DOI:
10.1039/D3SC04543A
(Edge Article)
Chem. Sci., 2024,
15, 3707-3710
Ambiphilicity of ring-expanded N-heterocyclic carbenes†
Received
29th August 2023
, Accepted 18th January 2024
First published on 31st January 2024
Abstract
N-heterocyclic carbenes, such as imidazole-2-ylidenes and imidazolin-2-ylidenes, the popular class of singlet carbenes introduced by Arduengo in 1991 have not been shown to be ambiphilic owing to the two σ-withdrawing, π-donating amino groups flanking the carbene centre. However, our experimental data suggest that ring-expanded N-heterocyclic carbenes (RE-NHCs), especially the seven and eight membered rings, are significantly ambiphilic. Our results also show that the steric environment in RE-NHCs can become a determining factor for controlling the E–H bond activation.
Introduction
Thanks to growing efforts in main group chemistry, the activation of enthalpically strong bonds and industrially relevant small molecules is no longer restricted to transition-metals.1 More than a decade ago, our group discovered that cyclic (alkyl)(amino)carbenes (CAAC-5),2,3 a class of highly ambiphilic carbenes, could react with carbon monoxide,4 H2,5 NH3 (ref. 5) and P4.6 More recently, it has been shown that CAAC-5s not only activate a variety of bonds (C–H, Si–H, B–H…)7 but also promote catalytic reactions.8 In comparison, imidazole-2-ylidenes9 and imidazolin-2-ylidenes,10 the classical N-heterocyclic carbenes (NHC-5s), are much less ambiphilic due to their two π-donating amino substituents. Consequently, they are reluctant to activate small molecules, as illustrated by their lack of reactivity with CO.11,12 Much less studied than NHC-5s are the so-called ring-expanded N-heterocyclic carbenes (RE-NHCs).13 Herein we compare the ambiphilic nature of NHC-5 with RE-NHCs (−6,13a −7 (ref. 14) and −8 (ref. 13c)) and CAAC-5 through DFT calculations and their reactivity with small molecules.
Results and discussion
Compared to NHC-5s, RE-NHCs display a larger N–C–N bond angle (∠carb) which imposes greater steric constraint when used as a ligand for transition metals, a feature used to enhance catalytic activity.15 Arguably less emphasized, is the larger carbene bond angle, which increases the p-character of the lone pair, and thus the energy level of the HOMO.16 Comparatively, the LUMO is less affected since ring expansion does not significantly disrupt the planarization of the α-amino fragments, which leaves the mesomeric stabilization of the pπ orbital by the nitrogen lone pairs nearly identical.
The ambiphilicity of a carbene can be estimated computationally by considering the singlet–triplet gap (ΔES–T) (Scheme 1). As expected, our calculations indicate a correlation between the ring size and ambiphilicity of a carbene. Interestingly, the data also suggests that the ambiphilicity of NHC-7 and NHC-8 approaches that of CAAC-5.
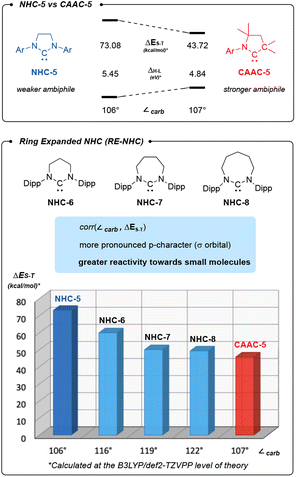 |
| Scheme 1
CAAC-5 is more ambiphilic than NHC-5. NHC ambiphilicity is improved in ring-expanded NHC (ReNHCs) as shown by their decreasing singlet–triplet gap (ΔES–T). | |
To compare experimentally the ambiphilicity of NHCs with that of CAAC-5, we first considered the activation of sp-hybridized CH bonds which has been reported with CAACs,17 but seldomly described with NHCs (one example has been reported using acetylene gas).18 We first investigated the reaction of p-tolylacetylene [pKa (DMSO) = 28.8 vs. 25 for acetylene] with NHC-5 at room temperature in benzene solution (Scheme 2). In this case, no reaction was observed within 1 hour. In marked contrast, using CAAC-5 the oxidative addition product 1a was quantitatively obtained within minutes. Under the same conditions a rapid and clean reaction was also observed with NHC-613aNHC-715 and NHC-813c giving adducts 1b–d as shown by characteristic 1H NMR signals at 6.04, 5.87 and 5.64 ppm, and 13C NMR signals at 72.3, 73.9 and 77.1 ppm, respectively. The structure of adduct 1c (from NHC-7) was confirmed by X-ray crystallography. Because of the significant difference in reactivity observed between NHC-5 and the RE-NHCs, we re-evaluated the reaction of NHC-5 with p-tolylacetylene and observed very slow conversion to adduct 1e upon performing the reaction at 80 °C for 4 hours.
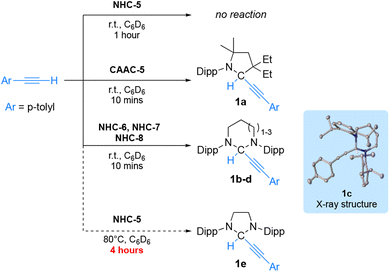 |
| Scheme 2 Reactivity of NHC-5–8 and CAAC-5 with p-tolylacetylene. | |
These initial results prompted us to search for more challenging molecules to activate. Examples of stable carbenes reacting with isonitriles to afford ketenimines are scarce.
They only include the anti-Bredt NHC19 and diamidocarbenes (DAC) 20 thanks to their enhanced electrophilicity resulting from reduced donation of the nitrogen lone-pair into the empty p-type orbital of the carbene carbon. Curious to probe the reactivity of RE-NHCs, we considered their reactivity and that of NHC-5 or CAAC-5 with adamantyl isocyanide (Scheme 3). CAAC-5 cleanly afforded the ketenimine 2a within minutes, while no reaction occurred with NHC-5 after 12 hours at room temperature in benzene solution.21 This result contrasts with CAAC-5 which cleanly afforded the ketenimine 2a within minutes. With NHC-6, no reaction was observed even after 12 hours. However, with NHC-7 and NHC-8, the quantitative formation of compound 2b and 2c, was observed after 10 minutes, as evidenced by the diagnostic 13C NMR signal for the central carbon of ketenimines at 216.9 ppm and 211.5 ppm, respectively. We confirmed the structure of compound 2b by X-ray crystallography. Interestingly, the solid-state structure of 2b revealed a pronounced bent geometry (CNHC–C–N angle: 158.5°) compared to that of diamido cyclohexylketenimine (CDAC–C–N angle: 173.8°)20b with a longer CNHC–Cket bond (133.8 pm vs. 129.7 pm for DAC). This observation indicates that NHC-7 is less electrophilic than DAC.
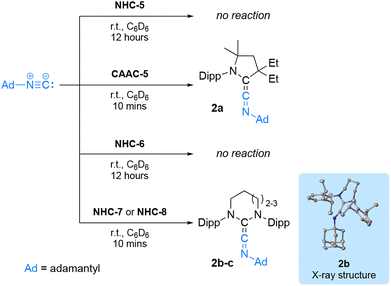 |
| Scheme 3 Reactivity of NHC-5–8 and CAAC-5 with adamantyl isocyanide. | |
Collectively, the reactions with terminal alkynes and isocyanides suggest that the ambiphilicity of the carbenes is in the order NHC-5 < NHC-6 < NHC-7 < NHC-8 < CAAC-5, which is in agreement with their singlet–triplet gap. To deconvolute these results further, we wondered if RE-NHCs, notwithstanding their lower electrophilicity could compare with CAAC-5 in the activation of ammonia.5 Under 2 atmospheres of NH3, no reaction occurred with NHC-5, which was expected since several diaminocarbenes have even been generated in liquid ammonia.22 (Scheme 4). In agreement with literature precedent,5 under the same conditions, CAAC-5 rapidly led to the ammonia adduct 3a. Switching to RE-NHCs, no reaction was observed with NHC-6 despite prolonged reaction time, while NHC-7 led to the clean formation of product 3b with distinctive 1H and 13C NMR signals at δ = 5.25 ppm and 85.8 ppm, respectively. This result was confirmed by single crystal X-ray diffraction. However, to our surprise, no reaction was observed with NHC-8.
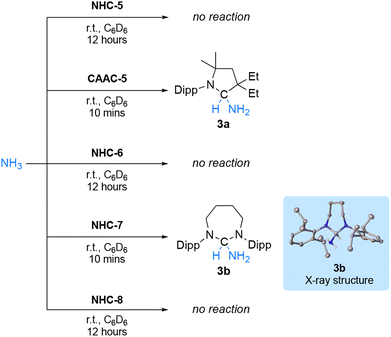 |
| Scheme 4 Reactivity of NHC-5–8 and CAAC-5 with ammonia. | |
We previously reported that the steric environment of CAAC-5 is a determining factor in controlling the reversibility of E–H bond activation (E = N–H, P–H).8 Compared to CAAC-5 (∠carb = 106°), NHC-8 is more sterically constrained around the carbene carbon due to its large N–CNHC–N bond angle (∠carb = 122°).13c We hypothesized this could explain its lack of reactivity with ammonia despite favourable electronics. To probe this hypothesis, we prepared the N-Mesityl (-Mes) substituted NHC-8 (MesNHC-8) since its steric profile is significantly smaller than that of the Dipp-substituted NHC-8. This is apparent from the solid state structures, when considering the steric maps (see ESI for details†) and percent buried volumes (%Vbur23) around the carbene carbon. NHC-8 (80.1%) compared to MesNHC-8 (77.2%) which is closer to that of NHC-7 (78.4.%). The larger steric hindrance is also apparent in solution when considering the unusual 77Se NMR downfield shift of the NHC-8-Se adduct 4a (571.1 ppm) compared to MesNHC-8-Se adduct 4b (437.9 ppm) (Scheme 5). Indeed, 77Se NMR is a spectroscopic marker for highlighting non-classical bonding (NCB) interactions between pendant N-Dipp substituents and the selenium atom.24 Note that when comparing the reactivity of N-tolyl and N-Dipp 8-membered NHCs with silver chloride, Cavell and co-workers discovered that in very large ring NHCs the steric environment provided by N-Dipp substituents can become so overwhelming that it prevents coordination.13c
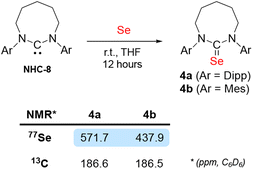 |
| Scheme 5 Selenium adducts of Dipp- and Mes-substituted NHC-8. | |
Having confirmed that MesNHC-8 is less sterically hindered than NHC-8 and even NHC-7, we evaluated its reactivity towards ammonia. Gratifyingly, rapid formation of the corresponding ammonia adduct was observed when performing the reaction in C6D6 under 2 atmospheres of NH3 (Scheme 6). To confirm these results, we also investigated the reactivity of the corresponding imidazolium salts with sodium amide which provided the expected adducts via nucleophilic addition of NH2− (Scheme 7). Note that under these conditions, reaction of NHC-8HBr with NaNH2 afforded the free NHC-8 and ammonia. Overall, these results suggest that for 8-membered ring NHCs, the activation of ammonia is controlled by steric parameters and possibly reversible.
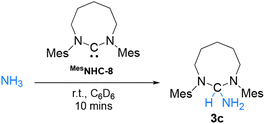 |
| Scheme 6 Reactivity of MesNHC-8 with ammonia. | |
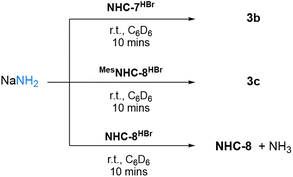 |
| Scheme 7 Reactivity of NHC-7, MesNHC-8 and NHC-8 conjugate acid salts with NaNH2. | |
Conclusions
Imidazole-2-ylidenes9 and imidazolin-2-ylidenes,10 the popular class of singlet carbenes introduced by Arduengo, have not proven to be ambiphilic owing to the two σ-withdrawing, π-donating amino groups stabilizing the carbene centre. However, our experimental data demonstrate that ring-expanded N-heterocyclic carbenes, NHC-7s and NHC-8s, belong to the class of ambiphilic carbenes. Our results also show that the steric environment in RE-NHCs can become a determining factor for controlling the E–H bond activation. We anticipate these results will have far reaching implications in the design and applications of large ring singlet carbene skeletons.
Data availability
All the data available has been provided in the ESI.†
Author contributions
F. V. and R. J. conceptualized this work. F. V., V. T. W. and M. A. performed the synthetic work. R. J. performed X-ray diffraction analysis. The manuscript was written by R. J. and G. B. and reviewed by all the authors. R. J. and G. B. guided the project.
Conflicts of interest
There are no conflicts to declare.
Acknowledgements
This work was supported by the U.S. Department of Energy, Office of Science, Basic Energy Sciences, Catalysis Science Program, under Award # DE-SC0009376, and the Agence Nationale de la Recherche (ANR-20-CE07-0000-cResolu).
References
-
(a) P. P. Power, Nature, 2010, 463, 171–177 CrossRef CAS PubMed;
(b) T. Chu and G. I. Nikonov, Chem. Rev., 2018, 118, 3608–3680 CrossRef CAS PubMed;
(c) D. Martin, M. Soleilhavoup and G. Bertrand, Chem. Sci., 2011, 2, 389–399 RSC.
- V. Lavallo, Y. Canac, C. Präsang, B. Donnadieu and G. Bertrand, Angew. Chem., Int. Ed., 2005, 44, 5705–5709 CrossRef CAS PubMed.
- Selected reviews:
(a) M. Melaimi, M. Soleilhavoup and G. Bertrand, Angew. Chem., Int. Ed., 2010, 49, 8810–8849 CrossRef CAS PubMed;
(b) M. Soleilhavoup and G. Bertrand, Acc. Chem. Res., 2015, 48, 256–266 CrossRef CAS PubMed;
(c) M. Melaimi, R. Jazzar, M. Soleilhavoup and G. Bertrand, Angew. Chem., Int. Ed., 2017, 56, 10046–10068 CrossRef CAS PubMed;
(d) U. S. D. Paul and U. Radius, Eur. J. Inorg. Chem., 2017, 2017, 3362–3375 CrossRef CAS;
(e) S. Kundu, S. Sinhababu, V. Chandrasekhar and H. W. Roesky, Chem. Sci., 2019, 10, 4727–4741 RSC;
(f) R. Jazzar, M. Soleilhavoup and G. Bertrand, Chem. Rev., 2020, 120, 4141–4168 CrossRef CAS PubMed;
(g) R. K. Singh, T. Khan, S. Misra and A. K. Singh, J. Organomet. Chem., 2021, 956, 122133 CrossRef CAS.
- V. Lavallo, Y. Canac, B. Donnadieu, W. W. Schoeller and G. Bertrand, Angew. Chem., Int. Ed., 2006, 45, 3488–3491 CrossRef CAS PubMed.
- G. D. Frey, V. Lavallo, B. Donnadieu, W. W. Schoeller and G. Bertrand, Science, 2007, 316, 439–441 CrossRef CAS PubMed.
- J. D. Masuda, W. W. Schoeller, B. Donnadieu and G. Bertrand, Angew. Chem., Int. Ed., 2007, 46, 7052–7055 CrossRef CAS PubMed.
-
(a) G. D. Frey, J. D. Masuda, B. Donnadieu and G. Bertrand, Angew. Chem., Int. Ed., 2010, 49, 9444–9447 CrossRef CAS PubMed;
(b) A. V. Zhukhovitskiy, M. G. Mavros, K. T. Queeney, T. Wu, T. Van Voorhis and J. A. Johnson, J. Am. Chem. Soc., 2016, 138, 8639–8652 CrossRef CAS PubMed;
(c) S. Würtemberger-Pietsch, H. Schneider, T. B. Marder and U. Radius, Chem.–Eur. J., 2016, 22, 13032–13036 CrossRef PubMed;
(d) A. Bakker, M. Freitag, E. Kolodzeiski, P. Bellotti, A. Timmer, J. Ren, B. Schulze Lammers, D. Moock, H. W. Roesky, H. Mönig, S. Amirjalayer, H. Fuchs and F. Glorius, Angew. Chem., Int. Ed., 2020, 59, 13643–13646 CrossRef CAS PubMed;
(e) G. Kaur, R. L. Thimes, J. P. Camden and D. M. Jenkins, Chem. Commun., 2022, 58, 13188–13197 RSC;
(f) A. V. Zhukhovitskiy, M. G. Mavros, K. T. Queeney, T. Wu, T. V. Voorhis and J. A. Johnson, J. Am. Chem. Soc., 2016, 138, 8639–8652 CrossRef CAS PubMed;
(g) C. A. Smith, M. R. Narouz, P. A. Lummis, I. Singh, A. Nazemi, C.-H. Li and C. M. Crudden, Chem. Rev., 2019, 119, 4986–5056 CrossRef CAS PubMed.
-
(a) D. Tolentino, S. Neale, C. Isaac, S. Macgregor, M. Whittlesey, R. Jazzar and G. Bertrand, J. Am. Chem. Soc., 2019, 141, 9823–9826 CrossRef CAS PubMed;
(b) J. Peltier, E. Tomás-Mendivil, D. Tolentino, M. Hansmann, R. Jazzar and G. Bertrand, J. Am. Chem. Soc., 2020, 142, 18336–18340 CrossRef CAS PubMed.
- A. J. Arduengo, R. L. Harlow and M. Kline, J. Am. Chem. Soc., 1991, 113, 361–363 CrossRef CAS.
- A. J. Arduengo, J. R. Goerlich and W. J. Marshall, J. Am. Chem. Soc., 1995, 117, 11027–11028 CrossRef CAS.
- D. A. Dixon, A. J. Arduengo III, K. D. Dobbs and D. V. Khasnis, Tetrahedron Lett., 1995, 36, 645–648 CrossRef CAS.
- C. Goedecke, M. Leibold, U. Siemeling and G. Frenking, J. Am. Chem. Soc., 2011, 133, 3557 CrossRef CAS PubMed.
-
(a) M. Iglesias, D. J. Beetstra, J. C. Knight, L.-L. Ooi, A. Stasch, S. Coles, L. Male, M. B. Hursthouse, K. J. Cavell, A. Dervisi and I. A. Fallis, Organometallics, 2008, 27, 3279–3289 CrossRef CAS;
(b) E. L. Kolychev, I. A. Portnyagin, V. V. Shuntikov, V. N. Khrustalev and M. S. Nechaev, J. Organomet. Chem., 2009, 694, 2454–2462 CrossRef CAS;
(c) W. Y. Lu, K. J. Cavell, J. S. Wixey and B. Kariuki, Organometallics, 2011, 30, 5649–5655 CrossRef CAS.
- J. J. Dunsford, D. S. Tromp, K. J. Cavell, C. J. Elsevier and B. M. Kariuki, Dalton Trans., 2013, 42, 7318–7329 RSC.
-
(a) M. J. Page, W. Y. Lu, R. C. Poulten, E. Carter, E. A. G. Algarra, B. M. Kariuki, S. A. Macgregor, M. F. Mahon, K. J. Cavell, D. M. Murphy and M. K. Whittlesey, Chem.–Eur. J., 2013, 19, 2158–2167 CrossRef CAS PubMed;
(b) A. Cervantes-Reyes, F. Rominger, M. Rudolph and A. S. K. Hashmi, Adv. Synth. Catal., 2020, 362, 2523–2533 CrossRef CAS;
(c) J. W. Hall, D. Bouchet, M. F. Mahon, M. K. Whittlesey and C. S. J. Cazin, Organometallics, 2021, 40, 1252–1261 CrossRef CAS.
-
(a) M. Eck, S. Würtemberger-Pietsch, A. Eichhorn, J. H. J. Berthel, R. Bertermann, U. S. D. Paul, H. Schneider, A. Friedrich, C. Kleeberg, U. Radius and T. B. Marder, Dalton Trans., 2017, 46, 3661–3680 RSC;
(b) L. García, K. H. M. Al Furaiji, D. J. D. Wilson, J. L. Dutton, M. S. Hill and M. F. Mahon, Dalton Trans., 2017, 46, 12015–12018 RSC;
(c) G. Kundu, R. Dixit, S. Tothadi, K. Vanka and S. S. Sen, Dalton Trans., 2022, 51, 14452–14457 RSC;
(d) K. Balayan, H. Sharma, K. Vanka, S. Ravindranathan, R. G. Gonnade and S. S. Sen, Chem. Commun., 2023, 59, 8540–8543 RSC.
- Z. R. Turner, Chem.–Eur. J., 2016, 22, 11461–11468 CrossRef CAS PubMed.
- A. J. Arduengo III, J. C. Calabrese, F. Davidson, H. V. Rasika Dias, J. R. Goerlich, R. Krafczyk, W. J. Marshall, M. Tamm and R. Schmutzler, Helv. Chim. Acta, 1999, 82, 2348–2364 CrossRef.
- D. Martin, N. Lassauque, B. Donnadieu and G. Bertrand, Angew. Chem., Int. Ed., 2012, 51, 6172–6175 CrossRef CAS PubMed.
-
(a) M. Braun, W. Frank, G. J. Reiss and C. Ganter, Organometallics, 2010, 29, 4418–4420 CrossRef CAS;
(b) T. W. Hudnall and C. W. Bielawski, J. Am. Chem. Soc., 2009, 131, 16039–16041 CrossRef CAS PubMed;
(c) V. César, S. Labat, K. Miqueu, J.-M. Sotiropoulos, R. Brousses, N. Lugan and G. Lavigne, Chem.–Eur. J., 2013, 19, 17113–17124 CrossRef PubMed.
- An alternative reactivity has been reported: Y. Kim, L. L. Liu and D. W. Stephan, Chem.–Eur. J., 2019, 25, 7110–7113 CrossRef CAS PubMed.
- W. A. Herrmann, L. J. Gooβen and M. Spiegler, J. Organomet. Chem., 1997, 547, 357–366 CrossRef CAS.
- L. Falivene, Z. Cao, A. Petta, L. Serra, A. Poater, R. Oliva, V. Scarano and L. Cavallo, Nat. Chem., 2019, 11, 872–879 CrossRef CAS PubMed.
-
(a) A. Liske, K. Verlinden, H. Buhl, K. Schaper and C. Ganter, Organometallics, 2013, 32, 5269–5272 CrossRef CAS;
(b) G. P. Junor, J. Lorkowski, C. M. Weinstein, R. Jazzar, C. Pietraszuk and G. Bertrand, Angew. Chem., Int. Ed., 2020, 59, 22028–22033 CrossRef CAS PubMed.
|
This journal is © The Royal Society of Chemistry 2024 |
Click here to see how this site uses Cookies. View our privacy policy here.