DOI:
10.1039/D4SC01149J
(Edge Article)
Chem. Sci., 2024,
15, 8888-8895
CoH-catalyzed asymmetric remote hydroalkylation of heterocyclic alkenes: a rapid approach to chiral five-membered S- and O-heterocycles†
Received
18th February 2024
, Accepted 7th May 2024
First published on 7th May 2024
Abstract
Saturated heterocycles, which incorporate S and O heteroatoms, serve as fundamental frameworks in a diverse array of natural products, bioactive compounds, and pharmaceuticals. Herein, we describe a unique cobalt-catalyzed approach integrated with a desymmetrization strategy, facilitating precise and enantioselective remote hydroalkylation of unactivated heterocyclic alkenes. This method delivers hydroalkylation products with high yields and excellent stereoselectivity, representing good efficiency in constructing alkyl chiral centers at remote C3-positions within five-membered S/O-heterocycles. Notably, the broad scope and good functional group tolerance of this asymmetric C(sp3)–C(sp3) coupling enhance its applicability.
Introduction
Saturated heterocycles, which incorporate S and O heteroatoms, stand as ubiquitous structural scaffolds found in natural products, bioactive molecules, and synthetic intermediates1 (Fig. 1a). These structurally valuable frameworks offer distinct advantages, including enhanced solubility, improved pharmacokinetics, and increased bioavailability,2 capturing significant attention from both industry and academia. Despite their importance, the aromatic driving force directs the construction toward five-membered heterocyclic aromatic compounds like triazole, imidazole, thiazole, thiophene, and furan. Consequently, synthesizing saturated heterocyclic compounds with diverse structures, especially chiral saturated S- and O-heterocyclic compounds, remains a challenging frontier in asymmetric synthesis. Traditional methods for achieving these compounds typically involve hydrogenation of heteroarenes,3 intramolecular cyclization,4 and annulation reactions.5 However, despite significant progress, these approaches frequently encounter limitations related to the accessibility of starting materials, substrate scope, or stereoselectivity. In light of these challenges, there is a significant demand for the development of more direct and highly stereoselective methods to synthesize chiral saturated S- and O-heterocycles from inexpensive and abundant heterocyclic substrates.
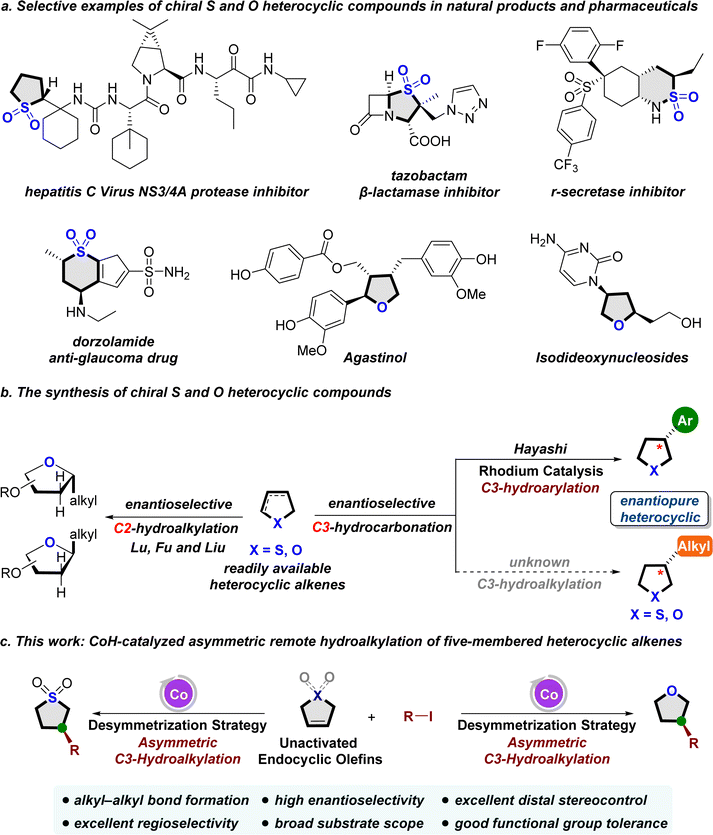 |
| Fig. 1 Background for the development of the current work. | |
Recently, the enantioselective hydrofunctionalization of alkenes catalyzed by transition metals6 has emerged as a central and extensively studied field. This method is particularly valued for its ability to efficiently construct intricate chiral functional molecules and heterocycles in organic synthesis. This approach significantly enhances precision in constructing chiral C–C bonds, offering a universal method to concurrently generate chiral carbon centers and regulate stereochemistry. This capability addresses a fundamental challenge in asymmetric synthesis, which is essential for shaping the core structures of a wide range of organic molecules. Recognizing the importance of chiral saturated heterocycles containing S and O heteroatoms, researchers have explored the feasibility of synthesizing these valuable compounds through a transition metal-catalyzed hydrofunctionalization of heterocyclic alkenes. In this innovative approach, readily available heterocyclic alkenes serve as pro-nucleophiles instead of highly active and unstable organometallic reagents7 in the hydrofunctionalization process to establish a new C–C bond, ultimately realizing the construction of the chiral center on the five-membered saturated S- and O-heterocycles. However, constructing chiral centers on the remote C3 position of a five-membered heterocyclic ring poses a significant challenge. Addressing this challenge, Hayashi et al. recently presented a solution through their report on the rhodium-catalyzed asymmetric hydroarylation of 2,5-dihydrothiophene-1,1-dioxide under neutral conditions (Fig. 1b).8 Despite the considerable advantage demonstrated by this methodology, existing studies predominantly focus on the asymmetric hydroarylation of alkenes, leading to the formation of chiral C3-arylated heterocycles.9 In contrast, methods for asymmetric hydroalkylation reactions, offering a promising pathway to generate diversified chiral alkylated heterocycles by introducing a chiral alkyl center at the remote C3 position after the association with the transition metal. Moreover, the rarity can be ascribed to the inherent challenges linked with establishing chiral C(sp3)–C(sp3) bonds in these reactions.10 To this end, CoH-catalyzed enantioselective reductive hydrocarbonation of alkenes11 has proven to be a feasible and promising method for enantioselective C–C bond formation compared to other transition metals, despite the remaining challenges in the enantioselectivity control. In 2021, Lu and Fu's research group achieved a significant breakthrough in the field of cobalt-catalyzed hydroalkylation reactions, enabling the highly efficient synthesis of chiral alkylated fluoroalkanes.12 Inspired by this work and based on our previous research,13 we wondered if asymmetric hydroalkylation catalyzed by cobalt could be used in the rapid construction of chiral five-membered O/S-heterocyclic rings. Although very recently, Lu, Fu, Liu and coworkers developed the CoH-catalyzed stereoselective reductive hydroalkylation reaction of five-membered glycals for the synthesis of biologically important ribofuranosyl 2-deoxy-C-glycosides constructed alkyl chiral centers at the C2 position (Fig. 1b),14a the asymmetric remote C3-hydroalkylation of five-membered O/S-heterocyclic alkenes still remains a challenge. Herein, we describe the development of new catalytic systems for the regio- and enantioselective hydroalkylation of O/S-endocyclic olefins to synthesize 3-alkylated heterocycles by a cobalt catalyst. The use of Co–H in the presence of different bisoxazoline (BOX) anchoring ligands enables precise stereoselectivity of hydroalkylation, allowing enantioselective access to different saturated heterocycles incorporating S and O heteroatoms. This approach offers precise control over regio- and enantioselectivity under mild reaction conditions (Fig. 1c).
Results and discussion
In our initial investigation, we employed 1-(2-iodoethyl)naphthalene 2a as the alkylation reagent to explore the asymmetric remote hydroalkylation of 2,5-dihydrothiophene-1,1-dioxide 1. Ligand screening experiments highlighted the crucial role of ligands in influencing the activity and stereoselectivity of the reaction (Table 1). The use of the chiral BOX ligand L1 resulted in the desired product in a trace yield. Interestingly, the isopropyl-substituted chiral IndaBox ligand L2 demonstrated promising results, providing the desired product in a 39% yield with 83% ee. However, further screening of modified chiral IndaBox ligands (L3–L6) resulted in significantly reduced yields and enantioselectivities. Similarly, the utilization of chiral Bn-Box ligand L7, chiral Ph-Box ligand L8, and ligand L9 yielded inferior outcomes in terms of both yield and enantioselectivity. To explore the influence of additional reaction parameters on both coupling efficiency and stereoselectivity, various silanes were then investigated, and the results indicated that (MeO)2MeSiH still provided the best outcomes (Table 1, entries 1–3). Evaluation of bases revealed that other bases such as KF, K3PO4, K3PO4·H2O, and Cs2CO3 were less effective than CsF (entries 4–7). The use of alternative cobalt precursors, including CoBr2·glyme, CoCl2, CoCl2(PPh3)2, and CoI2, instead of CoBr2, resulted in a substantial reduction in reactivity and enantioselectivity (entries 8–11). Solvent assessment showed that other ether solvents, such as isopropyl ether and cyclopentyl methyl ether, are unsuitable for this asymmetric hydroalkylation reaction (entries 12 and 13). Interestingly, 1,4-dioxane also yielded comparable results to dimethoxyethane (DME) (entry 14). Considering the importance of reaction temperature, we then explored the effect of different reaction temperatures. The reaction achieved a satisfying level of yield (73%) and enantioselectivity (94%) when conducted at −20 °C (entry 16). Subsequent attempts to refine the experimental conditions did not lead to noteworthy enhancements (further details can be found in the ESI†).
Table 1 Variation of reaction parametersa
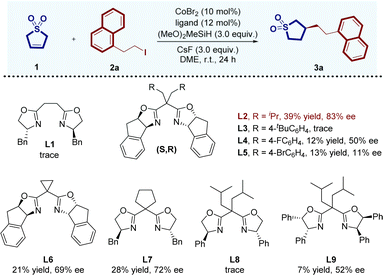
|
Entryb |
Variation from standard conditions |
Yield [%] |
ee [%] |
Summary of selected optimization of reaction conditions: conditions: 1 (0.1 mmol, 1.0 equiv.), 2a (0.2 mmol, 2.0 equiv.), catalyst (10 mol%), ligand (12 mol%), DMMS (0.3 mmol, 3.0 equiv.), CsF (0.3 mmol, 3.0 equiv.), DME (2.0 mL, 0.05 M), 24 h, isolated yields.
L2 as the ligand. The enantiomeric ratio was determined by HPLC analysis using a chiral stationary phase. Abbreviations: DME, 1,2-dimethoxyethane. DMMS, dimethoxymethylsilane.
|
1 |
(EtO)2MeSiH instead of (MeO)2MeSiH |
34 |
80 |
2 |
(MeO)3SiH instead of (MeO)2MeSiH |
23 |
81 |
3 |
(EtO)3SiH instead of (MeO)2MeSiH |
Trace |
— |
4 |
KF instead of CsF |
32 |
66 |
5 |
K3PO4 instead of CsF |
19 |
38 |
6 |
K3PO4·H2O instead of CsF |
19 |
38 |
7 |
Cs2CO3 instead of CsF |
28 |
69 |
8 |
CoBr2·glyme instead of CoBr2 |
27 |
56 |
9 |
CoCl2 instead of CoBr2 |
19 |
27 |
10 |
CoCl2(PPh3)2 instead of CoBr2 |
7 |
17 |
11 |
CoI2 instead of CoBr2 |
33 |
65 |
12 |
Isopropyl ether instead of DME |
Trace |
— |
13 |
Cyclopentyl methyl ether instead of DME |
Trace |
— |
14 |
1,4-Dioxane instead of DME |
33 |
80 |
15 |
0 °C instead of r.t. |
32 |
91 |
16
|
−20 °C instead of r.t. |
73
|
96
|
17 |
−40 °C instead of r.t. |
49 |
90 |
With the established optimized reaction conditions, we systematically explored the universality of the reaction, investigating the versatility of alkyl halides as depicted in Scheme 1. Terminal-substituted alkyl halides, featuring electron-rich and electron-withdrawing groups at the ortho-, meta-, and para-positions of the benzene ring, exhibited competency in this enantioselective reductive C(sp3)–C(sp3) bond formation reaction. This led to the synthesis of the corresponding chiral C3-alkylated sulfolanes 3a–3t, yielding consistently excellent enantiomeric excess values (89–99%) with yields ranging from 46% to 96%. The mild reaction conditions facilitated the incorporation of various synthetically valuable functional groups, including ether (3d), aryl fluoride (3f), and aryl chloride (3g). In addition to the previously mentioned alkyl iodides, a series of longer alkyl chain substituted alkyl iodides (3n–3s) were found to be suitable for this transformation, producing moderate to good yields with high enantioselectivity in the cobalt catalytic system. The relative and absolute configuration of 3p was unequivocally determined through single crystal X-ray analysis, while those of other products were assigned by analogy. Remarkably, the hydroalkylation process accommodated heterocyclic compounds, such as indole-substituted alkyl iodide, leading to smooth reactions with good yields and excellent enantioselectivity (3t).
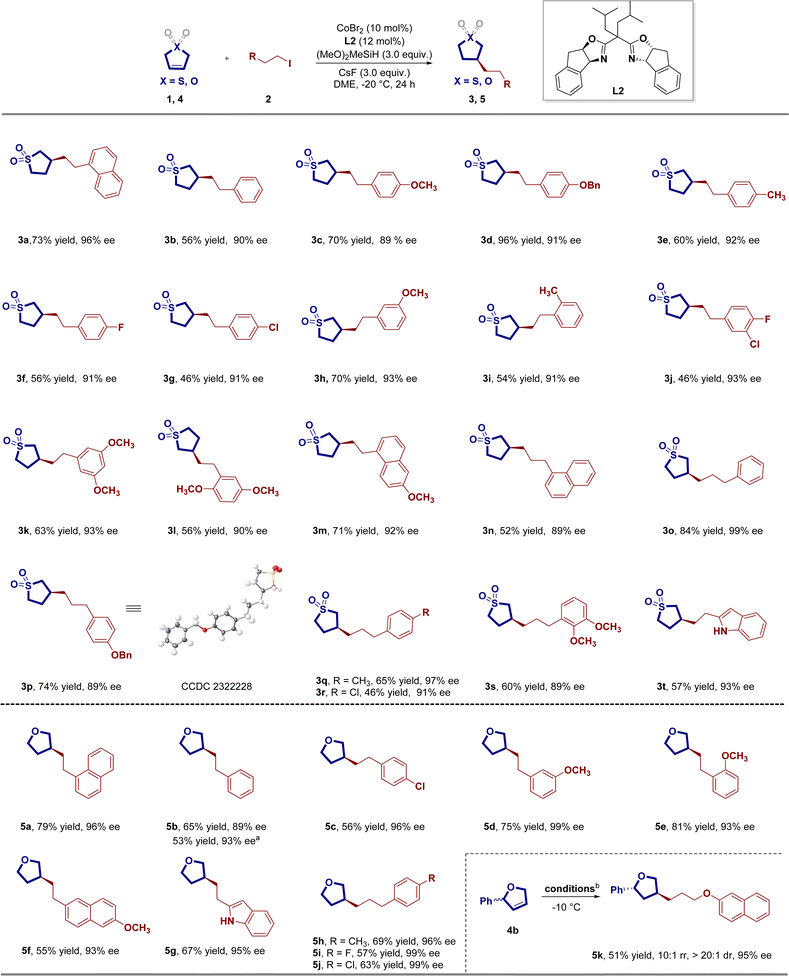 |
| Scheme 1 Reaction scope. Standard conditions: 1 or 4 (0.2 mmol, 1.0 equiv.), 2 (0.4 mmol, 2.0 equiv.), L2 (12 mol%), DMMS (0.6 mmol, 3.0 equiv.), CsF (0.6 mmol, 3.0 equiv.), DME (2.0 mL, 0.1 M), isolated yields. Conditions a: phenethyl bromide (2.0 equiv.) and sodium iodide (2.0 equiv.). Conditions b: Alkene (3.0 equiv.) and alkyl halide (1.0 equiv.). The enantiomeric ratio was determined by HPLC analysis using a chiral stationary phase. The dr and rr value was determined by NMR spectroscopy of the crude reaction mixture and GC analysis. DME = 1,2-dimethoxyethane, DMMS = dimethoxymethylsilane. | |
Efforts to obtain another enantioenriched class of important chiral organic skeletons, specifically C3-alkylated tetrahydrofurans, led us to explore the CoH-catalyzed asymmetric remote hydroalkylation of 2,5-dihydrofuran, with results summarized in Scheme 1. Encouragingly, using the established optimized reaction conditions, we successfully generated the desired hydroalkylation products in good yields with excellent enantiomeric excesses (ee). In the exploration of terminal-substituted alkyl halides, different substituents with varied electron properties at the ortho, meta, and para positions of the benzene ring were well-tolerated. This resulted in the formation of the desired chiral C3-alkylated tetrahydrofuran (5a–5f) with yields ranging from 60% to 82% and enantiomeric excess (ee) values between 96% and 99%. Notably, even reactions involving heterocycle-substituted alkyl halides proceeded efficiently, delivering the desired products 5g with high efficiency. Furthermore, we extended the applicability of aryl ethyl iodines to longer chain aryl propyl iodines, yielding the corresponding chiral C3-alkylated tetrahydrofurans 5h–5j with uniformly good yield and excellent stereoselectivity (57–69% yield, 96–99% ee). Notably, the cobalt-catalyzed hydroalkylation of racemic internal alkene 4b has also demonstrated the ability to furnish C(sp3)–C(sp3) coupling product 5k featuring 1,3-diastereocenters with remarkable levels of regio-, enantio-, and diastereoselectivity.
To showcase the synthetic utility of the reaction, several transformations were then conducted (Scheme 2). Firstly, this method proved valuable for accessing C3-alkylated sulfolane/tetrahydrofurans of drug molecules and natural products with high efficiency, such as acemetacin (product 3u and 5l) and estrone (product 5m). Additionally, the obtained chiral C3-alkylated sulfolane product 3b could be easily reduced to yield the chiral C3-alkylated tetrahydrothiophene 6b which is difficult to synthesize by traditional methods.
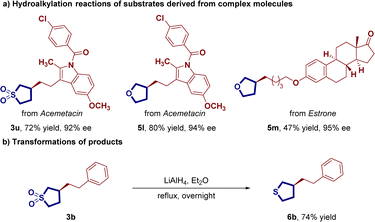 |
| Scheme 2 Synthetic applications. | |
To gain insight into the reaction mechanism, we conducted a series of preliminary experiments. In Scheme 3a, alkene 2,5-dihydrothiophene-1,1-dioxide 1, in the presence of 2.0 equivalent of the “radical-clock” (iodomethyl)cyclopropane 2v, was subjected to the standard reaction conditions, yielding the ring-opening product 3v in 17% yield. This result suggests that the C−I bond cleavage involves a radical pathway. Additionally, introducing a stoichiometric amount of the radical inhibitor 2,2,6,6-tetramethylpiperidinooxy (TEMPO) into the asymmetric hydroalkylation reaction of 1 with 2a significantly inhibited the reaction, strongly indicating the participation of a radical pathway in the reaction process. To delve deeper into the mechanism, we conducted isotopic labeling experiments using deuterated silane (PhSiD2) as the hydride source, as depicted in Scheme 3b. These experiments revealed deuterium incorporation at the C4-position of 3a–d, with 99% D, indicating that the migration insertion of Co–H with 1 might be irreversible.
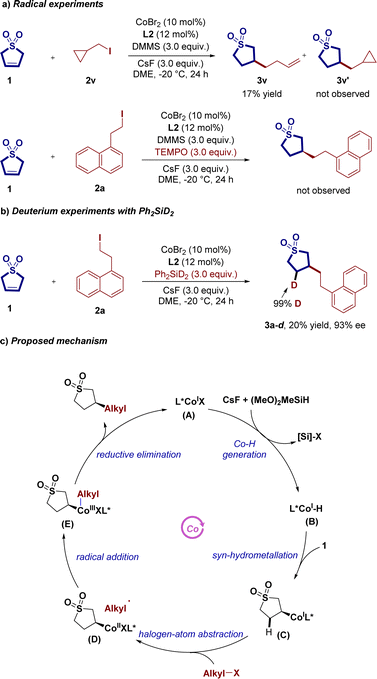 |
| Scheme 3 Mechanistic investigations and proposed mechanism. | |
Based on the conducted experiments and insights from previous literature reports,14 we present the proposed catalytic cycles in Scheme 3c. The catalytic cycle begins with the ligated CoIX precursor A, which can generate the cobalt hydride species CoIH (B) in the presence of silane and base. The intermediate B then undergoes alkene hydrometallation with substrate 1 to generate the intermediate C. Subsequently, C participates in halogen-atom abstraction and radical addition processes, producing alkylcobalt(II) (D) and dialkylcobalt(III) (E). Finally, reductive elimination of E releases the desired C3-selective hydroalkylation product and regenerates A.
Conclusions
In conclusion, we have described a highly efficient cobalt-catalyzed regio- and enantioselective hydroalkylation of unactivated O/S-heterocyclic alkenes. This method provides a practical approach to generating alkyl chiral centers at remote positions within five-membered O/S-heterocycles, achieving excellent regio- and enantioselectivity through a desymmetrization strategy. Notably, the broad scope and excellent functional group tolerance of this asymmetric C(sp3)–C(sp3) coupling enhance its applicability. Ongoing efforts in our laboratory are dedicated to exploring further applications and gaining a deeper understanding of the underlying mechanisms of this strategy.
Data availability
The data supporting this article have been uploaded as part of the ESI.†
Author contributions
Z. R. conceived the project. L. Z., F. L. and Y. Z. performed the experiments. L. Z., F. L. and Y. Z. analysed and interpreted the experimental data. L. Z., F. L. and Z. R. drafted the paper. Y. Z., M. S. and J. X. supervised the project. All the authors discussed the results and contributed to the preparation of the final manuscript.
Conflicts of interest
There are no conflicts to declare.
Acknowledgements
We are grateful for the generous financial support from the National Natural Science Foundation of China (21801206), Shaanxi Fundamental Science Research Project for Chemistry & Biology (22JHQ002), the Program for Young Talents of Shaanxi Province (5113190023), and Natural Science Basic Research Program of Shaanxi (2024JC-ZDXM-08).
Notes and references
-
(a) G. Zeni and R. C. Larock, Chem. Rev., 2004, 104, 2285–2310 CrossRef CAS PubMed;
(b) M. Yamada, T. Ichikawa, M. Ii, K. Itoh, N. Tamura and T. Kitazaki, Biorg. Med. Chem., 2008, 16, 3941–3958 CrossRef CAS PubMed;
(c) C. Liu, X. Kui, Q. Lu, H. Liu and D. Qian, Chem Catal., 2024, 4, 100798–100818 CrossRef CAS;
(d) B. R. Beno, K.-S. Yeung, M. D. Bartberger, L. D. Pennington and N. A. Meanwell, J. Med. Chem., 2015, 58, 4383–4438 CrossRef CAS PubMed;
(e) J. Bao, J.-F. Luo, X.-C. Qin, X.-Y. Xu, X.-Y. Zhang, Z.-C. Tu and S.-H. Qi, Bioorg. Med. Chem. Lett., 2014, 24, 2433–2436 CrossRef CAS PubMed.
-
(a) A. B. Smith and C. M. Adams, Acc. Chem. Res., 2004, 37, 365–377 CrossRef CAS PubMed;
(b) A. Bitto, L. Minutoli, F. Squadrito, F. Polito and D. Altavilla, Mini-Rev. Med. Chem., 2007, 7, 339–343 CrossRef CAS PubMed;
(c) N. Negoro, S. Sasaki, S. Mikami, M. Ito, Y. Tsujihata, R. Ito, M. Suzuki, K. Takeuchi, N. Suzuki, J. Miyazaki, T. Santou, T. Odani, N. Kanzaki, M. Funami, A. Morohashi, M. Nonaka, S. Matsunaga, T. Yasuma and Y. Momose, J. Med. Chem., 2012, 55, 3960–3974 CrossRef CAS PubMed;
(d) E. Vitaku, D. T. Smith and J. T. Njardarson, J. Med. Chem., 2014, 57, 10257–10274 CrossRef CAS PubMed;
(e) R. D. Taylor, M. MacCoss and A. D. G. Lawson, J. Med. Chem., 2014, 57, 5845–5859 CrossRef CAS PubMed;
(f) S. Serra, Tetrahedron: Asymmetry, 2014, 25, 1561–1572 CrossRef CAS;
(g) J. Liu, S. Dasgupta and M. P. Watson, Beilstein J. Org. Chem., 2015, 11, 2696–2706 CrossRef CAS PubMed;
(h) Y. Liu, W. Qin and H. Yan, Synlett, 2016, 27, 2756–2760 CrossRef CAS;
(i) Á. M. Montaña, S. Ponzano, M.-F. Sanasi and G. Kociok-Köhn, Org. Biomol. Chem., 2018, 16, 1557–1580 RSC;
(j) M. D. Delost, D. T. Smith, B. J. Anderson and J. T. Njardarson, J. Med. Chem., 2018, 61, 10996–11020 CrossRef CAS PubMed;
(k) T. Jia, M. Wang and J. Liao, Top. Curr. Chem., 2019, 377, 8–36 CrossRef PubMed;
(l) Y. Ning, T. Ohwada and F.-E. Chen, Green Synth. Catal., 2021, 2, 247–266 CrossRef;
(m) X.-B. Liu and Y. Huang, Coord. Chem. Rev., 2023, 489, 215173–215212 CrossRef CAS;
(n) X. Kong, Q. Liu, Y. Chen, W. Wang, H. Chen, W. Wang, S. Zhang, X. Chen and Z.-Y. Cao, Green Chem., 2024, 26, 3435–3440 RSC.
-
(a) Y.-G. Zhou, Acc. Chem. Res., 2007, 40, 1357–1366 CrossRef CAS PubMed;
(b) Y. Qin, J. Lv and S. Luo, Tetrahedron Lett., 2014, 55, 551–558 CrossRef CAS;
(c) L. Shi, B. Wei, X. Yin, P. Xue, H. Lv and X. Zhang, Org. Lett., 2017, 19, 1024–1027 CrossRef CAS PubMed;
(d) K. Meng, J. Xia, Y. Wang, X. Zhang, G. Yang and W. Zhang, Org. Chem. Front., 2017, 4, 1601–1605 RSC;
(e) Q. Yan, G. Xiao, Y. Wang, G. Zi, Z. Zhang and G. Hou, J. Am. Chem. Soc., 2019, 141, 1749–1756 CrossRef CAS PubMed;
(f) M. P. Wiesenfeldt, Z. Nairoukh, T. Dalton and F. Glorius, Angew. Chem., Int. Ed., 2019, 58, 10460–10476 CrossRef CAS;
(g) Z.-H. Zhang, H. Wei, Z.-L. Li and X.-Y. Liu, Synlett, 2020, 32, 362–369 CrossRef.
-
(a) I. Nakamura and Y. Yamamoto, Chem. Rev., 2004, 104, 2127–2198 CrossRef CAS PubMed;
(b) S. R. Chemler and P. H. Fuller, Chem. Soc. Rev., 2007, 36, 1153–1160 RSC;
(c) N. Krause and C. Winter, Chem. Rev., 2011, 111, 1994–2009 CrossRef CAS PubMed;
(d) R. I. McDonald, G. Liu and S. S. Stahl, Chem. Rev., 2011, 111, 2981–3019 CrossRef CAS PubMed;
(e) Y. Park, Y. Kim and S. Chang, Chem. Rev., 2017, 117, 9247–9301 CrossRef CAS PubMed;
(f) X. Bao, Q. Wang and J. Zhu, Angew. Chem., Int. Ed., 2017, 56, 9577–9581 CrossRef CAS PubMed;
(g) X. Ma, I. R. Hazelden, T. Langer, R. H. Munday and J. F. Bower, J. Am. Chem. Soc., 2019, 141, 3356–3360 CrossRef CAS PubMed;
(h) K. E. Poremba, S. E. Dibrell and S. E. Reisman, ACS Catal., 2020, 10, 8237–8246 CrossRef CAS;
(i) X. Huang, M. H. Nguyen, M. Pu, L. Zhang, Y. R. Chi, Y.-D. Wu and J. S. Zhou, Angew. Chem., Int. Ed., 2020, 59, 10814–10818 CrossRef CAS PubMed;
(j) Y. Ping and W. Kong, Synthesis, 2020, 52, 979–992 CrossRef;
(k) C.-X. Ye and E. Meggers, Acc. Chem. Res., 2023, 56, 1128–1141 CrossRef CAS PubMed;
(l) Y.-C. Nie, F. Yang, Y.-H. Li and R. Zhu, Org. Lett., 2023, 25, 889–894 CrossRef CAS PubMed;
(m) Z. Ma, W. Xu, Y.-D. Wu and J. S. Zhou, J. Am. Chem. Soc., 2023, 145, 16464–16473 CrossRef CAS PubMed.
-
(a) L.-Q. Lu, J.-R. Chen and W.-J. Xiao, Acc. Chem. Res., 2012, 45, 1278–1293 CrossRef CAS;
(b) Y. Deng, Q.-Q. Cheng and M. P. Doyle, Synlett, 2017, 28, 1695–1706 CrossRef CAS;
(c) N. Kaur, F. Wu, N.-E. Alom, J. P. Ariyarathna, S. J. Saluga and W. Li, Org. Biomol. Chem., 2019, 17, 1643–1654 RSC;
(d) L. Wei, X. Chang and C.-J. Wang, Acc. Chem. Res., 2020, 53, 1084–1100 CrossRef CAS PubMed;
(e) J. Ke, W.-C. C. Lee, X. Wang, Y. Wang, X. Wen and X. P. Zhang, J. Am. Chem. Soc., 2022, 144, 2368–2378 CrossRef CAS PubMed;
(f) G. Shen, F. He, W. Xie, H. Gu and X. Yang, ACS Catal., 2023, 13, 12472–12480 CrossRef CAS.
- For selected reviews, see:
(a) S. Z. Tasker, E. A. Standley and T. F. Jamison, Nature, 2014, 509, 299–309 CrossRef CAS PubMed;
(b) Y. Nakajima and S. Shimada, RSC Adv., 2015, 5, 20603–20616 RSC;
(c) J. R. Coombs and J. P. Morken, Angew. Chem., Int. Ed., 2016, 55, 2636–2649 CrossRef CAS PubMed;
(d) A. Vasseur, J. Bruffaerts and I. Marek, Nat. Chem., 2016, 8, 209–219 CrossRef CAS PubMed;
(e) S. W. M. Crossley, C. Obradors, R. M. Martinez and R. A. Shenvi, Chem. Rev., 2016, 116, 8912–9000 CrossRef CAS PubMed;
(f) J. Chen, J. Guo and Z. Lu, Chin. J. Chem., 2018, 36, 1075–1109 CrossRef CAS;
(g) J. V. Obligacion and P. J. Chirik, Nat. Rev. Chem, 2018, 2, 15–34 CrossRef CAS PubMed;
(h) J. Chen and Z. Lu, Org. Chem. Front., 2018, 5, 260–272 RSC;
(i) H. Wen, G. Liu and Z. Huang, Coord. Chem. Rev., 2019, 386, 138–153 CrossRef CAS;
(j) X.-X. Wang, X. Lu, Y. Li, J.-W. Wang and Y. Fu, Sci. China Chem., 2020, 63, 1586–1600 CrossRef CAS;
(k) X. Qi and T. Diao, ACS Catal., 2020, 10, 8542–8556 CrossRef CAS PubMed;
(l) D. Janssen-Müller, B. Sahoo, S.-Z. Sun and R. Martin, Isr. J. Chem., 2020, 60, 195–206 CrossRef;
(m) R. Y. Liu and S. L. Buchwald, Acc. Chem. Res., 2020, 53, 1229–1243 CrossRef CAS PubMed;
(n) J. Derosa, O. Apolinar, T. Kang, V. T. Tran and K. M. Engle, Chem. Sci., 2020, 11, 4287–4296 RSC;
(o) J. Guo, Z. Cheng, J. Chen, X. Chen and Z. Lu, Acc. Chem. Res., 2021, 54, 2701–2716 CrossRef CAS PubMed;
(p) X.-Y. Sun, B.-Y. Yao, B. Xuan, L.-J. Xiao and Q.-L. Zhou, Chem Catal., 2022, 2, 3140–3162 CrossRef CAS;
(q) Y. Wang, Y. He and S. Zhu, Acc. Chem. Res., 2022, 55, 3519–3536 CrossRef CAS PubMed;
(r) P. Lu and Z. Lu, Synthesis, 2023, 55, 1042–1052 CrossRef CAS;
(s) Z. Zhao, F. Zhang, D. Wang and L. Deng, Chin. J. Chem., 2023, 41, 3063–3081 CrossRef CAS;
(t) L.-J. Li, Y. He, Y. Yang, J. Guo, Z. Lu, C. Wang, S. Zhu and S.-F. Zhu, CCS Chem., 2023, 1–48 Search PubMed;
(u) J. Han, R. He and C. Wang, Chem Catal., 2023, 3, 100690–100700 CrossRef CAS;
(v) W. Zhao, H.-X. Lu, W.-W. Zhang and B.-J. Li, Acc. Chem. Res., 2023, 56, 308–321 CrossRef CAS PubMed ; for selected examples, see:;
(w) Y. Xi, T. W. Butcher, J. Zhang and J. F. Hartwig, Angew. Chem., Int. Ed., 2016, 55, 776–780 CrossRef CAS PubMed;
(x) J. Sun and L. Deng, ACS Catal., 2016, 6, 290–300 CrossRef CAS;
(y) S. Mishra, J. Liu and A. Aponick, J. Am. Chem. Soc., 2017, 139, 3352–3355 CrossRef CAS PubMed;
(z) L. Li, T. Gong, X. Lu, B. Xiao and Y. Fu, Nat. Commun., 2017, 8, 345–352 CrossRef PubMed;
(a
a) S.-Z. Sun, M. Börjesson, R. Martin-Montero and R. Martin, J. Am. Chem. Soc., 2018, 140, 12765–12769 CrossRef CAS PubMed;
(a
b) H. Sommer, F. Juliá-Hernández, R. Martin and I. Marek, ACS Cent. Sci., 2018, 4, 153–165 CrossRef CAS PubMed;
(a
c) J.-S. Lin, T.-T. Li, J.-R. Liu, G.-Y. Jiao, Q.-S. Gu, J.-T. Cheng, Y.-L. Guo, X. Hong and X.-Y. Liu, J. Am. Chem. Soc., 2019, 141, 1074–1083 CrossRef CAS PubMed;
(a
d) J. Long, P. Wang, W. Wang, Y. Li and G. Yin, iScience, 2019, 22, 369–379 CrossRef CAS PubMed;
(a
e) K. Duvvuri, K. R. Dewese, M. M. Parsutkar, S. M. Jing, M. M. Mehta, J. C. Gallucci and T. V. RajanBabu, J. Am. Chem. Soc., 2019, 141, 7365–7375 CrossRef CAS PubMed;
(a
f) W.-W. Zhang, S.-L. Zhang and B.-J. Li, Angew. Chem., Int. Ed., 2020, 59, 6874–6880 CrossRef CAS PubMed;
(a
g) X. Wei, W. Shu, A. García-Domínguez, E. Merino and C. Nevado, J. Am. Chem. Soc., 2020, 142, 13515–13522 CrossRef CAS PubMed;
(a
h) X.-X. Wang, X. Lu, S.-J. He and Y. Fu, Chem. Sci., 2020, 11, 7950–7956 RSC;
(a
i) X. Shen, X. Chen, J. Chen, Y. Sun, Z. Cheng and Z. Lu, Nat. Commun., 2020, 11, 783–791 CrossRef PubMed;
(a
j) L. Guo, M. Yuan, Y. Zhang, F. Wang, S. Zhu, O. Gutierrez and L. Chu, J. Am. Chem. Soc., 2020, 142, 20390–20399 CrossRef CAS PubMed;
(a
k) Y. He, C. Liu, L. Yu and S. Zhu, Angew. Chem., Int. Ed., 2020, 59, 21530–21534 CrossRef CAS PubMed;
(a
l) Y. Li, D. Wu, H.-G. Cheng and G. Yin, Angew. Chem., Int. Ed., 2020, 59, 7990–8003 CrossRef CAS PubMed;
(a
m) S. Cuesta-Galisteo, J. Schörgenhumer, X. Wei, E. Merino and C. Nevado, Angew. Chem., Int. Ed., 2021, 60, 1605–1609 CrossRef CAS PubMed;
(a
n) M. M. Parsutkar and T. V. RajanBabu, J. Am. Chem. Soc., 2021, 143, 12825–12835 CrossRef CAS PubMed;
(a
o) D. Qian, S. Bera and X. Hu, J. Am. Chem. Soc., 2021, 143, 1959–1967 CrossRef CAS PubMed;
(a
p) L. Wang, W. Lu, J. Zhang, Q. Chong and F. Meng, Angew. Chem., Int. Ed., 2022, 61, e202205624 CrossRef CAS;
(a
q) Y.-J. Liang, M.-J. Sun, G. Zhang, J.-J. Yin, W. Guan, T. Xiong and Q. Zhang, Chem Catal., 2022, 2, 2379–2390 CrossRef CAS;
(a
r) Y. Zhao and S. Ge, Angew. Chem., Int. Ed., 2022, 61, e202116133 CrossRef CAS PubMed;
(a
s) P. Lu, H. Wang, Y. Mao, X. Hong and Z. Lu, J. Am. Chem. Soc., 2022, 144, 17359–17364 CrossRef PubMed;
(a
t) L. Li, J. Ren, J. Zhou, X. Wu, Z. Shao, X. Yang and D. Qian, Nat. Commun., 2022, 13, 6861–6871 CrossRef PubMed;
(a
u) M. Hu, B. B. Tan and S. Ge, J. Am. Chem. Soc., 2022, 144, 15333–15338 CrossRef CAS PubMed;
(a
v) W. Dong, Z. Ye and W. Zhao, Angew. Chem., Int. Ed., 2022, 61, e202117413 CrossRef CAS PubMed;
(a
w) B. B. Tan, M. Hu and S. Ge, Angew. Chem., Int. Ed., 2023, 62, e202307176 CrossRef CAS PubMed;
(a
x) L. Talavera, R. R. A. Freund, H. Zhang, M. Wakeling, M. Jensen and R. Martin, ACS Catal., 2023, 13, 5538–5543 CrossRef CAS PubMed;
(a
y) M.-S. Liu and W. Shu, JACS Au, 2023, 3, 1321–1327 CrossRef CAS PubMed;
(b
`) S. Wang, L. Shi, X.-Y. Chen and W. Shu, Angew. Chem., Int. Ed., 2023, 62, e202303795 CrossRef PubMed;
(b
a) Z.-C. Wang, X. Luo, J.-W. Zhang, C.-F. Liu, M. J. Koh and S.-L. Shi, Nat. Catal., 2023, 6, 1087–1097 CrossRef CAS;
(b
b) C. Chen, W. Guo, D. Qiao, J. Zhou, Y. Wang and S. Zhu, CCS Chem., 2023, 1–7 Search PubMed;
(b
c) M. Zhang, Z. Ye and W. Zhao, Angew. Chem., Int. Ed., 2023, 62, e202306248 CrossRef PubMed;
(b
d) H.-D. He, R. Chitrakar, Z.-W. Cao, D.-M. Wang, L.-Q. She, P.-G. Zhao, Y. Wu, Y.-Q. Xu, Z.-Y. Cao and P. Wang, Angew. Chem., Int. Ed., 2024, 63, e202313336 CrossRef CAS PubMed.
- For selected reviews, see:
(a) J. Liu, Y. Ye, J. L. Sessler and H. Gong, Acc. Chem. Res., 2020, 53, 1833–1845 CrossRef CAS PubMed;
(b) K. Juhász, Á. Magyar and Z. Hell, Synthesis, 2020, 53, 983–1002 Search PubMed;
(c) D. Haas, J. M. Hammann, R. Greiner and P. Knochel, ACS Catal., 2016, 6, 1540–1552 CrossRef CAS;
(d) G. C. Fu, ACS Cent. Sci., 2017, 3, 692–700 CrossRef CAS PubMed;
(e) J. B. Diccianni and T. Diao, Trends Chem., 2019, 1, 830–844 CrossRef CAS;
(f) A. H. Cherney, N. T. Kadunce and S. E. Reisman, Chem. Rev., 2015, 115, 9587–9652 CrossRef CAS PubMed.
- K. M.-H. Lim and T. Hayashi, J. Am. Chem. Soc., 2015, 137, 3201–3204 CrossRef CAS PubMed.
- For selected examples about the construction of chiral arylated five-membered heterocycles, see:
(a) Z. Yang and J. Zhou, J. Am. Chem. Soc., 2012, 134, 11833–11835 CrossRef CAS PubMed;
(b) S. Xu, Y. Ping, W. Li, H. Guo, Y. Su, Z. Li, M. Wang and W. Kong, J. Am. Chem. Soc., 2023, 145, 5231–5241 CrossRef CAS PubMed;
(c) W.-Q. Wu, Q. Peng, D.-X. Dong, X.-L. Hou and Y.-D. Wu, J. Am. Chem. Soc., 2008, 130, 9717–9725 CrossRef CAS PubMed;
(d) T. Tu, X.-L. Hou and L.-X. Dai, Org. Lett., 2003, 5, 3651–3653 CrossRef CAS PubMed;
(e) X. Shu, D. Zhong, Y. Lin, X. Qin and H. Huo, J. Am. Chem. Soc., 2022, 144, 8797–8806 CrossRef CAS PubMed;
(f) Y. Shen, Y. Gu and R. Martin, J. Am. Chem. Soc., 2018, 140, 12200–12209 CrossRef CAS PubMed;
(g) F. Ozawa, A. Kubo and T. Hayashi, J. Am. Chem. Soc., 1991, 113, 1417–1419 CrossRef CAS;
(h) F. Ozawa and T. Hayashi, J. Organomet. Chem., 1992, 428, 267–277 CrossRef CAS;
(i) D. Mc Cartney and P. J. Guiry, Chem. Soc. Rev., 2011, 40, 5122–5150 RSC;
(j) K. M.-H. Lim and T. Hayashi, J. Am. Chem. Soc., 2017, 139, 8122–8125 CrossRef CAS PubMed;
(k) B. Li, B. Luo, H. Yang and W. Tang, Angew. Chem., Int. Ed., 2022, 61, e202209087 CrossRef CAS PubMed;
(l) H.-J. Jiang, X.-M. Zhong, J. Yu, Y. Zhang, X. Zhang, Y.-D. Wu and L.-Z. Gong, Angew. Chem., Int. Ed., 2019, 58, 1803–1807 CrossRef CAS PubMed;
(m) P. Jain, P. Verma, G. Xia and J.-Q. Yu, Nat. Chem., 2017, 9, 140–144 CrossRef CAS PubMed;
(n) X. Huang, S. Teng, Y. R. Chi, W. Xu, M. Pu, Y.-D. Wu and J. S. Zhou, Angew. Chem., Int. Ed., 2021, 60, 2828–2832 CrossRef CAS PubMed;
(o) L. F. Tietze and K. Thede, Chem. Commun., 1999, 1811–1812 RSC;
(p) F. de Azambuja, R. C. Carmona, T. H. D. Chorro, G. Heerdt and C. R. D. Correia, Chem.–Eur. J, 2016, 22, 11205–11209 CrossRef CAS PubMed;
(q) J. A. Carmona, V. Hornillos, P. Ramírez-López, A. Ros, J. Iglesias-Sigüenza, E. Gómez-Bengoa, R. Fernández and J. M. Lassaletta, J. Am. Chem. Soc., 2018, 140, 11067–11075 CrossRef CAS PubMed;
(r) During the submission of this manuscript, the efficient regiodivergent and enantioselective synthesis of cyclic sulfones through ligand-controlled nickel-catalyzed hydroalkylation was reported: C. Fan, U. Dhawa, D. Qian, D. Sakic, J. Morel and X. Hu, Angew. Chem., Int. Ed., 2024, e202406767 Search PubMed.
- For selected reviews, see:
(a) Z. Dong, Z. Ren, S. J. Thompson, Y. Xu and G. Dong, Chem. Rev., 2017, 117, 9333–9403 CrossRef CAS PubMed;
(b) T. Xiong and Q. Zhang, Chem. Soc. Rev., 2021, 50, 8857–8873 RSC;
(c) J. Diccianni, Q. Lin and T. Diao, Acc. Chem. Res., 2020, 53, 906–919 CrossRef CAS PubMed;
(d) Z. Zhang, S. Bera, C. Fan and X. Hu, J. Am. Chem. Soc., 2022, 144, 7015–7029 CrossRef CAS PubMed;
(e) S. Bera, R. Mao and X. Hu, Nat. Chem., 2021, 13, 270–277 CrossRef CAS PubMed;
(f) L. Shi, L.-L. Xing, W.-B. Hu and W. Shu, Angew. Chem., Int. Ed., 2021, 60, 1599–1604 CrossRef CAS PubMed;
(g) X.-X. Wang, L. Yu, X. Lu, Z.-L. Zhang, D.-G. Liu, C. Tian and Y. Fu, CCS Chem., 2021, 4, 605–615 CrossRef;
(h) J.-B. Ma, X. Zhao, D. Zhang and S.-L. Shi, J. Am. Chem. Soc., 2022, 144, 13643–13651 CrossRef CAS PubMed;
(i) J.-W. Wang, D.-G. Liu, Z. Chang, Z. Li, Y. Fu and X. Lu, Angew. Chem., Int. Ed., 2022, 61, e202205537 CrossRef CAS PubMed;
(j) X.-X. Wang, Y.-T. Xu, Z.-L. Zhang, X. Lu and Y. Fu, Nat. Commun., 2022, 13, 1890–1900 CrossRef CAS PubMed;
(k) L. Zhao, Y. Zhu, M. Liu, L. Xie, J. Liang, H. Shi, X. Meng, Z. Chen, J. Han and C. Wang, Angew. Chem., Int. Ed., 2022, 61, e202204716 CrossRef CAS PubMed;
(l) J.-W. Wang, Z. Li, D. Liu, J.-Y. Zhang, X. Lu and Y. Fu, J. Am. Chem. Soc., 2023, 145, 10411–10421 CrossRef CAS PubMed.
-
(a) Y. Li, D. Liu, L. Wan, J.-Y. Zhang, X. Lu and Y. Fu, J. Am. Chem. Soc., 2022, 144, 13961–13972 CrossRef CAS PubMed;
(b) X. Wu, H. Xia, C. Gao, B. Luan, L. Wu, C. Zhang, D. Yang, L. Hou, N. Liu, T. Xia, H. Li, J. Qu and Y. Chen, Nat. Chem., 2024, 16, 398–407 CrossRef CAS PubMed;
(c) G. Zhang and Q. Zhang, Chem Catal., 2023, 3, 100526 CrossRef CAS;
(d) Z. L. Zhang, Z. Li, Y. T. Xu, L. Yu, J. Kuang, Y. Li, J. W. Wang, C. Tian, X. Lu and Y. Fu, Angew. Chem., Int. Ed., 2023, 62, e202306381 CrossRef CAS PubMed;
(e) B. Liu and Q. Liu, ChemCatChem, 2024, 16, e202301188 CrossRef CAS;
(f) T. Xia, Y. Wu, J. Hu, X. Wu, J. Qu and Y. Chen, Angew. Chem., Int. Ed., 2024, e202316012 CAS;
(g) T. Xia, W. Wu, X. Wu, J. Qu and Y. Chen, Angew. Chem., Int. Ed., 2024, e202318991 Search PubMed;
(h) Y. Li, X. Lu and Y. Fu, CCS Chem., 2024, 6, 1130–1156 CrossRef.
- Y. Li, W. Nie, Z. Chang, J.-W. Wang, X. Lu and Y. Fu, Nat. Catal., 2021, 4, 901–911 CrossRef CAS.
- X. Wang, J. Xue and Z.-Q. Rong, J. Am. Chem. Soc., 2023, 145, 15456–15464 CrossRef CAS.
-
(a) B. Liu, D. Liu, X. Rong, X. Lu, Y. Fu and Q. Liu, Angew. Chem., Int. Ed., 2023, 62, e202218544 CrossRef CAS PubMed;
(b) Z.-L. Zhang, Z. Li, Y.-T. Xu, L. Yu, J. Kuang, Y. Li, J.-W. Wang, C. Tian, X. Lu and Y. Fu, Angew. Chem., Int. Ed., 2023, 62, e202306381 CrossRef CAS PubMed;
(c) M. Zeng, C. Yu, Y. Wang, J. Wang, J. Wang and H. Liu, Angew. Chem., Int. Ed., 2023, 62, e202300424 CrossRef CAS;
(d) During our manuscript preparation and submission process, an excellent work on a ligand-controlled cobalt-hydride-catalyzed regio-, enantio-, and diastereoselective oxyheterocyclic alkene hydroalkylation was reported: Z. Li, B. Liu, C.-Y. Yao, G.-W. Gao, J.-Y. Zhang, Y.-Z. Tong, J.-X. Zhou, H.-K. Sun, Q. Liu, X. Lu and Y. Fu, Ligand-Controlled Cobalt-Catalyzed Regio-, Enantio-, and Diastereoselective Oxyheterocyclic Alkene Hydroalkylation, J. Am. Chem. Soc., 2024, 146, 3405–3415 CrossRef CAS PubMed.
Footnotes |
† Electronic supplementary information (ESI) available. CCDC 2322228. For ESI and crystallographic data in CIF or other electronic format see DOI: https://doi.org/10.1039/d4sc01149j |
‡ These authors contributed equally to this work. |
|
This journal is © The Royal Society of Chemistry 2024 |
Click here to see how this site uses Cookies. View our privacy policy here.