-pores, low density three-dimensional covalent organic framework for controlled drug delivery, Angew. Chem., Int. Ed., 2023, 62, e202300172 CrossRef.
- J. Lv, W. Li, J. Li, Z. Zhu, A. Dong, H. Lv, P. Li and B. Wang, A triptycene-based 2D MOF with vertically extended structure for improving the electrocatalytic performance of CO2 to methane, Angew. Chem., Int. Ed., 2023, 62, e202217958 CrossRef PubMed.
- B. S. Ghanem, K. J. Msayib, N. B. McKeown, K. D. M. Harris, Z. Pan, P. M. Budd, A. Butler, J. Selbie, D. Book and A. Walton, A triptycene-based polymer of intrinsic microposity that displays enhanced surface area and hydrogen adsorption, Chem. Commun., 2007, 67–69 RSC.
- B. S. Ghanem, M. Hashem, K. D. M. Harris, K. J. Msayib, M. Xu, P. M. Budd, N. Chaukura, D. Book, S. Tedds, A. Walton and N. B. McKeown, Triptycene-based polymers of intrinsic microporosity: organic materials that can be tailored for gas adsorption, Macromolecules, 2010, 43, 5287–5294 CrossRef CAS.
- N. B. McKeown, Polymers of Intrinsic Microporosity (PIMs), Polymer, 2020, 202, 122736 CrossRef CAS.
- N. B. McKeown, The structure-property relationships of Polymers of Intrinsic Microporosity (PIMs), Curr. Opin. Chem. Eng., 2022, 36, 100785 CrossRef.
- Q.-P. Zhang, Z. Wang, Z.-W. Zhang, T.-L. Zhai, J.-J. Chen, H. Ma, B. Tan and C. Zhang, Triptycene-based chiral porous polyimides for enantioselective membrane separation, Angew. Chem., Int. Ed., 2021, 60, 12781–12785 CrossRef.
- B. S. Ghanem, R. Swaidan, E. Litwiller and I. Pinnau, Ultra-microporous triptycene-based polyimide membranes for high-performance gas separation, Adv. Mater., 2014, 26, 3688–3692 CrossRef PubMed.
- R. Swaidan, M. Al-Saeedi, B. Ghanem, E. Litwiller and I. Pinnau, Rational design of intrinsically ultramicroporous polyimides containing bridgehead-substituted triptycene for highly selective and permeable gas separation membranes, Macromolecules, 2014, 47, 5104–5114 CrossRef.
- J. R. Wiegand, Z. P. Smith, Q. Liu, C. T. Patterson, B. D. Freeman and R. L. Guo, Synthesis and characterization of triptycene-based polyimides with tunable high fractional free volume for gas separation membranes, J. Mater. Chem. A, 2014, 2, 13309–13320 RSC.
- J. R. Weidman and R. L. Guo, The use of iptycenes in rational macromolecular design for gas separation membrane applications, Ind. Eng. Chem. Res., 2017, 56, 4220–4236 CrossRef CAS.
- T. Zhang, L. Deng and P. Li, Decarboxylation cross-linking of triptycene-based Tröger's base polymers for gas separation, Ind. Eng. Chem. Res., 2020, 59, 18640–18648 CrossRef CAS.
- X. Ma, Z. Zhu, W. Shi, W. Ji, J. Li, Y. Wang and I. Pinnau, Unprecedented gas separation performance of a difluoro-functionalized triptycene-based ladder PIM membrane at low temperature, J. Mater. Chem. A, 2021, 9, 5404–5414 RSC.
- B. S. Ghanem, A facile synthesis of a novel triptycene-containing A-B monomer: precursor to polymers of intrinsic microporosity, Polym. Chem., 2012, 3, 96–98 RSC.
- A. Fuoco, B. Comesana-Gandara, M. Longo, E. Esposito, M. Monteleone, I. Rose, C. G. Bezzu, M. Carta, N. B. McKeown and J. C. Jansen, Temperature dependence of gas permeation and diffusion in triptycene-based ultrapermeable Polymers of Intrinsic Microporosity, ACS Appl. Mater. Interfaces, 2018, 10, 36475–36482 CrossRef CAS.
- T. M. Long and T. M. Swager, Minimization of free volume: alignment of triptycenes in liquid crystals and stretched polymers, Adv. Mater., 2001, 13, 601–604 CrossRef CAS.
- I. Rose, M. Carta, R. Malpass-Evans, M.-C. Ferrari, P. Bernardo, G. Clarizia, J. C. Jansen and N. B. McKeown, Highly permeable benzotriptycene-based polymer of intrinsic microporosity, ACS Macro Lett., 2015, 4, 912–915 CrossRef CAS.
- I. Rose, C. G. Bezzu, M. Carta, B. Comesaña-Gándara, E. Lasseuguette, M. C. Ferrari, P. Bernardo, G. Clarizia, A. Fuoco, J. C. Jansen, K. E. Hart, T. P. Liyana-Arachchi, C. M. Colina and N. B. McKeown, Polymer ultrapermeability from the inefficient packing of 2D chains, Nat. Mater., 2017, 16, 932–937 CrossRef CAS PubMed.
- M. Carta, M. Croad, R. Malpass-Evans, J. C. Jansen, P. Bernardo, G. Clarizia, K. Friess, M. Lanc and N. B. McKeown, Triptycene induced enhancement of membrane gas selectivity for microporous Troger's base polymers, Adv. Mater., 2014, 26, 3526–3531 CrossRef CAS.
- R. Swaidan, B. Ghanem and I. Pinnau, Fine-tuned intrinsically ultramicroporous polymers redefine the permeability/selectivity upper bounds of membrane-based air and hydrogen separations, ACS Macro Lett., 2015, 4, 947–951 CrossRef.
- B. Comesaña-Gándara, J. Chen, C. G. Bezzu, M. Carta, I. Rose, M.-C. Ferrari, E. Esposito, A. Fuoco, J. C. Jansen and N. B. McKeown, Redefining the Robeson upper bounds for CO2/CH4 and CO2/N2 separations using a series of ultrapermeable benzotriptycene-based polymers of intrinsic microporosity, Energy Environ. Sci., 2019, 12, 2733–2740 RSC.
- Y. Jiang and C.-F. Chen, Recent developments in synthesis and applications of triptycene and pentiptycene derivatives, Eur. J. Org Chem., 2011, 2011, 6377–6403 CrossRef.
- Y.-X. Ma, Z. Meng and C.-F. Chen, Synthesis of substituted iptycenes, Synlett, 2015, 26, 6–30 CrossRef.
- M. S. Taylor and T. M. Swager, Triptycenediols by rhodium-catalyzed [2 + 2 + 2] cycloaddition, Org. Lett., 2007, 9, 3695–3697 CrossRef CAS PubMed.
- Y. Aida, Y. Shibata and K. Tanaka, Enantioselective synthesis of distorted π-extended chiral triptycenes consisting of three distinct aromatic rings by rhodium-catalyzed [2+2+2] cycloaddition, Chem.–Eur. J., 2020, 26, 3004–3009 CrossRef CAS PubMed.
- B. VanVeller, D. Robinson and T. M. Swager, Triptycene diols: a strategy for synthesizing planar π systems through catalytic conversion of a poly(p-phenylene ethynylene) into a poly(p-phenylene vinylene), Angew. Chem., Int. Ed., 2012, 51, 1182–1186 CrossRef CAS.
- S. Umezu, G. d. P. Gomes, T. Yoshinaga, M. Sakae, K. Matsumoto, T. Iwata, I. Alabugin and M. Shindo, Regioselective One-Pot Synthesis of triptycenes via triple-cycloadditions of arynes to ynolates, Angew. Chem., Int. Ed., 2017, 56, 1298–1302 CrossRef CAS.
- T. Yoshinaga, T. Fujiwara, T. Iwata and M. Shindo, Synthesis of distorted 1,8,13-trisilyl-9-hydroxytriptycenes by triple cycloaddition of ynolates to 3-silylbenzynes, Chem.–Eur. J., 2019, 25, 13855–13859 CrossRef CAS PubMed.
- G. Wittig and R. Ludwig, Triptycen aus anthracen und dehydrobenzol, Angew. Chem., 1956, 68, 40 CrossRef.
- Y. Ryu, C.-S. Hyun and B.-K. An, Practical synthesis of triptycene trisquinone, Synth. Commun., 2022, 52, 1184–1189 CrossRef.
- T. F. Mich, E. J. Nienhous, T. E. Farina and J. J. Tufariel, Generation of benzyne – a warning, J. Chem. Educ., 1968, 45, 272 CrossRef.
- L. Friedman and F. M. Logullo, Benzynes via aprotic diazotization of anthranilic acids: a convenient synthesis of triptycene and derivatives, J. Am. Chem. Soc., 1963, 85, 1549 CrossRef.
- G. K. S. Prakash, C. Panja, A. Shakhmin, E. Shah, T. Mathew and G. A. Olah, BF3−H2O catalyzed hydroxyalkylation of aromatics with aromatic aldehydes and dicarboxaldehydes: efficient synthesis of triarylmethanes, diarylmethylbenzaldehydes, and anthracene Derivatives, J. Org. Chem., 2009, 74, 8659–8668 CrossRef CAS.
- J. B. Niederl and H. J. Vogel, Aldehyde—resorcinol condensations, J. Am. Chem. Soc., 1940, 62, 2512–2514 CrossRef CAS.
- E. Dalcanale, P. Soncini, G. Bacchilega and F. Ugozzoli, Selective complexation of neutral molecules in organic solvents. Host–guest complexes and cavitates between cavitands and aromatic compounds, J. Chem. Soc., Chem. Commun., 1989, 500–502 RSC.
- P. Timmerman, W. Verboom and D. N. Reinhoudt, Resorcinarenes, Tetrahedron, 1996, 52, 2663–2704 CrossRef CAS.
- G. Li, S. Zhou, G. Su, Y. Liu and P. G. Wang, Improved synthesis of aryl-substituted anthracenes and heteroacenes, J. Org. Chem., 2007, 72, 9830–9833 CrossRef CAS PubMed.
- J. A. Clement, R. Sivasakthikumaran, A. K. Mohanakrishnan, S. Sundaramoorthy and D. Velmurugan, Lewis-acid-mediated domino reactions of bis(diacetoxymethyl)-substituted arenes and heteroarenes, Eur. J. Org Chem., 2011, 2011, 569–577 CrossRef.
- R. Sivasakthikumaran, S. M. Rafiq, E. Sankar, J. A. Clement and A. K. Mohanakrishnan, Regioselective annulation of unsymmetrical 1,2-phenylenebis(diaryl/diheteroarylmethanol): a facile synthesis of anthracene, tetracene, and naphtho[b]thiophene analogues, Eur. J. Org Chem., 2015, 2015, 7816–7835 CrossRef CAS.
- K. Mohammadiannejad-Abbasabadi, I. Mohammadpoor-Baltork, S. Tangestaninejad, M. Moghadam, V. Mirkhani and R. Kia, Bi(OTf)3-catalysed domino Friedel–Crafts alkylation of arenes with aldehydes: an upgraded method for efficient synthesis of triarylmethanes and anthracene derivatives, Tetrahedron, 2016, 72, 1433–1439 CrossRef CAS.
- J. P. Kass, L. A. Slasor, C. H. Zambrano and E. E. Dueno, Improved synthesis and purification of cavitands, Org. Prep. Proced. Int., 2006, 38, 480–483 CrossRef CAS.
- H. Ito, T. Nakayama, M. Sherwood, D. Miller and M. Ueda, Characterization and lithographic application of calix[4]resorcinarene derivatives, Chem. Mater., 2008, 20, 341–356 CrossRef CAS.
-
A. J. Lacher, PhD thesis, Conformational analysis of some 7-substituted-7,12-dihydropleiadenes, University of Buffalo, 1967. See footnote 9 in ref. 101 Search PubMed.
- P. T. Lansbury, J. B. Bieber, F. D. Saeva and K. R. Fountain, 7,12-Dihydropleiadenes. VIII. Transannular 1,5 hydride shift accompanying synthesis of 7-alkyl-1,12-(o-phenylene)-7,12-dihydropleiadenes by cyclodehydration, J. Am. Chem. Soc., 1969, 91, 399–405 CrossRef CAS.
- L. F. Fieser and M. Fieser, Condensation and ring closures in the naphthalene series. V. Synthesis of phthaloylnaphthol, J. Am. Chem. Soc., 1933, 55, 3010 CrossRef CAS.
- M. P. Cava and R. H. Schlessinger, Reactive o-quinonoid aromatic hydrocarbons of the pleiadene series, J. Am. Chem. Soc., 1963, 85, 835–836 CrossRef CAS.
- IUPAC, Definitive rules for nomenclature of organic chemistry, J. Am. Chem. Soc., 1960, 82, 5545–5574 CrossRef.
- B. M. Babu, P. B. Thakur, N. N. Rao, G. S. Kumar and H. M. Meshram, A rapid, highly efficient, and general protocol for the synthesis of functionalized triarylmethanes: a straightforward access for the synthesis of (−)-tatarinoid C, Tetrahedron Lett., 2014, 55, 1868–1872 CrossRef CAS.
- J. R. Moran, S. Karbach and D. J. Cram, Cavitands: synthetic molecular vessels, J. Am. Chem. Soc., 1982, 104, 5826–5828 CrossRef CAS.
- J. R. Moran, J. L. Ericson, E. Dalcanale, J. A. Bryant, C. B. Knobler and D. J. Cram, Vases and kites as cavitands, J. Am. Chem. Soc., 1991, 113, 5707–5714 CrossRef CAS.
- P. M. Budd, B. Ghanem, K. Msayib, N. B. McKeown and C. Tattershall, A nanoporous network polymer derived from hexaazatrinaphthylene with potential as an adsorbent and catalyst support, J. Mater. Chem., 2003, 13, 2721–2726 RSC.
- M. Heuchel, D. Fritsch, P. M. Budd, N. B. McKeown and D. Hofmann, Atomistic packing model and free volume distribution of a polymer with intrinsic microporosity (PIM-1), J. Membr. Sci., 2008, 318, 84–99 CrossRef CAS.
- L. P. Skala, A. Yang, M. J. Klemes, L. Xiao and W. R. Dichtel, Resorcinarene cavitand polymers for the remediation of halomethanes and 1,4-dioxane, J. Am. Chem. Soc., 2019, 141, 13315–13319 CrossRef CAS.
- N. Ogawa, Y. Yamaoka, K.-i. Yamada and K. Takasu, Synthesis of π-extended fluoranthenes via a KHMDS-promoted anionic-radical reaction cascade, Org. Lett., 2017, 19, 3327–3330 CrossRef CAS PubMed.
- S. Norvez, Liquid crystalline triptycene derivatives, J. Org. Chem., 1993, 58, 2414–2418 CrossRef CAS.
- N. Seiki, Y. Shoji, T. Kajitani, F. Ishiwari, A. Kosaka, T. Hikima, M. Takata, T. Someya and T. Fukushima, Rational synthesis of organic thin films with exceptional long-range structural integrity, Science, 2015, 348, 1122–1126 CrossRef CAS.
- Y. Li, R. Cao and S. J. Lippard, Design and synthesis of a novel triptycene-based ligand for modeling carboxylate-bridged diiron enzyme active sites, Org. Lett., 2011, 13, 5052–5055 CrossRef CAS PubMed.
- F. Ishiwari, G. Okabe, T. Kajitani and T. Fukushima, Introduction of triptycene with a particular substitution pattern into polymer chains can dramatically improve the structural and rheological properties, ACS Macro Lett., 2021, 10, 1529–1534 CrossRef CAS PubMed.
- R. Kavitha and T. Stalin, Naphthalenediols: a new class of novel fluorescent chemosensors for selective sensing of Cu2+ and Ni2+ in aqueous solution, J. Lumin., 2015, 158, 313–321 CrossRef CAS.
Footnote |
† Electronic supplemental information (ESI) available. Crystallographic data can be obtained from the CCDC using the deposition numbers 2286804–2286809. See DOI:https://doi.org/10.1039/d4sc02755h. |
|
This journal is © The Royal Society of Chemistry 2024 |
Click here to see how this site uses Cookies. View our privacy policy here.